Abstract
Fusarium head blight (FHB), caused primarily by Fusarium graminearum, has rapidly become the most devastating wheat disease worldwide. Host resistance is the most effective and sustainable strategy to combat the disease. To date, more than 50 unique quantitative trait loci (QTLs) have been identified in diverse wheat populations, and Fhb1 consistently shows the highest level of resistance in different genetic backgrounds. In spite of worldwide efforts to deploy Fhb1 through wheat breeding, only a few cultivars currently used in commercial production carry Fhb1. Recently, Fhb1 has been cloned and diagnostic markers have been developed, which will facilitate successful deployment of Fhb1 in wheat breeding. Using marker-assisted backcross, Fhb1 has been successfully transferred into locally adapted cultivars that carry minor QTLs, and selected Fhb1 lines in different backgrounds showed high levels of FHB resistance. This approach may provide a quick solution to improvement of FHB resistance in commercial cultivars. In addition, gene editing that knocks down the susceptible allele of Fhb1 in commercial cultivars may also improve wheat FHB resistance. Most other QTLs identified to date show much smaller effects than Fhb1. These QTLs are distributed in many locally adapted cultivars, and thus are important QTLs for FHB resistance improvement. Currently, diagnostic markers are still not available for most of these minor QTLs; therefore, genomic selection may improve selection accuracy for these QTLs to create highly FHB-resistant cultivars.
Résumé: La brûlure de l’épi causée par le fusarium (BEF), engendrée principalement par Fusarium graminearum, est rapidement devenue la maladie du blé la plus dévastatrice au monde. La résistance de l’hôte est la stratégie la plus efficace et la plus durable pour la combattre. À ce jour, plus de 50 locus à caractère quantitatif (QTL) ont été répertoriés dans différentes populations de blé, et le gène Fhb1 affiche invariablement le plus haut taux de résistance des différents fonds génétiques. En dépit des efforts déployés partout dans le monde pour utiliser le gène Fhb1 en vue d’améliorer le blé, seuls quelques cultivars commerciaux utilisés couramment le portent. Récemment, le gène Fhb1 a été cloné et des marqueurs de diagnostic ont été développés pour en faciliter l’utilisation efficace afin d’améliorer le blé. En recourant au rétrocroisement assisté par marqueurs, le gène Fhb1 a été transféré avec succès dans des cultivars locaux adaptés, porteurs de QTL de moindre importance, et des lignées Fbh1 choisies dans différents fonds ont affiché un taux élevé de résistance à la BEF. Cette approche peut se révéler une solution rapide pour ce qui est d’améliorer la résistance à la BEF des cultivars commerciaux. De plus, la manipulation génétique qui invalide l’allèle réceptif du gène Fbh1 chez les cultivars commerciaux peut aussi contribuer à améliorer la résistance du blé à la BEF. La majorité des autres QTL identifiés à ce jour affiche des effets beaucoup plus modestes que le gène Fbh1. Ces QTL sont répartis dans plusieurs cultivars locaux adaptés et, par conséquent, sont essentiels à l’amélioration de la résistance à la BEF. Actuellement, les marqueurs de diagnostics ne sont pas encore disponibles pour la majorité de ces QTL, par conséquent, la sélection génomique peut contribuer à améliorer la précision du choix à l’égard de ces QTL afin de créer des cultivars hautement résistants à la BEF.
Introduction
Fusarium head blight (FHB), also called scab, is a damaging disease of cereal crops worldwide (Bai & Shaner, Citation2004). It is primarily incited by Fusarium graminearum Schwabe but other Fusarium species have also been reported as pathogens (Buerstmayr et al., Citation2009). FHB can cause significant reductions not only in grain yield but also in grain quality (Bai & Shaner, Citation2004). The pathogen produces mycotoxins during infection, in particular deoxynivalenol (DON). DON-contaminated grain is harmful to the health of human beings and livestock after consumption (Jiang et al., Citation2007a; Ferrigo et al., Citation2016). In the USA, Canada, Europe and several other countries, governments have imposed strict upper limits on the level of DON allowed in wheat grain and grain products used for food and feed (Buerstmayr et al., Citation2009; Ferrigo et al., Citation2016).
Epidemics of FHB were first reported in 1884 in England where it was called ‘wheat scab’ (Dubin et al., Citation1997). The disease has spread to other major wheat growing areas worldwide and has been considered a major threat to wheat production in many countries since the early 20th century (Bai & Shaner, Citation1994; Goswami & Kistler, Citation2004). In the USA, Arthur (Citation1891) first reported a FHB epidemic in Indiana. FHB epidemics have spread to all major wheat production states in the last several decades (De Wolf et al., Citation2003; McMullen et al., Citation2012). The economic losses in wheat and barley caused by FHB were estimated at about $7.67 billion from 1993 to 2001 for nine major FHB epidemic states in the northern Great Plains and the central USA (Illinois, Indiana, Kentucky, Michigan, Missouri, Minnesota, Ohio, South Dakota and North Dakota) (Nganje et al., Citation2004). In Kansas, FHB losses were valued at $57 million in 2008 alone (McMullen et al., Citation2012).
FHB epidemics have been reported in most of the wheat production areas worldwide (Dubin et al., Citation1997; Bai & Shaner, Citation2004; Buerstmayr et al., Citation2009; McMullen et al., Citation2012). Recent changes in climate and cropping systems have made FHB epidemics more frequent and severe, even in the regions where FHB has not been previously reported, such as in Oklahoma, Montana and Idaho in the USA and the Yellow and Huai River Valleys in China. Increased acreages of maize in these regions might contribute to expanded epidemics by providing rich sources of inoculum for initial infection (Gilbert & Haber, Citation2013). Applying fungicides to control FHB remains challenging because of the short time window for application, potential environmental contamination and increased cost of wheat production (McMullen et al., Citation2012). Growing resistant cultivars is the most effective and environment-friendly approach to minimizing the damage caused by the disease (Bai & Shaner, Citation2004; McMullen et al., Citation2012).
FHB resistance is a quantitative trait that is controlled by multiple quantitative trait loci (QTLs). Among more than 50 unique QTLs reported to date (Liu et al., Citation2009), Fhb1 was the first QTL reported from Chinese cultivars ‘Sumai3’ and ‘Ning7840’ (Bai et al., Citation1999; Waldron et al., Citation1999; Zhou et al., Citation2003; Cuthbert et al., Citation2006). Since then, several reviews have been published to summarize these QTLs from different sources (Buerstmayr et al., Citation2009; Liu et al., Citation2009; Loffler et al., 2009). The aim of this review is to provide a recent update on wheat FHB resistance with emphasis on several major FHB resistance QTLs and their potential application in FHB control in wheat.
Wheat responses to FHB infection
FHB infection in wheat spikes is a complicated process. Plant morphological and developmental factors and their growing environments all affect FHB infection. Plant height, spikelet density, awn character, flowering date, degree of floret opening during flowering, and grain filling rate have all been related to FHB resistance (Rudd et al., Citation2001; Ban, Citation2003). For example, wheat susceptibility decreases from early flowering to later grain filling, and thus genotypes with quick grain filling may have better resistance than these of slow grain filling genotypes. The Rht-D1b semi-dwarf allele in European wheat cultivars may have pleiotropic effects on FHB susceptibility; thus, tall plants may show better resistance than short ones (Holzapfel et al., Citation2008). Those morphological and developmental traits most likely help plants escape initial infection to provide passive resistance, whereas physiological resistance that produces chemical barriers through biochemical pathways to prohibit pathogen penetration to other tissues and growth in plants after initial infection might play a more important role in wheat defence against FHB (Pritsch et al., Citation2000; Browne & Brindle, Citation2007; Li & Yen, Citation2008; Ding et al., Citation2011; Zhang et al., Citation2013).
