Abstract
Various pathovars (pv.) of Xanthomonas campestris (Xc) are prevalent in South Korea, of which pv. campestris causing black rot threatens the production of cabbage in this major cabbage producing country of the world. Rapid and sensitive detection of the pathovar is an essential prerequisite for any plant protection programme. This study took an approach of re-aligning the whole genome sequences (upon availability) of representative strains of the bacteria and identified pathovar-specific genomic regions that are absent in other pathovars and other bacterial strains. Herein, eight Sequence Characterized Amplified Regions (SCAR) primer sets were designed, of which three sets, namely Xcc_48F/R, Xcc_53F/R and Xcc_79F/R, specifically amplified all Xcc strains and did not amplify other pathovars and bacterial strains. These primers also detected the Xcc strains collected from black rot infected field samples in South Korea. After optimizing the PCR conditions, one of the primers, Xcc_48F/R, was able to amplify as low as 6 × 10−3 ng μL−1 bacterial DNA. This unique method of marker development thus yielded sensitive, specific and reliable markers that can be used for efficient and inexpensive detection of Xcc for purposes including quarantine and disease forecasting by early detection from asymptomatic field samples. Additionally, the method can be replicated in developing markers from other phyto-pathogenic agents for which the variable genomic regions are not known.
Résumé
Différents pathovars (pv.) de Xanthomonas campestris (Xc) sévissent en Corée du Sud, dont pv. Campestris, causant la pourriture noire, menace la production de chou dans ce pays qui en est un des principaux producteurs dans le monde. Une détection rapide et sensible du pathovar est un préalable essentiel à tout programme de protection des végétaux. L’approche de cette étude est basée sur l’alignement des séquences du génome entier (en fonction de leur disponibilité) des souches représentatives des bactéries et des régions génomiques spécifiques des pathovars identifiées qui sont absentes chez les autres souches de pathovars et de bactéries. En cela, huit jeux d’amorces de régions amplifiées de séquences caractérisées ont été conçus, parmi lesquels les trois suivants, Xcc_48F/R, Xcc_53F/R et Xcc_79F/R, qui ont amplifié en particulier toutes les souches de Xcc et pas celles des autres pathovars ni des autres bactéries. Ces amorces ont également permis de détecter les souches de Xcc prélevées sur des échantillons collectés dans des champs infectés par la pourriture noire en Corée du Sud. Après avoir optimisé les conditions de la PCR, une des amorces, Xcc_48F/R, a pu amplifier aussi peu que 6 × 10−3 ng/μL d’ADN bactérien. Cette méthode unique a donc permis de concevoir des marqueurs sensibles, spécifiques et fiables qui peuvent être utilisés pour détecter, efficacement et économiquement, des souches de Xcc aux fins de quarantaine et de prévision de la maladie par un diagnostic précoce à partir d’échantillons asymptomatiques prélevés sur le terrain. De plus, la méthode peut être reproduite pour concevoir des marqueurs pour d’autres agents phytopathogènes pour lesquels les régions variables du génome sont inconnues.
Introduction
Xanthomonas, a large bacterial genus under the gamma subdivision of the Proteobacteria, consists of a range of pathogenic and non-pathogenic species (Vauterin et al., Citation1995; Vicente et al., Citation2017). These bacteria can cause a wide range of symptoms, including spots, rots, necrosis, blights and cankers on various plant parts of at least 268 dicotyledenous species and 124 monocotyledenous species (Boch & Bonas, Citation2010). In particular, X. campestris infects a wide range of cruciferous vegetables and wild relatives (Schaad et al., Citation2006). Strains of X. campestris from 140 pathovars were reclassified based on DNA–DNA hybridization studies into only six, which include X. campestris pv. aberrans, X. campestris pv. armoraciae, X. campestris pv. barbarae, X. campestris pv. campestris, X. campestris pv. incanae and X. campestris pv. raphani (Vauterin et al., Citation1995). These were later reclassified based on host specificity as X. campestris pv. campestris (Xcc), X. campestris pv. raphani (Xcr) and X. campestris pv. incanae (Xci), causing three distinct diseases in the genus Brassica (Fargier & Manceau, Citation2007).
Xanthomonas campestris pv. campestris (Xcc) causes the seed-borne disease black rot on Brassicaceae globally (Vicente & Holub, Citation2013), which can result in more than 50% yield reduction under favourable conditions (Williams, Citation1980). Pathogenic strains show substantial diversity across different countries and provinces of the same country (Jensen et al., Citation2010; Singh et al., Citation2014). Based on gene-for-gene models and interactions between Xcc strains and differential Brassica spp. cultivars, 11 pathogenic races of Xcc are known so far (Fargier & Manceau, Citation2007; Vicente & Holub, Citation2013; Cruz et al., Citation2017), of which races 1, 4, and 6 are the most virulent on cabbage (Taylor et al., Citation2002; Jensen et al., Citation2010; Singh et al., Citation2016).
