Abstract
A total of 252 aerial spore samples from the provinces of Quebec and Ontario were obtained during an extensive research programme focusing on aerobiology of fungal spores. Samples were collected on 95 sticky rods from rotary arm spore collectors and 157 filters from passive rain collectors. DNA from samples was PCR-amplified for fungal ribosomal ITS and sequenced using High Throughput Sequencing (HTS) methods. After bioinformatics analysis of DNA sequences, the presence of Diplodia corticola, an emerging tree pathogen in North America, was observed. In total, 313 DNA sequence reads from aerial spores of D. corticola were found in the Illumina data set, and 199 DNA sequence reads were obtained from the Ion Torrent data set. DNA of D. corticola was found in 16 of the 32 sampled sites, always less than 10 reads per site, with the exception of three sites – Quebec City, Aylmer and Ottawa – where 287, 125 and 73 DNA reads, respectively, were detected. This is not a first report of the presence of D. corticola causing a tree disease in Canada as symptomatic trees have not been identified. Typically, like many other species of the Botryosphaeriaceae, this fungal pathogen is believed to be an opportunistic plant endophyte capable of living asymptomatically for several years before showing up as a pathogen when conducive conditions arise. Its presence as singletons in nearly half the sampled sites in Quebec and Ontario may be the result of long-distance spore dispersal originating from known infected sites in Massachusetts, Maine and other unknown sites in the north-eastern USA. However, its much higher read counts in three sites may indicate the possibility of a few trees being asymptomatically infected and spreading conidia locally.
Résumé
En tout, 252 échantillons de spores aéroportées ont été collectés au Québec et en Ontario dans le cadre d’un vaste programme de recherche sur l’aérobiologie des spores fongiques. Les échantillons ont été collectés sur les 95 tiges, enduites d’une substance collante, de collecteurs de spores à bras rotatifs et sur les 157 filtres de capteurs d’eau pluviale passifs. L’ADN des échantillons a été amplifié par PCR en fonction de la région de l’ITS ribosomique fongique et séquencé en recourant à la méthode de séquençage à grande échelle. Après avoir procédé à l’analyse bio-informatique des séquences d’ADN, Diplodia corticola, un agent pathogène forestier émergent en Amérique du Nord, a été décelé. En tout, 313 lectures de séquences d’ADN de spores aéroportées de D. corticola ont été trouvées dans le jeu de données Illumina et 199 lectures de séquences d’ADN ont été obtenues du jeu de données Ion Torrent. L’ADN de D. corticola a été trouvé à 16 des 32 sites échantillonnés, toujours moins de 10 lectures par site, sauf pour 3 sites — Québec, Aylmer et Ottawa — où 287, 125 et 73 lectures d’ADN, respectivement, ont été détectées. Il ne s’agit pas d’une première mention de D. corticola causant une maladie chez les arbres au Canada, puisqu’on n’a pas trouvé d’arbres symptomatiques. Généralement, comme plusieurs autres espèces de la famille des Botryosphaeriaceae, cet agent pathogène fongique est considéré comme un endophyte opportuniste capable de vivre sans provoquer de symptômes pendant plusieurs années avant que des conditions propices lui permette de s’afficher en tant qu’agent pathogène. Sa présence en tant qu’organisme isolé dans presque la moitié des sites au Québec et en Ontario peut résulter de la dispersion, sur de longues distances, des spores provenant de sites infectés connus au Massachusetts et au Maine ainsi que de sites inconnus du nord-est des États-Unis. Toutefois, les nombres de lectures substantiellement plus élevés dans trois sites peuvent indiquer que quelques arbres asymptomatiques infectés disséminent localement des conidies.