Based on host response to pathogen infection, Schroeder & Christensen (Citation1963) first observed two types of resistance: resistance to initial pathogen penetration (Type I) and resistance to spread of FHB symptoms within a spike (Type II). Later, Miller et al. (Citation1985) added low mycotoxin accumulation as Type III resistance. These three types of resistance are widely accepted (Bai & Shaner, Citation1994); however, only type II resistance has been extensively characterized and used in breeding programmes because it is the most stable type of resistance and easiest to be assessed in wheat compared with other types (Bai & Shaner, Citation2004). Type II resistance is usually evaluated by single floret (spikelet) inoculation that injects inoculum into a central spikelet of a spike in greenhouse experiments or grain-spawn inoculation in the field (Bai & Shaner, Citation1994). Percentage of symptomatic spikelets in a spike (PSS) is used to measure the disease severity (Bai & Shaner, Citation1994). However, accurate assessment of type I resistance is more difficult than type II resistance. Usually plants are inoculated by spraying conidiospores over spikes or grain-spawn inoculation to provide natural infection. Infection rate is measured by percentage of initially infected spikelets in a spike or FHB incidence (percentage of initially infected spike in a plot) for type I resistance (Bai & Shaner, Citation1994). Many factors may affect type I resistance, including inoculation methods (spraying vs. grain-spawn inoculation) and time and inoculum concentration if spraying.
Degrees of FHB spread within an infected spike vary with levels of host resistance. The pathogen can successfully establish initial infection in any wheat genotype when it is point-inoculated by placing spores into one floret of a spike (Bai & Shaner, Citation1994). In a highly resistant genotype, FHB infection is usually confined in the inoculated spikelet or floret and does not spread to neighbouring spikelets, whereas in a highly susceptible genotype, FHB symptoms spread to uninoculated neighbouring spikelets until the entire spike is bleached (Fig. 1a), designated type II resistance (Schroeder & Christensen, Citation1963). However, in moderately resistant to susceptible genotypes, FHB symptoms start spreading upward and downward to neighbouring spikelets about 4–5 days after needle inoculation. The number of spikelets to which FHB can spread varies with host genotypes and environmental conditions where plants are grown. In moderately resistant genotypes, many spikelets in an inoculated spike remain uninfected, whereas in highly susceptible genotypes, the entire inoculated spike is bleached within 7–10 days after inoculation (Ribichich et al., Citation2000). Therefore, FHB severity score (PSS) evaluates type II resistance under greenhouse conditions. In the field, initial infection may occur in multiple spikelets of a single spike, and the genotypes with fewer initially infected spikelets in a spike have higher type I resistance (). Under field conditions, final PSS ratings usually reflect both type I and type II resistance of a genotype () (Burt et al., Citation2015).
Fig. 1 (Colour online) a, Wheat type II resistance. Wheat plant with a high level of type II resistance (right head) usually confines FHB infection to the inoculated spikelet or floret and does not spread to neighbouring spikelets; whereas in a highly susceptible genotype (left head), FHB symptoms spread from the inoculated spikelet to uninoculated neighbouring spikelets until the entire spike is bleached. Both genotypes were inoculated by injecting fusarium spores into a central spikelet of a spike under greenhouse conditions. b, Wheat type I resistance. Under field conditions, the FHB nursery was inoculated by scattering Fusarium infected corn kernels to induce natural infection. The plants with type I resistance show only one or a few infected spikelets (right head), whereas the type I susceptible plant shows multiple independently infected spikelets in a spike (left head). c, Comparison between type I and type II resistance under field conditions. Plant with good type II resistance has lower final disease severity even when multiple florets are infected under heavy disease pressure (left head); whereas plant without type II resistance has entire spike bleached even if only a single spikelet was initially infected (right head).
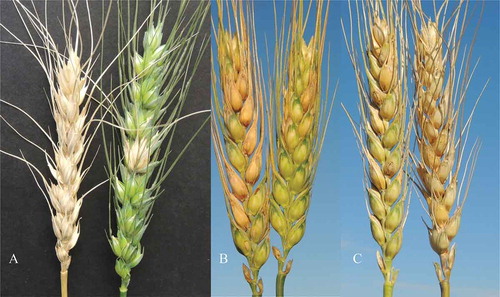
Type III resistance is important to grain end-use quality (Rudd et al., Citation2001), but it is highly correlated with type I and type II resistance (Paul et al., Citation2006); therefore, PSS can be used to predict DON contents in harvested wheat grains (Bai et al., Citation2001). Besides, accuracy of type III resistance assessment is significantly affected by threshing method used. In most cases, Fusarium damaged kernels (FDK) that contain a high level of DON can be blown out during threshing (Mesterhazy et al., Citation1999; Bai & Shaner, Citation2004); thus the DON measurement may not reflect actual content of DON produced in the lines tested. In addition, low FDK and FHB tolerance were described as two additional types of resistance (Mesterhazy, Citation1995). However, FDK is highly correlated with FHB severity, and may not be an independent type of resistance (Bai et al., Citation2001). For FHB tolerance, an evaluation method is not available; therefore, it has not been pursued further.
Biochemical mechanisms for FHB resistance have been frequently reported, but the conclusions remain equivocal. Resistant wheat plants may either produce physical barriers (such as a thickened cell wall) or accumulate toxic phenolic compounds and triticens to prevent or delay the mycelial growth after initial infection (Ribichich et al., Citation2000; Zhang et al., Citation2013). Fusarium graminearum may induce defence responsive genes that encode defence-related proteins, including PR-1, PR-2 (β-1,3-glucanase), PR-3 (chitinase), PR-4 and PR-5 (thaumatin-like protein) during early infection in wheat spikes (Pritsch et al., Citation2000). Some of the proteins are differentially expressed between resistant and susceptible genotypes (Zhang et al., Citation2013), but other studies observed that Fusarium inoculation induced biosynthesis of more jasmonic acid (JA) and higher expression of lipoxygenase (LOX2) and chalcone synthase in resistant wheat plants than in susceptible plants (Li & Yen, Citation2008; Ding et al., Citation2011). Ethylene (ET) production was also higher in resistant plants than in susceptible plants, which can cause plant organ senescence, cell wall degradation and finally cell death (Li & Yen, Citation2008). In addition, many other biochemical compounds, including choline, betaine, and amino acids glutamine, glutamate alanine, trans-aconitate and sucrose have also been associated with fungal hyphal growth (Browne & Brindle, Citation2007).
FHB resistance sources in wheat
Germplasm sources with various levels of resistance have been reported worldwide, although those with immunity to FHB have not been found (He et al., Citation2013). Among these FHB resistant germplasm lines, only a few showed a high level of FHB resistance, which are mostly found in China and Japan (Bai & Shaner, Citation2004; Yu et al., Citation2008a). In the 1980s, the Chinese Wheat Scab Cooperative Project identified 1765 accessions with various levels of resistance after screening 34 571 wheat landraces and other germplasm materials (Bai & Shaner, Citation1994; He et al., Citation2013). Among them, ‘Sumai3’ and its derivatives are improved cultivars with high FHB resistance and have received extensive attention by breeders and geneticists worldwide (Bai & Shaner, Citation1994; Rudd et al., Citation2001; Liu et al., Citation2009). A major QTL for FHB resistance on chromosome arm 3BS was first reported from ‘Sumai3’ and ‘Ning7840’ (Bai et al., Citation1999; Waldron et al., Citation1999). Later, the QTL, designated as Fhb1 (Cuthbert et al., Citation2006), was reported in landraces ‘Wangshuibai’, ‘Baishanyuehuang’, ‘Huangcandou’, ‘Huangfangzhu’ from China and ‘Nyu Bai’ from Japan (Ban, Citation2001; Bai & Shaner, Citation2004; Jia et al., Citation2006; Lin et al., Citation2006; Yu et al., Citation2008b). However, direct use of these landraces or cultivars as resistant parents in conventional breeding has not been successful due to their poor agronomic traits.
