Abstract
Clubroot caused by Plasmodiophora brassicae is an economically important disease of crucifer crops throughout the world and is a major threat to the quality and yield of cabbage (Brassica oleracea var. capitata L.) in China. Host resistance is essential for clubroot management. Although two cabbage lines (clubroot-resistant line CR21 and clubroot-susceptible line CS54) have been identified through screening 80 cabbage accessions, the molecular mechanisms underlying differential responses to clubroot remain elusive. Herein, RNA-seq analysis was performed to characterize the transcriptomic changes in CR21 and CS54 at 3 days after P. brassicae inoculation (DAI). In total, 1057 and 4741 differentially expressed genes (DEGs) were found in CR21 and CS54, respectively. Moreover, 541 genes were up-regulated in CR21, but showed either no significant change or were down-regulated in CS54. Gene Ontology (GO) analysis showed that most DEGs were involved in metabolism, transport, signal transduction and defence. Compared with the identified putative defence response genes in Arabidopsis thaliana, 165 resistance-related DEGs were obtained, including PAMPs-triggered immunity (PTI)-related genes and Effector-triggered-immunity (ETI)-related genes. Interestingly, the co-supported defence response DEGs contained the defence-related genes, wound response genes, RBOHB, WRKY28, TIR-NBS-LRR class (RPS4 etc.), pathogenesis-related (PR) gene (Bo7g005050), lipid transfer protein class (LTP1 etc.), plant hormone-related genes, ethylene signalling transduction (HRE1-like gene and EIN3), ABA signalling (PP2C and PYL13), and cell wall modification related genes (EXP17 and PME44, beta-1,3-glucanase and cytochrome P450 genes). Taken together, this study provided a comprehensive insight into transcriptomic responses to P. brassicae infection in two different cabbage lines, and identified several candidate genes that might contribute to clubroot resistance in cabbage and other Brassica crops.
Résumé
La hernie, causée par Plasmodiophora brassicae, est une importante maladie des crucifères au point de vue économique, et ce, partout dans le monde. En Chine, elle est une grave menace à la qualité et au rendement du chou (Brassica oleracea var. capitata L.). La résistance de l’hôte est essentielle à la gestion de la hernie. Bien que deux lignées de chou (lignée résistante à la hernie CR21 et lignée réceptive à l’égard de la hernie CS54) eussent été identifiées après avoir criblé 80 accessions, les mécanismes moléculaires qui sont à la base des différentes réactions à la hernie demeurent ambigus. Ici, l’analyze Séq. ARN a été utilisée pour caractériser les changements transcriptomiques chez CR21 et CS54, trois jours après inoculation avec P. brassicae. En tout, 1057 et 4741 gènes exprimés différemment (GED) ont été trouvés chez CR21 et CS54, respectivement. En outre, 541 gènes ont été régulés à la hausse chez CR21, mais n’ont affiché aucun changement important ou ont été régulés à la baisse chez CS54. L’analyze découlant de Gene Ontology a montré que la plupart des GED étaient impliqués dans le métabolisme, le transport, la transduction du signal et la défense. Comparativement aux gènes putatifs impliqués dans les réactions de défense chez Arabidopsis thaliana, 165 GED associés à la résistance ont été obtenus, y compris des gènes associés à l’immunité déclenchée par les PAMP et des gènes associés à l’immunité déclenchée par des effecteurs. Chose intéressante, les GED soutenus conjointement, issus de la réaction de défense, contenaient les gènes associés à la défense, ceux associés aux blessures, ceux de la classe des RBOHB, WRKY28, TIR-NBS-LRR (RPS4, etc.), le gène associé à la pathogenèse (Bo7g005050), la classe des protéines de transfert des lipides (LTP1, etc.), les gènes associés aux hormones végétales, la transduction du signal éthylène (gène semblable à HRE1 et EIN3), les molécules de signalisation ABA (PP2C et PYL13) et les gènes associés à la modification de la membrane cellulaire (gènes EXP17 et PME44, beta-1,3-glucanase et cytochrome P450). Prise dans son ensemble, cette étude a fourni un aperçu détaillé des réactions transcriptomiques à l’infection causée par P. brassicae dans deux lignées différentes de chou et ciblé plusieurs gènes susceptibles de contribuer au développement de la résistance à la hernie chez le chou et d’autres plantes de la famille des crucifères.
Introduction
Plasmodiophora brassicae Woronin, a soil-borne biotrophic protist in the eukaryotic kingdom of Rhizaria, is the causal agent of clubroot disease in cabbage, cauliflower, radish and other crucifer plants (Dixon, Citation2009; Burki et al., Citation2010). This protist infects Brassica crops mainly by two steps: primary infection and secondary infection. During the primary infection stage, the primary zoospores adhere to the surface of root hairs and each inject into the cell an amoeboid unit, which undergoes multiple cell divisions to form multinucleate primary plasmodia. Then the primary plasmodia are further cleaved into secondary zoospores. During the secondary infection, the secondary zoospores invade the cortical tissues of the main roots and develop into secondary plasmodia, causing gall formation (Kageyama & Asano, Citation2009). Notably, the resting spores released from decayed galls are rich in lipid droplets, which presumably contribute to its persistence in soil, further complicating management of clubroot disease (Hwang et al., Citation2014; Bi et al., Citation2016).
Given the enormous economic impact caused by clubroot, many efforts have been devoted to develop proper measures for controlling clubroot disease. Cultivating resistant cultivars is the most cost-effective and environmentally friendly way to prevent or mitigate clubroot (Peng et al., Citation2014; Liu et al. Citation2018b). The development of resistant cultivars largely depends on the identification of clubroot resistance (CR) loci, which has been documented in B. rapa, B. napus and B. oleracea (Piao et al., Citation2009). The 10 CR loci documented in B. rapa are Crr1 (Crr1aand Crr1b), Crr2, Crr3, Crr4, CRa, CRb, CRk, PbBa3.1 and PbBa3.3, and most of them were identified in European fodder turnips (B. rapa ssp. rapifera) (Piao et al., Citation2009; Chen et al., Citation2013). Three of these, CRa, Crr1a, CRb have been characterized as classical resistance genes encoding Toll-interleukin receptor (TIR)-nucleotide-binding site (NBS)-leucine-rich repeat (LRR) (Ueno et al., Citation2012; Hatakeyama et al., Citation2013, Citation2017). However, the resistance mechanisms to P. brassicae infection associated with these three CR genes have yet to be clarified.
