Abstract
Diseases caused by pathogens are one of the most important stress factors that affect crop yield and quality and plants have evolved a complex network of responses to infection. Tomato UV-damaged DNA-binding protein-1 (DDB1) has been implicated in playing a role in resistance against Agrobacterium infection. However, the effect of DDB1 protein on plant disease resistance is still largely unknown. Pseudomonas syringae pv. tomato DC3000 (Pst DC3000) is an important pathogen of tomato and has been used as a model for testing disease susceptibility. In this work, we compared the sensitivity to Pst DC3000 among wild-type seedlings AC+, DDB1-deficient mutants (hp1) and DDB1 over-expression (DDB1-OE) seedlings. Results showed that the hp1 mutant is more susceptible to Pst DC3000, whereas the resistance was increased in the DDB1-OE seedlings. Hydrogen peroxide, the key component of the plant defence response and the corresponding cell death phenotype were compromised in the hp1 mutant and enhanced in the DDB1-OE plants. Consistent with that, salicylic acid production and PR1a1 gene expression were positively regulated by the DDB1 gene upon infection with Pst DC3000 at 24 h after inoculation. Other pathogenesis-related genes (Gras2, Lrr22, Pti5, Wrky28 and mitogen-activated protein kinase 3) were also induced in the DDB1-OE plants but compromised to different degrees in the hp1 mutant. Overall, this study suggests that DDB1 plays a regulatory role in tomato defence responses likely through modulating SA-associated signalling pathways.
Résumé
Les maladies causées par des agents pathogènes sont un des plus importants facteurs de stress qui influencent le rendement et la qualité des cultures, et les plantes ont développé un réseau complexe de réactions à l’infection. La protéine-1 liée à l’ADN (DDB1) de la tomate, endommagé par le rayonnement UV, a joué un rôle dans la résistance à l’infection causée par Agrobacterium. Toutefois, l’effet de la protéine DDB1 sur la résistance de la plante à la maladie est largement inconnu. Pseudomonas syringae pv. tomate DC3000 (Pst DC3000) est un agent pathogène important de la tomate et il a été utilisé comme modèle afin de tester la susceptibilité aux maladies. Dans cette étude, nous avons comparé la sensibilité à Pst DC3000 de plantules sauvages AC+, de mutants déficients en DDB1 (hp1) et de plantules affichant une surexpression de la DDB1 (DDB1-OE). Les résultats ont montré que le mutant hp1 est plus réceptif à l’égard de Pst DC3000, tandis que, chez les plantules DDB1-OE, la résistance était accrue. Le peroxyde d’hydrogène, le composant clé du mécanisme de défense de la plante, et le phénotype de mort cellulaire correspondant ont été compromis chez le mutant hp1 et accrus chez les plantules DDB1-OE. En concordance à cela, la production d’acide salicylique et l’expression du gène PR1a1 ont été positivement régulées par le gène de la DDB1 lors de l’infection causée par Pst DC3000, 24 heures après l’inoculation. D’autres gènes liés à la pathogenèse (Gras2, Lrr22, Pti5, Wrky28 et protéine kinase 3 activée par des agents mitogènes) ont aussi été induits dans les plantules DDB1-OE, mais compromis à différents degrés chez les mutants hp1. En général, cette étude suggère que la DDB1 joue un rôle de régulation dans les mécanismes de défense de la tomate, probablement en modulant les voies de signalisation associées à l’acide salicylique.
Introduction
Plants possess multiple defence signalling pathways to recognize and prevent pathogen invasion (Jones & Dangl, Citation2006). Microbe patterns such as flagellin, peptidoglycan, and chitin can induce a basal defence known as pattern triggered immunity (PTI). Pattern recognition receptors mediate microbial pattern recognition and plant immune activation (Albert et al., Citation2015). This leads to the activation of a series of cellular events to eventually prevent pathogen colonization, including callose deposition in the cell wall, reactive oxygen species (ROS) accumulation, and induction of plant pathogenesis-related gene induction (Segonzac & Zipfel, Citation2011; Kadota et al., Citation2015). Generally, PTI is effective in preventing the invasion by most microorganisms. However, some pathogens can secrete defence-suppressing effector proteins into the apoplastic space or cytoplasm to overcome PTI (Chisholm et al., Citation2006). In response, plants have evolved effector-triggered immunity (ETI) to cope with specific effectors released by pathogens (Jones & Dangl, Citation2006; Tsuda et al., Citation2009). Numerous resistance proteins are expressed to detect the pathogen effectors before a hypersensitive response is induced that leads to plant cell death. Consequently, the pathogen growth is retarded (Jones & Dangl, Citation2006).