Many locally adapted cultivars may also carry some minor QTLs for FHB resistance (Zhang et al., Citation2012a, Citation2012b). These minor QTLs individually show much smaller effects than Fhb1 from ‘Sumai3’, but they may show additive effects when combined (Cai, Citation2016; Li et al., Citation2016). These cultivars have good adaptation and desirable agronomic traits, and thus can be easily included in elite breeding lines without penalty of yield and other agronomic traits. The examples of these cultivars include ‘Chokwang’ from Korea (Yang et al., Citation2005a), ‘Frontana’ (Mardi et al., Citation2006) and ‘Encruzilhada’ from Brazil (Mesterhazy, Citation1995; Ban, Citation2001), ‘Ernie’, ‘Freedom’ and ‘Roane’ from the USA (Rudd et al., Citation2001; Liu et al., Citation2005, Citation2007; Jin et al., Citation2013), and ‘Arina’, ‘Renan’ and ‘Fundulea 201R’ from Europe (Gervais et al., Citation2003; Paillard et al., Citation2004; Somers et al., Citation2004; Steiner et al., Citation2004). These sources may carry some different QTLs from those present in Asian sources (Jin et al., Citation2013) and can be used to pyramid FHB resistance QTLs from Asian sources.
FHB resistance was also reported in alien species, including tetraploid wheat species, such as Ae. ventricosa, Ae. speltoides, Thinopyrum ponticum, Th. elongatum, Th. intermedium, Dasypyrum villosum, Leymous racemosus and Elymus tsukushiensis (Oliver et al., Citation2005; Cai et al., Citation2008; Qi et al., Citation2008; Cainong et al., Citation2015). Various cytogenetic approaches have been used to transfer resistance genes from these alien sources to generate addition, substitution, translocation or recombinant lines by backcrossing them with adapted common wheat. Three alien FHB resistant fragments, designated as Fhb3, Fhb6 and Fhb7, have been successfully transferred into wheat from alien species Leymus racemosus (Qi et al., Citation2008), 1Ets#1S of Elymus tsukushiensis (Cainong et al., Citation2015), and Thinopyrum ponticum (Guo et al., Citation2015), respectively, and selected lines were reported to show a high level of resistance in wheat backgrounds. Among them, Fhb3 has been used to pyramid with Fhb1 by marker-assisted backcross. Evaluation of FHB resistance of BC2F4 progenies indicated that Fhb3 did not reduce FHB severity in both greenhouse and field experiments, either alone or together with Fhb1 (Fatima, Citation2016). Therefore, the effects of these alien genes on FHB resistance need to be evaluated in different backgrounds before they can be deployed in breeding.
QTLs for FHB resistance
FHB resistance is a quantitative trait and controlled by several QTLs (Bai & Shaner, Citation2004). To date, more than 100 QTLs for different types of resistance have been reported from different studies (Buerstmayr et al., Citation2009). Further comparisons of the chromosome locations of these QTLs from different studies narrowed the list down to about 50 QTLs with unique chromosome locations (Liu et al., Citation2009). These unique QTLs have been reported in at least two populations, in particular those on chromosomes 3A, 3B, 4B, 5A and 6B that were reported in several populations of different geographic origins (Buerstmayr et al., Citation2009; Liu et al., Citation2009). Seven QTLs have been formally assigned with a gene name. Fhb1 on chromosome arm 3BS from ‘Sumai3’ was named first (Cuthbert et al., Citation2006). Fhb2 on chromosome 6B from ‘Sumai3’ (Cuthbert et al., Citation2007), Fhb4 (Xue et al., Citation2010) and Fhb5 (Xue et al., Citation2011) on chromosomes 4B and 5A, respectively, from ‘Wangshuibai’. As mentioned above, Fhb3 on chromosome 7AS, Fhb6 on chromosome 1A and Fhb7 on chromsome 7D were all from alien species.
Among the seven named QTLs, Fhb1 shows the largest effect on FHB type II resistance among all QTLs identified to date and is the only QTL that shows stable type II resistance across multiple backgrounds (Bai et al., Citation1999; Waldron et al., Citation1999; Zhou et al., 2002; Buerstmayr et al., Citation2003; Bai & Shaner, Citation2004; Cuthbert et al., Citation2006; Yu et al., Citation2008b; Schweiger et al., Citation2016). This QTL has also been reported in many wheat landraces that are not related to ‘Sumai3’ (Somers et al., Citation2003; Cuthbert et al., Citation2006; Lin et al., Citation2006; Yu et al., Citation2008b; Li et al., Citation2012; Zhang et al., Citation2012b; Cai & Bai, Citation2014). We surveyed a worldwide collection of wheat landraces and cultivars and only found Fhb1 resistance alelle in landraces from east Asia (southern China and Jappan), not from other parts of the world; therefore, Fhb1 most likely originated from southern China.
Due to its major and stable effect on FHB type II resistance, Fhb1 has been extensively studied and utilized in wheat breeding programmes. It was first prioritized for map-based cloning (Rawat et al., Citation2016; Schweiger et al., Citation2016). Previous fine mapping identified Xumn10 as a tightly linked marker for marker-assisted selection (MAS) of Fhb1 (Liu et al., Citation2008). This marker has been sucessfully used as the near diagnostic marker of Fhb1 in US breeding programmes for about a decade. However, Xumn10 is not diagnostic in Chinese germplasm because many susceptible Chinese landraces and cultivars carry the ‘Sumai3’ allele at the marker locus. As more Chinese germplasm sources are used in US wheat breeding programmes, Xumn10 becomes less diagnostic for Fhb1 in these programmes. SNP markers such as SNP319 and SNP8 (Bernardo et al., Citation2011) have been developed as alternative markers for Fhb1, but false positive alleles were also reported for these markers. More recently, significant progress has been made in Fhb1 cloning. Schweiger et al. (Citation2016) sequenced a 1 Mb contig harbouring Fhb1 in ‘CM-8203’, a ‘Sumai3’ derivative, and found that Fhb1 is in a suppressed recombination region of 703 kb. Rawat et al. (Citation2016) further characterized one of the candidates in the region, a chimeric lectin with agglutinin domains and a pore-forming toxin-like domain (PFT), for Fhb1 and reported that the genotype with the resistance allele of Fhb1 carried the functional PFT, whereas the genotypes with susceptible allele had no PFT or a malfunctioned PFT in a set of wheat materials they used. However, when a large collection of wheat samples, especially Chinese landraces, was analysed for PFT, many susceptible genotypes were found to carry functional PFT, suggesting PFT may not be the determinant of Fhb1. We conducted map-based cloning and EcoTILLING of candidate genes in the Fhb1 region and found a hypothetical protein, designated TaHRC, that is responsible for Fhb1 resistance (Su et al., Citation2017). We found that the wild type allele of the gene confers FHB susceptibility, and a large deletion mutation in the open reading frame of the gene caused a loss-of-function of the gene and thus results in FHB resistance. We developed diagnostic markers to capture the deletion mutation for genotyping using either gel-based or Kompetitive allele specific PCR (KASP) protocols (Su et al., Citation2016). These markers are highly diagnostic for Fhb1 when they were analysed in a large worldwide wheat collection; therefore, they are ideal markers for MAS of Fhb1 in breeding.