Analysis of global gene expression provides another avenue for exploring the molecular mechanism of interactions between the host plant and P. brassicae, particularly with regard to mechanisms of resistance and the basal defence response. For example, at 4, 7 and 10 days after inoculation (DAI) with P. brassicae, a microarray analysis of Arabidopsis thaliana revealed a larger number of DEGs at 4 DAI (Agarwal et al., Citation2011). A study using the complete Arabidopsis transcriptome microarray (CATMA) showed that when compared with immune response or susceptible response, metabolic changes in the partial resistance response were reduced or delayed, and abnormal cell enlargement and proliferation were actively inhibited at 7 DAI on A. thaliana (Bur-0) (Jubault et al., Citation2013). Based on microarray data and laser microdissection of Arabidopsis roots, Schuller et al. (Citation2014) confirmed that genes involved in auxin and cytokinin metabolism and signalling were up-regulated to promote clubroot development. A study using a proteomic approach indicates that the perception of P. brassicae and activation of defence responses were triggered via a unique signalling pathway distinct from common modes of recognition receptors reported with many other plant–pathogen interactions, and may require the ubiquitin-26S proteasome (Song et al., Citation2016). Recently, Chen et al. (Citation2016) and Jia et al. (Citation2017) elucidated differential responses of clubroot-resistant and -susceptible near-isogenic lines and doubled haploid lines in B. rapa during P. brassicae early infection, respectively. However, understanding of the interaction between cabbage (Brassica oleracea var. capitata L.) and P. brassicae is lacking, due to limited sources of resistance in cabbage.
In a previous study, we obtained a clubroot-resistant line CR21 by screening 80 cabbage accessions (Wang et al., Citation2016). In this study, we used CR21 and a clubroot-susceptible line CS54 in a transcriptomic analysis at the early stage of P. brassicae infection (3 DAI) to explore the differences in gene expression between the two lines and the molecular mechanisms underlying resistance to P. brassicae infection in CR21.
Materials and methods
Plant and pathogen materials
Two self-incompatible cabbage lines were selected in this study. One was highly resistant (CR21) and the other one was highly susceptible (CS54) to clubroot. Both lines were spheroidal and introduced from Japan. Under identical inoculum pressure, the clubroot incidence was 5.56% on CR21 and 100% on CS54 (Wang et al., Citation2016). The P. brassicae strain was collected from Lichuan (30°20′N, 109°36′E, Hubei Province) and identified as ECD16/4/0 according to European Clubroot Differential Set (Buczacki et al., Citation1975). The pathogen was routinely propagated on CS54 and stored at −20°C for further use.
Preparation of resting spore suspension for inoculation
For collection of resting spores of P. brassicae, the clubs were blended in distilled water and passed through eight layers of cheesecloth. After centrifugation at 2000 rpm for 5 min, the pellet was re-suspended with distilled water to make resting spore suspension (1 × 106 mL−1) for inoculation. Inoculation of cabbage was performed as described previously with modifications (Ji et al., Citation2014). After germinating on moist filter paper for 5 days, seedlings of CR21 and CS54 were transplanted to Hoagland’s nutrient solution (pH 7.0) to grow for another 7 days. Then the cabbage seedlings were cultured with the 1 × 106 mL−1 resting spore suspension (pH 6.2–6.5) for 24 h, followed by transfer of seedlings into Hoagland’s nutrient solution (pH 6.2–6.5) for 3 days. The uninfected plants cultured with Hoagland’s nutrient solution (pH 6.2–6.5) for 3 days were served as the control. After that, infected and uninfected root samples of CR21 and CS54 were collected and used for further analysis. Each time point with two biological replicates was used to eliminate the variability during sampling process, and each biological replicate was comprised of 10 plants.
Microscopic investigation
To confirm the infection process of P. brassicae in cabbage roots, root samples were washed thoroughly with distilled water to remove spores, stained with aniline blue (125 mg L−1 aniline blue in 50% acetic acid), rinsed again with distilled water, and subjected to microscopic observation (Olympus BX41, Japan). Each sample from three individual plants was used.
RNA isolation, cDNA library construction and sequencing
Total RNA was extracted using the TRIzol reagent (Invitrogen, Carlsbad, CA, USA) according to the manufacturer’s instructions. RNA quality was determined using a Nanodrop spectrophotometer (Thermo Fisher Scientific, Wilmington, MA), 1% formaldehyde gel electrophoresis and a 2100 Bioanalyzer (Agilent Technologies, Santa Clara, CA). cDNA library construction and sequencing were conducted by the Gene Denovo Biological Technology Co., Ltd (Guangzhou, China). mRNA was isolated using beads with Oligo (dT), and then broken into short fragments. These short fragments of mRNA were used as templates to synthesize the first-strand cDNA with random primers. Second-strand cDNA was synthesized in a reaction. The resulting cDNA was then treated with end-repair polymerase and mixed with Solexa adapters. Suitable fragments were recovered from an agarose gel. Next, PCR amplification was performed to enrich the purified cDNA template. Finally, all the eight libraries, S3 mock-1, S3 mock-2, S3-1, S3-2, R3 mock-1, R3 mock-2, R3-1 and R3-2 (S, CS54; R, CR21) from two cabbage lines with two biological replicates (−1, −2) were sequenced by Illumina HiSeq™ 2500, paired-end 125. All the raw data of this project have been deposited at NCBI under the accession number SRP119672.