It has been suggested that several hormone molecules, including salicylic acid (SA), jasmonic acid (JA) and ethylene regulate the plant immune response. Among them, SA regulates defence responses associated with biotrophic pathogen infection, whereas JA and ethylene mediate resistance against necrotrophic pathogens (Halim et al., Citation2009; Yang et al., Citation2015). The induction of PR-1 has been confirmed as a marker gene for the SA signalling pathway (Durrant & Dong, Citation2004). Pseudomonas syringae pv. tomato DC3000 (Pst DC3000) has been used as a model pathogen for testing disease susceptibility and hormone signalling transduction in plants, both in its natural host tomato (Solanum lycopersicum), but also in Arabidopsis thaliana (Xin & He, Citation2013). It is known that SA is vital for promoting disease resistance in response to Pst DC3000 (Agorio & Vera, Citation2007). Accumulation of SA at infection sites can diffuse in plant tissues and lead to systemic resistance against Pst DC3000 throughout the plant. This phenomenon is called systemic acquired resistance (Durrant & Dong, Citation2004).
Tomato is the one of the most important vegetable crops worldwide and is also an experimentally important crop (Kimura & Sinha, Citation2008). A high pigment mutant (high pigment-1, hp1) with exaggerated photo responsiveness and elevated pigmentation has been described in tomato (Kendrick et al., Citation1997). The HIGH P1GMENT-1 gene encodes UV-damaged DNA-Binding Protein-1 (DDB1) (Liu et al., Citation2004) that was initially identified as an important component of a UV-induced DNA damage repairing complex. Later, studies revealed that DDB1 is also a component of more than 90 ubiquitin-E3 ligases and functions as a substrate or adapter protein between Cullin 4A (CUL4A) and CUL4-associated factors to target substrates in many cellular processes, including cell cycling (Roodbarkelari et al., Citation2010), ABA signal transduction (Lee et al., Citation2010) and epigenetic regulation (Pazhouhandeh et al., Citation2011). A previous study indicated that the hp1 mutant is more susceptible to Agrobacterium tumefaciens infection compared with the wild-type (Liu et al., Citation2012a). However, the role of DDB1 in plant defence responses is still largely unclear. In this study, we inoculated the tomato wild-type ‘Ailsa Craig’ plus (AC+), hp1 mutant and DDB1 over-expression (DDB1-OE) plants with Pst DC3000 to identify the function of DDB1 in tomato defence responses.
Materials and methods
Plants and growth conditions
Tomato plants, including AC+, hp1 mutant and DDB1-OE were used in this study. The DDB1-OE plant was constructed previously (Liu et al., Citation2012b) and had been identified as homozygous. All plants were grown in a greenhouse under diffused daylight and temperature range of 22–26°C with 16 h light (100 μmol m−2 s−1) and 8 h dark.
Pathogen inoculation
Pst DC3000 (virulent) was cultured at 28°C in King’s B medium (Zhao et al., Citation2013) for 48 h. Cells were harvested by centrifugation at 5000 × g for 5 min, resuspended to 1 × 108 colony-forming units (cfu) mL−1 in 10 mM MgCl2 with 0.006% Silwet L-77. Eight-week-old plants were sprayed with the suspension and incubated at 26°C in an incubator (MLR-352H, SANYO, Japan) with a relative humidity of 90%. The plants treated with 10 mM MgCl2 served as the mock inoculation.
