Abstract
Christmas trees produced in Quebec, Canada, mostly include balsam fir (Abies balsamea), which is a species susceptible to Delphinella shoot blight. Over the past decade, the Canadian forests and Christmas tree industry have encountered an increase in Delphinella shoot blight, and only a few studies have assessed and quantified the damage caused by this disease. The different developmental stages of the disease were studied and the presumed causal pathogenic agent was isolated from infected needles in this work. Delphinella balsameae was isolated less frequently than its phylogenetically closely related pathogen Sydowia polyspora. Furthermore, species-specific PCR protocols to detect both D. balsameae and S. polyspora were developed and the occurrence of these species in infected and symptomless needles sampled in Christmas tree plantations was evaluated. While D. balsameae was detected only in diseased needles, S. polyspora was frequently found in both asymptomatic needles and in needles displaying shoot blight. Experiments showed that D. balsameae is the more prevalent agent associated with fir tree shoot blight in Quebec.
Résumé
La majorité des sapins de Noël produits au Québec, Canada, sont des sapins baumiers (Abies balsamea), une espèce sensible à l’infection par Delphinella. Durant la dernière décennie, il y a eu une augmentation de la maladie de la brûlure des pousses dans les forêts canadiennes et les plantations d’arbres de Noël; toutefois, peu d’études ont évalué et quantifié les dommages causés par cette maladie. Cette étude présente les différents stades de développement de la maladie, et l’agent pathogène supposé la causer a été isolé d’aiguilles infectées. Le Delphinella balsameae était moins fréquemment isolé d’aiguilles montrant des symptômes que le Sydowia polyspora, un agent pathogène qui lui est phylogénétiquement apparenté. De plus, des protocoles de PCR spécifiques à l’espèce pour détecter le D. balsameae et le S. polyspora ont été développés, et l’occurrence de ces derniers dans des aiguilles infectées et saines d’apparence échantillonnées dans des plantations d’arbres de Noël a été évaluée. Alors que le D. balsameae a été détecté seulement dans les aiguilles malades, le S. polyspora était fréquemment trouvé dans les aiguilles asymptomatiques ainsi que dans les aiguilles infectées par la brûlure des pousses. Les expériences réalisées ont démontré que le D. balsameae est l’agent le plus souvent associé à la brûlure des pousses de sapin au Québec.
Introduction
True firs (Abies spp.) play an important ecological role, but are also aesthetic components of recreational landscapes and economically important as Christmas trees and greenery products (Filip & Schmill, Citation1990). Firs are susceptible to a number of fungal diseases such as shoot blights which are characterized by wilting needles and shoots, and afflicted coniferous structures curl or bend and frequently carry visible dark fruiting bodies (Talgø, Citation2012).
Delphinella shoot blight of firs is caused by two fungal species, D. abietis (O. Rostr.) E. Müll. and D. balsameae (A. M. Waterman) E. Müll. (Funk, Citation1985). The occurrence of Delphinella in fir tissues can be determined by morphological observations of the sexual spores in pseudothecia on needles and by the isolation of the pathogens from infected material. The isolation from tissues infected by D. abietis has been reported to be laborious (Talgø et al., Citation2016) and isolation of D. balsameae has, to our knowledge, been reported only once, in the 1940s (Waterman, Citation1945).
The Delphinella genus belongs to Dothideomycetes, an ascomycetous class which contains several plant pathogenic species (Schoch et al., Citation2009; Hyde, Citation2011) including Sydowia polyspora that has been reported as an endophytic fungus, but also as a causal agent of current season needle necrosis. This genus, formerly named Rehmiellopsis, is morphologically characterized by asci containing hyaline, uniseptate spores (Barr, Citation2001). A morphological characteristic that discriminates the two species causing Delphinella shoot blight is the size of reproduction structures. Delphinella balsameae generally harbours larger asci (80–140 × 33–41 µm) and ascospores (30–50 × 7–12 µm) than D. abietis (50–90 × 18–22 µm and 11–21 × 4–7 µm, respectively) (Funk, Citation1985).
Over the past decades, there has been an increase in shoot blight worldwide. For instance, a study on noble fir (Abies procera Rehd.) for greenery production in Norway showed an alarming situation where the ratio of discarded branches due to Delphinella infection increased from 31% to 87% between 1994 and 2000 (Solheim & Skage, Citation2002). Moreover, a recent intensive study of this disease has shown that D. abietis could be isolated from asymptomatic fir seedlings (Talgø et al., Citation2016), raising the concern of potential seed colonization by the genus Delphinella.
