Abstract
Stripe rust (yellow rust), caused by Puccinia striiformis Westend. f. sp. tritici (Pst), is one of the most destructive fungal disease in wheat(Triticum aestivum L.). Growing resistant cultivars is the best strategy to control stripe rust. The wheat introgression line H9015-17–1-9–6 was developed from an interspecific hybridization between the common wheat cultivar 7182 and Psathrostachys huashanica Keng (2n = 2x = 14, NsNs). H9015-17–1-9–6 has all-stage resistance to all known Chinese stripe rust races, including three widely virulent races: CYR32, CYR33 and CYR34. To identify stripe rust resistance genes in the introgression line, H9015-17–1-9–6 was crossed with the susceptible genotype ‘Mingxian169' and the F1, F2, F3 and BC1 generations were inoculated with Pst races under controlled greenhouse conditions. The results indicated that a single dominant gene in H9015-17–1-9–6, temporarily designated as YrH9015, confers resistance to race CYR32. Using bulked segregant analysis methods, we identified seven resistance gene analogue polymorphism (RGAP) markers and three simple sequence repeat (SSR) markers associated with YrH9015 on the long arm of chromosome 5D. The genetic distances of the two closest flanking markers, namely M4 and M5, were 2.4 and 3.1 centimorgans, respectively. Based on the chromosomal location, reaction patterns and pedigree analysis, YrH9015 is likely a novel resistance gene. This gene and the flanking markers obtained from this study are expected to be useful in pyramiding YrH9015 with other Yr genes to develop wheat cultivars with high-level and durable resistance to stripe rust and may also benefit marker assisted selection (MAS) in breeding programmes.
Résumé
La rouille jaune, causée par Puccinia striiformis Westend. f. sp. tritici. (Pst), est une des maladies fongiques du blé (Triticum aestivum L.) parmi les plus destructrices. L’utilisation de cultivars résistants est la meilleure stratégie de lutte contre cette maladie. La lignée d’introgression de blé H9015-17-1-9-6 a été développée à partir d’une hybridation interspécifique entre le cultivar de blé commun ‘7182' et Psathrostachys huashanica Keng (2n=2x=14, NsNs). H9015-17-1-9-6 possède la résistance, à tous les stades, et ce, à toutes les races chinoises de rouille jaune, y compris aux trois races communément virulentes suivantes: CYR32, CYR33 et CYR34. Pour distinguer les gènes de résistance à la rouille jaune dans la lignée d’introgression, H9015-17-1-9-6 a été croisée avec le génotype réceptif à l’égard de la rouille ‘Mingxian169', puis les générations F1, F2, F3 et BC1 ont été inoculées avec des races de Pst dans les conditions contrôlées d’une serre. Les résultats ont indiqué qu’un gène dominant unique chez H9015-17-1-9-6, désigné temporairement comme YrH9015, confère la résistance à la race CYR32. En utilisant des méthodes d’analyses de ségrégants regroupés, nous avons décelé sept marqueurs du polymorphisme d’analogues de gènes de résistance (RGAP) et trois marqueurs de type microsatellite (SSR) associés à YrH9015 sur le bras long du chromosome5D. Les distances génétiques des deux marqueurs adjacents les plus proches, à savoir M4 et M5, étaient de 2.4 et 3.1 cM, respectivement. En se basant sur l’emplacement chromosomique, les modèles de réaction et l’analyse du pedigree, YrH9015 constitue, de toute évidence, un nouveau gène de résistance. On s’attend à ce que ce gène et les marqueurs adjacents obtenus dans le cadre de cette étude soient utiles au pyramidage du gene YrH9015 avec d’autres gènes Yr pour développer des cultivars de blé possédant un degré élevé et durable de résistance à la rouille jaune, et de contribuer également à la sélection assistée par marqueurs dans le cadre des programmes de sélection.
Introduction
Stripe rust, caused by Puccinia striiformis Westend. f. sp. tritici (Pst), is one of the most important and destructive fungal diseases in common wheat (Triticum aestivum L.) worldwide (Chen et al., Citation2009; Hovmøller et al., Citation2016; Wang et al., Citation2017). China is the area most widely and seriously damaged by wheat stripe rust epidemics (Chen et al., Citation2013). To date, China has suffered seven nationwide epidemics, which have had a great impact on the wheat yield (Li et al., Citation2011; Juliana et al., Citation2017).
Growing resistant cultivars has generally been accepted as the best strategy to manage stripe rust. About 80 named Yr resistance genes have been reported in wheat, and there are many additional genes and quantitative trait loci (QTLs) with temporary names (McIntosh et al., 2016, Citation2017). Consequently, gene pyramiding, gene deployment and multi-line cultivar strategies are considered useful for protecting and prolonging cultivar resistance. The use of such strategies depends greatly on the effectiveness of the resistance genes. However, few known Yr genes confer effective all-stage resistance against the current pathogen populations worldwide. In some wheat lines, the stripe rust resistance genes lose their effectiveness after a few years of commercial production. The virulence spectrum of races also varies frequently because of the emergence of new Pst variants. In particular, the appearance and increased incidence of the Pst races CYR32, CYR33 and CYR34 in China can cause epidemics. Most commercial and candidate varieties exhibited a loss of their resistance to stripe rust races in China due to the appearance of the new races.