Bacterial growth assay
About 0.2 g of leaf samples were collected at 1, 3, 5, 7 days post-inoculation (dpi) to determine cfu mL−1 of Pst DC3000. Samples were accurately weighed and homogenized in 2 mL of 10 mM MgCl2. The suspension was diluted and plated on King’s B medium containing 50 µg mL−1 of rifampicin. The plates were kept at 28°C for 2 days and the number of bacteria per gram fresh weight (cfu g−1 FW) was counted. Each treatment contained three replicates with five plants per replicate; the experiment was repeated three times.
Chemical treatments
Leaf samples were collected at 48 h post inoculation (hpi) for hydrogen peroxide (H2O2) detection and dead cell staining. The accumulation of H2O2 in AC+, hp1 mutant and DDB1-OE plants was detected by 3,3′-diaminobenzidine (DAB) staining as described by Thordal-Christensen et al. (Citation1997). Trypan blue staining was performed to detect the lesion sites using lactic acid-phenol-trypan blue solution as described by Bowling et al. (Citation1997). Each treatment contained three replicates with five plants per replicate; and the experiment was repeated three times.
SA content determination and gene expression analysis by qRT-PCR
For SA content determination, leaf samples were collected at 24 hpi. Free SA accumulation was extracted and analysed by HPLC according to López-Gresa et al. (Citation2016); plants treated with 10 mM MgCl2 served as the mock inoculation group. For gene expression analysis, leaf samples were collected at 0, 6, 24 and 48 hpi. Total mRNA was extracted using Trizol reagent (Invitrogen, Carlsbad, CA) according to the supplier’s instructions. About 100 ng of mRNA was used for cDNA synthesis. Real-time qRT-PCR was performed using the cDNA; the gene-specific primers used are presented in . A Step ONE Plus Real-Time PCR System (Applied Biosystems) was applied for the qRT-PCR. PCR was performed using SYBR Green PCR Master Mix (Transgen, Beijing, China). The PCR conditions were as follows: 95°C for 30 s, followed by 40 cycles of 95°C for 5 s and 60°C for 15 s. A melting curve analysis of amplification products was performed at the end of the PCR reaction. The melting cycle was 95°C for 15 s, 60°C for 1 min and 95°C for 15 s. The UBI3 gene was used as the internal control, using the 2−ΔΔCT method according to Livak & Schmittgen (Citation2001). Each treatment contained three replicates with 5 plants per replicate, and the experiment was repeated three times.
Table 1. Primers used in qRT-PCR reactions in the present study.
Statistical analysis
All statistical analyses were performed using SAS 9.2 (Cary, NC). Data were analysed by one-way analysis of variance. Mean separations were performed by Duncan’s multiple range tests. Least square means (LSMEANS) and standard errors of least-squares means (SEM) were calculated using the LSMEANS. Differences at P < 0.05 were considered significant.
Results and discussion
Effect of DDB1 on disease resistance
To test whether DDB1 contributes to the basal defence against virulent pathogens, AC+, hp1 and DDB1-OE plants were inoculated with Pst DC3000. Three days after inoculation, plants began to develop disease symptoms; these were visibly more severe on the hp1 mutant compared with AC+ or DDB1-OE. At 7 dpi, there were more spots on hp1 leaves than on AC+. Conversely, symptoms on DDB1-OE plants were relatively moderate (). Additionally, the bacteria growth assessment showed that the bacteria number increased gradually in all plants tested within 7 dpi () and was higher in the hp1 mutant (P < 0.05) and lower in DDB1-OE compared with AC+ (P < 0.05).
Fig. 1 (Colour online) Disease symptoms and bacterial growth in leaves of ‘Ailsa Craig’ plus (AC+), hp1 mutant and DDB1 over-expression (DDB1-OE) plants upon infection with Pst DC3000. Photographs were taken at 7 dpi (A). Bacterial growth was determined at 0, 3, 5 and 7 dpi (B). Values followed by different letters differ significantly according to Duncan’s multiple range test at P < 0.05. FW, fresh weight; dpi, days post inoculation; * (P < 0.05) indicated significant differences from AC+.