Alignment and annotation
Raw reads were filtered by removing adapter reads and low quality reads in which the percentage of low Q-value (≤ 10) base was more than 50%. Clean reads were mapped to the reference genome (http://plants.ensembl.org/Brassica_oleracea) by TopHat2 (Parkin et al., Citation2014). Not more than two mismatched bases were permitted, and unique mapped reads were obtained. The number of unique mapped reads was calculated and normalized to RPKM (reads per kb per million reads) for gene expression analysis.
Differentially expressed gene analysis
The DEseq R package was used to perform the differential expression analysis of the mock and infected library of CR21 and CS54 at primary infection stages for comparisons among samples with two biological replicates (Anders et al., Citation2010). Adjusted P-value of 0.05 was set as the threshold for determination of differentially expressed genes (DEGs) by default (padj < 0.05) (Benjamini & Yekutieli, Citation2001). The false discovery rate (FDR) was applied to determine the P-value threshold in multiple tests and analysis. We used FDR< 0.001 and absolute value of the |log2 ratio| > 1 as the threshold to determine the significant difference in gene expression. GO annotation and functional classification of the DEGs were analysed by Blast2GO software. KOBAS 3.0 web server (http://kobas.cbi.pku.edu.cn/) was used for gene functional annotation and functional gene set enrichment.
Quantitative RT-PCR analysis
The eight samples for RNA-seq were analysed by qRT-PCR for the expression of 10 selected genes. The qRT-PCR reactions were performed using Maxima SYBR Green qPCR MasterMix (RR820A, Takara, Dalian, China) on a StepOnePlus Real-Time PCR system (Thermo Fisher Scientific). The primers are listed in Table S1. Three technical replicates and two biological replicates were conducted. BoACTIN2 was used as an internal control. The conditions for amplification were as follows: 10 min denaturation at 95°C, followed by 45 cycles of 95°C for 15 s, 55°C for 15 s and 72°C for 45 s. Following amplification, dissociation was performed by 1 cycle of 95°C for 15 s, 60°C for 30 s and 95°C for 15 s to confirm the specificity of the PCR amplification. The fold change was estimated in terms of threshold cycles by the 2− ΔΔCT method (Livak & Schmittgen, Citation2001).
Results
Histological examination of cabbage roots after P. brassicae inoculation
To determine the appropriate time points for RNA-seq analysis and to understand the mechanisms of resistance conferred by CR21, histological analysis was employed to investigate the differences between cabbage lines CR21 and CS54 in the presence or absence of P. brassicae challenge. In the absence of pathogen, both lines show normal root growth, but the number of root hairs in CR21 was greater than that in CS54. In the presence of pathogen, primary zoospores attached on root hairs were visible in both lines, and this stage (3 DAI) was called primary infection stage (). It has been reported that root hair attachment and penetration can occur from day 4 onwards after P. brassicae inoculation (Agarwal et al., Citation2009). Another study by Agarwal et al. (Citation2011) indicated that most DEGs were detected at 4 DAI, during which root hair attachment and penetration by P. brassicae occurs. In cabbage, we found that root hair attachment and penetration occurred at 3 DAI through microscopic examination; therefore, sampling was conducted at 3 DAI to maximize the number of DEGs to be detected.
Digital overview analysis of sequences from cDNA libraries
To further elucidate the initial molecular response to clubroot, cDNA libraries of CR21 and CS54 at 3 DAI and non-inoculated treatments were constructed and analysed by RNA-seq. The Pearson correlation between two biological replicates was 0.9656, 0.9893, 0.9812 and 0.9781, indicating the high reliability of experimental results and stability of operating (Fig. S1). Based on Illumina sequencing, a total of 351.25 million raw reads were generated by 125 bp pair-end sequencing (Table S2). After discarding the adaptor sequences and low quality reads, about 350.01 million high quality (HQ) clean reads were generated from the eight cDNA libraries (Table S2). GC% of HQ clean data from all libraries was around 48%, and Q30% were all larger than 92%, indicating that the accuracy and quality of the sequencing data were sufficient for further analysis. Then, we aligned the HQ clean data with rRNA database and deleted a few mapped reads. After that, about 346.58 million reads (rRNA data excluded) were generated, and ~79% of the sequenced reads were successfully mapped to the B. oleracea reference genome ().
Table 1. Statistics of the sequenced reads and comparison to the B. oleracea reference genome.
Approximately 35 000 genes were mapped to the reference genome based on sequence reads, among which the number of known genes mapped to the B. oleracea genome was around 33 000 and the number of new genes was around 1,700 (Table S3). When the two biological replicates were grouped together, the number of total mapped genes of R3 mock, R3, S3 mock and S3 libraries were 36 091, 36 789, 36 562 and 36 200, respectively. The number of new genes of R3 mock, R3, S3 mock and S3 libraries were 1741, 1751, 1809 and 1750 (Table S3; Fig. S2).
Transcriptome analysis of cabbage in response to P. brassicae
The total number of DEGs in the clubroot-resistant and clubroot-susceptible cabbage lines was estimated. A total number of 1057 DEGs and 4741 DEGs were identified in CR21 (R3-vs-R3 mock) and CS54 (S3-vs-S3 mock) at 3 DAI, respectively. In CR21, 587 DEGs were up-regulated and 470 DEGs were down-regulated when compared with mock. In CS54, 1699 DEGs were up-regulated and 3042 DEGs were down-regulated (). In addition, a total of 336 DEGs were detected as common DEGs in both lines, and 721 and 4405 DEGs were unique in CR21 and CS54, respectively.
Fig. 2 (Colour online) Number of DEGs in the clubroot-resistant cabbage line CR21 and clubroot-susceptible cabbage line CS54. A, number of up-regulated genes and down-regulated genes (FDR < 0.001 and fold change > 2.0 or < −2.0) in CR21 or CS54 following P. brassicae challenge. B, the number of DEGs and common DEGs in the two cabbage lines. Numerals inside the parentheses indicate the number of genes expressed at 3 DAI. R3 and S3 indicate pathogen-challenged CR21 and CS54 respectively while R3 mock and S3 mock indicate mock controls of CR21 and CS54, respectively.