Therefore, new stripe rust resistance genes need to be identified to pyramid genes from different resistance sources to achieve high-level and durable resistance. Wheat landraces and wild wheat relatives are also important resources of stripe rust resistance genes in wheat breeding. Amongst the officially designated Yr genes, some originate from wild wheat relatives. For example, Yr5 and Yr15 discovered in Triticum spelta L. and Triticum dicoccoides, respectively, remain resistant to most prevalent Pst races in China (Macer, Citation1966; Gerechteramitai & Stubbs, Citation1970; Yang et al., Citation2003).
Psathyrostachys huashanica Keng (2n = 2x = 14, NsNs), a perennial cross-pollinating plant and a wheat-relative found only in Huashan Mountain, has been listed as an endangered and protected wild species in China. This plant possesses valuable properties such as winter hardiness, drought tolerance and disease resistance. Therefore, the species is an important resource for wheat breeding. Through interspecific hybridization between wheat line 7182 and P. huashanica, we exploited embryo rescue technology and subsequent extensive backcrossing to develop several wheat translocation lines. Previous research indicated that P. huashanica possesses valuable disease-resistance genes that can be highly expressed in a wheat background (Chen et al., Citation1991; Jing et al., Citation1999).
The F1 hybrid H881 (2n = 28, ABDNs) was selected from wheat line 7182 and P. huashanica by embryo rescue and backcrossed with 7182 to generate line H8911-2. Line H8911-2 was backcrossed with 7182 to produce line H9015, which was backcrossed once more with 7182 (four total backcrosses) to produce wheat-like derivative lines. H9015-17–1-9–6 was isolated from a large number of progenies that showed high resistance to Chinese stripe rust races in seedling tests. The objective of the present study was to identify the stripe rust resistance gene(s) in H9015-17–1-9–6, and use molecular markers to construct genetic and physical maps of the gene(s) for its further application to molecular breeding.
Materials and methods
Plant materials
The introgression line H9015-17–1-9–6, which originates from interspecific hybridization between bread wheat line 7182 (female parent) and P. huashanica (male parent), was created and provided by Professor Jie Fu, Northwest Agricultural & Forestry University, Yangling, China. ‘Mingxian169', a winter wheat cultivar, is highly susceptible to all Pst races in China. As the male parent, H9015-17–1-9–6 was crossed with ‘Mingxian169' to produce F1, F2, F2:3 and BC1 generations to determine the gene number and inheritance for all-stage Yr resistance in H9015-17–1-9–6. The phenotypic data of the 142 F2 plants, confirmed by the F2:3 lines, were used to map the resistance gene(s). In addition, Chinese spring (CS) and 21 CS nulli-tetrasomic (NT) lines (provided by Professor X. M. Chen, US Department Agriculture, Agricultural Research Service, Washington State University) were used to verify the gene location in the study.
Genomic in situ hybridization (GISH) and fluorescence in situ hybridization (FISH)
To detect introgressed alien gene segments from H9015-17–1-9–6, we performed GISH and FISH by using the root chromosomes of H9015-17–1-9–6 as a template and high-quality total genomic DNA of P. huashanica as a probe. Root tips from germinating seeds were squashed in a drop of 45% acetic acid and frozen at −80°C. Total genomic DNA was extracted from fresh leaves of P. huashanica through the cetyl trimethyl ammonium bromide (CTAB) method. GISH preparation and operation were performed as described previously (Ma et al., Citation2016).
Psathyrostachys huashanica DNA was labelled with a digoxigenin-11-dUTP probe by nick translation in accordance with the manufacturer’s protocol (Roche, Mannheim, Germany). Denatured hybridization solution (40%), consisting of six parts corresponding to 29 sodium chloride-sodium (SSC), 0.25% sodium dodecyl sulphate, 10% dextran sulphate, 50% deionized formamide, 0.125l g L−1 salmon sperm DNA (5l g L−1), and 2.5 ng L−1 labelled probe DNA, was covered on each slide. Denaturation was conducted for 10 min at 95°C. After hybridization in a humid Petri dish, post-hybridization washes were performed with 29 SSC buffer. Anti-digoxigenin-fluorescein (Roche, Mannheim, Germany) was used to detect and visualize the labelled chromosomes. FISH using Oligo-p Ta535 (red) and Oligo-p Sc119.2 (green) as probes was applied following previous procedures (Tang et al., Citation2014a, Citation2014b). The chromosome slides were counterstained with 4,6-diamidino-2-phenylindole and washed in 2 × SSC solution before observation. Fluorescence signals were captured using an Olympus BX60 microscope.