In both the European and North American continents, D. abietis is reported as a fir pathogen (Funk, Citation1985; Solheim & Skage, Citation2002; Solheim, Citation2003; Talgø et al., Citation2016), while D. balsameae seems to be predominant in the northern USA and in eastern and western Canada (Magasi, Citation1974; Merrill et al., Citation1997). Both fungal species have been identified as fir pathogens since the 1960s in British Columbia, Canada. While globally regarded as a threat of minor significance, they were reported to cause important local infections (Forest Research Laboratory, Citation1966; Fiddick, Citation1969). However, the situation has changed in the past few years. In the province of Quebec, high occurrence of Delphinella shoot blight, attributed to D. balsameae, has been reported since 2010 (Natural Resources Canada, 2010; Bouchard et al., Citation2012; Forêts, Faune et Parcs Québec, Citation2018). Considering the economic importance of the Christmas tree industry in this province (Statistics Canada, Citation2017), this disease is raising serious concerns given that most Christmas trees produced in Quebec are balsam fir (Abies balsamea), a species susceptible to Delphinella infection (Agriculture Pêcheries et Alimentation Québec, Citation2018). Despite these facts, few studies have aimed to understand the pathogen biology and quantify the damage caused by Delphinella shoot blight in Christmas tree plantations and Canadian natural and planted fir forests (Unger & Fiddick, Citation1978; Unger & Vallentgoed, Citation1988).
Disease management strategies rely on the ability to efficiently detect and quantify the pathogen and the resulting disease. Molecular approaches, such as species-specific PCR (Polymerase Chain Reaction), emerged as a promising avenue to detect plant pathogens (Henson & French, Citation1993; McCartney et al., Citation2003; Sankaran et al., Citation2010). PCR-based assays have successfully been applied to detect the causal agents of coniferous tree diseases (Stenström & Ihrmark, Citation2005; Zeng et al., Citation2005; Smith & Stanosz, Citation2006; Morgenstern et al., Citation2014).
In this work, we aimed to develop PCR assays for the detection of fungal species involved in fir shoot blight diseases. In addition, we describe the window period when ascospores of D. balsamea are released to infect new shoots in the Eastern-Townships and Chaudière-Appalaches (Quebec) plantations of Christmas tree.
Materials and methods
Christmas tree plantations under investigation
lists the Christmas tree plantations that were sampled in this study. These plantations are located in the Eastern-Townships and Chaudière-Appalaches regions (administrative regions of Quebec, Canada) ().
Table 1. Locations of the Christmas tree plantations included in this study.
Observation of pseudothecia of Delphinella balsameae
Christmas trees (A. balsamea (L.) Mill.) were sampled every other week from 11 May 2015 to 24 August 2015 in infected plantations #1−4, and from 19 May 2016 to 14 July 2016 in plantations #4−6. In each of the plantations included in the study, at every sampling time, shoots were collected from three different trees bearing shoot blight symptoms () and from three asymptomatic trees. For each symptomatic sampled shoot, diseased needles were collected. Five pseudothecia were dissected from needles, fresh-mounted on a slide, and observed under a microscope (Carl Zeiss 47–30-14–9901, Oberkochen, Germany) to record the presence of asci and ascospores.
Fig. 1 (Colour online) Symptoms and fungal reproduction structures observed on balsam fir and growth of two fungi isolated from these structures. (A) shoot blight symptoms, (B) pseudothecia on the superior side of a needle, (C) ascus from pseudothecia releasing uniseptate ascospores characteristic of Delphinella balsameae, (D) colonies of D. balsameae showing a dissemination pattern, (E) 2-week-old mycelium of D. balsameae on PDA medium, (F) 2-week-old mycelium of Sydowia polyspora on PDA medium.
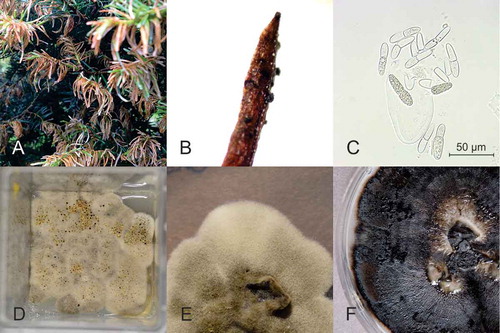
Isolation and identification of Delphinella balsameae
Two methods were used to isolate D. balsameae from infected tissues. In 2015, fungi were isolated from symptomatic needles according to Plante & Bernier (Citation1997) with the following modifications. Pseudothecia were simply crushed in sterile distilled water before being plated on potato dextrose agar (PDA, Bioshop, Burlington, ON) medium supplemented with 50 mg L−1 of streptomycin (Sigma-Aldrich, St. Louis, MO). Fungal colonies were further purified by serial transfers on the same medium. Purified isolates were maintained on PDA without the antibiotic, and incubated in the dark at room temperature.
In 2016, D. balsameae isolates were recovered using a protocol modified from Talgø et al. (Citation2016). In order to recover ejected ascospores, symptomatic shoots with mature ascospores (pseudothecia), were attached to the cover of sterile magenta culture boxes GA7 (Caisson Laboratories, Smithfield, UT) over Petri dishes containing PDA medium, and incubated up to 12 days at room temperature. Colonies found multiple times on the agar were then sub-cultured on new Petri dishes containing PDA medium. Identification of the isolated fungi was carried out by partial sequencing of the internal transcribed spacer (ITS) region of the rRNA genes using the primer pair ITS4-ITS5 (see ). The elongation factor 1 alpha (EF1-α) and the β-tubulin (BTUB) genes were also sequenced for some isolates. These genes are routinely used in taxonomy of fungal species (Stielow et al., Citation2015). Between 20−50 mg of mycelium were homogenized using a FP120 FastPrep cell disrupter (Thermo, Milford, MA). Fungal genomic DNA was extracted using the FastDNATM SPIN kit for soil (MP Biomedicals, Santa Ana, CA) according to the manufacturer’s protocol, and eluted in 50 µL in DES buffer. Primers used to amplify ITS, EF1-α and BTUB are listed in . The PCR was carried out in standard Taq Reaction Buffer (New England Biolabs, Ipswich, MA) with 200 µM dNTPs (New England Biolabs), 0.2 µM each primer (Integrated DNA Technologies, Coralville, IA), 1.25 unit Taq DNA polymerase and approximately 0.4 ng of genomic DNA. PCR reactions were performed in a T100 thermocycler (Bio-Rad, Hercules, CA) using the conditions described in . PCR amplicons were resolved by electrophoresis on an agarose and visualized with a Gel DocTM XR+ using the Image LabTM Software (Bio-Rad).