Introduction
In recent years, Diplodia corticola Phillips, Alves & Luque has emerged as an important new fungal pathogen causing bot canker of oak in Maine (Aćimović et al., Citation2016), Massachusetts (Munck et al., Citation2017), West Virginia (Martin et al., Citation2017), Florida (Dreaden et al., Citation2011), Texas, California (Úrbez-Torres et al., Citation2009, Citation2010) and Wisconsin (Smith & Stanosz, Citation2018). Originally identified in Europe and then reported in Africa (Alves et al., Citation2004; Linaldeddu et al., Citation2013), it was first reported in California in 2010 on trees diseased since 2002 (Lynch et al., Citation2010). Some of these reports mentioned the occurrence of the pathogen at one single location, leading to speculation that it reflected a relatively recent introduction into North America. However, Smith & Stanosz (Citation2018) showed the pathogen was well established almost two decades ago, a fact questioning its origin. As an emerging disease reported close to Canadian borders, it was highlighted in the June 2016 Canadian Food Inspection Agency (CFIA) Plant Science Scan (Canadian Food Inspection Agency [CFIA], Citation2016a) and was the object of a Pest Categorization (Canadian Food Inspection Agency [CFIA], Citation2016b).
High Throughput Sequencing (HTS) methods of PCR amplicons with metabarcoding approaches are a powerful method to detect plant pathogens from environmental samples. It allows detection of low abundance species which may remain undetected by conventional detection methods. Initial studies on fungal plant pathogens using amplicon cloning and Sanger sequencing (Fierer et al., Citation2007; Bérubé & Nicolas, Citation2015) were followed with the real HTS capacity of Roche 454 sequencing (Prigigallo et al., Citation2016; Malacrinò et al., Citation2017). The more productive sequencing methods Illumina MiSeq (Adhikari et al., Citation2013; Lyon et al., Citation2016; Miller et al., Citation2016) and Ion Torrent (Leboldus et al., Citation2015) made possible plant pathogen identifications from large environmental sampling sets (see Lindahl et al., Citation2013 for complete method comparison).
The objective of this study was to use HTS methods coupled with a bioinformatics pipeline to detect new invasive forest pathogens from aerial spore samples. Here, we describe the discovery of the presence of D. corticola aerial spores in Ontario and Québec.
Materials and methods
DNA extraction
A total of 143 samples were obtained during an intensive research programme focusing on fungal aerial spores. Samples were collected on 95 sticky rods from rotary arm spore collectors and 48 filters from passive rain filter collectors. Rods and filters were collected after 1 week in the field from July to November in 2014 (at 16 sites) and 2015 (at 15 sites), for a total of 29 sites (), and kept frozen at −20°C until extraction. To avoid forest pathogen contaminations during processing of samples, our lab facility does not deal with symptomatic plant material or Petri culture collections.
Fig. 1 Diplodia corticola Illumina/Ion Torrent DNA read counts in 32 aerial spore sampling sites in the provinces of Quebec and Ontario in 2014 and 2015.
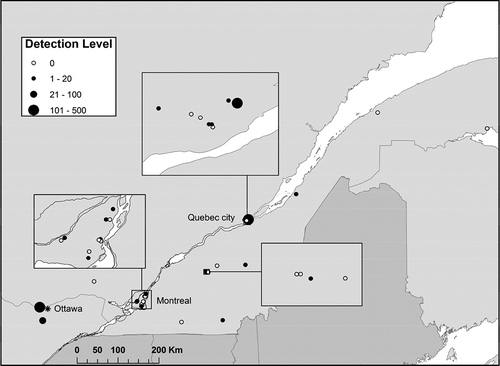
Passive funnel-shaped JB rain collectors (described in Hambleton et al., Citation2007), 29 cm in diameter and designed with filter assemblage for immediate filtration and spore capture on a 25 mm Whatman GF/D glass fibre (2.7 µm pore size) were deployed in the field. All rain collector samples originated from Montreal and Quebec City. Total DNA extraction was done on a quarter pie-shaped glass fibre section. Sections were added to a round-bottom 2-mL extraction tube with 700 uL of Tris pH 8.0 buffer and incubated at 65°C for 5 min, then sonicated at 65°C for 5 min. This step was repeated again, and tubes were centrifuged at 10 000 rpm for 5 min. The supernatant was removed and filters with spores at the bottom were used for DNA extraction with Fast DNA Spin kit for Soil (MP Bio).