Korea is the fifth largest cabbage producer in the world, producing 2.12 million metric tonnes annually, worth approximately US $66 million (Food and Agriculture Organization, Citation2015). Black rot on Korean cabbage cultivars was recognized since the 1970s (Kim, Citation1986). Accurate detection of pathovars in seed lots and at early symptomless stages in the field is essential to deploy preventive measures, as symptomatology-based detection is only possible after the disease has occurred. However, in many cases, detection of black rot may become confusing as symptoms are not always obvious and other biotic and abiotic factors may produce similar symptoms (Palacio-Bielsa et al., Citation2009). Various techniques have been used for Xcc detection, including isolation on semi-selective agar media and pathogenicity tests on seedlings (Alvarez et al., Citation1994; Koenraadt et al., Citation2005; Roberts & Koenraadt, Citation2005), and flow cytometry for Xcc (Chitarra et al., Citation2002). These techniques are often time-consuming, labour-intensive and may not be sensitive (Singh et al., Citation2014).
This study reports the development of PCR-based SCAR markers via whole genome re-alignment to rapidly and accurately distinguish X. campestris pv. campestris from other pathovars. These findings will be helpful for early detection of the pathogen, enabling the deployment of timely control measures before the appearance of symptoms and protect commercial cabbage cultivation along with seed lot inspection and transboundary quarantine programmes.
Materials and methods
Bacterial strains: collection and culture
Bacterial strains belonging to various species and pathovars of Xanthomonas and other bacteria used in this study are listed in . These strains were collected from various sources, such as the International Collection of Microorganisms from Plants (ICMP), New Zealand; and Korean Agricultural Culture Collection (KACC), Korea. Depending on the suitability, various growth media were used to culture and incubate (28 ± 2°C for 48 h) these strains ().
Table 1. List of plant pathogenic bacterial strains belonging to various species and pathovars of Xanthomonas and other genus used in this study along with their hosts and sources of the media with references used for culturing.
Extraction of bacterial genomic DNA
Bacterial strains were harvested from bacteria specific culture media using a scraper and genomic DNA was extracted using the DNeasy Plant Mini Kit (QIAGEN, Hilden, Germany) according to the manufacturer’s instructions. Extracted DNA was stored at −20°C for further use. The DNA concentration was measured by Nanodrop ND-1000 spectrophotometer (NanoDrop, Wilmington, DE, USA).
Retrieval and alignment of whole genomic sequences of bacteria
The whole genome sequences of 13 different strains of X. campestris pv. campestris such as B100 and CFBP1869 (race 1), ATCC 33 913 (race 3), CFBP 5817 (race 4), strain 8004 (race 9), ICMP4013, ICMP 21 080, strain 17, CN14, CN15, CN16, Xca5 and strain 32 and one strain for each of X. campestris pathovars incanae (CFBP1606R), raphani (756C) and one of X. euvesicatoria (Strain 85–10) were retrieved from the National Centre for Biotechnology Information (NCBI) database (www.ncbi.nlm.nih.gov) (Table S1). The whole genomic sequences of available strains were aligned using B100 (race 1) as reference by the genome alignment tool ‘progressive Mauve’ version v.2.4.0 with default parameters (). The variable DNA fragments between the X. campestris pathovar campestris and other pathovars were identified using bioinformatics tool ‘Geneious’ free trial version (https://www.geneious.com/free-trial/). The uniqueness of the X. campestris pv. campestris specific DNA fragments were further confirmed by ‘Xcc-BLAST’ tool (http:/210.110.86.160/lab/home.html).
Figure. 1 Multiple alignment of whole genome sequence (a) of 13 X. campestris pv. campestris (Xcc) strains and one strain of each of X. campestris pathovars incanae and raphani and another species (X. euvesicatoria) using Mauve tool, version 2.4.0. The alignment consists of several rearranged pieces larger than 1 Kb. Each genome is laid out horizontally with homologous segments (LCBs) outlined as coloured rectangular solid boxes. Lines collate aligned segments between genomes. Regions inverted relative to Xcc B100 (race 1) are set below those that match in the forward orientation. Average sequence similarities within an LCB, measured in sliding windows, are proportional to the heights of interior coloured bars. Large sections of white within blocks and gaps between blocks indicate lineage specific sequence; Comparative homology among the bacterial strains using Geneious software (Free trial version) facilitated the identification of variable regions from where SCAR primers Xcc_48F/R (b), Xcc_53F/R (c) and Xcc_79F/R (d) were designed. Rectangular boxes of similar colour in a–d indicate similar genomic segments.