Evaluation of stripe rust resistance in greenhouse
To determine the resistance type of H9015-17–1-9–6, 20 plants each of H9015-17–1-9–6, P. huashanica, 7182 and ‘Mingxian169' were tested with 12 single-pustule derived Pst races (CYR23, CYR25, CYR27, CYR29, CYR30, CYR31, CYR32, CYR33, CY34, Su11-4, Su11-11 and CYR29-mut3). Inoculations were performed at the two-leaf stage with fresh urediniospores in controlled environmental conditions in the greenhouse with a 16 h light/8 h dark photoperiod and diurnal temperatures gradually changing from 15°C to 20°C. After inoculations, the seedlings were kept in a chamber at 10°C and 80% relative humidity for 24 h. Infected plants were then moved into the greenhouse with a 16 h light/8 h dark photoperiod and diurnal temperatures gradually changing from 10°C to 17°C. Seedling stripe rust reaction was evaluated using a 0–4 scale for infection type (IT) at 16–17 days after inoculation. Plants with IT 0–2+ and IT 3–4 were considered to be resistant and susceptible, respectively (Zhou et al., Citation2011).
About 10 seeds from each parent, F1, BC1, 142 F2 seeds, and each F2:3 line were planted in 10 cm diameter pots. Seedlings of the parents, F1, F2, BC1 and F2:3 populations were grown in the greenhouse. The F1, BC1, F2 population and F2:3 lines were inoculated with the predominant race CYR32 (Wan et al., Citation2004; Bai et al., Citation2014). IT data were recorded as described above.
DNA extraction and bulk segregant analysis
Seedling leaf DNA was extracted by the CTAB procedure and quantified by spectrophotometry in this study (Rogers & Bendich, Citation1985). The mapping population was constructed using F2 plants inoculated with CYR32. Resistant bulks (BR) and susceptible bulks (BS), consisting of equal amounts of DNA from 15 resistant (IT = 0) and 15 susceptible (IT = 4) F2 plants, respectively, were constructed for bulked segregant analysis (Michelmore et al., Citation1991). Each plant used to construct the bulks was subsequently inoculated using corresponding F2:3 lines to verify the F2 genotypes.
Molecular marker screen and segregation analysis
Resistance gene analogue polymorphism (RGAP) and simple sequence repeats (SSR) markers were used to map resistance gene YrH9015 using the bulk segregant analysis (BSA) method in the F2 mapping population. Using the F2 plant phenotype, a total of 1680 RGAP markers were developed. These markers, identified in two bulks, were tested for polymorphism on the parents and then used to screen the F2 population. In this study, 48 RGAP markers were designed based on the conserved domain of cloned plant disease resistance genes (Chen et al., Citation1998; Shi et al., Citation2001) and 27 other RGAP markers were designed by Dr Meinan Wang and synthesized by Invitrogen (Ren et al., Citation2012; Zhou et al., Citation2014). In addition, 182 SSR markers were synthesized by Shanghai Generay Biotech Co (Shanghai, China) on the basis of the sequences published on the GrainGenes2.0 website (https://wheat.pw.usda.gov/GG3/).
PCR reactions were performed in a PTC-200 thermocycler (MJ Research, USA). The total volume of the PCR reaction was 15 μL, and consisted of 45 ng template DNA; 1.5 μL of Mg-free 10× PCR buffer; 5 mM MgCl2; 2.5 mM each of dCTP, dGTP, dTTP and dATP; and 1.5 μM of each primer; 0.6 unit of Taq DNA Polymerase; and 5.8 μL of ddH2O. The cycling conditions were as follows: after 5 min of denaturation at 94°C, amplifications were programmed for 45 consecutive cycles. Each cycle included 1 min at 94°C, 1 min at 45°C, 55°C to 65°C (on the basis of individual primers), and 2 min at 72°C. A final 7 min extension step was applied at 72°C.
After amplification, each 15 μL of each amplification product was mixed with 6.5 μL of formamide-loading buffer 0.5% (w/v) xylene cyanol and 0.5% (w/v) bromophenol blue, 98% formamide and 10 mM EDTA (pH 8.0). Then, 4.5 μL of the PCR product and loading buffer mixture for each sample was loaded for electrophoresis in a 4% polyacrylamide gel as previously described. The gels were visualized through strong base silver staining method (Chen et al., Citation1998).
Data analyses
To evaluate the goodness of fit of the observed and expected segregation ratios of the stripe rust resistance genes and mode of inheritance, our study applied chi-square tests. The ‘chitest’ procedure in Microsoft Office Excel 2007 software was used to calculate the P values. Linkage analyses and map construction were performed using the computer program Mapmaker, Version 3.0 (Lincoln et al., Citation1992). A LOD score should be set to 3.0 for gene linkage (Kosambi, Citation1943). Kosambi mapping function and the Mapdraw3.0 program were used to establish the linkage group. The linkage group was assigned to a chromosome by testing RGAP markers with the set of nulli-tetrasomic Chinese Spring lines and a chromosomal arm with SSR markers referring to the wheat SSR consensus map (Somers et al., Citation2004).