Sticky rods were prepared as described in Fall et al. (Citation2015). Airborne spores were also collected on silicone-coated (Versilube ® G697, Novagard Solutions, Cleveland, OH) rods prepared by dipping them into a silicone-hexane solution (7%, w/v). Rods were set on a rotating-arm impaction spore sampler (Phytodata Inc., Sherrington, QC, Canada) running for 5 min every hour, and changed once a week. They consisted of two vertical rods (sampling surface of 1.65 mm × 20 mm) separated by 83 mm. Airborne particles impacted with the leading edges of the rotation rods coated with silicone grease. After exposure, rods were stored at −20°C. Spore recovery from rods was done as described in Lamarche et al. (Citation2016). One rod from each pair was placed in a 2-mL Eppendorf ® Safe-Lock microtube, and 1 mL of hexane was added to the tubes. Tubes were then vortexed for 30 s and rods were removed. Tubes were centrifuged for 2 min at 20 000 × g, and the supernatant was discarded. Pellets were rinsed with 1 mL of hexane to remove residual silicone. Finally, the spore pellets were dried using a SpeedVac™ Concentrator and samples were kept at −80°C until needed. DNA extraction was done with the QIAamp DNA Micro kit (Qiagen) according to the manufacturer’s instructions. Briefly, spores were resuspended in 180 µL of the ATL lysing buffer supplemented with 1 µL of Reagent DX (Qiagen) and disrupted with a 3-mm tungsten bead, in a MixerMill 300 for two periods of 90 s at 30 Hz. Samples were then quickly centrifuged and 20 µL of Proteinase K was added. Samples were then incubated at 56°C overnight with 10 s vortexing every 10 min during the first hour of incubation. The next day, DNA was extracted according to the manufacturer’s instructions and eluted in 100 µL of EB Buffer.
Amplicon preparation for Illumina sequencing
The eluted DNA (gDNA) was used as template for polymerase chain reaction (PCR). ITS regions of the ribosomal DNA fragment (ITS1-5.8S) were first amplified in PCR-1 using the Illumina Fusion Primers 1 and 2 (see for fusion primer constructs). The Illumina DNA sequencer faces a problem called phasing (Wu et al., Citation2015) when dealing with environmental samples. To avoid that, the ITS specific primers of PCR 1 were staggered () and used in corresponding pairs. One quarter of the samples were amplified using Primer forward A with Reverse primer H, then the next quarter with primers B and G, the next quarter with primers C and F, and the final quarter with primers D and E. The amplicons obtained were then reamplified in PCR 2 with Illumina Universal Indexed Primer 1 and Illumina Indexed Primer 2 compatible with Illumina technology. These primers contained an index sequence for tagging every sequence to a sample. Fifteen forward indexed sequences were used in combination with 15 reverse indexed sequences to provide 225 indexed combinations (Supplementary Table S1).
Table 1. Illumina fusion primer constructions used amplify fungal DNA from aerial spore samples.
Each PCR1 reaction contained 3 µL of 1/10 diluted genomic DNA, 2.5 µL of PCR buffer 10× (Invitrogen, Carlsbad, CA), 50 mM MgCl2, 10 µM of each dNTPs, 1 ng BSA, 25 µM of each of the Illumina Fusion Primers 1 and 2, and 0.2 µL of Platinum® Taq DNA Polymerase (Invitrogen). The final volume was adjusted to 25 µL using 15.6 µL of Ultrapure Water (Invitrogen). The PCR cycle parameters consisted of an initial denaturation at 95°C for 2 min, followed by 35 cycles of denaturation at 94°C for 20 s, annealing at 50°C for 60 s and extension at 72°C for 60 s, and a final extension step at 72°C for 10 min. The target amplicon products (~ 450 base pairs) for each PCR were then verified on a 1.5% agarose gel.
Each PCR 2 reaction contained 1 µL of 1/50 diluted PCR 1 amplicons, 2.5 µL of PCR buffer 10× (Invitrogen), 50 mM MgCl2, 10 µM of each dNTPs, 25 µM of each of the Illumina Universal Primers 1 and 2, and 0.2 µL of Platinum® Taq DNA Polymerase (Invitrogen). The final volume was adjusted to 25 µL using 12.6 µL of Ultrapure Water (Invitrogen). The PCR cycle parameters consisted of an initial denaturation at 95°C for 10 min, followed by 15 cycles of denaturation at 95°C for 15 s, annealing at 60°C for 30 s, extension at 72°C for 60 s, and a final extension step at 72°C for 3 min.