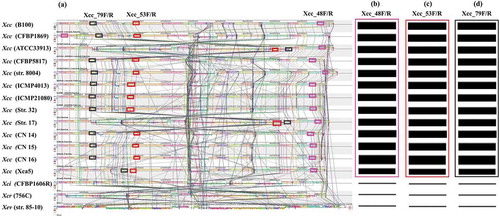
Designing and in silico specificity testing of primers
Eight sets of primers were designed from the X. campestris pv. campestris specific signature sequences using web based tool ‘OligoCalc: oligonucleotide properties calculator’ available from http://www.basic.northwestern.edu/biotools/oligocalc.html (Kibbe, Citation2007) with the view to detect the pathovar campestris from the all tested pathovars and other bacterial strains (). Specificity of the primers was checked by ‘Xcc-BLAST’ tool (http:/210.110.86.160/lab/home.html) and in silico PCR amplification tool (http://insilico.ehu.es/PCR/index.php?mo=Xanthomonas) prior to actual wet lab validation. The detailed genomic positions of the three Xcc unique primer pairs across all Xcc strains are shown in Table S2.
Table 2. List of previously reported primer sets tested for their effectiveness with their amplification status in detecting Xcc from our collection of bacterial strains via PCR assay.
Table 3. List of the primers used for Sequence-characterized amplified regions (SCAR) amplification of X. campestris pv. campestris by PCR assay.
Standardization of PCR conditions
PCR was performed with 20 µL of reaction mixture containing 9 µL of Emerald PCR master mix (Takara, Shiga, Japan), 1 µL of forward primer, 1 µL reverse primer, 8 µL of ultra-pure water and 1 µL of template DNA. To obtain specific amplification of target fragments (by minimizing the possibility of non-specific amplification), PCR conditions were optimized for each of the three Xcc specific primer pairs. Fine-tuned thermocycler (Takara, Shiga, Japan) conditions for each of the primer pairs were used which generally consisted of an initial strand denaturation at 95°C for 5 min followed by variable cycles of amplification and terminated by a final extension at 72°C for 5 min (). Electrophoresis was carried out on 1.2% agarose gels stained with blue mango (BioD, Gwangmyeong, Korea) in TAE buffer at 100 V for 30 min and the products were visualized on ENDUROTMGDS Gel Documentation system under UV light (302 nm). HIQ 100 bp plus DNA Ladder Mix (BIONEER, Republic of Korea) was used as size markers.
Isolation of Xcc strains from black rot infected leaf samples
Two hundred and sixty leaf samples of two widely cultivated cabbage cultivars (‘Daebakna’ and ‘Winstom’) showing characteristic symptoms of black rot disease (V-shaped lesions with blackened veins) were collected from 13 regions and 10 fields of each region (as replications) in South Korea from December 2017 to January 2018. The infected leaf samples were first dried by placing them between sheets of paper at room temperature (25–27°C) prior to cutting into small pieces and incubating on King’s B medium (20 g proteose peptone No. 3, 1.5g K2HPO4, 1.5g MgSO4.7H2O, 15 g agar powder per L ultra-pure water (King et al., Citation1954) at 30°C for 48 h). The colonies that grew from the pieces of dried leaf samples were examined and typical Xcc colonies (pale yellow, raised, mucoid) were sub-cultured several times to obtain pure Xcc colonies. The well separated and typical pure Xcc colonies were picked up by sterile toothpicks and suspended in 500 µL of liquid King’s B medium followed by incubating overnight at 28°C on a rotary shaker at 150 rpm. Finally, the samples were preserved in 50% glycerol suspension at −80°C for further use.
Pathogenicity testing by artificial inoculation of the purified Xcc strains
For pathogenicity tests, 5 µL of the stored Xcc isolates were sub-cultured 2–3 times on King’s medium and each time streaking was done by taking the bacterial inocula just from the previous culture. The sub-cultures were grown at 30°C for 48 h and purified bacterial colonies were harvested in 5 mL ultra-pure water by scraping from the single plate with a sterile microscope slide. The bacterial suspension was then concentrated by centrifugation and adjusted to 4 × 108 CFU mL−1. Seeds of the susceptible cabbage line BA33 were germinated in a Petri dish and transplanted to 6 cm diameter pots containing coco peat soil (Coconut Peat, Tamil Nadu, India) and allowed to grow for 5 weeks in a growth chamber at 24°C and 14/10 h (light/dark) photoperiod and 70–75% RH. The plants were then inoculated by clipping the secondary veins of the tip of two young leaves with sterile scissors followed by dipping in purified Xcc suspension (4 × 108 CFU mL−1). The growth chamber conditions were maintained at 24 ± 2°C with 16/8 h (light/dark) and 70–75% RH. For each of the 13 Xcc field isolates, three plants were inoculated. Control plants were inoculated with sterile water. The occurrence of the disease was recorded based on characteristic black rot symptoms on the wounded sites after 14 days.