Table 2. PCR primers used in this study.
Table 3. Thermocycler programme settings used with different pairs of PCR primers.
Amplicons were sequenced at the Plateforme de séquençage et de génotypage des génomes of the Centre de recherche du CHUL (Quebec City, Canada) using an ABI 3730xl sequencer (Applied Biosystems, Foster City, CA). Fungal sequences were analysed using the BLAST (Basic Local Alignment Search Tool) program from GenBank database (http://www.ncbi.nlm.gov/BLAST) and DNA sequences were aligned with Clustal Omega software. A dendrogram was constructed with MEGA7 software (Kumar et al., Citation2016) by applying the neighbour joining algorithm (Saitou & Nei, Citation1987). A bootstrap analysis was then performed using 1000 resamplings with Penicillium chrysogenum as an outgroup.
Development of a detection assay for Delphinella balsameae and Sydowia polyspora
To develop a specific detection assay for D. balsameae and S. polyspora (Bref. & Tavel) E. Müll., primers were designed using specific DNA sequences in the three genetic regions ITS, EF1-α and BTUB based on gene alignments including closely related species. Designed primers were optimized considering hairpin loops, dimers, homology with non-specific sequences and Tm using the OligoAnalyzer 3.1 software (Integrated DNA Technologies, Coralville, IA).
Amplification protocols coupled to selected primers combinations were optimized to ensure specific and sensitive detection of D. balsameae and S. polyspora. Specificity of the detection method was determined on three D. balsameae isolates (this study), three S. polyspora isolates (this study), four other fungi isolated from balsam fir (Allantophomopsis sp., Phoma sp., Rhizoctonia sp. and Rhizosphaera sp.), and other fungi (Cladosporium cladosporioides, Lecythophora sp., Phaeosphaeria sp., Penicillium glaucoalbidum formerly Thysanophora penicillioides, Scleroconidioma sphagnicola, Aureobasidium pullulans, Endoconidioma populi, Sydowia polyspora) obtained from the UAMH centre for global microfungal biodiversity Toronto (Canada) culture collection. Sensitivity was assessed on serial dilutions of DNA from the target species, ranging from 10 ng to 1 fg. Both characterizations were made using the primers and the optimized PCR protocol described in and .
Detection of Delphinella balsameae and Sydowia polyspora in samples from balsam fir plantations
DNA isolation from symptomatic and asymptomatic needles was carried out following homogenization of approximately 20 mg of plant tissues using a FP120 FastPrep (4.5 m s−1 for 2 min). DNA was then recovered with the PowerPlant® Pro DNA Isolation Kit (Qiagen, Hilden, Germany) using manufacturer’s recommendations for high phenolic contents and problematic samples. The quality and concentration of the resulting DNA were assessed using a NanoDropTM 2000 (NanoDrop Technologies, Wilmington, DE). When necessary, further DNA purification was performed using Monarch® PCR & DNA Cleanup Kit (New England Biolabs). Approximately 10 ng of DNA extracted from balsam fir needles were used in the PCR reaction.
Ability of Delphinella balsameae ascospores to infect balsam fir trees
Twenty 2-year-old balsam fir seedlings were used for this study. They were divided in two groups of 10 trees that were each planted in a 99-L plastic box sealed with polypropylene film and a plastic cover. Ten balsam tree shoots were attached underneath the container lid at approximately 30 cm above the balsam fir seedlings. One group was exposed to balsam fir shoots bearing mature fruiting bodies of D. balsameae, while the second group was exposed to asymptomatic shoots. Five days after exposure, the overlaid shoots were removed and the balsam fir seedlings were grown for 2 months in a CMP3244 incubation chamber (Conviron, Winnipeg, MB). The growing conditions were 90% relative humidity, a 16-h photoperiod, day temperature of 24°C, and night temperature of 15°C. Needles from each seedling were then sampled and the presence of D. balsameae in the tissue was tested using the Delphinella BTUB assay described above.
Results
Symptoms and observations of shoot blight
Microscopic observations of the balsam fir needles exhibiting Delphinella shoot blight symptoms confirmed the presence of D. balsameae (,c). As described by Waterman (Citation1945), we observed cylindric-clavate bitunicate asci with a typical size of about 100 µm that contain multiple ellipsoidal, uniseptate and hyaline ascospores (usually 16) of 36.8 × 10.3 ± 1.7 × 1.4 µm.