Amplicons were then purified using an Agencourt® AMPure® XP (Beckman Coulter, Brea, CA) magnetic PCR clean-up system to eliminate primer dimers (fragments < 80 bp) during the first purification using a 1.8:1 volume ratio of magnetic beads for amplicons. Fragments smaller than 290 bp were eliminated during the second purification with a ratio of 0.7:1. The clean PCR amplicons were quantified with the Quant-iT ™ Picogreen® ds DNA Assay kit (Invitrogen, Eugene, OR). DNA concentrations were measured with a Fluoroskan Ascent Labsystem (Thermo Electron Corporation, Vantaa, Finland).
Each of the 143 samples tagged with differing indexes were then pooled in equimolar amounts of 4 ng DNA per samples. Final quantification of pool, verification of primer artefact removal and amplicon quality check were done with the Agilent 2100 BioAnalyzer (Agilent Technologies, Santa Clara, CA). Seventy-five nanograms of pooled DNA samples were sent to the Next Generation Sequencing Platform, Genomics Centre, CHU de Québec, Université Laval Research Centre, Quebec City, QC, which performed paired-end 300-bp sequencing using MiSeq Reagent Kit v3 (600 cycles) with an Illumina MiSeq system.
Another set of samples were obtained, processed and analysed as described in Tremblay et al. (Citation2018). It consisted of 109 passive rain filter collector samples from three sites (Ottawa, Aylmer and Montreal) that were extracted and amplified using a somewhat similar protocol as the one described below, but sequenced using Ion Torrent technology. A blank sample consisting of molecular grade water was added to the protocol.
Bioinformatics analysis: Illumina reads treatment and clusterization
A bioinformatics treatment of HTS DNA sequences was executed to create Operational Taxonomic Units (OTUs), our proxies for fungal species (Huse et al., Citation2010; Kunin et al., Citation2010). Sequence assembly was done using PANDASeq v2.7 (Masella et al., Citation2012). Sequences were then filtered and trimmed with Illumicut (Gagné & Bérubé, Citation2017a), sequences with homopolymer > 9 bp were removed with HomopRemover (Gagné & Bérubé, Citation2017b) and reads < 120 bp were discarded.
Dereplication on the full-length of the set of sequences was performed before construction of clusters with MOTHUR v.1.28.0 (Schloss et al., Citation2009). While not recommended by some authors (Nilsson et al., Citation2011; Lindahl et al., Citation2013), all singletons passing the quality control step were kept for analysis because the assignment of singletons as a sequencing artefact is not always justified after reads processing (Lentendu et al., Citation2011; Penton et al., Citation2013) and could lead to a loss from the data set of new emerging pathogens found at very low frequency. The set of sequences was then organized into clusters with USEARCH 64 bit v8.0.1623 (Edgar, Citation2010), with a sequence similarity threshold of 97%, to agglomerate reads and form the OTUs, with the most abundant sequence type serving as cluster seeds. As no single similarity threshold will accurately reflect the species level throughout the fungal kingdom, a 3% dissimilarity cutoff was selected as a compromise in order to avoid overestimating fungal diversity versus masking rare OTUs and putative new emerging fungal pathogens (Nilsson et al., Citation2008; Huse et al., Citation2010; Schoch et al., Citation2012). Representative sequences, which are the most frequent sequence in each OTU, were extracted and then screened against GenBank nt database using local BLAST.
BLAST Output files were then organized alphabetically by Latin names and then parsed for plant pathogens of interest and those on the quarantine species list of Canada and other industrialized countries.
A tree showing phylogenetic relationships among D. corticola and closely related Botryosphaeriaceae species was built using the Neighbour-joining method (Saitou & Nei, Citation1987). The percentage of replicate trees in which the associated taxa clustered together in the bootstrap test (10 000 replicates) are shown next to the branches. The tree is drawn to scale, with branch lengths in the same units as those of the evolutionary distances used to infer the phylogenetic tree. The evolutionary distances were computed using the Jukes–Cantor method (Jukes & Cantor, Citation1969) and are in the units of the number of base substitutions per site. Evolutionary analyses were conducted in MEGA7 v.7.0.26 (Kumar et al., Citation2016).