Testing of primer efficacy in detecting field Xcc strains
The efficacy of the developed Xcc specific primers was further tested to detect the Xcc in samples collected and purified from diseased field samples. For PCR amplification, genomic DNA (1 µL) of each of the 24 purified Xcc colonies which were isolated from field collection of 13 cabbage growing regions were randomly taken.
Determination of detection limit of the Xcc genomic DNA concentration by PCR assay
To determine the suitable genomic DNA concentration of Xcc strains that can be detected in the PCR assay, a 10-fold dilution series (starting from 60 ng µL−1 to 6 × 10−4 ng µL−1) of the genomic DNA of Xcc strain HRI-W-3811 was used. One µL of each dilution was directly used as template DNA in PCR.
Results
Specificity of primers in detecting Xcc strains
Previously reported Xcc specific primers such as ZUP2311–ZUP2312 and ZUP2309–ZUP2310 (Rijlaarsdam et al., Citation2004); DLH120–DLH125 (Berg et al., Citation2005); DhrpF–DhrpR (Singh et al., Citation2014) and XCF/XCR ((Park et al., Citation2004) () were tested in our collection of bacterial strains. None of the primers were found to amplify the Xcc strains exclusively as besides detecting Xcc, other pathovars such as X. campestris pv. incanae, X. campestris pv. raphani and other species such as X. axonopodis pv. dieffenbachiae and X. axonopodis pv. glycines and other bacteria such as P. syringae pv. maculicola, E. carotovora subsp. carotovora and P. brassicae were also amplified by at least one of those previously published primers (; ). Hence, we attempted to design eight new primers towards Xcc specifically () from complete genome re-alignment data. Of the eight primer sets designed, three sets, namely Xcc_48F/R, Xcc_53 F/R and Xcc_79F/R, produced specific amplicons with 855 bp, 930 bp and 1573 bp sizes, respectively (), while the remaining five sets – (Xcc_18F/R, Xcc.3_48F/R, Xcc.1_59F/R, Xcc.2_59F/R and Xcc.3_59F/R) – did not show Xcc specific amplifications (Fig. S1). The three primer pairs which showed positive PCR amplification were specific to known genes in Xcc, such as putative prolyl aminopeptidase, putative exported protein and avirulence protein/Type III secretion system effector protein detected by the primer sets Xcc_53F/R, Xcc_48F/R and Xcc_79F/R, respectively (Fig. S2). Among these three genes, avirulence protein/Type III secretion system effectors protein is directly linked to pathogenicity. No amplicon was produced for the other pathovars of X. campestris such as X. campestris pv. incanae (HRI-W-6377), X. campestris pv. raphani (HRI-W-8305) and X. campestris pv. zinniae (KACC17126) and for other species of Xanthomonas such as X. euvesicatoria (KACC11153), X. axonopodis pv. dieffenbachiae (KACC17821) and X. axonopodis pv. glycines (KACC10491), bacteria (Pseudomonas syringae pv. maculicola (ICMP13051)), Erwinia carotovora subsp. carotovora (ICMP12464), and the protist (Plasmodiophora brassicae) were also not amplified by these three Xcc specific primer sets ().
Figure. 2 Evaluation of primer specificity of the previously published Xcc-specific markers; (a) Dhrp marker (Singh et al., Citation2014); (b) XCF/XCR marker (Park et al., Citation2004); (c–d) ZUP 2309/2310-ZUP2311/2312 marker (Rijlaarsdam et al., Citation2004) and (e) DLH120/125 marker (Berg et al., Citation2005); in detecting target bacterial strains. Xcc, X. campestris pv. campestris; Xci, X. campestris pv. incanae; Xcr, X. campestris pv. raphani; Xev, X. euvesicatoria; Xez, X. campestris pv. zinniae, Xad, X. axonopodis pv. dieffenbachiae; Xag. X. axonopodis pv. glycines; Psm, Pseudomonas syringae pv. maculicola; Ecc, Erwinia carotovora subsp. carotovora; Pb, Plasmodiophora brassicae.
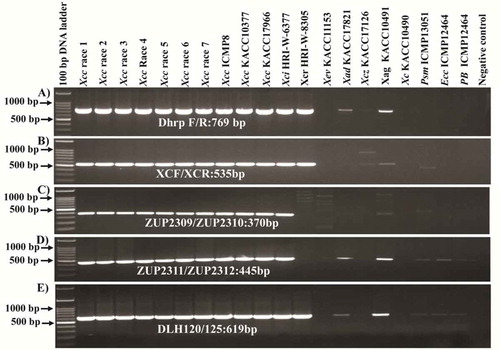
Figure. 3 Specificity of the X. campestris pv. campestris (Xcc) specific primer pairs; (a) Xcc_48F and Xcc_48R, (b) Xcc_53F and Xcc_53R and (c) Xcc_79F and Xcc_79R that produced amplicons for all Xcc strains but not for the other pathovars and other bacterial species. A 100 bp DNA ladder was used as size marker and DDW was used as negative control. Genomic DNA concentrations of all samples were adjusted to 60 ng µL−1. Xci, X. campestris pv. incanae; Xcr, X. campestris pv. raphani; Xev, X. euvesicatoria; Xev, X. campestris pv. zinniae; Xad, X. axonopodis pv. dieffenbachiae; Xag, X. axonopodis pv. glycines; Psm, Pseudomonas syringae pv. maculicola; Ecc, Erwinia carotovora subsp. carotovora; Pb, Plasmodiophora brassicae. Blue colour indicates, only Xcc strains were given positive amplification.