Results
Genetic analysis of the stripe rust resistance gene in H9015-17–1-9–6
Our results indicated that the parent P. huashanica and H9015-17–1-9–6 were resistant to all the tested stripe rust races, whereas 7182 and ‘Mingxian169' were susceptible (). When tested with race CYR32, F1 plants showed the same phenotype as that of H9015-17–1-9–6 and indicated dominant resistance (). Segregation of resistant and susceptible plants in the F2 plants fitted a 3:1 resistant/susceptible ratio (χ2 = 0.34, P = 0.50), which indicated a single dominant gene confers resistance to race CYR32. Segregation of resistant and susceptible plants fit a 1:1 ratio (χ2 = 0.05, P = 0.65) in the BC1 population. Finally, the F2:3 generation segregated 36 homozygous resistant, 67 segregating, and 38 homozygous susceptible lines and fitted a 1:2:1 (resistant/segregating/susceptible) ratio (χ2 = 0.40, P = 0.82) (). Overall, the stripe rust resistance to race CYR32 in H9015-17–1-9–6 displayed dominant inheritance controlled by a single gene/locus, which was temporarily designated as YrH9015.
Table 1. Infection types on Psathyrostachys huashanica, 7182, H9015-17–1-9–6 and ‘Mingxian169' to 12 Chinese races of Puccinia striiformis f. sp. tritici (Pst) at the seedling stage under greenhouse conditions.
Table 2. Genetic analysis of resistance to race CYR32 in H9015-17–1-9–6.
GISH and FISH analyses
The somatic cells of the resistant line H9015-17–1-9–6 probed with genomic DNA from P. huashanica showed no visible introgression in H9015-17–1-9–6 (). This result suggested that the sizes of the introgression segments from P. huashanica may be excessively small to be detected by GISH. The FISH results indicated that the 5D chromosomes of H9015-17–1-9–6 were similar to 7182 () (Yang et al., Citation2015). Theoretically, H9015-17–1-9–6 was an introgression line.
Fig. 1 (Colour online) Chromosome composition of H9015-17–1-9–6 by GISH and FISH. (A) Chromosome composition of H9015-17–1-9–6 by GISH using Leymus mollis genomic as the probe. (B) Chromosome composition of H9015-17–1-9–6 by FISH analysis using Oligo-p Ta535 (red), Oligo-p Sc119.2 (green) as probes. (C) Chromosome 5D from H9015-17–1-9–6. (D) Chromosome 5D from wheat parent line 7182. (Yang et al., Citation2015).
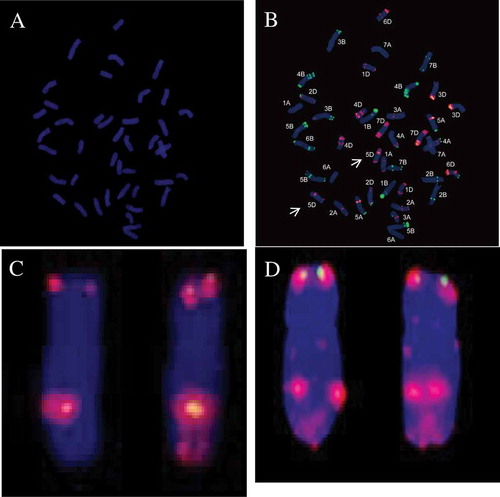
Molecular mapping of YrH9015
All of the 142 F2 plants with confirmed phenotypes and validated F2:3 data were used to identify molecular markers. Seven RGAP markers (M1, M2, M3, M4, M5, M6 and M7), showed clear polymorphisms between the parents and bulks. All markers were dominant, with bands amplified in the resistant parent and resistant bulk but not in the susceptible parent and susceptible bulk. Subsequently, the 142 F2 progeny were used to confirm genetic linkage detection for this resistance gene with the seven polymorphic markers (, ). Partial RGAP markers amplification results are shown in .
Table 3. PCR amplification result of associated sequences of resistance gene analogue polymorphism (RGAP) markers and simple sequence repeat (SSR) markers with YrH9015 in the F2 population from ‘Mingxian169' × H9015-17–1-9–6.
Table 4. Sequence of resistance gene analogue polymorphism (RGAP) primers and simple sequence repeat (SSR) primers used to identify linked markers in stripe rust resistance genes in wheat cultivar H9015-17–1-9–6.
Fig. 2 Polyacrylamide gels showing RGAP marker M4 (A) and M5 (B) amplifications in the resistant parent (PR, H9015-17–1-9–6), resistant bulk (RB), susceptible parent (SP, Mingxian169), susceptible bulk (SB), and F2 plants of cross Mingxian169/H9015-17–1-9–6. RGAP marker M6 (C) amplification in resistant parent (RP, H9015-17–1-9–6), resistant bulk (RB), susceptible parent (SP, Mingxiang169), susceptible bulk (SB), Chinese Spring (CS) and 21 nullisomic (N) or monosomic (M)-tetrasomic (T) CS lines. The arrow shows the absence of the marker in line N5DT5A, indicating its location on chromosome 5D.