Results
A total of 12 million clean double-stranded Illumina DNA reads were obtained after filtering. Cluster analysis, with a sequence similarity threshold of 97%, gave 76 267 OTUs, our proxies for fungal species. After conducting a BLAST search, organizing the file alphabetically by Latin names and parsing for plant pathogens of interest on quarantine species lists, we noticed the presence of B. corticola, a newly emerging tree pathogen in North America (Lynch et al., Citation2010). A total of 313 DNA sequences from aerial spores of D. corticola were found in the Illumina data set, all belonging to a single OTU, among the 24 000 fungal OTUs observed in this study (when excluding singletons OTUs). DNA of D. corticola was found in 13 of the 29 sampled sites (), always less than 10 reads per site, with the exception of one site in Quebec City, where a total of 287 reads were found over 3 separate weeks in 2014 (July 8: 9 reads; July 16: 275 reads and August 6: 3 reads). It was present on both types of spore collectors throughout the collection period, in both years. It was also found in aerial spore filter samples in all three additional sites from the Ion Torrent study (authors, unpublished) with the two sites from the Ottawa region showing DNA read counts of 73 and 125, from a data set of 45 million DNA reads. The blank sample (water) contained different fungal ascomycetes and basidiomycetes species at very low frequencies (< 50 counts): Aureobasidium sp., Candida sp., Cladosporium sp., Dothideomycetes sp., Microbotryomycetes sp., Mortierella sp., Neonectria sp., Phoma sp., Rhodotorula sp., Taphrina sp., Tremellomycetes sp., Trichocomaceae sp. and other uncultured fungus.
Phylogenetic analysis using Botryosphaericeae species from Europe and North America showed that both fungal OTUs (OTU 1427 from Quebec and OTU 01 from Ontario) clustered with other D. corticola isolates from the USA and Europe (). All DNA sequences from this study are deposited in GenBank (accession numbers: MG195116–MG195122).
Fig. 2 Phylogenetic relationships among Diplodia DNA sequence reads from aerial fungal spore samples based on Neighbour-joining analysis on the ITS1 partial 5.8s ribosomal sequence data. Bootstrap percentages are indicated at nodes (10 000 replicates), and branch length is proportional to the number of changes indicated. GenBank accession numbers or CBS isolate numbers are indicated after species names.
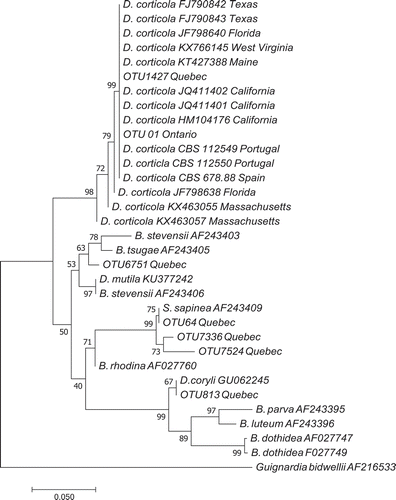
DNA from three other species from the Botryosphaeriaceae family were also found in this study: the very common OTU 64 (15 496 DNA reads), OTU 7336 and OTU 7524 clustered with Sphaeropsis sapinea, OTU 813 clustered with D. coryli, and OTU 6751 clustered among a group of B. stevensii, B. mutila and B. tsugae ().
Discussion
This is not a first report on the presence of D. corticola in Canada as we do not have cultured isolates from infected trees or aerial spore collections. A field survey of the Quebec City area, where the read counts were highest, failed to show trees with signs or symptoms of D. corticola. Branch samples collected on five unhealthy and five healthy oak trees in the vicinity and processed like aerial spore samples also failed to show the presence of D. corticola DNA in plant tissues of surrounding trees (data not shown). Typically, like many other species of the Botryosphaeriaceae, this fungal pathogen is believed to be an opportunistic plant endophyte (Canadian Food Inspection Agency [CFIA], Citation2016b), capable of living asymptomatically for several years before showing up as a pathogen when conducive conditions arise.
Is the fungus present on trees and established in Canada? Its presence as singletons in nearly half the sampled sites may be the result of long-distance spore dispersal originating from known infected sites in Massachusetts, Maine and other unknown sites in the north-eastern USA. However, its much higher read counts in three locations may indicate the possibility of a few trees being asymptomatically infected and spreading conidia locally. Further tree samplings are planned in 2018 to locate possibly infected trees, obtain fungal isolates, and test Koch’s postulates. It is important to mention that read counts in HTS methods are considered semi-quantitative (Amend et al., Citation2010), meaning there is no strong quantitative relationship between read count and spore count. Read count only gives an indication of the order of magnitude of spore presence. If precise indication of aerial spore load for one specific fungal species is required, quantitative PCR would become the tool of choice.