For the other three named genes from wheat, Fhb2 on chromosome 6B explained a wide range of the phenotypic variation (4.4–23%) for mainly type II FHB resistance (Waldron et al., Citation1999; Anderson et al., Citation2001; Yang et al., Citation2005b; Semagn et al., Citation2007; Bonin & Kolb, Citation2009; Li et al., Citation2011, Citation2012), but type I and type III resistance was also reported (Szabó-Hevér et al., Citation2012; Ágnes et al., Citation2014). Fhb2 is located in the interval between Xgwm508 and Xbarc79 with Xgwm644 as a common marker for most populations (Liu et al., Citation2009). It is present not only in Chinese germplasm, but also in germplasm sources from different continents, including ‘Arina’ and ‘Apache’ from Europe, ‘Patton’ from the USA, and ‘DH181’ from Canada.
Fhb4 on chromosome 4B showed type I resistance in ‘Wangshuibai’ (Jia et al., Citation2006; Lin et al., Citation2006; Xue et al., Citation2010), and type II resistance in ‘Ernie’ (Liu et al., Citation2005, Citation2007), ‘Chokwang’ (Yang et al., Citation2005a), and ‘Wuhan1’ (Somers et al., Citation2003) etc., and explained 4.7% (Yang et al., Citation2005a) to 17.5% (Lin et al., Citation2006) of the phenotypic variation. It was located between Xbarc20 and Xwmc349 (Liu et al., Citation2009). Fhb4 is not only present in Chinese germplasm, but also in several US germplasm sources, including ‘Becker’ and ‘IL-95-1653ʹ (Liu et al., Citation2009).
Fhb5 has been associated with type I resistance (Lin et al., Citation2006), but it was also associated with type II resistance in several populations (Liu et al., Citation2009). Fhb5 is present in more than 10 populations including the resistant landraces/cultivars from China (‘Wangshuibai’), Japan (‘Nyu Bai’), Europe (‘F201R’) and America (‘Frontana’ and ‘Ernie’). It shows much smaller effects than Fhb1 on average but can explain up to 30% of the phenotypic variation in some studies (Buerstmayr et al., Citation2011, Citation2012). Based on the consensus maps, this QTL is located in a ~ 10 cM interval between Xgwm293 and Xbarc180 on chromosome 5A (Buerstmayr et al., Citation2009; Liu et al., Citation2009). Chu et al. (Citation2011) mapped Qfhb.rwg-5A.1 in PI277012 at the same chromosome position as Fhb5, showing a major effect on type II resistance. They also found another QTL, designated Qfhb.rwg-5A.2, on chromosome arm 5AL that showed an even larger effect on type II resistance than Qfhb.rwg-5A.1. The QTL peak for Qfhb.rwg-5A.2 is at Xcfd39, flanked by Xwmc470 and Xbarc48, and explained up to 32% of phenotypic variation for type II resistance. This QTL is in the same interval as the Q gene, but recombination was identified between Q and Qfhb.rwg-5A.2. In previous studies, QTLs in the vicinity of Qfhb.rwg-5A.2 have also been reported from several European cultivars, including ‘Renan’, ‘Apache’, ‘Pirat’ and ‘Arina’, but with a much smaller effect than in Qfhb.rwg-5A.2 (Gervais et al., Citation2003; Holzapfel et al., Citation2008; Buerstmayr et al., Citation2009). Currently, we are pyramiding Qfhb.rwg-5A.2 with Fhb1 to achieve highly FHB resistant germplasm lines for breeding or cultivars for production.
Besides seven named QTLs, QTL on 2DL also shows a major effect on type II resistance and was reported in several cultivars, including ‘Wuhan1’ (Somers et al., Citation2003), ‘Soru#1’ (He et al., Citation2016), ‘Kenyon’ (McCartney et al., Citation2016), ‘Sumai3’ (Suzuki et al., 2012; Yang et al., Citation2005b), and ‘CJ9306’ (Jiang et al., Citation2007a, Citation2007b). This QTL is located between Xgwm539 and Xwmc245 with Xgwm539 as a common marker among these mapping studies (Buerstmayr et al., Citation2009; Liu et al., Citation2009). It confers both type II and type III resistance and showed an additive effect with Fhb1 in different backgrounds (Clark et al., Citation2016; Jiang et al., Citation2007a; Citation2007b).
QTLs on 3AS have been detected in germplasm from several countries including ‘F201R’ from Europe (Shen et al., Citation2003), ‘Huapei57-2’ (Bourdoncle & Ohm, Citation2003), ‘Wangshuibai’ (Yu et al., Citation2008b) and several other landraces (Zhang et al., Citation2012b; Cai & Bai, Citation2014; Cai, Citation2016) from China, ‘Heyne’ from the USA (Zhang et al., Citation2012a), ‘DH181R’ (Yang et al., Citation2005b) and ‘Frontana’ from Brazil (Steiner et al., Citation2004). A QTL near centromere region of 3BS (3BSc) has been reported in ‘Baishanyuehuang’ (Zhang et al., Citation2012b), ‘Wangshuibai’ (Zhou et al., Citation2004) from China, ‘Nyu Bai’ from Japan (Somers et al., Citation2003), ‘DH181R’ (Yang et al., Citation2005b), and ‘Ernie’ from the USA (Liu et al., Citation2007). These QTLs with either a major effect or a minor effect detected in multiple genetic backgrounds can be good sources of resistance for gene pyramiding.
Breeding for FHB resistance
Many QTLs for FHB resistance show additive effects (Bai et al., Citation2001; Jiang et al., Citation2007a, Citation2007b), and pyramiding major QTLs from different sources to locally adapted cultivars may achieve a high level of resistance (Rudd et al., Citation2001). Fhb1 is the QTL showing the largest effect identified to date and usually shows additive effects when it is combined with other QTLs. Therefore, transferring Fhb1 into locally adapted moderately susceptible or moderately resistant cultivars may significantly improve FHB resistance in commercial wheat cultivars (Kolb et al., Citation2001). Direct use of Fhb1 from Chinese sources in US hard winter wheat breeding programmes has not been successful because they usually have many undesired agronomic traits. To remove these poor agronomic traits and reduce the linkage drag in Fhb1 carrying Chinese landraces, marker-assisted backcross was used to transfer Fhb1 into locally adapted backgrounds. Recently, the US Department of Agriculture Central Small Grain Genotyping Laboratory has transferred Fhb1 to 16 locally adapted hard winter wheat cultivars or breeding lines from five hard winter wheat states using marker-assisted backcross. Fhb1 usually reduces FHB severity by 20–50% in different genetic backgrounds compared with their recurrent parents. Significant variations in FHB severity were observed between Fhb1 lines within a population and across populations. The differences among Fhb1 lines might be due to segregation of other minor QTLs in Fhb1 lines. However, the minor QTLs in the backgrounds usually are unknown and markers for these QTLs are not available; therefore, phenotypic selection of Fhb1 lines is necessary for selecting highly resistant lines. Most of the selected Fhb1 lines showed moderate to high FHB resistance. These lines have been used in the hard winter wheat breeding programmes as Fhb1 donor parents (Jin et al., Citation2013, Citation2014). One of the selected lines, ‘OverlandFHB-10‘, has been promoted to the 2017 Northern Regional Performance Nursery for yield trails. Using marker-assisted backcrossing, Fhb1 has also been transferred into a durum wheat and shows a large major effect on type II resistance (Prat et al., Citation2017), which is an important achievement for durum breeding because QTL with high FHB resistance has not been found in durum to date. These selected Fhb1 lines with different genetic backgrounds are useful resources for breeders to deploy Fhb1 in wheat and durum.