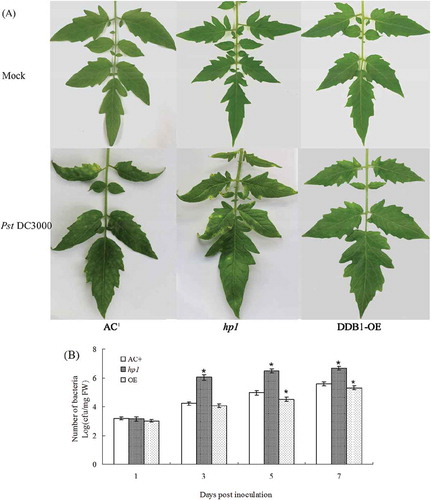
In a previous study, the hp1 mutant was observed to be more susceptible to A. tumefaciens GV2260 and EHA105 than the wild-type AC+ (Liu et al., Citation2012a), consistent with our results using Pst DC3000. The slower bacterial growth and milder symptoms on DDB1-OE plants suggested that DDB1 was required for plant resistance against bacterial infection. The loss of function of DDB1 facilitated the Pst DC3000 infection, while over-expression of DDB1 contributed to the increased level of disease resistance.
Effect of DDB1 on H2O2 accumulation in tomato leaves
H2O2, the most stable ROS, has been implicated in plant responses to Pst DC3000 (Parker et al., Citation2013). In our study, the production of H2O2 in AC+, hp1 mutant and DDB1-OE leaves was compared after Pst DC3000 inoculation. The DAB staining assay showed that, upon Pst DC3000 inoculation, less H2O2 accumulation was detected in the hp1 mutant whereas more H2O2 accumulation was observed in the DDB1-OE plants compared with AC+ (). ROS are known to be important secondary messengers that induce the expression of pathogenesis-related genes (Foyer & Noctor, Citation2005). The burst of H2O2 can lead to cell damage or even cell death that would help to suppress the spread of pathogens and result in enhanced disease resistance (Foyer & Noctor, Citation2005). The ROS accumulation and cell death phenotypes were compromised in the hp1 plants and enhanced in DDB1-OE plants, suggesting that DDB1 affects the ROS-mediated responses to tomato-pathogen interactions.
Fig. 2 (Colour online) Hydrogen peroxide (H2O2) accumulation and cell death in leaves of Ailsa Craig plus (AC+), hp1 mutant and DDB1 over-expression (DDB1-OE) plants upon infection with Pst DC3000. The accumulation of H2O2 was detected by 3,3′-diaminobenzidine (DAB) staining (A) and the cell death phenotype was assayed by trypan blue staining (B) at 48 dpi. Plants were sprayed with 10 mM MgSO4 (mock, top) or Pst DC3000 at 1 × 108 cfu mL−1 (bottom). dpi, day post inoculation. Bar = 100 μm.
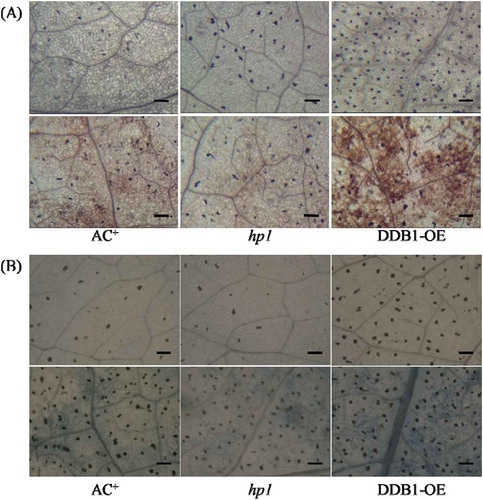
DDB1 is required for the SA signalling pathway
The free SA content in AC+ and DDB1-OE plants increased significantly (P < 0.05) after Pst DC3000 inoculation but did not change in the hp1 mutant. At 24 hpi, SA was significantly higher in DDB1-OE plants than in AC+ (P < 0.05; ). This result revealed that the SA signalling pathway was disrupted in the hp1 mutant and promoted in DDB1-OE plants. Liu et al. (Citation2012a) observed that exogenous SA treatment could not induce defence responses in the hp1 mutant. As a well-known marker gene in the SA signalling pathway, PR1a1 expression was determined at different time points after Pst DC3000 inoculation. This showed that PR1a1 was significantly induced in AC+ (P < 0.01) and DDB1-OE (P < 0.05) plants at 24 hpi (P < 0.05); in the hp1 mutant, it was also significantly higher than at 0 hpi (P < 0.01), although this increase was only 2-fold and was notably lower than that observed in AC+ or DDB1-OE (P < 0.05; ). This confirmed that DDB1 is positively involved in the SA signalling pathway upon bacterial infection in tomato.