Emerging forest diseases have an unknown incubation period as there is rarely data available on the initial introduction event, only data on its first appearance as a disease. With D. corticola, a unique opportunity for monitoring the incubation time until the disease symptoms appear may be provided. However, as it may be an asymptomatic endophyte, it is possible symptoms may never express as a tree disease in Canada.
The detection of D. corticola demonstrates that the monitoring of emerging forest pathogens using HTS is an efficient method that is not limited in its scope by the number and availability of DNA probes targeting specific fungal species. A typical Illumina run yields tens of thousands of fungal species, which provides in-depth information on specific species present, and also on potentially new putative pathogens not previously discovered, but identified only by their DNA sequence similarity to known pathogen genera. Compared with DNA probes, detection data based on DNA sequences help to eliminate the possibility of false positives provided that: (a) contaminants can be ruled out (which is the case when the pathogen has only been found abroad and has never entered the research facility); (b) there is no taxonomic uncertainty that could blur species differentiation; (c) DNA sequences that can distinguish closely related species are available. In our study, we met all these requirements. However, our data do not provide information about the viability of the spores detected, an issue that can be resolved using a genomics approach (e.g. detecting presence of D. corticola mRNAs in spores) when spore bulks from field traps cannot be cultured to assay their viability.
Acknowledgements
We thank the field collaborators who hosted and operated the rotary arm spore collectors: Normand Bérubé, Jean-Francois Pépin, Jacques Turcotte, Réjean Ouellet, Martin Lepage, Pascale St-Laurent, Robert Morisset and Alexander Bates. We also thank Robert Lavallée and Philippe Labrie for rain sample collections and Stéphane Bourassa for preparation of figures.
References
- Aćimović SG, Harmon CL, Bec S, Wyka S, Broders K, Doccola JJ. 2016. First report of Diplodia corticola causing decline of red oak (Quercus rubra) trees in Maine. Plant Dis. 100:649.
- Adhikari BN, Hamilton JP, Zerillo MM, Tisserat N, Lévesque CA, Buell CR. 2013. Comparative genomics reveals insight into virulence strategies of plant pathogenic oomycetes. PLoS One. 8:e75072.
- Alves A, Correia A, Luque J, Phillips A. 2004. Botryosphaeria corticola, sp. nov. on Quercus species, with notes and description of Botryosphaeria stevensii and its anamorph, Diplodia mutila. Mycologia. 96:598–613.
- Amend AS, Seifert KA, Bruns TD. 2010. Quantifying microbial communities with 454 pyrosequencing: does read abundance count? Mol Ecol. 19:5555–5565.
- Bérubé JA, Nicolas GG. 2015. Alien fungal species on asymptomatic live woody plant material imported into Canada. Can J Plant Pathol. 37:67–81.
- Canadian Food Inspection Agency [CFIA]. 2016a Jul. Plant Science Scan.Update: first report of Botryosphaeria corticola (bot canker of oak) in Maine, 16. [accessed 2017 Nov 22]. http://publications.gc.ca/site/eng/9.802674/publication.html. ISSN 2369-4254.
- Canadian Food Inspection Agency [CFIA]. 2016b. Pest categorization. Botryosphaeria corticola Phillips, Alves & Luque. Bot canker of oak. Plant health risk assessment unit. Plant Health Science Division, Ottawa, ON, Canada 2016 Dec 16. Request 2016-52.
- Dreaden TJ, Shin K, Smith JA. 2011. First report of Diplodia corticola causing branch cankers on live oak (Quercus virginiana) in Florida. Plant Dis. 95:1027.
- Edgar RC. 2010. Search and clustering orders of magnitude faster than BLAST. Bioinformatics. 26:2460–2461.
- Fall ML, Tremblay DM, Gobeil-Richard M, Couillard J, Rocheleau H, Van Der Heyden H, Lévesque CA, Beaulieu C, Carisse O. 2015. Infection efficiency of four Phytophthora infestans clonal lineages and DNA-based quantification of sporangia. PLoS One. 10:e0136312.