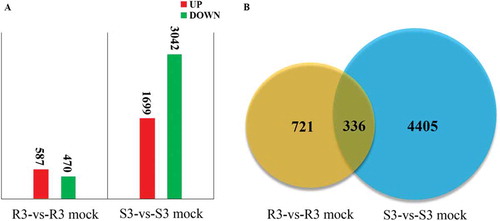
Functional annotation of DEGs
All of the 5462 DEGs were annotated by searching through the NCBI database (https://www.ncbi.nlm.nih.gov) and the Brassica database (http://brassicadb.org/brad). In the resistant line, 811 (76.73%) DEGs were found to have significant matches in the GO database (Table S4), while there were 3315 (66.92%) DEGs that matched significantly in the susceptible line (Table S5). Based on GO terms, all of the matched DEGs were assigned to three GO classes: biological process, cellular component and molecular function. In the biological process categories, ‘metabolic process’, ‘cellular process’ and ‘biological regulation’ represented the top three highly expressed subcategories (). ‘Cell’ and ‘cell part’ were among the most commonly represented cellular components categories. Most of the genes categorized under molecular function were involved in ‘catalytic activity’ and ‘binding’.
Fig. 3 (Colour online) GO assignment of DEGs in clubroot-resistant cabbage line CR21 (a) and clubroot-susceptible cabbage line CS54 (b). The unigenes were assigned to three main categories: biological process, cellular component and molecular function. The x-axis indicates the number of annotated DEGs.
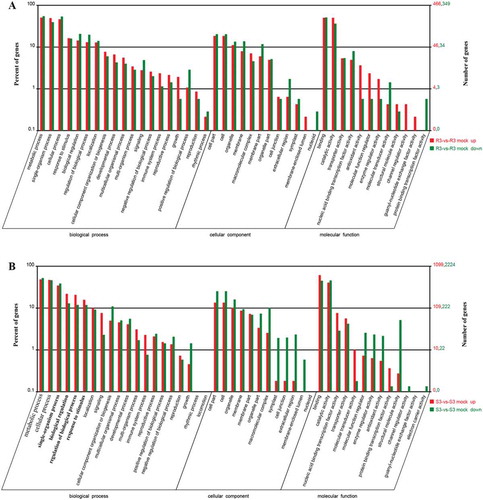
To identify the biological pathways that are active in cabbage, we mapped the 246 (23.27%) DEGs of CR21 (Table S4) and 916 (19.32%) of CS54 (Table S5) to the reference canonical pathways in KEGG. In CR21, a total of 246 DEGs were annotated in the KEGG database and assigned to 86 KEGG pathways, of which 16 pathways with P ≤ 0.01 were detected. In total, 916 DEGs in the CS54 line were annotated and assigned to 116 KEGG pathways, of which only 15 pathways with P ≤ 0.01 were found. ‘Metabolic pathways’ was the most enriched KEGG pathway, containing 155 (43.79%) and 449 (31.96%) DEGs in CR21 and CS54 respectively, followed by ‘Biosynthesis of secondary metabolites’ (93 in CR21, 271 in CS54). Here, co-clustered ‘Glycolysis/Gluconeogenesis’ related to respiration, ‘Glycolysis/Gluconeogenesis’, ‘Phenylalanine metabolism’, ‘Stilbenoid, diarylheptanoid and gingerol biosynthesis’, ‘Pentose and glucuronate interconversions’, ‘Carbon fixation in photosynthetic organisms’, ‘Starch and sucrose metabolism’, ‘Plant hormone signal transduction’ and ‘Plant-pathogen interaction’ were the cabbage basic response to P. brassicae. Pathways like ‘Limonene and pinene degradation’, ‘Cysteine and methionine metabolism’, ‘Pyruvate metabolism’, ‘DNA replication’ were enriched in CR21, presumably involved in incompatible interactions with P. brassicae. Meanwhile, pathways like ‘Ribosome’, ‘Amino sugar and nucleotide sugar metabolism’, ‘Protein processing in endoplasmic reticulum’ were enriched in CS54, presumably involved in compatible interactions with P. brassicae ().
Table 2. The enriched KEGG pathways in the two cabbage lines.
DEGs involved in resistance to P. brassicae
There were five expression patterns of the 1057 DEGs in response to P. brassicae challenge (). There were 721 genes (68.21%) expressed differentially only in CR21 with 368 genes up-regulated and 353 genes down-regulated. In total, 111 genes showed the same trend (46 genes up-regulated and 65 genes down-regulated) in CR21 and CS54. While 173 genes were up-regulated in CR21, but down-regulated in CS54. There were 52 genes down-regulated in CR21, but up-regulated in CS54. In total, there were 541 genes up-regulated in CR21, but down-regulated (173) or non-differentially expressed (368) in CS54.
Fig. 4 (Colour online) The five expression patterns of DEGs in clubroot-resistant cabbage line CR21 compared with the clubroot-susceptible cabbage line CS54. Numerals inside the parentheses indicate the number of genes expressed at 3 DAI. The total number of DEGs is noted in each pillar.