We observed mature pseudothecia from early to mid-May, D. balsameae ascospore release from early until the end of June, and signs and symptoms of infection on new shoots in the following few weeks. The presence of pseudothecia, asci and ascospores of D. balsameae were recorded on a regular basis through summers 2015 (every week) and 2016 (every other week). In both years and for all plantations under study, asci and ascospores from the needles infected the previous year appeared mature at the first observation dates (early to mid-May). According to the decreasing number of spores in asci, we established that in 2015 the dissemination of ascospores started in early June and by mid-June the asci were mostly empty (). Early symptoms of the disease such as chlorosis before browning of needles () and emergence of immature fruiting bodies were observed at the end of June in new shoots. In 2016, dissemination ended between mid- and end of June, and no visible symptoms appeared on the new developing shoots before mid-July.
Isolation of Delphinella balsameae and Sydowia polyspora from infected needles
Sydowia polyspora was the dominant species isolated from symptomatic infected needles. The method of Plante & Bernier (Citation1997) allowed the isolation of fungi that were divided into nine morphotypes. One morphotype (morphotype 1) widely predominated. Members of this morphotype exhibited dimorphism both on solid and liquid media. Growth started as yeast-like cells and changed into filamentous melanized mycelium (). The PCR-amplified ITS regions of five members of the predominant morphotype and of one isolate for each of the other eight morphotypes were sequenced. The ITS sequences of isolates belonging to the predominant group (NCBI accession number: MF034402) showed a high level of similarity with the ITS sequence of S. polyspora (at 100% identity with only one exception at 99% homology with two nt differences). Fungal isolates from the predominant group (morphotype 1) were thus identified as Sydowia sp. (). The only isolate of morphotype 2 presented a morphology consisting of white puffy colonies usually secreting dark yellow droplets on solid media (). The ITS sequence of this isolate corresponded to D. abietis with a 99% similarity and only a single base disparity (accession number: KY997059, ). The ITS sequences of the other morphotypes isolates corresponded to common conifer endophytes or opportunistic pathogens frequently found in fir needles (data not shown).
Fig. 2 Phylogenetic tree based on ITS region of species closely related to the Delphinella balsameae strains isolated from diseased needles. Penicillium chrysogenum as an outgroup. GenBank accession numbers are presented in parentheses.
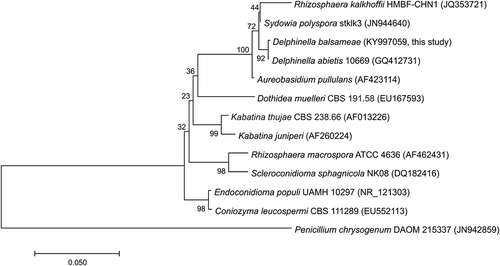
Delphinella balsameae colonies were obtained from ascospores cast from pseudothecia found on infected needles. The second isolation method consisting of a dissemination assay from diseased needles bearing pseudothecia characteristic of D. balsameae allowed the recovery of D. balsameae in one out of six PDA-containing magenta culture boxes of multiple similar-looking colonies (). These colonies matched the phenotype of the only isolate of morphotype 2 (see above) and displayed identical ITS sequences. They were thus identified as D. balsameae.
The isolates of Delphinella balsameae and Sydowia polyspora have been deposited in the UAMH culture collection (University of Toronto, Canada). UAMH numbers are UAMH 11 930 for Delphinella balsameae and UAMH 11 931 for Sydowia polyspora.
Design of specific primers for the detection of Delphinella balsameae and Sydowia polyspora
The ITS, EF1-α and BTUB genes sequences of S. polyspora and D. balsameae isolates were obtained to develop primers that could distinguish both species. PCR amplification of the ITS, EF1-α and BTUB genetic regions in D. balsameae isolates produced fragments of approximately 620, 1000 and 430 bp, respectively. Sequences are available in GenBank under the accession numbers KY997059, KY997060 and MF034404. PCR amplification of the S. polyspora ITS, EF1-α and BTUB genes produced fragments of approximately 620, 1030 and 420 bp, respectively registered in GenBank under the accession numbers MF034402, MF034403 and MF034405.
Specific and sensitive PCR assays were designed for D. balsameae and S. polyspora. PCR amplification of D. balsameae DNA with primers DbalBT1F and DbalBT2R (), designed in the BTUB gene, generated a 179-bp amplicon. The specificity of this assay was assessed on a panel of DNA extracted from other fungal species (). Under the PCR conditions described in , only genomic DNA from D. balsameae led to a positive signal, while no amplification product was detected when DNA from the other fungal species was used as template in the PCR reactions. Using this PCR assay on a serial dilutions D. balsameae DNA, we showed that the detection limit was 10 pg of D. balsameae genomic DNA ().
Fig. 3 a, Sensitivity and b, specificity assays for detection tools developed to identify Delphinella balsameae (upper panels) and Sydowia polyspora (lower panels). a, lane M: DNA marker (100-bp ladder), lanes 1a to 1h: 10-fold dilutions from 10 ng to 1 fg, lane i: no DNA template control. b, lane Mw: DNA marker (1-kb ladder), 2a: Allantophomopsis sp., 2b: Scleroconidioma sphagnicola, 2c: Aureobasidium pullulans, 2d: Endoconidioma populi, 2e: S. polyspora, 2f: D. balsameae, 2g: Rhizosphaera pini, 2h: Rhizoctonia sp., 2i: Phoma sp.