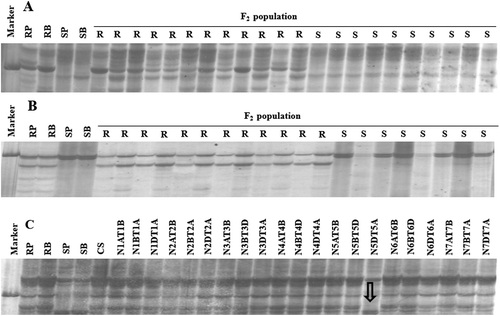
The seven RGAP markers were used to amplify the genomic DNA of Chinese Spring (CS). Amongst these markers, only M6 (S2-INV/LM638) present in CS was used to test the 21 nulli-tetrasomic lines. The unique bands were detected in all lines except N5DT5A, as shown by marker M6 in , indicating that the RGAP marker and the linked resistance gene were located on wheat chromosome 5D. To confirm the exact chromosome location, 182 SSR markers covering the 5A, 5B and 5D wheat chromosomes were chosen for polymorphic selection in H9015-17–1-9–6, ‘Mingxian169' and two bulks. Three SSR markers (Xcfd266, Xcfd8 and Xcfd26) displayed clear concordant polymorphisms between the two parents and the two bulks () and were co-dominant. Combined with known marker information, the gene in H9015-17–1-9–6 was initially located on the long arm of chromosome 5D.
Linkage map of YrH9015
Analyses with MapMaker3.0 software were conducted to confirm the linkage and calculate corresponding genetic distances between markers and YrH9015. The genetic distances of the two closest flanking markers M4 (Cre3LR-R/LM638) and M5 (XalLR-R/Nkin2) were 2.4 cM and 3.1 cM, respectively. Markers M1 (RLRR-R/NLRR-INV1) and M7 (RLRR-F/LM638) were distal and proximal, respectively ().
Fig. 3 Linkage maps for the dominant genes YrH9015 on chromosome 5DL. Linked simple sequence repeat (SSR) markers were assigned to chromosomes 5DL based on the consensus map (Somers et al., Citation2004).
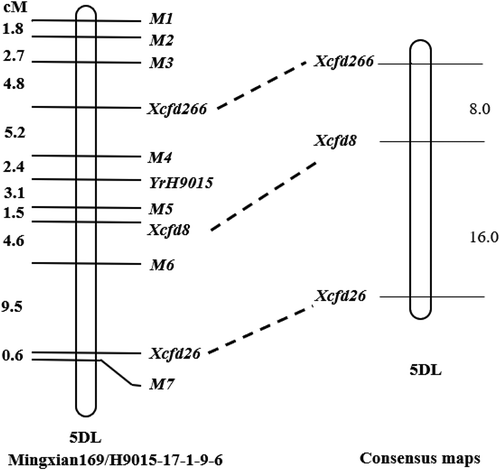
STS markers
The two markers most closely linked with YrH9015 were RGAP markers. Using these two marker sequences to design STS primers was difficult. The STS markers STSM4 (primer: 5ʹ-CAGGAGCCAAAAATACGTAAG and 3ʹ-TGGTCGTCTTCCCCACCCCCCC) and STSM5 (primer: 5ʹ-CGATGACTATGCAGTCCTAC and 3ʹ-GCTACTGATACGTCAGGATG) were successfully developed from RGAP markers M4 and M5. The STS markers STSM4239bp () and STSM5135bp () showed the same patterns as the original RGAP markers when tested on the parents, bulks and the mapping population.
Polymorphism of the flanking STS markers among wheat cultivars and breeding lines
Markers STSM4 and STSM5 were genotyped on a set of 35 main wheat cultivars to check for the presence of YrH9015-linked alleles in these markers (Table S1). STSM4 was only present in wheat ‘Emai580' (susceptible to race CYR32, IT = 4) and STSM5 was absent in all, showing 97.1% and 100% polymorphism, respectively. The results indicated that none of the cultivars likely have YrH9015 and that the STS markers can be used for marker-assistant incorporation of the genes into various wheat cultivars.
Discussion
The results of this study showed that the introgression line H9015-17–1-9–6 was highly resistant to 12 stripe rust races with wide virulence in China. The gene showing resistance to race CYR32 was temporarily designated as YrH9015 and was located on chromosome 5DL. There are three stripe rust resistance genes in wheat, Yr40 (Kuraparthy et al., Citation2007), Yr70 (Bansal et al., Citation2017) and YrDa2 (Chen et al., Citation1995), located on chromosome 5D. Yr40 was originally derived from the diploid Aegilops geniculata. Yr70 was discovered in the introgression line IL 393–4 developed by Chhuneja et al. (Citation2008) and was transferred into the Indian wheat cultivar PBW550. The source of resistance in line IL393-4 is from A. umbellata. YrDa2 was located on chromosome 5D by monosomic analysis, but the precise location remains unclear. The wheat line carrying YrDa2 (Daws) was developed by hybridizing wheat lines CI 14 484, CI 13 645 and PI 178 383 (Zeven & Zevenhissink, Citation1976). Therefore, the gene may be derived from CI 14 484, CI 13 645 or the Washington Selection 101 (Zeven & Zevenhissink, Citation1976). YrH9015 is different from any known stripe rust resistance gene on chromosome 5D and appears to be novel. Only four stripe rust resistance genes (Yr5, Yr10, Yr15 and Yr26) in wheat provide resistance to stripe rust races in China since race CYR32 became predominant. These genes are all alien derived except Yr10, which was derived from spelt wheat and was first discovered in PI 178 383 (Metzger & Silbaugh, Citation1970). Yr5 was originally obtained from hexaploid Triticum spelta album, whereas Yr15 was acquired from the tetraploid wild emmer wheat Triticum dicoccoides. Yr26 derives from Haynaldia villosa. In this study, the wheat line H9015-17–1-9–6 showed high resistance to race CYR32, which provides a new resistance gene resource.