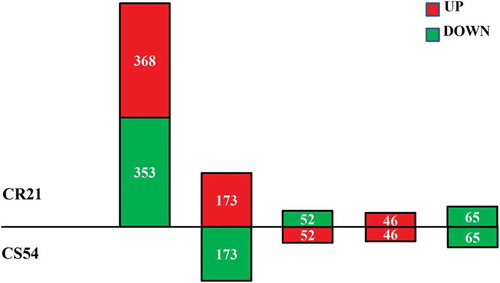
To gain a deeper insight into the defence molecular mechanisms in B. oleracea, 541 DEGs were further divided into two groups (, Tables S6, S7, S8): DEGs up-regulated in clubroot resistant CR21 but showed no detectable changes in CS54 (UR/NS) or DEGs up-regulated in clubroot resistant CR21 but down-regulated in clubroot susceptible CS54 (UR/DS). A large number of DEGs were involved in the metabolism. The remaining 165 genes were related to disease resistance responses. Two pattern recognition receptors (PRRs) in group UR/NS, three TIR-NBS-LRR (two in UR/NS, one in UR/DS), five LRR (four in UR/NS, one in UR/DS), four Ca2+ influx genes in UR/NS, one MAPK cascades in UR/DS, 12 hormone signal transduction factors, two brassinosteroid synthesis related genes (one in UR/NS and one in UR/DS), 27 cell wall modification related genes were detected.
Validation of differentially expressed genes by qRT-PCR
To confirm the differential expression profiles of DEGs from RNA-seq, 10 DEGs were randomly selected for qRT-PCR assays (Table S1), including genes from cytokinin signalling pathway (BoHK3, Bo1g055190), pathogenesis-related genes (BoCAPE5, Bo2g030230; BoPR5, Bo5g027350), PPR gene (BoRLP30, Bo5g025040), ethylene signalling pathway (BoEIN3, Bo3g100500), Ca2+ influx related gene (BoACA9, Bo3g023040), MYC transcription factor (BoJAI1, Bo5g086990), MAPK signalling pathway (BoMAPKKK13, Bo5g007990), and cell wall-related gene (BoCESA7, Bo2g013670; BoXTH7, Bo7g118760). All 10 DEGs showed the same expression tendency as in the RNA-seq analysis ().
Discussion
Transcriptomic profiling of crucifer crops in response to P. brassicae is a valuable tool for understanding the basis of quantitative resistance. In the current study, 5462 DEGs were identified between clubroot-resistant and -susceptible cabbage accessions. Interestingly, the DEGs in the susceptible line CS54 were significantly more than that in the resistant line CR21. One possible explanation is that the growth of CS54 was disrupted by P. brassicae invasion; therefore, more genes were differentially expressed.
As PTI (PAMPs-triggered immunity) and ETI (Effector-triggered-immunity) constitute the major defence systems in plant responses to pathogen invasion (Jones & Dangl, Citation2006), DEGs related to PTI and ETI were further investigated. A total of 165 DEGs involved in PTI and ETI were found in the UR/NS and UR/DS expression pattern in resistant cabbage line CR21.
PRRs play a fundamental role and are a key step in the early stages of PTI. The two key genes related to PRRs were induced at 3 DAI with the UR/NS pattern, one was concanavalin A-like lectin protein kinase (Bo2g025000), and the other was cysteine-rich receptor like protein kinase 37 (CRK37, Bo3g048450). Surprisingly, the key PRR genes, such as brassinosteroid insensitive 1-associated kinase 1 (BAK1), chitin elicitor receptor kinase (CERK) and flagellin sensing 2 (FLS2) (Dodds & Rathjen, Citation2010), which transduce signals that trigger PTI, showed no difference between clubroot-resistant and -susceptible cabbage. These phenomena were also observed in the interaction between B. rapa and P. brassicae (Chen et al., Citation2016).
In the signal transduction pathways, calcium serves as an essential second messenger that regulates plant responses to abiotic and biotic stresses (Clay et al., Citation2009; Zipfel, Citation2009). The CBL (calcineurin B-like proteins)-interacting protein kinase (CIPK21, CIPK13), Calcium-binding endonuclease/exonuclease/phosphatase and Calcium-dependent protein kinase (CDPK), were up-regulated in response to P. brassicae at 3 DAI. Ca2+ can activate respiratory burst oxidase homologue (RBOH) protein which is a key player in the production of reactive oxygen species during the plant response (Sagi & Fluhr, Citation2001). Two RBOH genes showed elevated expression with UR/NS pattern. This result was in agreement with previous studies in B. rapa (Chen et al., Citation2016), and soybean (Kim et al., Citation2011). It indicated that a higher ROS level was involved in the resistance response, thereby inhibiting the root invasion by the pathogen (You & Chan, Citation2015). In our study, 12 peroxidase (POD) genes on chromosomes No. 1, 2, 3, 7, 9 of cabbage, were highly expressed in the two significant UR/NS and UR/DS patterns.
Chitin is the main component of the cell wall in P. brassicae resting spores and known to function as PAMP in many other pathogens (Latge & Beauvais, Citation2014). Three chitinases were elevated in the UR/NS and UR/DS patterns in this study. In Chinese cabbage, chitinase genes were differentially regulated in response to P. brassicae (Chen et al., Citation2016), so it has yet to be investigated what the role of different chitinase subfamilies is in the interaction between P. brassica and Brassica plants.
The second type of plant immunity mechanism ETI is driven by plant disease resistance proteins (major R gene products) that recognize directly or indirectly specific pathogen-derived effectors (Pandey & Somssich, Citation2009). Thirteen positively regulated R proteins were found in the UR/NS and UR/DS patterns of CR21, including three TIR-NBS-LRR genes, five LRR-kinase genes and five protein kinase genes.
Defence-related genes have been shown to act in plant immune responses to pathogen attack. Twenty cabbage genes homologous to defence-related genes were up-regulated in CR21 after P. brassicae infection. Among these genes, there was one pathogenesis-related gene, two pathogenesis-related thaumatin family protein genes and 17 lipid transfer protein (LTP) genes. The number of differentially expressed PR genes was less in cabbage than in Chinese cabbage during early stages of P. brassicae infection (Chen et al., Citation2016; Jia et al., Citation2017), but more lipid transfer proteins are found, which may change membrane permeabilization in the pathogen to inhibit fungal growth like the Ha-AP10 in sunflower (Regente et al., Citation2005). As LTPs take part in the signal transduction process of host hypersensitive response (Blein et al., Citation2002), LTPs might also be vital for mediating resistance against P. brassicae in cabbage.