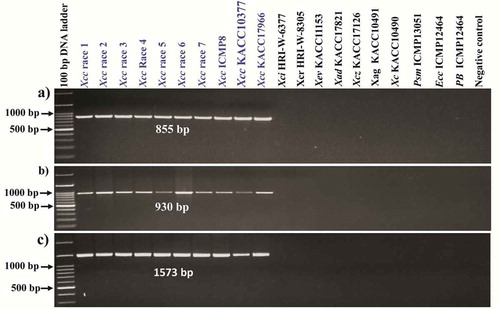
Validation of developed Xcc specific primers
The three Xcc specific primer pairs, Xcc_48, Xcc_53 and Xcc_79, were used to test their effectiveness in detecting the Xcc strains from randomly selected bacterial colonies isolated from 24 naturally infected field samples of Jeju island and Suncheon. The primers Xcc_48, Xcc_53 and Xcc_79 detected 10 and 9 colonies from Jeju island and Suncheon collection, respectively, as Xcc strain (Fig. S3). The reported Xcc specific Dhrp primer (Singh et al., Citation2014; see ) detected 17 and 16 colonies from Jeju island and Suncheon collection, respectively. Our primers specifically amplified only Xcc strains that were confirmed by PCR, using 10 known Xcc strains (see ), while Dhrp primer amplified Xcc, Xci and Xcr strains as well (see ). This result indicates that Dhrp primer does not specifically detect Xcc but also other bacterial strains, like Xcr and Xci. Hence, we speculated that the field isolates of Jeju island and Suncheon might also have other bacterial strains along with Xcc, giving rise to higher number of colonies amplified by Dhrp primer compared to ours.
For extensive validation of our primers, we collected a wide range of field samples from 13 black rot infected regions in Korea. The black rot susceptible cabbage line BA33 was inoculated with a bacterial suspension (4 × 108 CFU) collected from all 13 regions and maintained in greenhouse for observing whether the characteristic symptoms of black rot appeared. After 14 days, typical V-shaped symptoms were recorded (Fig. S4) indicating the presence of Xcc strains in the field isolates. In addition, presence of race 1 of X. campestris pv. campestris in the field isolates was confirmed by the previously published Xcc race 1 specific marker (Rubel et al., Citation2017) in Jeju island collection, but not in Suncheon collection, indicating differences at race level among the field isolates.
The well separated and typical pale yellow, raised and mucoid Xcc colonies from the cultured leaf samples of all 13 regions were also tested. The three Xcc specific primer pairs detected all the Xcc isolates as shown by the expected amplicons of 855 bp, 930 bp and 1573 bp specific to the primer pairs Xcc_48F and Xcc_48R, Xcc_53F and Xcc_53R and Xcc_79F and Xcc_79R (Fig. S3A-B), respectively.
Sensitivity of the Xcc genomic DNA concentration in colony PCR assay
A 10-fold dilution series of genomic DNA of Xcc strain HRI-W-3811 was used to determine the sensitivity of the colony PCR assay. Among the three Xcc specific primer pairs, Xcc_48F and Xcc_48R showed the best sensitivity as up to fifth dilution (0.006 ng µL−1) of genomic DNA was amplified by this primer (). The other two primer pairs, namely Xcc_53F and Xcc_53R and Xcc_79F and Xcc_79R, were able to detect up to fourth dilution (0.06 ng µL−1) of genomic DNA. However, the third dilution (0.6 ng µL−1) was found to be the best concentration of genomic DNA as all three primer pairs produced sharp and clear amplicons at this concentration.
Discussion
Black rot disease in cabbage, caused by the bacterial pathogen Xcc, is the second most important disease after clubroot (Basur, Citation2012). Integrative control measures are necessary to reduce disease development and early detection of the pathogen in seed lots or in seedlings before symptom appearance is very important in this regard. This study focused on the development of SCAR markers by identifying novel Xcc specific signature sequences via whole genome re-alignment based approach that can precisely detect Xcc via PCR based assay.