In recent years, new physiological races of wheat stripe rust have emerged and have caused serious decline or loss of resistance in many cultivars. Many researchers have exploited exogenous germplasm to enrich the stripe rust resistance gene resources in wheat. A large number of wheat varieties with introgressed genes have been bred through hybridization between common wheat and related species in Secale, Elytrigia, Aegilops and H. villosa (Yao et al., Citation2010). These exotic resistance genes have greatly broadened the genetic basis of stripe rust resistance in common wheat. In many wheat-rye 1BL/1RS translocation lines, chromosome 1BL in bread wheat was replaced by rye chromosome arms of 1RS (Zhou et al., Citation2004).
Psathyrostachys huashanica Keng (2n = 2x = 14, NsNs) is amongst the wild related species of hexaploid wheat. High homology exists between the genomes of P. huashanica and bread wheat, and the former is considered to be an important genetic resource of disease resistance for wheat variety improvement. Several genes, YrHA (Ma et al., Citation2013), YrHy (Yao et al., Citation2010), YrHu (Ma et al., Citation2016), YrHua (Cao et al., Citation2005) and YrH9020 (Li et al., Citation2012), derived from P. huashanica that were resistant to most Chinese stripe rust races, have previously been reported. YrHA, conferring resistance to CYR31, was localized on the long arm of chromosome 1A. YrHy, conferring resistance to CYR32, was localized on the short arm of chromosome 2B. YrHu, conferring resistance to both CYR29 and CYR33, was localized on the short arm of chromosome 3A. Based on GISH analyses, we found that alien translocation for YrHu is either a small alien segment derived from P. huashanica or a small wheat segment derived from gene recombination. More studies are needed to determine the source of YrHu. YrHua, conferring resistance to CYR30, was unclear on the chromosomal position of this gene. YrH9020, conferring resistance to CYR33, was localized on the short arm of chromosome 2D. YrH9015 is different from any known stripe rust resistance gene from P. huashanica. However, the utilization rate of these resistance genes is low.
Psathyrostachys huashanica is resistant to stripe rust and powdery mildew, and some resistance genes of this plant have exhibited a strong genetic stability (Jing et al., Citation1999; Wang & Shang, Citation2000). The Nh5 and Nh4 chromosomes of P. huashanica can compensate for the deletion of the fifth and third chromosomes of the fifth and third homologous groups of common wheat (Zhao et al., Citation2003). This implies that the chromosomes of P. huashanica can be easily fixed in the wheat genome and can be a useful source of disease resistance in cultivated wheat. Further research to characterize the gene YrH9015 by developing near-isogenic lines, fine mapping and cloning is underway.
Supplemental Table 1
Download MS Word (14.6 KB)Acknowledgements
We thank Dr Yongli Qiao and Dr Simόn-Mateo Carmen for critical review of the manuscript.
Supplemental material
Supplemental data for this article can be accessed online here: https://doi.org/10.1080/07060661.2018.1523230.
Additional information
Funding
References
- Bai B, Du JY, Lu QL, He CY, Zhang LJ, Zhou G, Xia XC, He ZH, Wang CS. 2014. Effective resistance to wheat stripe rust in a region with high disease pressure. Plant Dis. 98:891–897.
- Bansal M, Kaur S, Dhaliwal H, Bains N, Bariana H, Chhuneja P, Bansal U. 2017. Mapping of Aegilops umbellulata‐derived leaf rust and stripe rust resistance loci in wheat. Plant Pathol. 66:38–44.
- Cao ZJ, Wang XP, Wang MN, Cao SH, Jing JX, Shang HS, Li ZQ, Zhang XQ. 2005. Genetic analysis and molecular markers of a novel stripe rust resistance gene YrHua in wheat originated from Psathyrostachys huashanica keng. Acta Genet Sin. 32:738.
- Chen SY, Zhang AJ, Fu J. 1991. The hybridization between Triticum aestivum and Psathyrostachys huashanica. Acta Genet Sin. 18:508–512.
- Chen WQ, Kang ZS, Ma ZH, Xu SC, Jin SL, Jiang YL. 2013. Integrated management of wheat stripe rust caused by Puccinia striiformis f. sp. tritici in China. Sci Agric Sin. 46:4254–4262.
- Chen WQ, Wu LR, Liu TG, Xu SC, Jin SL, Peng YL, Wang BT. 2009. Race dynamics, diversity, and virulence evolution in Puccinia striiformis f. sp. tritici, the causal agent of wheat stripe rust in China from 2003 to 2007. Plant Dis. 93:1093–1101.