- Fierer N, Breitbart M, Nulton J, Salamon P, Lozupone C, Jones R, Robeson M, Edwards RA, Felts B, Rayhawk S. 2007. Metagenomic and small-subunit rRNA analyses reveal the genetic diversity of bacteria, archaea, fungi, and viruses in soil. Appl Environ Microbiol. 73:7059–7066.
- Gagné P, Bérubé JA 2017a. Illumicut, a C++ program specially designed to efficiently detect and remove forward and reverse sequencing primers in paired-end reconstructed sequences. [accessed 2017 Nov 22]. https://github.com/Patg13/Illumicut
- Gagné P, Bérubé JA 2017b. HomopRemover, a program designed to efficiently remove sequences containing very long homopolymers. [accessed 2017 Nov22]. https://github.com/Patg13/HomopRemover
- Hambleton S, Tenuta A, Anderson T, Tropiano R, Bergeron J, Van Herk C 2007. Asian SBR monitoring program pays off in 2007 with first detections in Canada. 2007 National SBR Symposium; Dec 12-14; Louisville (KY): American Phytopathological Society. [accessed 2017 Nov 22] http://www.plantmanagementnetwork.org/infocenter/topic/soybeanrust/2007/posters/
- Huse SM, Welch DM, Morrison HG, Sogin ML. 2010. Ironing out the wrinkles in the rare biosphere through improved OTU clustering. Environ Microbiol. 12:1889–1898.
- Jukes TH, Cantor CR. 1969. Evolution of protein molecules. In: Munro HN, editor. Mammalian Protein Metabolism. New York (NY): Academic Press; p. 21–132.
- Kumar S, Stecher G, Tamura K. 2016. MEGA7: Molecular Evolutionary Genetics Analysis version 7.0 for bigger datasets. Mol Bio Evol. 33:1870–1874.
- Kunin V, Engelbrekston A, Ochman H, Hugenholtz P. 2010. Wrinkles in the rare biosphere: pyrosequencing errors can lead to artificial inflation of diversity estimates. Environ Microbiol. 12:118–123.
- Lamarche J, Potvin A, Stewart D, Blais M, Pelletier G, Shamoun SF, Hamelin RC, Tanguay P. 2016. Real-time PCR assays for the detection of Heterobasidion irregulare, H. occidentale, H. annosum sensu stricto and the Heterobasidion annosum complex. For Pathol. 47:e12321.
- Leboldus JM, Kinzer K, Richards J, Ya Z, Yan C, Friesen TL, Brueggeman R. 2015. Genotype‐by‐sequencing of the plant‐pathogenic fungi Pyrenophora teres and Sphaerulina musiva utilizing Ion Torrent sequence technology. Mol Plant Pathol. 16:623–632.
- Lentendu G, Zinger L, Manel S, Coissac E, Choler P, Geremia RA, Melodelima C. 2011. Assessment of soil fungal diversity in different alpine tundra habitats by means of pyrosequencing. Fungal Divers. 49:113–123.
- Linaldeddu BT, Franceschini A, Alves A, Phillips AJ. 2013. Diplodia quercivora sp. nov.: a new species of Diplodia found on declining Quercus canariensis trees in Tunisia. Mycologia. 105:1266–1274.
- Lindahl BD, Nilsson RH, Tedersoo L, Abarenkov K, Carlsen T, Kjoller R, Koljalg U, Pennanen T, Rosendahl S, Stenlid J, et al. 2013. Fungal community analysis by high-throughput sequencing of amplified markers – a user’s guide. New Phytol. 199:288–299.
- Lynch SC, Eskalen A, Zambino P, Scott T. 2010. First report of bot canker caused by Diplodia corticola on coast live oak (Quercus agrifolia) in California. Plant Dis. 94:1510.
- Lyon R, Correll J, Feng C, Bluhm B, Shrestha S, Shi A, Lamour K. 2016. Population structure of Peronospora effusa in the southwestern United States. PloS One. 11:e0148385.
- Malacrinò A, Rassati D, Schena L, Mehzabin R, Battisti A, Palmeri V. 2017. Fungal communities associated with bark and ambrosia beetles trapped at international harbours. Fungal Ecol. 28:44–52.