Significant progress in using Fhb1 resistance has been made in US hard spring wheat (HSW) and soft winter wheat breeding programmes. Several hard spring wheat cultivars with Fhb1, including ‘Bacup’ and ‘Sabin’ from Minnesota, and ‘Alsen’, ‘ND2710’ and ‘Glenn’ from North Dakota, have been released for production (Mergoum et al., Citation2007; Anderson, Citation2012a; Anderson et al., Citation2012b). In this region, moderate FHB-resistant cultivars occupied about 50% of the total spring wheat acreage (Anderson, Citation2012a) and these Fhb1-derived cultivars have played a significant role in reducing FHB damage in US hard spring wheat. For soft winter wheat in the USA, some cultivars harbouring Fhb1 such as Pioneer Brands ‘25R18’, ‘25R42’ and ‘25R51’ have been released for commercial production (Brown-Guedira et al., Citation2008).
Besides Fhb1 from China, many cultivars from different countries may contain native resistance QTLs. Several moderately FHB resistant cultivars without Fhb1 have been released in US soft winter wheat (SWW) regions, including ‘Truman’, ‘Massy’, ‘Ernie’, ‘Roane’ and ‘Freedom’ (Rudd et al., Citation2001; Sneller et al., Citation2012; Jin et al., Citation2013; Liu et al., Citation2013). ‘Everest’, ‘Overland’, ‘Lyman’, ‘Heyne’ and ‘Hondo’ are hard winter wheat (HWW) that carry native resistance QTLs (Bockus et al., Citation2009; Zhang et al., Citation2012a; Jin et al., Citation2013). To improve FHB resistance in these wheat cultivars, they can serve as recurrent parents for pyramiding major QTLs from Asian sources. Also, since these cultivars may carry different QTLs, combining these QTLs together may provide highly resistant cultivars by selecting transgressive segregants. One example is a HWW cultivar ‘Everest’ (HBK1064-3/Betty ‘S’//VBF0589-1/IL89-6483) from Kansas. It has moderate FHB resistance under field conditions, especially type I resistance, but none of its parents (HBK1064-3/Betty ‘S’//VBF0589-1/IL89-6483) have any noticeable resistance. Maintaining FHB screening nursery with proper, but consistent, disease pressure across years may be critical for successful selection of these types of resistance. To improve selection efficiency, genomic selection may be a promising strategy for combining these minor QTLs from adapted cultivars in breeding programmes and provide moderate to high prediction accuracies for FHB resistance (Arruda et al., Citation2015).
Creating transgenic wheat expressing resistance genes provides another approach to improve FHB resistance. For example, Li et al. (Citation2015, Citation2017) transferred a barley UDP-glucosyltransferase into wheat. The transgenic plants showed significantly higher type II resistance and lower toxin content than non-transformed controls. In another study, transgenic plants overexpressing a β-1,3-glucanase showed enhanced type II, type III and type IV resistance (Mackintosh et al. Citation2007). Although the transgenic approach has been used to enhance wheat resistance for decades, transgenes showing consistent resistance against FHB are still not available for breeding. Recently, identification of TaHRC (Su et al., Citation2017) as Fhb1 opens another avenue for using gene editing to improve FHB resistance. TaHRC is a susceptibility gene for FHB and is a conserved gene present not only in wheat, but also in durum, barley and other cereal crops. Using gene editing, we can modify the gene sequence of TaHRC in susceptible wheat to improve FHB resistance (Su et al., Citation2017). Therefore, gene editing shows promise to improve wheat FHB resistance by knockdown of susceptible genes in commercial cultivars.
Prospectives for future FHB research
Use of wheat resistance to FHB is the most effective and sustainable strategy to manage FHB. To date, many QTLs for different types of resistance have been identified in wheat, but only Fhb1 shows high and stable FHB resistance in different genetic backgrounds. Recently, Fhb1 has been cloned and diagnostic markers have been developed for marker-assisted breeding. Direct transfer of Fhb1 to adapted wheat backgrounds using marker-assisted backcrossing can provide a quick solution to improve FHB resistance of commercial wheat cultivars. Resistance genes from alien species may be useful, but experience suggests it may be ineffective in some wheat backgrounds. For the minor QTLs in adapted cultivars, diagnostic markers are not available for marker-assisted breeding. Phenotypic selection and genomic selection may be able to combine several QTLs from different sources to create highly resistant cultivars. In addition, gene editing may provide another alternative to improve wheat resistance.
Acknowledgements
This is contribution number 17-297-J from the Kansas Agricultural Experiment Station. This project was partly funded by the US Wheat and Barley Scab Initiative; and the National Research Initiative Competitive Grants 2017-67007-25939 and 2017-67007-25929 from the US Department of Agriculture, National Institute of Food and Agriculture. Mention of trade names or commercial products in this publication is solely for the purpose of providing specific information and does not imply recommendation or endorsement by the US Department of Agriculture. US Department of Agriculture is an equal opportunity provider and employer.
Additional information
Funding
References
- Ágnes SH, Szabolcs LK, Mónika V, László P, János P, Csaba L, Ákos M. 2014. Differential influence of QTL linked to Fusarium head blight, Fusarium-damaged kernel, deoxynivalenol contents and associated morphological traits in a ‘Frontana’-derived wheat population. Euphytica. 200:9–26.
- Anderson JA. 2012a. Overview of breeding for FHB resistance in wheat-where we’ve come from and where we are. In: Canty S, Clark A, Anderson-Scully A, Van Sanford D, editors. Proceedings of the 2012 National fusarium head blight forum. US Wheat and Barley Scab initiative; p. 45.
- Anderson JA, Stack RW, Liu S, Waldron BL, Fjeld AD, Coyne C, Sevilla BM, Fetch JM, Song QJ, Cregan PB, et al. 2001. DNA markers for Fusarium head blight resistance QTLs in two wheat populations. Theor Appl Genet. 102:1164–1168.
- Anderson JA, Wiersma JJ, Linkert GL, Kolmer JA, Jin Y, Dill-Macky R, Wiersma JV, Hareland GA. 2012b. Registration of ‘Sabin’ Wheat. J Plant Reg. 6:174.
- Arruda MP, Brown PJ, Lipka AE, Krill AM, Kolb FL. 2015. Genomic selection for predicting Fusarium head blight resistance in a wheat breeding program. Plant Gen. 8:3.
- Arthur JC. 1891. Wheat scab. Ind Agric Exp Stat Bull. 36:129–138.
- Bai G, Kolb FL, Shaner G, Domier LL. 1999. Amplified fragment length polymorphism markers linked to a major quantitative trait locus controlling scab resistance in wheat. Phytopathology. 89:343–348.
- Bai G, Shaner G. 2004. Management and resistance in wheat and barley to Fusarium head blight. Annu Rev Phytopathol. 42:135–161.
- Bai GH, Plattner R, Desjardins A, Kolb F. 2001. Resistance to Fusarium head blight and deoxynivalenol accumulation in wheat. Plant Breed. 120:1–6.
- Bai GH, Shaner G. 1994. Scab of wheat: prospects and control. Plant Dis. 78:760–766.
- Ban T. 2001. Studies on the genetics of resistance to Fusarium head blight caused by F. graminearum Schwabe in wheat (Triticum aestivum L.). Bull Kyushu Natl Agric Exp Stn. 38:27–28.
- Ban T. 2003. Comparative genetic analysis of FHB-resistant germplasm for wheat improvement. In: Canty SM, Lewis J, Siler L, Ward RW, editors, Proceedings of the National Fusarium Head Blight Forum. US Wheat and Barley Scab initiative, Michigan State University; p. 215–217.
- Bernardo AN, Ma H, Zhang D, Bai G. 2011. Single nucleotide polymorphism in wheat chromosome region harboring Fhb1 for Fusarium head blight resistance. Mol Breed. 29:477–488.
- Bockus WW, Fritz AK, Martin TJ. 2009. Reaction of the 2008 Kansas Intrastate Nursery to Fusarium head blight. Plant Dis Man Rep. 3:CF009.
- Bonin CM, Kolb FL. 2009. Resistance to Fusarium head blight and kernel damage in a winter wheat recombinant inbred line population. Crop Sci. 49:1304–1312.