The hormone salicylic acid (SA)-, jasmonic acid (JA)- and ethylene-mediated signalling pathways are important for plant defence gene expression regulation as they function downstream in PTI and ETI. These pathways are well studied compared with other immunity processes (Aarts et al., Citation1998; Gfeller et al., Citation2010). At 3 DAI, several genes were up-regulated, including those involved in auxin (IAA), ethylene (Eth), brassinosteroid (BR) and abscisic acid (ABA) metabolism in UR/NS and UR/DS patterns of CR21.
Plant defence responses may be tailored to the attacking pathogen, with SA-dependent defences acting against biotrophs, and JA-, ET-dependent responses acting against necrotrophs (Glazebrook, Citation2005). As P. brassicae is an obligate biotrophic protist, firstly, we examined the expression pattern of SA-related DEGs to explore whether a SA-mediated pathway was involved in the CR21 resistance to P. brassicae. NPR1 (non-expressor of PR gene 1) is a key player in SA signalling and ICS1 (isochorismate synthase 1) is a key enzyme for SA biosynthesis (Glazebrook, Citation2005). However, both NPR1 and ICS1 were not significantly elevated in cabbage. We speculated that the genes related to a SA signalling pathway might be involved in resistance progress at the later stage (Jia et al., Citation2017). ACO (1-aminocyclopropane-1-carboxylic acid oxidase) and ACS (1-amino-cyclopropane-1-carboxylate synthase) function primarily by promoting ethylene synthesis (Bleecker & Kende, Citation2000). Three ACOs and four ACSs were up-regulated in CR21 and several genes in the ethylene-mediated pathway were also up-regulated, including EIN3 (Ethylene insensitive 3), ETR (Ethylene response), HRE (Hypoxia responsive ethylene response factor), which could confer resistance to pathogens like Penicillium digitatum (Lu et al., Citation2014). Brassinolide (BL) plays an important role in cell division, elongation and stress response to pathogens. In our study, two BR6OX2 (brassinosteroid-6-oxidase 2) responsible for BL biosynthesis were up-regulated, which was different from B. rapa (Chen et al., Citation2016; Jia et al., Citation2017).
Some genes involved in cell division and expansion were up-regulated in A. thaliana after P. brassicae infection (Siemens et al., Citation2006), but in clubroot-resistant B. rapa, most genes related to cell division and expansion were down-regulated (Chen et al., Citation2016). Here we identified some vital genes up-regulated in the two UR/NS and UR/DS patterns of CR21. Nine expansin genes, which were cell wall loosening agents or influencing root hair initiation and root growth, were expressed (Cho & Cosgrove, Citation2002; Marowa et al., Citation2016). These genes have been reported to be involved in the resistance to P. brassicae (Irani et al., Citation2018), and the resistance to virus in tobacco (Chen et al., Citation2018). Four pectin methylesterases which change pectin pattern to regulate the permeability and stability of cell walls (Pelloux et al., Citation2007), were also up-regulated in B. rapa under P. brassicae challenge. Pectin lyase catalyses the cleavage of pectin (Louvet et al., Citation2006), and 13 pectin lyases were found, which were not reported in B. rapa (Chen et al., Citation2016; Jia et al., Citation2017). There was only one cellulose synthase involved in repairing cell wall through reinforcing cellulose, in agreement with a study on Arabidopsis (Scheible et al., Citation2003). We hypothesized that cell wall modification in response to P. brassicae invasion had occurred. Five wound response genes were actively regulated in response to P. brassicae infection. This wound response was also observed in tomato (Chen et al., Citation2014; Liu et al., Citation2018a).
Roots are the major infection site by P. brassicae (Kageyama & Asano, Citation2009). At 3 DAI, root hair infection took place in CR21, accompanied by the up-regulation of wound response genes; therefore, many genes related to root development were expressed in the UR/NS and UR/DS patterns. Besides, one MYB72 was obtained. MYB72 is known to regulate the production of coumarin, influencing the beneficial microbes to enhance plant root growth and protection (Stringlis et al., Citation2018).
In summary, we investigated the transcriptomic responses in roots of clubroot-resistant and clubroot-susceptible cabbage lines at the early stage of P. brassicae infection, and identified several resistance-related genes. These genes are involved in pathogen recognition, cell wall modification, plant hormone signalling, generation of reactive oxygen species and transcriptional regulation. Overall, data generated from this study provide comprehensive insights into the transcriptomic landscape to reveal molecular mechanisms concerned with pathogen resistance and/or susceptibility after P. brassicae infection in two different cabbage accessions.
supplementary_materials.rar
Download (1.5 MB)Acknowledgements
We thank Dr Xiaolin Yu and Dr Li Huang from Zhejiang University for critical reading of this manuscript. We are also grateful to the anonymous reviewers and section editor for their valuable comments.
Supplemental material
Supplemental data for this article can be accessed online here: https://doi.org/10.1080/07060661.2019.1567592.
Additional information
Funding
References
- Aarts N, Metz M, Holub E, Staskawicz BJ, Daniels MJ, Parker JE. 1998. Different requirements for EDS1 and NDR1 by disease resistance genes define at least two R gene-mediated signaling pathways in Arabidopsis. Proc Natl Acad Sci USA. 95:10306–10311.
- Agarwal A, Kaul V, Faggian R, Cahill DM. 2009. Development and use of a model system to monitor clubroot disease progression with an australian field population of plasmodiophora brassicae. Australas Plant Path. 38:120-127.
- Agarwal A, Kaul V, Faggian R, Rookes JE, Ludwigmuller J, Cahill DM. 2011. Analysis of global host gene expression during the primary phase of the Arabidopsis thaliana–plasmodiophora brassicae interaction. Func Plant Biol. 38:462–478.
- Anders S, Huber W, Halsall JA, Page P, Illingworth RS, Taylor AMR, Davison V, O’Neill LP, Turner BM. 2010. Differential expression analysis for sequence count data. Genome Biol. 11:R106.