Fig. 3 Salicylic acid (SA) induction in ‘Ailsa Craig’ plus (AC+), hp1 mutant and DDB1 over-expression (DDB1-OE) plants. Free SA production (A) was determined in leaves sprayed with 10 mM MgSO4 (mock) or Pst DC3000 at 1 × 108 cfu mL−1 at 24 hpi. Expression levels of the marker gene PR1a1 (B) were determined in leaves sprayed with Pst DC3000 at 1 × 108 cfu mL−1 at 0, 6, 24 and 48 hpi. Values followed by different letters differ significantly according to Duncan’s multiple range test at P < 0.05. FW, fresh weight; hpi, hours post inoculation; ** (P < 0.01) or * (P < 0.05) indicate significant differences from mock (A) or 0 hpi (B).
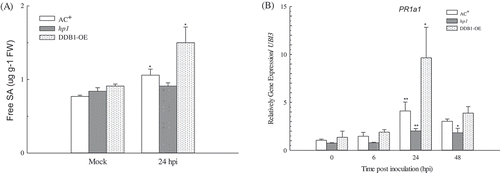
Fig. 4 Expression patterns of pattern-triggered immunity (PTI)-related genes in ‘Ailsa Craig’ plus (AC+), hp1 mutant, and DDB1 over-expression (DDB1-OE) leaves. The expression levels of Gras2, Lrr22, Pti5 and Wrky28 were determined in leaves sprayed with Pst DC3000 at 1 × 108 cfu mL−1 at 0, 6, 24 and 48 hpi. Values followed by different letters differ significantly according to Duncan’s multiple range test at P < 0.05. hpi, hour post inoculation; ** (P < 0.01) or * (P < 0.05) indicate significant differences from AC+.
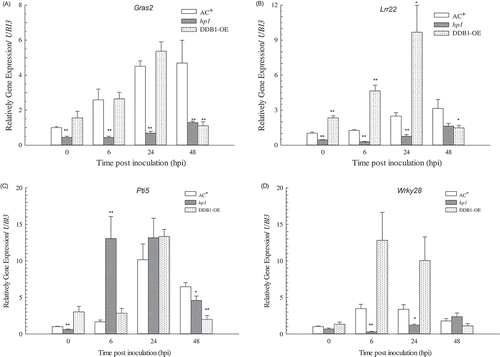
Salicylic acid is known to enhance the generation of ROS (Chen et al., Citation1993) and ROS, in turn, increases endogenous SA content (Enyedi, Citation1999). Therefore, the suppression of H2O2 generation in the hp1 mutant might be associated with SA transduction.
It has been suggested that SA caused DNA breaks after Pst DC3000 infection, leading to defence-related gene expression during DNA repair (Yan et al., Citation2013). Song & Bent (Citation2014) also observed that Pst DC3000 triggered DNA breaks in Arabidopsis. DDB1 was identified as a damaged DNA binding protein that formed an E3–ubiquitin ligase complex with another WD40 repeat-containing protein to recognize DNA lesions and initiate DNA repair in plants and animals (Iovine et al., Citation2011). Therefore, the mediation of the SA signalling pathway and defence response regulation by DDB1 in tomato might be related to DNA breakage and repair upon pathogen infection.