- Martin DKH, Turcotte RM, Miller TM, Munck IA, Aćimović SG, Macias AM, Stauder CM, Kasson MT. 2017. First report of Diplodia corticola causing stem cankers and associated vascular occlusion of northern red oak (Quercus rubra) in West Virginia. Plant Dis. 101:380.
- Masella AP, Bartram AK, Truszkowski JM, Brown DG, Neufeld JD. 2012. PANDAseq: paired-end assembler for Illumina sequences. BMC Bioinformatics. 13:31.
- Miller KE, Hopkins K, Inward DJ, Vogler AP. 2016. Metabarcoding of fungal communities associated with bark beetles. Ecol Evol. 6:1590–1600.
- Munck IA, Wyka SA, Bohne MJ, Green WJ, Siegert NW. 2017. First report of Diplodia corticola causing bleeding cankers on black oak (Quercus velutina). Plant Dis. 101:257.
- Nilsson RH, Kristiansson E, Ryberg M, Hallenberg N, Larsson KH. 2008. Intraspecific ITS variability in the kingdom Fungi as expressed in the international sequence databases and its implications for molecular species identification. Evol Bioinform. 4:193–201.
- Nilsson RH, Tedersoo L, Lindahl BD, Kjøller R, Carlsen T, Quince C, Abarenkov K, Pennanen T, Stenlid J, Bruns T, et al. 2011. Towards standardization of the description and publication of next-generation sequencing datasets of fungal communities. New Phytol. 191:314–318.
- Penton CR, St. Louis D, Cole JR, Luo Y, Wu L, Schuur EA, Zhou J, Tiedje JM. 2013. Fungal diversity in permafrost and tallgrass prairie soils under experimental warming conditions. Appl Environ Microbiol. 79:7063–7072.
- Prigigallo MI, Abdelfattah A, Cacciola SO, Faedda R, Sanzani SM, Cooke DE, Schena L. 2016. Metabarcoding analysis of Phytophthora diversity using genus-specific primers and 454 pyrosequencing. Phytopathology. 106:305–313.
- Saitou N, Nei M. 1987. The neighbor-joining method: a new method for reconstructing phylogenetic trees. Mol Biol Evol. 4:406–425.
- Schloss PD, Westcott SL, Ryabin T, Hall JR, Hartmann M, Hollister EB, Lesniewski RA, Oakley BB, Parks DH, Robinson CJ, et al. 2009. Introducing Mothur: open-source, platform-independent, community-supported software for describing and comparing microbial communities. Appl Environ Microbiol. 75:7537–7541.
- Schoch CL, Seifert KA, Huhndorf S, Robert V, Spouge JL, Lévesque CA, Chen W. 2012. Fungal Barcoding Consortium. Nuclear ribosomal internal transcribed spacer (ITS) region as a universal DNA barcode marker for Fungi. Proc Natl Acad Sci USA. 109:6241–6246.
- Smith DR, Stanosz GR. 2018. Occurrence of Diplodia corticola, including new oak host records, in Wisconsin, USA. For Pathol. p.e12427. https://doi.org/10.1111/efp.12427.
- Tremblay ED, Duceppe MO, Bérubé JA, Kimoto T, Lemieux C, Bilodeau GJ. 2018. Screening for exotic forest pathogens to increase survey capacity using metagenomics. Phytopathology. https://doi.org/10.1094/PHYTO-02-18-0028-R.
- Úrbez-Torres JR, Adams P, Kamas J, Gubler WD. 2009. Identification, incidence, and pathogenicity of fungal species associated with grapevine dieback in Texas. Am J Enol Vitic. 60:497–507.
- Úrbez-Torres JR, Peduto F, Rooney-Latham S, Gubler WD 2010. First report of Diplodia corticola causing grapevine (Vitis vinifera) cankers and trunk cankers and dieback of canyon live oak (Quercus chrysolepis) in California. Plant Dis. 94:785.
- Wu L, Wen C, Qin Y, Yin H, Tu Q, Van Nostrand JD, Zhou J. 2015. Phasing amplicon sequencing on Illumina Miseq for robust environmental microbial community analysis. BMC Microbiol. 15:125.