- Benjamini Y, Yekutieli D. 2001. The control of the false discovery rate in multiple testing under dependency. Ann Stat. 29:1165–1188.
- Bi K, He Z, Gao Z, Zhao Y, Fu Y, Cheng J, Xie J, Jiang D, Chen T. 2016. Integrated omics study of lipid droplets from Plasmodiophora brassicae. Sci Rep. 6:36965.
- Bleecker AB, Kende H. 2000. Ethylene: a gaseous signal molecule in plants. Annu Rev Cell Dev Biol. 16:1–18.
- Blein J, Coutosthevenot P, Marion D, Ponchet M. 2002. From elicitins to lipid-transfer proteins: a new insight in cell signalling involved in plant defence mechanisms. Trends Plant Sci. 7:293–296.
- Buczacki ST, Toxopeus H, Mattusch P, Johnston TD, Dixon GR, Hobolth LA. 1975. Study of physiologic specialization in Plasmodiophora brassicae: proposals for attempted rationalization through an international approach. Trans Brit Mycol Soc. 65:295–303.
- Burki F, Kudryavtsev A, Matz MV, Aglyamova GV, Bulman S, Fiers M, Keeling PJ, Pawlowski J. 2010. Evolution of Rhizaria: new insights from phylogenomic analysis of uncultivated protists. BMC Evol Biol. 10:377.
- Chen J, Jing J, Zhan Z, Zhang T, Zhang C, Piao Z, Schönbach C. 2013. Identification of novel QTLs for isolate-specific partial resistance to Plasmodiophora brassicae in Brassica rapa. PLoS One. 8:e85307.
- Chen J, Pang W, Chen B, Zhang C, Piao Z. 2016. Transcriptome analysis of Brassica rapa near-isogenic lines carrying clubroot-resistant and -susceptible alleles in response to Plasmodiophora brassicae during early infection. Front Plant Sci. 6:1183.
- Chen L, Zou W, Wu G, Lin H, Xi D. 2018. Tobacco alpha-expansin EXPA4 plays a role in Nicotiana benthamiana defence against Tobacco mosaic virus. Planta. 247:355–368.
- Chen Y, Lee C, Cheng K, Chang W, Huang R, Nam HG, Chen Y. 2014. Quantitative peptidomics study reveals that a wound-induced peptide from PR-1 regulates immune signaling in tomato. Plant Cell. 26:4135–4148.
- Cho H, Cosgrove DJ. 2002. Regulation of root hair initiation and expansin gene expression in Arabidopsis. Plant Cell. 14:3237–3253.
- Clay NK, Adio AM, Denoux C, Jander G, Ausubel FM. 2009. Glucosinolate metabolites required for an Arabidopsis innate immune response. Science. 323:95–101.
- Dixon GR. 2009. The occurrence and economic impact of Plasmodiophora brassicae and clubroot disease. J Plant Growth Regul. 28:194–202.
- Dodds PN, Rathjen JP. 2010. Plant immunity: towards an integrated view of plant-pathogen interactions. Nat Rev Genet. 11:539–548.
- Gfeller A, Liechti R, Farmer EE. 2010. Arabidopsis jasmonate signaling pathway. Sci Signal. 3:cm4.
- Glazebrook J. 2005. Contrasting mechanisms of defense against biotrophic and necrotrophic pathogens. Annu Rev Phytopathol. 43:205–227.
- Hatakeyama K, Niwa T, Kato T, Ohara T, Kakizaki T, Matsumoto S. 2017. The tandem repeated organization of NB-LRR genes in the clubroot-resistant CRb locus in Brassica rapa L. Mol Genet Gen. 292:397–405.
- Hatakeyama K, Suwabe K, Tomita RN, Kato T, Nunome T, Fukuoka H, Matsumoto S, Wu K. 2013. Identification and characterization of Crr1a, a gene for resistance to clubroot disease (Plasmodiophora brassicae Woronin) in Brassica rapa L. PLoS One. 8:e54745.
- Hwang SF, Howard RJ, Strelkov SE, Gossen BD, Peng G. 2014. Management of clubroot (Plasmodiophora brassicae) on canola (Brassica napus) in western Canada. Can J Plant Pathol. 36(Supp.1):49–65.
- Irani S, Trost B, Waldner M, Nayidu NK, Tu J, Kusalik A, Todd CD, Wei Y, Bonhamsmith PC. 2018. Transcriptome analysis of response to Plasmodiophora brassicae infection in the Arabidopsis shoot and root. BMC Genomics. 19:23.
- Ji R, Zhao L, Xing M, Shen X, Bi Q, Peng S, Feng H. 2014. Infection of Plasmodiophora brassicae in Chinese cabbage. Genet Mol Res. 13:10976–10982.
- Jia H, Wei XC, Yang Y, Yuan YX, Wei F, Zhao YY, Yang SJ, Yao QJ, Wang ZY, Tian BM, et al. 2017. Root RNA-seq analysis reveals a distinct transcriptome landscape between clubroot-susceptible and clubroot-resistant Chinese cabbage lines after Plasmodiophora brassicae infection. Plant Soil. 421:93–105.
- Jones JD, Dangl JL. 2006. The plant immune system. Nature. 444:323–329.
- Jubault M, Lariagon C, Taconnat L, Renou J, Gravot A, Delourme R, Manzanaresdauleux MJ. 2013. Partial resistance to clubroot in Arabidopsis is based on changes in the host primary metabolism and targeted cell division and expansion capacity. Funct Integr Genomic. 13:191–205.
- Kageyama K, Asano T. 2009. Life cycle of Plasmodiophora brassicae. J Plant Growth Regul. 28:203–211.
- Kim KH, Kang YJ, Kim DH, Yoon MY, Moon JK, Kim MY, Van K, Lee SH. 2011. RNA-Seq analysis of a soybean near-isogenic line carrying bacterial leaf pustule-resistant and -susceptible alleles. DNA Res. 18:483–497.