- Chen X, Line RF, Jones SF. 1995. Chromosomal location of genes for resistance to Puccinia striiformis in winter wheat cultivars Heines VII, Clement, Moro, Tyee, Tres, and Daws. Phytopathology. 85:1362–1367.
- Chen XM, Line RF, Leung H. 1998. Genome scanning for resistance-gene analogs in rice, barley, and wheat by high-resolution electrophoresis. Theor Appl Genet. 97:345–355.
- Chhuneja P, Kaur S, Goel RK, Aghaee-Sarbarzeh M, Prashar M, Dhaliwal HS. 2008. Transfer of leaf rust and stripe rust resistance from Aegilops umbellulata Zhuk. to bread wheat (Triticum aestivum L.). Genet Resour Crop Evol. 55:849–859.
- Gerechteramitai ZK, Stubbs RW. 1970. A valuable source of yellow rust resistance in Israeli populations of wild emmer, Triticum dicoccoides Koern. Euphytica. 19:12–21.
- Hovmøller MS, Walter S, Bayles RA, Hubbard A, Flath K, Sommerfeldt N, Leconte M, Czembor P, Rodriguez-A J, Thach T, et al. 2016. Replacement of the european wheat yellow rust population by new races from the centre of diversity in the near‐Himalayan region. Plant Pathol. 65:402–411.
- Jing JX, Fu J, Yuan HX, Wang MN, Shang HS, Li ZQ. 1999. A preliminary study on heredity of the resistance to stripe rust in three wild relatives of wheat. Acta Phytopathol Sin. 29:147–150.
- Juliana P, Singh RP, Singh PK, Crossa J, Huerta-Espino J, Lan C, Bhavani S, Rutkoski JE, Poland JA, Bergstrom GC, et al. 2017. Genomic and pedigree-based prediction for leaf, stem, and stripe rust resistance in wheat. Theor Appl Genet. 13:1415–1430.
- Kanazin V, Marek LF, Shoemaker RC. 1996. Resistance gene analogs are conserved and clustered in soybean. Proc Natl Acad Sci USA. 93:11746–11750.
- Kosambi DD. 1943. The estimation of map distances from recombination values. Ann Human Genet. 12:172–175.
- Kuraparthy V, Chhuneja P, Dhaliwal HS, Kaur S, Bowden RL, Gill BS. 2007. Characterization and mapping of cryptic alien introgression from Aegilops geniculata with new leaf rust and stripe rust resistance genes Lr57 and Yr40 in wheat. Theor Appl Genet. 114:1379–1389.
- Li Q, Huang J, Hou L, Liu P, Jing JX, Wang BT, Kang ZS. 2012. Genetic and molecular mapping of stripe rust resistance gene in wheat–psathyrostachys huashanica translocation line H9020-1-6-8-3. Plant Dis. 96:1482–1487.
- Li Q, Xia T, Li JJ, Li GB, Wang F, Wang BT. 2011. Determination of pathogenic range of T4 new strains of Puccinia strifformis f.sp. tritici to ‘Zhou4ʹ. Acta Phytopathol Sin. 41:604–610.
- Lincoln S, Daly M, Lander E, Lincoln S, Daly M, Lander E, Lincoln S, Daly M, Lander E 1992. Constructing genetic maps with Mapmarker/EXP3.0. Whitehead Institute Technical Report. Whitehead Institute. p. 3.
- Ma DF, Fang ZW, Yin JL, Chao KX, Jing JX, Li Q, Wang BT. 2016. Molecular mapping of stripe rust resistance gene YrHu derived from Psathyrostachys huashanica. Mol Breed. 36:1–9.
- Ma DF, Zhou XL, Hou L, Bai YB, Li Q, Wang HG, Tang MS, Jing JX. 2013. Genetic analysis and molecular mapping of a stripe rust resistance gene derived from Psathyrostachys huashanica keng in wheat line H9014-121-5-5-9. Mol Breed. 32:365–372.
- Macer RCF. 1966. The formal monosomic genetic analysis of stripe rust (Puccinia striiformis) resistance in wheat. Proc. Int. Wheat Genet. Symp., 2nd. Hereditas Suppl. 2:127–142.
- McIntosh RA, Dubcovsky J, Rogers J, Morris C, Appels R, Xia XC. 2015. Catalogue of gene symbols for wheat: 2015–2016 Supplement. https://shigen.nig.ac.jp/wheat/komugi/genes/macgene/supplement2015.pdf.
- McIntosh RA, Dubcovsky J, Rogers J, Morris C, Appels R, Xia XC 2017. Catalogue of gene symbols for wheat: 2017 Supplement. https://shigen.nig.ac.jp/wheat/komugi/genes/macgene/supplement2017.pdf.
- Metzger RJ, Silbaugh BA. 1970. Inheritance of resistance to stripe rust and its association with brown glume color in Triticum aestivum L. ‘P.I. 178383ʹ. Crop Sci. 10:567–568.