Effect of DDB1 on the expression of pathogenesis-related genes
The expression levels of several marker genes involved in the tomato PTI pathway, including Gras2, Lrr22, Wrky28 and Pti5 were determined previously (Nguyen et al., Citation2010; Liu et al., Citation2012a). The expression level of Gras2 was induced at 6 hpi in both AC+ and DDB1-OE plants in this study, but not in the hp1 mutant. A similar phenomenon was observed for Wrky28 and Lrr22 gene expression. These genes were, however, expressed notably more in DDB1-OE plants than in AC+ plants. Unlike the other genes detected, Pti5 was also up-regulated approximately 13-fold in the hp1 mutant at 6 hpi compared with the base level (P < 0.01), and was reduced at 48 hpi (). The expression pattern was similar to that induced by Agrobacterium (Liu et al., Citation2012a). These results showed that DDB1 played a role in the PTI signalling pathway and that PTI signalling was not totally abolished in the hp1 mutant. This might be associated with a partial loss of function of DDB1 in the hp1 mutant, as DDB1 is essential for normal development. In mouse and Arabidopsis model systems, the total deletion of DDB1 would be lethal in the early embryo stage (Cang et al., Citation2006; Bernhardt & Hellmann, Citation2010).
Mitogen-activated protein kinase 3 (MPK3) is induced in DDB1-OE plants
MAPK cascades are highly conserved signalling mechanisms in eukaryotes that play essential roles in response to multiple environmental stresses (Pitzschke, Citation2009). Several MAPKs, including MPK3, MPK6 and MPK4, are activated in Arabidopsis upon Pst DC3000 infection (Brader et al., Citation2007; Beckers et al., Citation2009). MPK3 and MPK6, which are functionally redundant and have a positive role in plant immunity responses, are two of the most important MAPK genes (Pitzschke, Citation2009). In this study, the expression level of MPK3 in tomato seedlings was determined through qRT-PCR. This showed that MPK3 was rapidly reduced in DDB1-OE plants at 6 hpi (P < 0.01); in AC+ plants, MPK3 was induced approximately 2.5-fold compared with the base level (P < 0.05) at 6 hpi (). In the hp1 mutant, it was not induced (P > 0.05; ), suggesting that the MAPK signalling pathway was compromised in the hp1 mutant. It was reported that MPK3 could be induced by both ROS and SA and leads to enhanced disease resistance (Miura & Tada, Citation2014; Jalmi & Sinha, Citation2015), whereas H2O2 and SA production in the hp1 mutant were compromised during infection. Therefore, we presumed that the tomato DDB1 protein might affect the MAPK signalling pathway through the mediation of ROS accumulation and that this was also associated with SA production. However, the interactions among DDB1, ROS, SA, and MAPK are complicated and require further investigation.
Fig. 5 Expression patterns of Mitogen-activated protein kinase 3 (MPK3) in ‘Ailsa Craig’ plus (AC+), hp1 mutant and DDB1 over-expression (DDB1-OE) plants. Gene expression levels were determined in leaves sprayed with Pst DC3000 at 1 × 108 cfu mL−1 at 0, 6, 24 and 48 hpi. Values followed by different letters differ significantly according to Duncan’s multiple range test at P < 0.05. hpi, hours post inoculation; ** (P < 0.01) or * (P < 0.05) indicate significant differences from AC+.
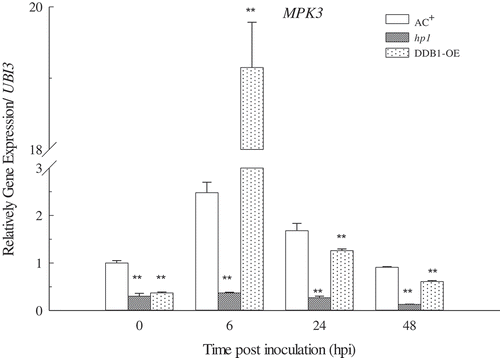
In conclusion, DDB1 played a positive role in tomato defence response. ROS accumulation, SA production, several PTI marker genes, and MPK3 expression were compromised in a DDB1 function-deficient mutant and enhanced in the DDB1-OE plants.
Acknowledgements
We thank Dr Emma Tacken from Edanz Group (www.edanzediting.com/ac) for editing a draft of this manuscript.
Additional information
Funding
References
- Agorio A, Vera P. 2007. ARGONAUTE4 is required for resistance to pseudomonas syringae in arabidopsis. Plant Cell. 19:3778–3790.
- Albert I, Böhm H, Albert M, Feiler CE, Imkampe J, Wallmeroth N, Brancato C, Raaymakers TM, Oome S, Zhang H, et al. 2015. An RLP23-SOBIR1-BAK1 complex mediates NLP-triggered immunity. Nat Plants. 1:15140.