- Bourdoncle W, Ohm HW. 2003. Quantitative trait loci for resistance to Fusarium head blight in recombinant inbred wheat lines from the cross ‘Huapei 57-2ʹ/‘Patterson’. Euphytica. 131:131–136.
- Browne RA, Brindle KM. 2007. H NMR-based metabolite profiling as a potential selection tool for breeding passive resistance against Fusarium head blight (FHB) in wheat. Mol Plant Pathol. 8:401–410.
- Brown-Guedira G, Griffey C, Kolb F, McKendry A, Murphy J, Sanford D. 2008. Breeding FHB-resistant soft winter wheat: progress and prospects. Cereal Res Commun. 36:31–35.
- Buerstmayr H, Ban T, Anderson JA. 2009. QTL mapping and marker-assisted selection for Fusarium head blight resistance in wheat: a review. Plant Breed. 128:1–26.
- Buerstmayr H, Steiner B, Hartl L, Griesser M, Angerer N, Lengauer D, Miedaner T, Schneider B, Lemmens M. 2003. Molecular mapping of QTLs for Fusarium head blight resistance in spring wheat. II. Resistance to fungal penetration and spread. Theor Appl Genet. 107:503–508.
- Buerstmayr M, Huber K, Heckmann J, Steiner B, Nelson JC, Buerstmayr H. 2012. Mapping of QTL for Fusarium head blight resistance and morphological and developmental traits in three backcross populations derived from Triticum dicoccum x Triticum durum. Theor Appl Genet. 125:1751–1765.
- Buerstmayr M, Lemmens M, Steiner B, Buerstmayr H. 2011. Advanced backcross QTL mapping of resistance to Fusarium head blight and plant morphological traits in a Triticum macha x T. aestivum population. Theor Appl Genet. 123:293–306.
- Burt C, Steed A, Gosman N, Lemmens M, Bird N, Ramirez-Gonzalez R, Holdgate S, Nicholson P. 2015. Mapping a Type 1 FHB resistance on chromosome 4AS of Triticum macha and deployment in combination with two Type 2 resistances. Theor Appl Genet. 128:1725–1738.
- Cai J 2016. Meta-analysis of QTL for Fusarium head blight resistance in Chinese heat landraces using genotyping-by-sequencing [ Ph.D. Thesis]. Kansas State University.
- Cai J, Bai G. 2014. Quantitative trait loci for Fusarium head blight resistance in ‘Huangcandou’ × ‘Jagger’ wheat population. Crop Sci. 54:2520–2528.
- Cai X, Xu S, Oliver R, Zhang Q, Stack R, Zhong S, Friesen T, Halley S, Elias E 2008. Alien introgression for FHB resistance in wheat - challenges and strategies. In: Appels R, Eastwood R, Lagudah E, Langridge P,Mackay M,McIntyre L and Sharp P, editors. Proceedings of the 11th International Wheat Genet Symposium; Aug 24–29; Brisbane, Qld, Australia: Sydney Univ Press, Sydney (Australia).
- Cainong JC, Bockus WW, Feng Y, Chen P, Qi L, Sehgal SK, Danilova TV, Koo DH, Friebe B, Gill BS. 2015. Chromosome engineering, mapping, and transferring of resistance to Fusarium head blight disease from Elymus tsukushiensis into wheat. Theor Appl Genet. 128:1019–1027.
- Chu C, Niu Z, Zhong S, Chao S, Friesen TL, Halley S, Elias EM, Dong Y, Faris JD, Xu SS. 2011. Identification and molecular mapping of two QTLs with major effects for resistance to Fusarium head blight in wheat. Theor Appl Genet. 123:1107–1119.
- Clark AJ, Sarti-Dvorjak D, Brown-Guedira G, Dong Y, Baik B-K, Van Sanford DA. 2016. Identifying rare FHB resistant segregants in intransigent backcross and F2 winter wheat populations. Front Microbiol. 7:277.
- Cuthbert PA, Somers DJ, Brule-Babel A. 2007. Mapping of Fhb2 on chromosome 6BS: a gene controlling Fusarium head blight field resistance in bread wheat (Triticum aestivum L.). Theor Appl Genet. 114:429–437.
- Cuthbert PA, Somers DJ, Thomas J, Cloutier S, Brule-Babel A. 2006. Fine mapping Fhb1, a major gene controlling Fusarium head blight resistance in bread wheat (Triticum aestivum L.). Theor Appl Genet. 112:1465–1472.
- De Wolf ED, Madden L, Lipps P. 2003. Risk assessment models for wheat Fusarium head blight epidemics based on within-season weather data. Phytopathology. 93:428–435.
- Ding L, Xu H, Yi H, Yang L, Kong Z, Zhang L, Xue S, Jia H, Ma Z. 2011. Resistance to hemi-biotrophic F. graminearum infection is associated with coordinated and ordered expression of diverse defense signaling pathways. PLoS One. 6:e19008.
- Dubin J, Gilchrist L, Reeves J, McNab A, editors. 1997. Fusarium head scab: global status and future prospects. Proceedings of a Workshop, El Batan (Mexico); 10- 13-96– 10-17-96. 1997; Mexico (DF (Mexico)): CIMMYT. vi, 130 p.
- Fatima N 2016. Identification and deployment of QTLs for Fusarium head blight resistance in U.S. winter wheat. [ Ph.D. thesis]. Kansas State University.
- Ferrigo D, Raiola A, Causin R. 2016. Fusarium toxins in cereals: occurrence, legislation, factors promoting the appearance and their management. Molecules. 21:627.
- Gervais L, Dedryver F, Morlais JY, Bodusseau V, Negre S, Bilous M, Groos C, Trottet M. 2003. Mapping of quantitative trait loci for field resistance to Fusarium head blight in an European winter wheat. Theor Appl Genet. 106:961–970.
- Gilbert J, Haber S. 2013. Overview of some recent research developments in Fusarium head blight of wheat. Can J Plant Pathol. 35:149–174.
- Goswami RS, Kistler HC. 2004. Heading for disaster: fusarium graminearum on cereal crops. Mol Plant Pathol. 5:515–525.
- Guo J, Zhang X, Hou Y, Cai J, Shen X, Zhou T, Xu H, Ohm HW, Wang H, Li A, et al. 2015. High-density mapping of the major FHB resistance gene Fhb7 derived from Thinopyrum ponticum and its pyramiding with Fhb1 by marker-assisted selection. Theor Appl Genet. 128:2301–2316.
- He X,Lillemo M, Shi J, Wu J, Bjørnstad Å, Belova T, Dreisigacker S, Duveiller E, Singh P. 2016. QTL characterization of Fusarium head blight resistance in CIMMYT bread wheat line Soru#1. PLoS ONE 11: e0158052.
- He X, Singh PK, Schlang N, Duveiller E, Dreisigacker S, Payne T, He Z. 2013. Characterization of Chinese wheat germplasm for resistance to Fusarium head blight at CIMMYT, Mexico. Euphytica. 195:383–395.
- Holzapfel J, Voss H, Miedaner T, Korzun V, Häberle J, Schweizer G, Mohler V, Zimmermann G, Hartl L. 2008. Inheritance of resistance to Fusarium head blight in three European winter wheat populations. Theor Appl Genet. 117:1119–1128.
- Jia G, Chen P, Qin G, Bai G, Wang X, Wang S, Zhou B, Zhang S, Liu D. 2006. QTLs for Fusarium head blight response in a wheat DH population of ‘Wangshuibai’/’Alondra‘s”. Euphytica. 146:183–191.
- Jiang GL, Dong Y, Shi J, Ward RW. 2007a. QTL analysis of resistance to Fusarium head blight in the novel wheat germplasm ‘CJ 9306ʹ. II. Resistance to deoxynivalenol accumulation and grain yield loss. Theor Appl Genet. 115:1043–1052.