The increases in whole genome sequence information available for bacterial strains has opened up new avenues for comparative studies of genomic variability, allowing the development of polymerase chain reaction (PCR) based molecular markers. The PCR based detection methods are now widely used as they are rapid, accurate, inexpensive, less laborious and do not require isolation of pure bacterial cultures (Trindade et al., Citation2007; Adikini et al., Citation2011). Primers designed from various genomic regions, such as highly conserved repetitive elements, namely 35–40 bp REP (repetitive extragenic palindromic) sequence, 124–127 bp ERIC (enterobacterial repetitive intergenic consensus), 154 bp BOX element, hrp (hypersensitive response and pathogenicity) and gyrase gene were used as a fingerprinting method for different bacteria, including Xcc (Berg et al., Citation2005; Trindade et al., Citation2007; Rouhrazi & Khodakaramian, Citation2014). Various economically important Xanthomonads were detected using PCR based assays (Park et al., 2004; Vicente et al., Citation2006; Zaccardelli et al., Citation2007; Adikini et al., Citation2011; Raghavendra et al., Citation2013; Rubel et al., Citation2017).
There exists considerable species diversity in Xanthomonas (Gillings et al., Citation2005; Rademaker et al., Citation2005) which can be attributed to the highly plastic and rapidly evolving genomes of Xanthomonas spp. (Ryan et al., Citation2011). Comparative genomic analysis has revealed that genome diversity between various Xanthomonas spp. is due to the variation in certain conserved genes and gene clusters, and a large set of insertion sequence (IS) elements that are associated with virulence (Ryan et al., Citation2011). At a molecular level, the pathogenesis-associated gene clusters encoding type III effectors, type II and type IV secretion systems and some proteins of unknown function confer diversity in effector proteins which determines the specialization to various species, pathovars and races (Hajri et al., Citation2009; Vos, Citation2011; Schwartz et al., Citation2015). Owing to this diversity in effector proteins, the Xanthomonas spp. are further classified into various pathovars based on their specific adaptation to exploit the diversity of plant R genes to develop a specific pathogenicity reaction and cause a particular disease on a specific host plant and tissue (Tsygankova et al., Citation2004; Fargier & Manceau, Citation2007; Ryan et al., Citation2011; Cruz et al., Citation2017). The various pathovars in the Xanthomonas genus are remarkably uniform in all traits except for the ability to cause disease on particular hosts mediated by specific effector proteins (Van Den Mooter & Swings, Citation1990; Vauterin et al., Citation2000) and does not involve major modifications of pathogenicity related gene clusters, rather only subtle changes in a small number of genes manifest such pathovar specificity (Ryan et al., Citation2011). Such narrow differences between various pathovars of X. campestris may explain why the culture based pathogen detection, and conventional serological and enzymological assays, often yield inconclusive results. Thus, detection tools targeting differences in pathogenicity related genes between pathovars, which are the actual determinants of pathovar speciation, can precisely detect specific pathovars.
In this study, the whole genome re-alignment of diverse bacterial strains identified three Xcc specific regions harbouring one Type III secretion system effector protein and two putative genes, namely, putative prolyl aminopeptidase and putative export protein. Development of molecular markers from these genes helped to specifically detect Xcc. The detection of non-Xcc strains by the previous primer of Rijlaarsdam et al. (Citation2004) could be attributed to the fact that this primer was designed from a hypothetical protein gene (XCC2585) which may not be involved in pathogenesis, may have no variation between our tested diverse bacterial strains and thus, cannot distinguish Xcc specifically. But, the primers of Singh et al. (Citation2014) and Berg et al. (Citation2005) were designed from the pathogenesis related hrp (hypersensitive reaction and pathogenicity) gene which encodes for components of type III secretion systems (T3SS) and HR (hypersensitive reaction) elicitors (Pandey et al., Citation2018). Yet, this too detected strains belonging to pathovars other than Xcc. The most likely reason for the non-specificity of these hrp gene based primers could be that there is no Xcc specific variation for this gene in our tested bacterial strains. Thus, whole genome re-alignment based screening of variation in pathogenicity genes, especially type III secretion system components and effectors involved in pathogenesis could not only be helpful to explain host adaptation within X. campestris pathovars, as described for other plant pathogenic bacteria (Grant et al., Citation2006; Sarkar et al., Citation2006; Guy et al., Citation2013), but also to detect those specific pathovars via PCR based assays (Rubel et al., Citation2017).
Use of healthy pathogen free seeds is recommended for reducing black rot disease. In addition, early detection of the pathogen before appearance of symptoms is also crucial for timely deploying of effective chemical control measures. The reliable detection of Xcc in seed lots and asymptomatic plants is thus very important and PCR based assays are recommended by International Seed Testing Association (ISTA) in this regard (Leu et al., Citation2010; Roberts & Koenraadt, Citation2014). Our primers are highly reproducible, sensitive and robust and hence, will be useful for any plant protection and quarantine programmes that require the accurate detection of the Xcc pathovar.