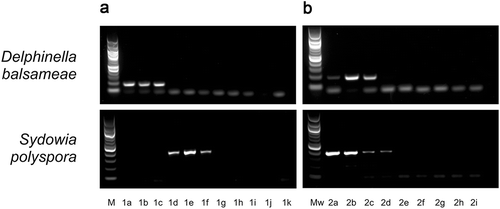
Primers SpolEF4F and SpolEF3R (), designed in the S. polyspora EF1-α gene produce a 356-bp amplicon. Under the PCR conditions listed in , this primer pair specifically amplified S. polyspora DNA (), and as low as 10 pg of the target species DNA could be detected with this assay ().
Delphinella balsameae and Sydowia polyspora were detected in fir tree needles
The D. balsameae assay successfully detected the pathogen from naturally infected symptomatic needles. Primers DbalBT1F and DbalBT2R allowed the amplification of approximately a 180-bp DNA fragment, corresponding to the expected amplicon size of D. balsameae target DNA, in all samples of needles showing blight symptoms. Amplification was only recorded once in asymptomatic needles from diseased trees and D. balsameae was never detected by PCR amplification in healthy trees (). The primer pair SpolEF4F and SpolEF3R was used on the same samples to determine the occurrence of S. polyspora. A PCR product of approximately 360-bp, corresponding to the expected S. polyspora amplicon size, was amplified from most DNA samples extracted from symptomatic needles of trees harbouring shoot blight symptoms. While less frequent, PCR-based detection of S. polyspora from asymptomatic needles of diseased and healthy trees also occurred ().
Table 4. PCR detection of Delphinella balsameae and Sydowia polyspora in fir needles sampled in Christmas tree plantations in Quebec.
Fir seedlings exposed to D. balsameae mature fruiting bodies showed signs of stress (reddening followed by fading), but no symptom characteristic of the shoot blight disease was recorded. Nevertheless, two months after pathogen exposure, the D. balsameae PCR assay performed on these saplings yielded positive signals for five out of 10 trees exposed to the pathogen, while no amplification was recorded in the negative control saplings.
Discussion
For a long time, fir shoot blight was considered a minor disease in Canadian Christmas tree plantations. However, in the past decade, the disease has become a serious concern in many Christmas tree plantations in Quebec, causing significant economic losses. The main aim of the work was to determine whether D. balsameae, which has been reported to cause the shoot blight in Christmas tree farms of the north-eastern USA (Merrill et al., Citation1997), was responsible for the recent disease outbreak in Quebec plantations. Fruiting bodies observed on the diseased needles contained asci and ascospores characteristic of D. balsameae. The size of large ascopores in this pathogen allowed it to be distinguished from D. abietis, another causal agent of the disease in forests of western Canada and western USA (Funk, Citation1985; Chastagner et al., Citation2017) and Scandinavian countries (Talgø et al., Citation2016). The uniseptate ascospores observed in the pseudothecia also differed from those of the closely related genus Sydowia that may have muriform ascospores (Funk, Citation1985). Observation of spores in fruit bodies throughout the summers of 2015 and 2016 suggested that D. balsameae represented the main pathogenic agent causing needle blight symptoms of balsam fir trees in Quebec.
Field observations also revealed that the dissemination period of ascospores was similar to what has already been described (Waterman, Citation1945). Mature ascospores were already present in early May, one year after the initial inoculation, which corresponds to bud break of balsam fir trees (Powell, Citation1982; Frank, Citation1990), as reported by the Forest Ministry of Quebec Province. First symptoms on new shoots appeared 6 weeks after the beginning of spore dissemination, which is also in accordance with the description proposed by Forêts Faunes et Parcs Québec. Observations of infections made in Norway and Canada therefore showed similar temporal patterns of spore dissemination.
Isolation of D. balsameae directly from pseudothecia is rarely successful. In the present study, the most frequently isolated fungus was identified as S. polyspora. Talgø et al. (Citation2016) reported that isolation of D. abietis was also arduous since secondary pathogens masked Delphinella growth on culture media. In the present study, the rare colonies of morphotype 2 were associated with D. balsameae which showed a single base pair difference with D. abietis ITS sequence. The possibility that these isolates are involved in fir shoot blight was reinforced by the fact that the same morphotype was obtained by direct isolation from diseased needles as well as in the dissemination assay. This work is the first to report the isolation of D. balsameae supported by genetic evidence.