- Jiang GL, Shi J, Ward RW. 2007b. QTL analysis of resistance to Fusarium head blight in the novel wheat germplasm ‘CJ 9306ʹ. I. Resistance to fungal spread. Theor Appl Genet. 116:3–13.
- Jin F, Bai GH, Zhang DD, Dong YH, Ma LJ, Bockus W, Dowell F. 2014. Fusarium-damaged kernels and deoxynivalenol in Fusarium-infected U.S. winter wheat. Phytopathology. 104:472–478.
- Jin F, Zhang D, Bockus W, Baenziger PS, Carver B, Bai G. 2013. Fusarium head blight resistance in U.S. winter wheat cultivars and elite breeding lines. Crop Sci. 53:2006–2013.
- Kolb FL, Bai GH, Muehlbauer GJ, Anderson JA, Smith KP, Fedak G. 2001. Host plant resistance genes for Fusarium head blight: mapping and manipulation with molecular markers. Crop Sci. 41:611–619.
- Li G, Yen Y. 2008. Jasmonate and ethylene signaling pathway may mediate Fusarium head blight resistance in wheat. Crop Sci. 48:1888–18976.
- Li T, Bai G, Wu S, Gu S. 2011. Quantitative trait loci for resistance to Fusarium head blight in a Chinese wheat landrace ‘Haiyanzhong’. Theor Appl Genet. 122:1497–1502.
- Li T, Bai G, Wu S, Gu S. 2012. Quantitative trait loci for resistance to Fusarium head blight in the Chinese wheat landrace ‘Huangfangzhu’. Euphytica. 185:93–102.
- Li T, Luo M, Zhang D, Wu D, Li L, Bai G. 2016. Effective marker alleles associated with type II resistance to Fusarium head blight infection in fields. Breed Sci. 66:350–357.
- Li X, Michlmayr H, Schweiger W, Malachova A, Shin S, Huang Y, Dong YH, Wiesenberger G, McCormick S, Lemmens M, et al. 2017. A barley UDP-glucosyltransferase inactivates nivalenol and provides fusarium head blight resistance in transgenic wheat. J Exp Bot. 68:2187–2197.
- Li X, Shin S, Heinen S, Dill-Macky R, Berthiller F, Nersesian N, Clemente T, McCormick S, Muehlbauer G. 2015. Transgenic wheat expressing a barley UDP-glucosyltransferase detoxifies deoxynivalenol and provides high levels of resistance to Fusarium graminearum. Mol Plant-Microb Interact. 28:1237–1246.
- Lin F, Xue SL, Zhang ZZ, Zhang CQ, Kong ZX, Yao GQ, Tian DG, Zhu HL, Li CJ, Cao Y, et al. 2006. Mapping QTL associated with resistance to Fusarium head blight in the ‘Nanda2419ʹ x ‘Wangshuibai’ population II: type I resistance. Theor Appl Genet. 112:528–535.
- Liu S, Abate ZA, Lu H, Musket T, Davis GL, McKendry AL. 2007. QTL associated with Fusarium head blight resistance in the soft red winter wheat ‘Ernie’. Theor Appl Genet. 115:417–427.
- Liu S, Abate ZA, McKendry AL. 2005. Inheritance of Fusarium head blight resistance in the soft red winter wheat ‘Ernie’. Theor Appl Genet. 110:454–461.
- Liu S, Griffey CA, Hall MD, McKendry AL, Chen J, Brooks WS, Brown-Guedira G, Van Sanford D, Schmale DG. 2013. Molecular characterization of field resistance to Fusarium head blight in two U.S. soft red winter wheat cultivars. Theor Appl Genet. 126:2485–2498.
- Liu S, Hall MD, Griffey CA, McKendry AL. 2009. Meta-analysis of QTL associated with Fusarium head blight resistance in wheat. Crop Sci. 49:1955–1968.
- Liu S, Pumphrey MO, Gill BS, Trick HN, Zhang JX, Dolezel J, Chalhoub B, Anderson JA. 2008. Toward positional cloning of fhb1, a major QTL for Fusarium head blight resistance in wheat. Cereal Res Commun Suppl. 36:195–201.
- Löffler M, Schön C, Miedaner T. 2009. Revealing the genetic architecture of FHB resistance in hexaploid wheat (Triticum aestivum L.) by QTL meta-analysis. Mol Breed. 23:473–488.
- Mackintosh CA, Lewis J, Radmer LE, Shin S, Heinen SJ, Smith LA, Wyckoff MN, Dill-Macky R, Evans CK, Kravchenko S, et al. 2007. Overexpression of defense response genes in transgenic wheat enhances resistance to Fusarium head blight. Plant Cell Rep. 26:479–488.
- Mardi M, Pazouki L, Delavar H, Kazemi MB, Ghareyazie B, Steiner B, Nolz R, Lemmens M, Buerstmayr H. 2006. QTL analysis of resistance to Fusarium head blight in wheat using a ‘Frontana’-derived population. Plant Breed. 125:313–317.
- McCartney CA, Brûlé-Babel AL, Fedak G, Martin RA, McCallum BD, Gilbert J, Hiebert CW and Pozniak CJ. 2016. Fusarium head blight resistance QTL in the spring wheat cross Kenyon/86ISMN 2137. Front. Microbiol. 7:1542.
- McMullen M, Bergstrom G, De Wolf E, Dill-Macky R, Hershman D, Shaner G, Van Sanford D. 2012. A unified effort to fight an enemy of wheat and barley: fusarium head blight. Plant Dis. 96:1712–1728.
- Mergoum M, Frohberg RC, Stack RW 2007. Breeding hard red spring wheat for Fusarium head blight resistance, successes and challenges. In: Buck HT, Nisi JE, Salomo´N N, editors. Wheat production in Stressed Environments. Proceedings of the 7th International Wheat Conference; Nov 27–Dec 2, 2005; Mar del Plata, Argentina, Netherlands: Springer. p. 161–167.
- Mesterhazy A. 1995. Types and components of resistance to Fusarium head blight of wheat. Plant Breed. 114:377–386.
- Mesterhazy A, Bartok T, Mirocha CG, Komoroczy AR. 1999. Nature of wheat resistance to Fusarium head blight and the role of deoxynivalenol for breeding. Plant Breed. 118:97–110.
- Miller JD, Young JC, Sampson RD. 1985. Deoxynivalenol and Fusarium head blight resistance in spring cereals. Phytopathol Z. 113:359–367.
- Nganje WE, Kaitibie S, Wilson WW, Leistritz FL, Bangsund DA 2004. Economic impacts of Fusarium head blight in wheat and barley:1993-2001. Agribus Appl Econ Rep. No 538.
- Oliver RE, Cai X, Xu SS, Chen X, Stack RW. 2005. Wheat-alien species derivatives. Crop Sci. 45:1353.
- Paillard S, Schnurbusch T, Tiwari R, Messmer M, Winzeler M, Keller B, Schachermayr G. 2004. QTL analysis of resistance to Fusarium head blight in Swiss winter wheat (Triticum aestivum L). Theor Appl Genet. 109:323–332.
- Paul PA, Lipps P, Madden LV. 2006. Meta-analysis of regression coefficients for the relationship between Fusarium head blight and deoxynivalenol content of wheat. Phytopathology. 96:951–961.
- Prat1 N, Guilbert C, Prah U, Wachter E, Steiner B, Langin T, Robert O, Buerstmayr H. 2017. QTL mapping of Fusarium head blight resistance in three related durum wheat populations. Theor Appl Genet. 130:13–27.