- Latge J, Beauvais A. 2014. Functional duality of the cell wall. Curr Opin Microbiol. 20:111–117.
- Liu MJ, Sugimoto K, Uygun S, Panchy N, Campbell MS, Yandell M, Howe GA, Shiu SH. 2018a. Regulatory divergence in wound-responsive gene expression between domesticated and wild tomato. Plant Cell. 30:1445–1460.
- Liu Y, Xu A, Liang F, Yao X, Wang Y, Liu X, Zhang Y, Dalelhan J, Zhang B, Qin M, et al. 2018b. Screening of clubroot-resistant varieties and transfer of clubroot resistance genes to Brassica napus using distant hybridization. Breed Sci. 68:258–267.
- Livak KJ, Schmittgen TD. 2001. Analysis of relative gene expression data using real-time quantitative PCR and the 2−ΔΔCT method. Methods. 25:402–408.
- Louvet R, Cavel E, Gutierrez L, Guenin S, Roger D, Gillet F, Guerineau F, Pelloux J. 2006. Comprehensive expression profiling of the pectin methylesterase gene family during silique development in Arabidopsis thaliana. Planta. 224:782–791.
- Lu L, Xu S, Zeng L, Zheng X, Yu T. 2014. Rhodosporidium paludigenum induced resistance in Ponkan mandarin against Penicillium digitatum requires ethylene-dependent signaling pathway. Postharvest Biol Tech. 97:93–101.
- Marowa P, Ding A, Kong Y. 2016. Expansins: roles in plant growth and potential applications in crop improvement. Plant Cell Rep. 35:949–965.
- Pandey SP, Somssich IE. 2009. The role of WRKY transcription factors in plant immunity. Plant Physiol. 150:1648–1655.
- Parkin IAP, Koh C, Tang H, Robinson SJ, Kagale S, Clarke WE, Town CD, Nixon J, Krishnakumar V, Bidwell SL, et al. 2014. Transcriptome and methylome profiling reveals relics of genome dominance in the mesopolyploid Brassica oleracea. Genome Biol. 15:R77.
- Pelloux J, Rusterucci C, Mellerowicz EJ. 2007. New insights into pectin methylesterase structure and function. Trends Plant Sci. 12:267–277.
- Peng G, Lahlali R, Hwang SF, Pageau D, Hynes RK, Mcdonald MR, Gossen BD, Strelkov SE. 2014. Crop rotation, cultivar resistance, and fungicides/biofungicides for managing clubroot (Plasmodiophora brassicae) on canola. Can J Plant Pathol. 36:99–112.
- Piao Z, Ramchiary N, Yong PL. 2009. Genetics of clubroot resistance in Brassica species. J Plant Growth Regul. 28:252–264.
- Rayapuram C, Jensen MK, Maiser F, Shanir JV, Hornshoj H, Rung JH, Gregersen PL, Schweizer P, Collinge DB, Lyngkjaer MF. 2012. Regulation of basal resistance by a powdery mildew-induced cysteine-rich receptor-like protein kinase in barley. Mol Plant Pathol. 13:135–147.
- Regente M, Giudici AM, Villalain J, La Canal LD. 2005. The cytotoxic properties of a plant lipid transfer protein involve membrane permeabilization of target cells. Lett Appl Microbiol. 40:183–189.
- Sagi M, Fluhr R. 2001. Superoxide production by plant homologues of the gp91phox NADPH oxidase. Modulation of activity by calcium and by tobacco mosaic virus infection. Plant Physiol. 126:1281–1290.
- Scheible W, Fry BA, Kochevenko A, Schindelasch D, Zimmerli L, Somerville S, Loria R, Somerville C. 2003. An Arabidopsis mutant resistant to thaxtomin A, a cellulose synthesis inhibitor from Streptomyces species. Plant Cell. 15:1781–1794.
- Schuller A, Kehr J, Ludwig-Müller J. 2014. Laser microdissection coupled to transcriptional profiling of Arabidopsis roots inoculated by Plasmodiophora brassicae indicates a role for brassinosteroids in clubroot formation. Plant Cell Physiol. 55:392–411.
- Siemens J, Keller I, Sarx J, Kunz S, Schuller A, Nagel W, Schmulling T, Parniske M, Ludwig-Muller J. 2006. Transcriptome analysis of Arabidopsis clubroots indicate a key role for cytokinins in disease development. Mol Plant-Microbe Interact. 19:480–494.
- Song T, Chu M, Lahlali R, Yu F, Peng G. 2016. Shotgun label-free proteomic analysis of clubroot (Plasmodiophora brassicae) resistance conferred by the gene Rcr1 in Brassica rapa. Front Plant Sci. 7:1013.
- Stringlis IA, Yu K, Feussner K, de Jonge R, van Bentum S, Van Verk MC, Berendsen RL, Bakker P, Feussner I, Pieterse CMJ. 2018. MYB72-dependent coumarin exudation shapes root microbiome assembly to promote plant health. Proc Natl Acad Sci USA. 115:E5213–E5222.
- Ueno H, Matsumoto E, Aruga D, Kitagawa S, Matsumura H, Hayashida N. 2012. Molecular characterization of the CRa gene conferring clubroot resistance in Brassica rapa. Plant Mol Biol. 80:621–629.
- Wang SY, Qiang W, Hong W, Li Y, Jianbin L, Wanxia D. 2016. Identification of Plasmodiophora brassicae Wor. and resistance assessment of cabbage (Brasisica oleracea var. capitata L.) germplasm. J Plant Genet Resour. 17:1123–1132. in Chinese.
- You J, Chan Z. 2015. ROS regulation during abiotic stress responses in crop plants. Front Plant Sci. 6:1092.
- Zipfel C. 2009. Early molecular events in PAMP-triggered immunity. Curr Opin Plant Biol. 12:414–420.