Comparisons between ITS region sequences of D. balsameae isolates and the only D. abietis sequence (accession number GQ412731) available in the NCBI database revealed a close genetic relatedness between the species. These two pathogenic fungi are phylogenetically related to S. polyspora (Funk, Citation1985). As S. polyspora has been previously identified as the causal agent of the current season needle necrosis of true firs (Talgø et al., Citation2010; Jankowiak et al., Citation2016), its involvement in the development of shoot blight symptoms could thus not be excluded, supporting the importance of developing tools that could discriminate both genera in fir tissues. While the ITS region comparison between D. balsameae and S. polyspora resulted in only few nucleotide dissimilarities in their sequences, other genetic regions such as EF1-α and BTUB, that are often used in phylogenetic studies, allowed their discrimination at a molecular level. The discrepancy in these genetic regions allowed the development of species-specific PCR assays for both D. balsameae and S. polyspora. Both primer pairs DbalBT1F/DbalBT2R and SpolEF4F/SpolEF3R could differentiate their respective targeted fungi from each other, from other endophytic fungi isolated from diseased fir needles and from members of other fungal genera. Unfortunately, some close species that were used for the design of primers were not tested with the detection assays due to the lack of availability of cultures. Detection limits of the assays were 10 pg genomic DNA, which represents similar values to the ones described for detection assays developed for other plant pathogens (Smith & Stanosz, Citation2006; Durán et al., Citation2009; Meng & Wang, Citation2010; Lan et al., Citation2013).
The molecular tools developed to detect the presence of D. balsameae and S. polyspora in fir needles were used in symptomatic and asymptomatic trees. In contrast to S. polyspora that was detected in both, D. balsameae was not detected in healthy trees, providing additional evidence that the fungus is the causal agent of shoot blight disease in Quebec plantations. Furthermore, D. balsameae was usually not detected in asymptomatic needles sampled in trees presenting shoot blight symptoms. This suggests that needle blight probably originates from an initial contamination by D. balsameae ascospores scattered from diseased trees rather than from a systemic infection of the tree. All attempts to obtain D. balsameae pseudothecia and ascospores in culture were unsuccessful, impeding the fulfillment of Koch’s postulates. Nevertheless, the fact that D. balsameae was detected in needles from 50% of the test plants that were exposed to branches carrying pathogen pseudothecia, but not in the tissues of control plants, emphasizes the role of ascospores in the pathogen dissemination. Moreover, the evidence described above complements the original characterization research made on D. balsameae by Waterman (Citation1945). While Waterman’s observations of fruiting bodies were made on trees inoculated with mycelium and afflicted needles, isolation of the pathogen from experimentally infected needles was unsuccessful. Therefore, detection of D. balsameae in infected saplings fulfills one of Koch’s postulates. However, the hypothesis that needle blight symptoms could originate from a systemic infection cannot be ruled out as D. abietis has been detected in fir seeds (Talgø et al., Citation2016).
The S. polyspora detection assay was positive at least at one sampling date for all healthy and diseased trees included in the study. The fungus thus appears to be a common endophyte of fir trees, not only in Quebec, but in other regions of the world (Talgø et al., Citation2010). Sydowia polyspora has also been reported as a frequent fungal species carried by insects (Muñoz-Adalia et al., Citation2017). The high frequency of S. polyspora in the fungal community collected from insects in conifer plantations may thus be due to its abundance in conifer shoots during the insects' feeding period.
Sydowia polyspora was detected in 67% of the tested asymptomatic needles and was associated with all needles exhibiting blight symptoms. The prevalence of S. polyspora in symptomatic needles may indicate a higher biomass in diseased tissue, while the detection threshold could not always be reached in apparently healthy needles. Some interactions between S. polyspora and D. balsameae may influence the emergence of disease development, as has been observed in other plant diseases where endophytes enhance the severity of symptoms caused by a pathogenic agent (Ridout & Newcombe, Citation2015; Busby et al., Citation2016). Sydowia polyspora has been identified as the causal agent of current season needle necrosis (Talgø et al., Citation2010) and was shown to be able to colonize stem phloem (Pan et al., Citation2018). It may thus display an endophytic lifestyle before entering a pathogenic state as observed with other pathogens. For instance, Dothistroma pini and D. septosporum, two closely related needle blight pathogenic agents also included in Dothideomycetes, have been detected on seedlings from nurseries (Millberg et al., Citation2016) and were shown to have an asymptomatic stage before causing visible symptoms (Kabir et al., Citation2015). Another example is Rhabdocline pseudotsugae, a well-known pathogenic agent of Douglas fir (Pseudotsuga menziesii) seedlings and multiple tissues of infected trees (Krabel et al., Citation2013; Morgenstern et al., Citation2013, Citation2014) which implies an endophytic lifestyle prior to becoming a pathogenic fungus.
In this study, strong evidence that D. balsameae is the major pathogen causing needle shoot blight on fir in Quebec province has been presented. The development of detection tools that specifically identify the occurrence of this fungus will enhance the understanding of the infection cycle and other epidemiological aspects. Furthermore, this detection assay will open new ways to discover biotic and abiotic factors that promote the establishment of Delphinella shoot blight in fir trees.
Acknowledgements
JFG gratefully acknowledges the receipt of a Mitacs acceleration scholarship. We would like to thank Dominique Choquette from the Ministère de l’agriculture, des pêcheries et de l’alimentation du Québec for her support to this project, Sylvain Lerat for reviewing the article and two anonymous reviewers who greatly contributed to improve the manuscript.
Additional information
Funding
References
- Agriculture Pêcheries et Alimentation Québec. MAPAQ - Culture des arbres de Noël. [Internet]. revised 2018 May 7; accessed 2018 May 7; accessed 2018 May 7; accessed 2018 Jul 7; accessed 2018 Jul 10. https://www.mapaq.gouv.qc.ca/fr/Productions/Production/culturearbresnoel/Pages/culturearbresnoel.aspx.