- Michelmore RW, Paran I, Kesseli RV. 1991. Identification of markers linked to disease-resistance genes by bulked segregant analysis: a rapid method to detect markers in specific genomic regions by using segregating populations. Proc. Natl. Acad. Sci. 88:9828–9832.
- Pahalawatta V, Chen XM. 2005. Genetic analysis and molecular mapping of wheat genes conferring resistance to the wheat stripe rust and barley stripe rust pathogens. Phytopathology. 95:427–432.
- Ren RS, Wang MN, Chen XM, Zhang ZJ. 2012. Characterization and molecular mapping of Yr52 for high-temperature adult-plant resistance to stripe rust in spring wheat germplasm PI 183527. Theor Appl Genet. 125:847–857.
- Rogers SO, Bendich AJ. 1985. Extraction of DNA from milligram amounts of fresh, herbarium and mummified plant tissues. Plant Mol Biol. 5:69–76.
- Shi ZX, Chen XM, Line RF, Leung H, Wellings CR. 2001. Development of resistance gene analog polymorphism markers for the Yr9 gene resistance to wheat stripe rust. Genome. 44:509–516.
- Somers DJ, Isaac P, Edwards K. 2004. A high-density microsatellite consensus map for bread wheat (Triticum aestivum L.). Theor App Genet. 109:1105–1114.
- Tang ZX, Li M, Chen L, Wang YY, Ren ZL, Fu SL. 2014a. New types of wheat chromosomal structural variations in derivatives of wheat-rye hybrids. Plos One. 9:e110282.
- Tang ZX, Yang ZJ, Fu SL. 2014b. Oligonucleotides replacing the roles of repetitive sequences pAs1, pSc119.2, pTa-535, pTa71, CCS1 and pAWRC.1 for FISH analysis. J Appl Genet. 55:313–318.
- Wan AM, Zhao ZH, Chen XM, He ZH, Jin SL, Jia QZ, Yao G, Yang JX, Wang BT, Li GB. 2004. Wheat stripe rust epidemic and virulence of Puccinia striiformis f. sp tritici in China in 2002. Plant Dis. 88:896–904.
- Wang MN, Shang HS. 2000. Evaluation of resistance in Psathyrostachys huashaica to wheat take-all fungus. J Northwest Agric Univ. 28:69–71.
- Wang Y, Zhang HZ, Xie JZ, Guo BM, Chen YX, Zhang HY, Lu P, Wu QH, Li MM, Zhang DY, et al. 2017. Mapping stripe rust resistance genes by BSR-Seq: yrMM58 and YrHY1 on chromosome 2AS in Chinese wheat lines Mengmai 58 and Huaiyang 1 are Yr17. Crop J. 1:91–98.
- Yan GP, Chen XM, Line RF, Wellings CR. 2003. Resistance gene-analog polymorphism markers co-segregating with the YR5 gene for resistance to wheat stripe rust. Theor Appl Genet. 106:636–643.
- Yang XF, Wang CY, Li X, Chen CH, Tian ZG, Wang YJ, Ji WQ. 2015. Development –andmolecular cytogenetic identification of a novel wheat–leymus mollis Lm#7Ns (7D) disomic substitution line with stripe rust resistance. Plos One. 10:e0140227.
- Yang ZM, Xie C, Sun Q. 2003. Situation of the sources of stripe rust resistance of wheat in the post-cy32 era in China. Acta Agrono Sin. 29:161–168.
- Yao Q, Wang Y, He MM, Li Y, Zhou XL, Wang BT, Jing JX. 2010. SSR molecular mapping ofstripe rust resistance gene of wheat translocation line H9020-20-12-1-8 derived from Psathyrostachys huashanica Keng. J Agric Biotechnol. 18:676–681.
- Zeven AC, Zevenhissink NC. 1976. Genealogies of 14,000 wheat varities. Mexico: The Netherlands Cereal Center-NGC, Wageningen and the International Maize and Wheat Improvement Center; p. 121.
- Zhao JX, Chen XH, Wang XL, Wu J, Fu J, He BR, Song YZ, Sun ZG. 2003. C-Banding identification of alien substitution linesand alien additional lines in Triticum-Psathyrostachys. J Northwest Sci-Tech Univ Agric Forestry. 31:1–4.
- Zhou XL, Wang MN, Chen XM, Lu Y, Kang ZS, Jing JX. 2014. Identification of Yr59 conferring high-temperature adult-plant resistance to stripe rust in wheat germplasm PI 178759. Theor Appl Genet. 127:935–945.
- Zhou XL, Wang WL, Wang LL, Hou YD, Jing JX, Wang Y, Xu ZQ, Yao JL, Ma DF. 2011. Genetics and molecular mapping of genes for high-temperature resistance to stripe rust in wheat cultivar xiaoyan 54. Theor Appl Genet. 123:431–438.
- Zhou Y, He ZH, Zhang GS, Xia LQ, Chen XM, Gao YC, Jing ZB, Yu GJ. 2004. Utilization of 1BL/1RS translocation in wheat breeding in China. Acta Agro Sin. 30:531–535.