- Beckers GJM, Jaskiewicz M, Liu Y, Underwood WR, He SY, Zhang S, Conrath U. 2009. Mitogen-activated protein kinases 3 and 6 are required for full priming of stress responses in Arabidopsis thaliana. Plant Cell. 21:944–953.
- Bernhardt A, Hellmann H. 2010. Arabidopsis DDB1a and DDB1b are critical for embryo development. Planta. 232:555–566.
- Bowling SA, Clarke JD, Liu Y, Klessig DF, Dong X. 1997. The cpr5 mutant of Arabidopsis expresses both NPR1-dependent and NPR1-independent resistance. Plant Cell. 9:1573–1584.
- Brader G, Djamei A, Teige M, Palva ET, Hirt H. 2007. The MAP kinase kinase MKK2 affects disease resistance in Arabidopsis. Mol Plant-Microbe Interact. 20:589–596.
- Cang Y, Zhang J, Nicholas SA, Bastien J, Li B, Zhou P, Goff SP. 2006. Deletion of DDB1 in mouse brain and lens leads to p53-dependent elimination of proliferating cells. Cell. 127:929–940.
- Chen Z, Silva H, Klessig DF. 1993. Active oxygen species in the induction of plant systemic acquired resistance by salicylic acid. Science. 262:1883–1886.
- Chisholm ST, Coaker G, Day B, Staskawicz BJ. 2006. Host-microbe interactions: shaping the evolution of the plant immune response. Cell. 124:803–814.
- Durrant WE, Dong X. 2004. Systemic acquired resistance. Annu Rev Phytopathol. 42:185–209.
- Enyedi AJ. 1999. Induction of salicylic acid biosynthesis and systemic acquired resistance using the active oxygen species generator rose bengal. J Plant Physiol. 154:106–112.
- Foyer CH, Noctor G. 2005. Oxidant and antioxidant signalling in plants: a re-evaluation of the concept of oxidative stress in a physiological context. Plant Cell Environ. 28:1056–1071.
- Halim VA, Altmann S, Ellinger D, Eschen-Lippold L, Miersch O, Scheel D, Rosahl S. 2009. PAMP-induced defense responses in potato require both salicylic acid and jasmonic acid. Plant J. 57:230–242.
- Iovine B, Iannella ML, Bevilacqua MA. 2011. Damage-specific DNA binding protein 1 (DDB1): a protein with a wide range of functions. Int J Biochem Cell Biol. 43:1664–1667.
- Jalmi SK, Sinha AK. 2015. ROS mediated MAPK signaling in abiotic and biotic stress – striking similarities and differences. Front Plant Sci. 6:1–9.
- Jones JDG, Dangl JL. 2006. The plant immune system. Nature. 444:323–329.
- Kadota Y, Shirasu K, Zipfel C. 2015. Regulation of the NADPH oxidase RBOHD during plant immunity. Plant Cell Physiol. 56:1472–1480.
- Kendrick RE, Peters JL, Kerckhoffs LH, Van Tuinen A, Koornneef M. 1997. Photomorphogenic mutants of tomato. Biochem Soc Symp. 20:746–751.
- Kimura S, Sinha N. 2008. Tomato (Solanum lycopersicum): A model fruit-bearing crop. Cold Spring Harbor Protoc. 3:1–9.
- Lee JH, Yoon HJ, Terzaghi W, Martinez C, Dai M, Li J, Byun MO, Deng XW. 2010. DWA1 and DWA2, two Arabidopsis DWD protein components of CUL4-based E3 ligases, act together as negative regulators in ABA signal transduction. Plant Cell. 22:1716–1732.
- Liu J, Li H, Miao M, Tang X, Giovannoni J, Xiao F, Liu Y. 2012a. The tomato UV-damaged DNA-binding protein-1 (DDB1) is implicated in pathogenesis-related (PR) gene expression and resistance to Agrobacterium tumefaciens. Mol Plant Pathol. 13:123–134.