- Barr ME. 2001. Revisionary studies on the Dothioraceae. Harvard Pap Bot. 6:25–34.
- Bouchard J, Innes L, Blouin M, Carrière-Pagé PO, Durand J, Dussault C. 2012. Nouvelles détections au laboratoire de pathologie et d’entomologie forestière. Phytoprotection. 92:39.
- Busby PE, Peay KG, Newcombe G. 2016. Common foliar fungi of Populus trichocarpa modify Melampsora rust disease severity. New Phytol. 209:1681–1692.
- Chastagner GA, Riley K, Coats KP, Eikemo H, Talgø V. 2017. Delphinella shoot blight and Grovesiella canker on Abies lasiocarpa in Western USA. Scand J For Res. 32:432–437.
- Durán A, Slippers B, Gryzenhout M, Ahumada R, Drenth A, Wingfield BD, Wingfield MJ. 2009. DNA-based method for rapid identification of the pine pathogen, Phytophthora pinifolia. FEMS Microbiol Lett. 298:99–104.
- Fiddick RL. 1969. Annual district reports. Government of Canada, Department of Fisheries and Forestry, Forest Research Laboratory, Victoria (BC).
- Filip GM, Schmill CL 1990. Rx for Abies: silvicultural options for diseased firs in Oregon and Washington. U.S. For. Serv., PNW-GTR-252. p. 34.
- Forest Research Laboratory. 1966. Annual district reports. Government of Canada, Department of Fisheries and Forestry, Forest Research Laboratory, Victoria (BC): Forest Insect and Disease Survey.
- Forêts, Faune et Parcs Québec. MFFP - La brûlure des pousses du sapin et le rouge des aiguilles. [Internet]. Revised unknown, accessed 2018 Jul 10. http://www.mffp.gouv.qc.ca/forets/fimaq/insectes/fimaq-insectes-maladies-brulure.jsp.
- Frank RM. 1990. Abies balsamea (L.) Mill. Balsam fir. In: Burns RM, Honkala BH, editors. Silvics of North America: 1. Conifers. Washington (DC, USA): U.S. Department of Agriculture, Forest Service; p. 26–35.
- Funk A. 1985. Foliar Fungi of Western Trees. 1st ed. Victoria (BC): Minister of Supply & Services Canada.
- Glass NL, Donaldson GC. 1995. Development of primer sets designed for use with the PCR to amplify conserved genes from filamentous ascomycetes. Appl Environ Microbiol. 61:1323–1330.
- Henson JM, French RC. 1993. The polymerase chain reaction and plant disease diagnosis. Annu Rev Phytopathol. 31:81–109.
- Hyde KD. 2011. Dothideomycetes. Fungal Divers. 51:249–277.
- Jankowiak R, Bilanski P, Paluch J, Kolodziej Z. 2016. Fungi associated with dieback of Abies alba seedlings in naturally regenerating forest ecosystems. Fungal Ecol. 24:61–69.
- Kabir MS, Ganley RJ, Bradshaw RE. 2015. The hemibiotrophic lifestyle of the fungal pine pathogen Dothistroma septosporum. For Pathol. 45:190–202.
- Krabel D, Morgenstern K, Herzog S. 2013. Endophytes in changing environments - do we need new concepts in forest management? iForest - Biogeosciences For. 6:109–112.
- Kumar S, Stecher G, Tamura K. 2016. MEGA7: molecular evolutionary genetics analysis version 7.0 for bigger datasets. Mol Biol Evol. 33:1870–1874.
- Lan CZ, Liu PQ, Li BJ, Chen QH, Weng QY. 2013. Development of a specific PCR assay for the rapid and sensitive detection of Phytophthora capsici. Australas Plant Pathol. 42:379–384.
- Magasi LP. 1974. Forest Fungi Collected in National Parks in the Maritime Provinces. Canadian Forestry Service, Department of the Environment, Fredericton (NB).
- McCartney HA, Foster SJ, Fraaije BA, Ward E. 2003. Molecular diagnostics for fungal plant pathogens. Pest Manag Sci. 59:129–142.
- Meng J, Wang Y. 2010. Rapid detection of Phytophthora nicotianae in infected tobacco tissues and soil samples based on its Ypt1 gene. J Phytopathol. 158:1–7.
- Merrill W, Wenner NG, Kelley R. 1997. Delphinella balsameae tip blight of Abies lasiocarpa in Vermont. Plant Dis. 81:229.
- Millberg H, Hopkins AJM, Boberg J, Davydenko K, Stenlid J. 2016. Disease development of Dothistroma needle blight in seedlings of Pinus sylvestris and Pinus contorta under Nordic conditions. For Pathol. 46:515–521.
- Morgenstern K, Döring M, Krabel D. 2013. Rhabdocline needle cast-investigations on various Douglas fir tissue types. Eur J Plant Pathol. 137:495–504.
- Morgenstern K, Döring M, Krabel D. 2014. Rhabdocline needle cast — most recent findings of the occurrence of Rhabdocline pseudotsugae in Douglas-fir seeds. Botany. 92:465–469.