Author Contributions
I-SN, J-IP and H-TK conceived and designed the study. SN conducted the in silico analysis. MHR prepared and cultured the samples, isolated DNA and performed wet lab validation. MHR and MRH wrote the manuscript and prepared the tables and figures. UKN extensively revised the manuscript and edited it. KSA cultured the field infected samples and helped in PCR validation. JHL & HJJ prepared the bacterial inocula and managed the field infected samples. All authors read and approved the final draft of the manuscript.
Supplementary Figures 1-4
Download MS Power Point (5.1 MB)Supplementary Tables 1-2
Download MS Word (85.5 KB)Acknowledgements
The authors would like to acknowledge Joana G. Vicente, University of Warwick, UK for providing known races of Xcc, Xci and Xcr and the Korean Agriculture Culture Collection (KACC), Korea and the International Collection of Microorganisms from Plants (ICMP), New Zealand for providing respective bacterial strains.
Supplemental material
Supplemental data for this article can be accessed here.
Additional information
Funding
References
- Adikini S, Tripathi L, Beed F, Tusiime G, Magembe E, Kim D. 2011. Development of a specific molecular tool for detecting Xanthomonas campestris pv. musacearum. Plant Pathol. 60:443–452.
- Alvarez A, Benedict A, Mizumoto C, Hunter J, Gabriel D. 1994. Serological, pathological, and genetic diversity among strains of Xanthomonas campestris infecting crucifers. Phytopathology. 84:1449–1457.
- Assis SM, Mariano RL, Michereff SJ, Silva G, Maranhão EA. 1999. Antagonism of yeasts to Xanthomonas campestris pv. campestris on cabbage phylloplane in field. Rev Microbiol. 30:191–195.
- Basur VN 2012. Investigation on Cabbage Black Rot Caused by Xanthomonas campestris pv. campestris (Pammel) [ MS Thesis]. Department of Plant Pathology, University of Agricultural Sciences, Dharwad (India). pp. 1–85.
- Berg T, Tesoriero L, Hailstones D. 2005. PCR‐based detection of Xanthomonas campestris pathovars in Brassica seed. Plant Pathol. 54:416–427.
- Boch J, Bonas U. 2010. Xanthomonas AvrBs3 family-type III effectors: discovery and function. Annu Rev Phytopathol. 48:419–436.
- Chitarra L, Langerak C, Bergervoet J, Van Den Bulk R. 2002. Detection of the plant pathogenic bacterium Xanthomonas campestris pv. campestris in seed extracts of Brassica sp. applying fluorescent antibodies and flow cytometry. Cytom Part A. 47:118–126.
- Cruz J, Tenreiro R, Cruz L. 2017. Assesment of diversity of Xanthomonas campestris pathovars affecting cruciferous plants in Portugal and disclosure of two novel X. campestris pv. campestris races. J Plant Pathol. 99:403–414.
- Fargier E, Manceau C. 2007. Pathogenicity assays restrict the species Xanthomonas campestris into three pathovars and reveal nine races within X. campestris pv. campestris. Plant Pathol. 56:805–818.
- Food and Agriculture Organization. 2015. FAO Statistical Pocketbook World food and agriculture. Rome: Food and Agriculture Organization of the United Nations. accessed 2018 Feb 24. http://www.fao.org/3/a-i4691e.pdf.
- Gillings MR, Holley MP, Stokes HW, Holmes AJ. 2005. Integrons in Xanthomonas: a source of species genome diversity. PNAS (Proceedings of the National Academy of Sciences), USA. 102:4419–4424.
- Grant SR, Fisher EJ, Chang JH, Mole BM, Dangl JL. 2006. Subterfuge and manipulation: type III effector proteins of phytopathogenic bacteria. Ann Rev Microbiol. 60:425–449.
- Guy E, Genissel A, Hajri A, Chabannes M, David P, Carrere S, Lautier M, Roux B, Boureau T, Arlat M, et al. 2013. Natural genetic variation of Xanthomonas campestris pv. campestris pathogenicity on Arabidopsis revealed by association and reverse genetics. mBio. 4:e00538–12.
- Hajri A, Brin C, Hunault G, Lardeux F, Lemaire C, Manceau C, Boureau T, Poussier S. 2009. A «repertoire for repertoire» hypothesis: repertoires of type three effectors are candidate determinants of host specificity in Xanthomonas. PLoS One. 4:e6632.
- Jensen BD, Vicente JG, Manandhar HK, Roberts SJ. 2010. Occurrence and diversity of Xanthomonas campestris pv. campestris in vegetable Brassica fields in Nepal. Plant Dis. 94:298–305.
- Kibbe WA. 2007. OligoCalc: an online oligonucleotide properties calculator. Nucleic Acids Res. 35(suppl_2):W43–W46.
- Kim BS. 1986. Testing for detection of Xanthomonas campestris pv. campestris in crucifer seeds and seed disinfection. Kor J Plant Pathol. 2:96–101.
- King EO, Ward MK, Raney DE. 1954. Two simple media for the demonstration of pyocyanin and fluorescin. Transl Res. 44:301–307.