- Liu J, Tang X, Gao L, Gao Y, Li Y, Huang S, Sun X, Miao M, Zeng H, Tian X, et al. 2012b. A role of tomato UV-damaged DNA binding protein 1 (DDB1) in organ size control via an epigenetic manner. PLoS One. 7:e42621.
- Liu Y, Roof S, Ye Z, Barry C, Van Tuinen A, Vrebalov J, Bowler C, Giovannoni J. 2004. Manipulation of light signal transduction as a means of modifying fruit nutritional quality in tomato. Proc Natl Acad Sci USA. 101:9897–9902.
- Livak KJ, Schmittgen TD. 2001. Analysis of relative gene expression data using real-time quantitative PCR and the 2–ΔΔCt method. Methods. 25:402−408.
- López-Gresa MP, Lisón P, Yenush L, Conejero V, Rodrigo I, Bellés JM. 2016. Salicylic acid is involved in the basal resistance of tomato plants to Citrus exocortis viroid and tomato spotted wilt virus. PLoS One. 11:e0166938.
- Miura K, Tada Y. 2014. Regulation of water, salinity, and cold stress responses by salicylic acid. Front Plant Sci. 5:1–12.
- Nguyen HP, Chakravarthy S, Velásquez AC, McLane HL, Zeng L, Nakayashiki H, Park DH, Collmer A, Martin GB. 2010. Methods to study PAMP-triggered immunity using tomato and Nicotiana benthamiana. Mol Plant-Microbe Interact. 23:991–999.
- Parker J, Koh J, Yoo MJ, Zhu N, Feole M, Yi S, Chen S. 2013. Quantitative proteomics of tomato defence against Pseudomonas syringae infection. Proteomics. 13:1934–1946.
- Pazhouhandeh M, Molinier J, Berr A, Genschik P. 2011. MSI4/FVE interacts with CUL4-DDB1 and a PRC2-like complex to control epigenetic regulation of flowering time in Arabidopsis. Proc Natl Acad Sci USA. 108:3430–3435.
- Pitzschke A, Schikora A, Hirt H. 2009. MAPK cascade signalling networks in plant defence. Curr Opin Plant Biol. 12:421–426.
- Roodbarkelari F, Bramsiepe J, Weinl C, Marquardt S, Novák B, Jakoby MJ, Lechner E, Genschik P, Schnittger A. 2010. Cullin 4-ring finger-ligase plays a key role in the control of endoreplication cycles in Arabidopsis trichomes. Proc Natl Acad Sci USA. 107:15275–15280.
- Segonzac C, Zipfel C. 2011. Activation of plant pattern-recognition receptors by bacteria. Curr Opin Microbiol. 14:54–61.
- Song J, Bent AF. 2014. Microbial pathogens trigger host DNA double-strand breaks whose abundance is reduced by plant defence responses. PLoS Pathog. 10:e1004226.
- Thordal-Christensen H, Zhang Z, Wei Y, Collinge DB. 1997. Subcellular localization of H2O2 in plants. H2O2 accumulation in papillae and hypersensitive response during the barley-powdery mildew interaction. Plant J. 11:1187–1194.
- Tsuda K, Sato M, Stoddard T, Glazebrook J, Katagiri F. 2009. Network properties of robust immunity in plants. PLoS Genet. 5:e1000772.
- Xin XF, He SY. 2013. Pseudomonas syringae pv. tomato DC3000: a model pathogen for probing disease susceptibility and hormone signaling in plants. Annu Rev Phytopathol. 51:473–498.
- Yan S, Wang W, Marqués J, Mohan R, Saleh A, Durrant WE, Song J, Dong X. 2013. Salicylic acid activates DNA damage responses to potentiate plant immunity. Mol Cell. 52:1–18.
- Yang YX, Ahammed GJ, Wu C, Fan SY, Zhou YH. 2015. Cross talk among jasmonate, salicylate and ethylene signaling pathways in plant disease and immune responses. Curr Protein Pept Sci. 16:450–461.
- Zhao J, Devaiah SP, Wang C, Li M, Welti R, Wang X. 2013. Arabidopsis phospholipase Dβ1 modulates defense responses to bacterial and fungal pathogens. New Phytol. 199:228–240.