- Muñoz-Adalia EJ, Sanz-Ros AV, Flores-Pacheco JA, Hantula J, Diez JJ, Vainio EJ, Fernández M. 2017. Sydowia polyspora dominates fungal communities carried by two tomicus species in pine plantations threatened by Fusarium circinatum. Forests. 8:127.
- Natural Resources Canada. 2010. Forest pest management forum. In: Proceedings of the forest pest management forum 2010. Library and Archives Canada. Gatineau (QC): Natural Resources Canada. p. 210.
- Pan Y, Ye H, Lu J, Chen P, Zhou XD, Qiao M, Yu ZF. 2018. Isolation and identification of Sydowia polyspora and its pathogenicity on Pinus yunnanensis in Southwestern China. J Phytopathol. 166:386–395.
- Plante F, Bernier L. 1997. Variability of virulence of Nectria galligena towards northern hardwoods. Eur J For Pathol. 27:261–272.
- Powell GR. 1982. Shoot and bud development in balsam fir: implications for pruning of Christmas trees. For Chron. 58:168–172.
- Rehner SA, Buckley E. 2005. A Beauveria phylogeny inferred from nuclear ITS and EF1-a sequences: evidence for cryptic diversification and links to Cordyceps teleomorphs. Mycologia. 97:84–98.
- Ridout M, Newcombe G. 2015. The frequency of modification of Dothistroma pine needle blight severity by fungi within the native range. For Ecol Manage. 337:153–160.
- Saitou N, Nei M. 1987. The neighbor-joining method: a new method for reconstructing phylogenetic trees. Mol Biol Evol. 4:406–425.
- Sankaran S, Mishra A, Ehsani R, Davis C. 2010. A review of advanced techniques for detecting plant diseases. Comput Electron Agric. 72:1–13.
- Schoch CL, Crous PW, Groenewald JZ, Boehm EWA, Burgess TI, de Gruyter J, de Hoog GS, Dixon LJ, Grube M, Geidan C, et al. 2009. A class-wide phylogenetic assessment of Dothideomycetes. Stud Mycol. 64:1–15.
- Smith DR, Stanosz GR. 2006. . A species-specific PCR assay for detection of Diplodia pinea and D. scrobiculata in dead red and jack pines with collar rot symptoms. Plant Dis. 90:307–313.
- Solheim H. 2003. The needle blight fungus Delphinella abietis attacks Abies species in western Norway. In: Koch NE, editor. Forest health problems in older forest stands. Proceedings of the Nordic/Baltic Forest Pathology Meeting, Denmark, September 2002. Danish Center for Forest, Landscape and Planning; Copenhagen, Denmark, p. 40–43.
- Solheim H, Skage JO. 2002. Losses caused by the needle blight fungus Delphinella abietis in a greenery trial of Nobel fir in western Norway. For Pathol. 32:373–377.
- Statistics Canada. 2017. Christmas trees… by the numbers. [Internet]. accessed 2018 Aug 27. http://www.statcan.gc.ca/eng/dai/smr08/2017/smr08_222_2017.
- Stenström E, Ihrmark K. 2005. Identification of Lophodermium seditiosum and L. pinastri in Swedish forest nurseries using species-specific PCR primers from the ribosomal ITS region. For Pathol. 35:163–172.
- Stielow JB, Lévesque CA, Seifert KA, Meyer W, Iriny L, Smits D, Renfurm R, Verkley GJM, Groenewald M, Chaduli D, et al. 2015. One fungus, which genes? Development and assessment of universal primers for potential secondary fungal DNA barcodes. Persoonia. 35:242–263.
- Talgø V. 2012. Foliage diseases on true fir (Abies spp.) in Norway. J Agric Ext Rural Dev. 4:246–251.
- Talgø V, Chastagner G, Thomsen IM, Cech T, Riley K, Lange K, Klemsdal SS, Stensvand A. 2010. Sydowia polyspora associated with current season needle necrosis (CSNN) on true fir (Abies spp.). Fungal Biol. 114:545–554.
- Talgø V, Skage JO, Steffenrem A, Junker C, Eikemo H, Brurberg MB, Johnskås OR. 2016. Delphinella shoot blight on Abies lasiocarpa provenances in Norway. Forests. 7:1–17.
- Unger L, Vallentgoed J. 1988. Forest insect and disease conditions. Victoria (BC): Nelson Forest Region.
- Unger LS, Fiddick RL. 1978. Forest insect and disease conditions. Prince George Region. Victoria (BC).
- Waterman AM. 1945. Tip blight of species of Abies caused by a new species of Rehmiellopsis. J Agric Res. 70:315–337.
- White TJ, Bruns S, Lee S, Taylor J. 1990. Amplification and direct sequencing of fungal ribosomal RNA genes for phylogenetics. In: Innis MA, Gelfand DH, Sninsky JJ, Thomas JW, editors. PCR protocols: a guide to methods and applications. San Diego: Academic Press; p. 315–322.
- Zeng QY, Hansson P, Wang XR. 2005. Specific and sensitive detection of the conifer pathogen Gremmeniella abietina by nested PCR. BMC Microbiol. 5:65.