- Pritsch C, Muehlbauer GJ, Bushnell WR, Somers DA, Vancel CP. 2000. Fungal development and induction of defense response genes during early infection of wheat spikes by Fusarium graminearum. Mol Plant-Microbe Interact. 13:159–169.
- Qi LL, Pumphrey MO, Friebe B, Chen PD, Gill BS. 2008. Molecular cytogenetic characterization of alien introgressions with gene Fhb3 for resistance to Fusarium head blight disease of wheat. Theor Appl Genet. 117:1155–1166.
- Rawat N, Pumphrey MO, Liu S, Zhang X, Tiwari VK, Ando K, Trick HN, Bockus WW, Akhunov E, Anderson JA, et al. 2016. Wheat Fhb1 encodes a chimeric lectin with agglutinin domains and a pore-forming toxin-like domain conferring resistance to Fusarium head blight. Nat Genet. 48:1576–1580.
- Ribichich KF, Lopez SE, Vegetti AC. 2000. Histopathological spikelet changes produced by Fusarium graminearum in susceptible and resistant wheat cultivars. Plant Dis. 84:794–802.
- Rudd JC, Horsley RD, McKendry AL, Elias EM. 2001. Host plant resistance genes for Fusarium head blight: sources, mechanisms, and utility in conventional breeding. Crop Sci. 41:620–627.
- Schroeder H, Christensen J. 1963. Factors affecting resistance of wheat to scab caused by Gibberella zeae. Phytopathology. 53:831–838.
- Schweiger W, Steiner B, Vautrin S, Nussbaumer T, Siegwart G, Zamini M, Jungreithmeier F, Gratl V, Lemmens M, Mayer KF, et al. 2016. Suppressed recombination and unique candidate genes in the divergent haplotype encoding Fhb1, a major Fusarium head blight resistance locus in wheat. Theor Appl Genet. 129:1607–1623.
- Semagn K, Skinnes H, Å B, Marøy AG, Tarkegne Y. 2007. Quantitative trait loci controlling Fusarium head blight resistance and low deoxynivalenol content in hexaploid wheat population from ‘Arina’ and ‘NK93604ʹ. Crop Sci. 47:294–303.
- Shen XR, Ittu M, Ohm HW. 2003. Quantitative trait loci conditioning resistance to Fusarium head blight in wheat line F201R. Crop Sci. 43:850–857.
- Sneller C, Paul P, Herald L, Sugerman B. 2012. Report on the 2011-2012 Northern uniform winter wheat scab nurseries (NUWWSN and PNUWWSN). In: Canty S, Clark A, Anderson-Scully A, Van Sanford D, editors. Proceedings of the 2012 National Fusarium Head Blight Forum. US Wheat and Barley Scab initiative; ASAP Printing, Inc., Lansing, MI; p. Pg. 100–104.
- Somers DJ, Fedak G, Savard M. 2003. Molecular mapping of novel genes controlling Fusarium head blight resistance and deoxynivalenol accumulation in spring wheat. Genome. 46:555–564.
- Somers DJ, Isaac P, Edwards K. 2004. A high-density microsatellite consensus map for bread wheat (Triticum aestivum L.). Theor Appl Genet. 109:1105–1114.
- Steiner B, Lemmens M, Griesser M, Scholz U, Schondelmaier J, Buerstmayr H. 2004. Molecular mapping of resistance to Fusarium head blight in the spring wheat cultivar ‘Frontana’. Theor Appl Genet. 109:215–224.
- Su Z, Bernardo A, Tian B, Wang S, Ma H, Cai S, Liu D, Zhang D, Li T, Trick HN, et al. 2016. Development of high-throughput diagnostic markers for Fhb1, a major gene for FHB resistance in wheat. In: Canty S, Clark A, Anderson-Scully A, Van Sanford D, editors. Proceedings of the 2016 National Fusarium Head Blight Forum. US Wheat and Barley Scab initiative; p. 95.
- Su Z, Jin S, Bernardo A, St Amand P, Bai G 2017. A sequence deletion in HRC-like gene confers Fhb1 resistance to Fusarium head blight in wheat. Proceedings of the Plant Animal Genome XXV Conference, Jan 14–18, San Diego, CA. P0894.
- Suzuki T, Sato M, Takeuchi T. 2012. Evaluation of the effects of five QTL regions on Fusarium head blight resistance and agronomic traits in spring wheat (Triticum aestivum L.). Breed Sci. 62:11–17.
- Szabó-Hevér Á, Lehoczki-Krsjak S, Tóth B, Purnhauser L, Buerstmayr H, Steiner B, Mesterházy Á. 2012. Identification and validation of Fusarium head blight and Fusarium-damaged kernel QTL in a ‘Frontana’/’Remus’ DH mapping population. Can J Plant Pathol. 34:224–238.
- Waldron BL, Moreno-Sevilla B, Anderson JA, Stack RW, Frohberg RC. 1999. RFLP mapping of QTL for Fusarium head blight resistance in wheat. Crop Sci. 39:805–811.
- Xue S, Li G, Jia H, Xu F, Lin F, Tang M, Wang Y, An X, Xu H, Zhang L, et al. 2010. Fine mapping Fhb4, a major QTL conditioning resistance to Fusarium infection in bread wheat (Triticum aestivum L.). Theor Appl Genet. 121:147–156.
- Xue S, Xu F, Tang M, Zhou Y, Li G, An X, Lin F, Xu H, Jia H, Zhang L, et al. 2011. Precise mapping Fhb5, a major QTL conditioning resistance to Fusarium infection in bread wheat (Triticum aestivum L). Theor Appl Genet. 123:1055–1063.
- Yang J, Bai G, Shaner GE. 2005a. Novel quantitative trait loci (QTL) for Fusarium head blight resistance in wheat cultivar ‘Chokwang’. Theor Appl Genet. 111:1571–1579.
- Yang Z, Gilbert J, Fedak G, Somers DJ. 2005b. Genetic characterization of QTL associated with resistance to Fusarium head blight in a doubled-haploid spring wheat population. Genome. 48:187–196.
- Yu J, Bai G, Cai S, Dong Y, Ban T. 2008a. New Fusarium head blight resistant sources from Asian wheat germplasm. Crop Sci. 48:1090–1097.
- Yu JB, Bai GH, Zhou WC, Dong YH, Kolb FL. 2008b. Quantitative trait loci for Fusarium head blight resistance in a recombinant inbred population of ‘Wangshuibai’/’Wheaton’. Phytopathology. 98:87–94.
- Zhang X, Bai G, Bockus W, Ji X, Pan H. 2012a. Quantitative trait loci for Fusarium head blight resistance in U.S. hard winter wheat cultivar ‘Heyne’. Crop Sci. 52:1187–1194.
- Zhang X, Fu J, Hiromasa Y, Pan H, Bai G. 2013. Differentially expressed proteins associated with Fusarium head blight resistance in wheat. PLoS ONE. 8:e82079.
- Zhang X, Pan H, Bai G. 2012b. Quantitative trait loci responsible for Fusarium head blight resistance in Chinese landrace ‘Baishanyuehuang’. Theor Appl Genet. 125:495–502.
- Zhou W, Kolb FL, Yu J, Bai GH, Boze LK, Domier LL. 2004. Molecular characterization of Fusarium head blight resistance in ‘Wangshuibai’ with simple sequence repeat and amplified fragment length polymorphism markers. Genome. 47:1137–1143.
- Zhou WC, Kolb FL, Bai GH, Dromie LL, Boze LK, Smith NJ. 2003. Validation of a major QTL for scab resistance with SSR markers and use of marker-assisted selection in wheat. Plant Breed. 122:40–46.
- Zhou WC, Kolb FL, Bai GH, Shaner GE, Domier LL. 2002. Genetic analysis of scab resistance QTL in wheat with microsatellite and AFLP markers. Genome: 45:1–9.