- Koenraadt H, Van Bilsen J, Roberts S. 2005. Comparative test of four semi-selective agar media for the detection of Xanthomonas campestris pv. campestris in Brassica seeds. Seed Sci Technol. 33:115–125.
- Laila R, Robin AHK, Yang K, Choi GJ, Park J-I, Nou I-S. 2017. Detection of ribosomal DNA sequence polymorphisms in the protist Plasmodiophora brassicae for the identification of geographical isolates. Int J Mol Sci. 18:84–100.
- Leu YS, Deng WL, Yang WS, Wu YF, Cheng AS, Hsu ST, Tzeng KC. 2010. Multiplex Polymerase Chain Reaction for Simultaneous Detection of Xanthomonas campestris pv. campestris and X. campestris pv. raphani. Plant Pathol Bullet. 19:137–147.
- Massomo SM, Nielsen H, Mabagala RB, Mansfeld-Giese K, Hockenhull J, Mortensen CN. 2003. Identification and characterisation of Xanthomonas campestris pv. campestris strains from Tanzania by pathogenicity tests, biolog, rep-PCR and fatty acid methyl ester analysis. Eur J Plant Pathol. 109:775–789.
- Palacio-Bielsa A, Cambra M, López M. 2009. PCR detection and identification of plant-pathogenic bacteria: updated review of protocols (1989-2007). J Plant Pathol. 91:249–297.
- Pandey SS, Patnana PK, Padhi Y, Chatterjee S. 2018. Low‐iron conditions induces the hypersensitive reaction and pathogenicity hrp genes expression in Xanthomonas and is involved in modulation of hypersensitive response and virulence. Env Microbiol Rep. 10:522–531.
- Park YJ, Lee BM, Ho-Hahn J, Lee GB, Park DS. 2004. Sensitive and specific detection of Xanthomonas campestris pv. campestris by PCR using species-specific primers based on hrpF gene sequences. Microbiol Res. 159:419–423.
- Rademaker JLW, Louws FJ, Schultz MH, Rossbach U, Vauterin L, Swings J, De Bruijn FJ. 2005. A comprehensive species to strain taxonomic framework for Xanthomonas. Phytopathology. 95:1098–1111.
- Raghavendra B, Singh D, Yadava D, Mondal KK, Sharma P. 2013. Virulence analysis and genetic diversity of Xanthomonas campestris pv. campestris causing black rot of crucifers. Arch Phytopathol Plant Protect. 46:227–242.
- Rijlaarsdam A, Woudt B, Simons G, Koenraadt H, Oosterhof J, Asma M, Buddiger P, Roorda P, Grimault V, De Koning J 2004. Development of specific primers for the molecular detection of Xanthomonas campestris pv. campestris. In EPPO Conference on Quality of Diagnosis and New Diagnostic Methods for Plant Pests. Noordwijkerhout, NL. pp. 4–19.
- Roberts S, Koenraadt H. 2005. Detection of Xanthomonas campestris pv. campestris (Black rot) in Brassica spp. International Rules for Seed Testing: Annex to Chapter 7 Seed Health Methods. Bassersdorf: International Seed Testing Association (ISTA). p. 15.
- Roberts SJ, Koenraadt H. 2014. 7-019a: detection of Xanthomonas campestris pv. campestris on Brassica spp. International rules for seed testing, annexe to chapter seed health testing methods. Bassersdorf: International Seed Testing Association (ISTA).
- Rouhrazi K, Khodakaramian G. 2014. Genetic fingerprinting of Iranian Xanthomonas campestris pv. campestris strains inducing black rot disease of crucifers. Eur J Plant Pathol. 139:175–184.
- Rubel MH, Robin AHK, Natarajan S, Vicente JG, Kim H-T, Park J-I, Nou I-S. 2017. Whole-genome re-alignment facilitates development of specific molecular markers for races 1 and 4 of Xanthomonas campestris pv. campestris, the cause of black bot disease in Brassica oleracea. Int J Mol Sci. 18:2523–2538.
- Ryan RP, Vorhölter FJ, Potnis N, Jones JB, Van Sluys MA, Bogdanove AJ, Dow JM. 2011. Pathogenomics of Xanthomonas: understanding bacterium–plant interactions. Nat Rev Microbiol. 9:344–355.
- Sarkar SF, Gordon JS, Martin GB, Guttman DS. 2006. Comparative genomics of host-specific virulence in Pseudomonas syringae. Genetics. 174:1041–1056.
- Schaad NW, Postnikova E, Lacy G, Sechler A, Agarkova IV, Stromberg PE, Stromberg VK, Vidaver AM. 2006. Emended classification of Xanthomonad pathogens on citrus. Papers Plant Pathology. 29:690–695.
- Schwartz AR, Potnis N, Timilsina S, Wilson M, Patané J, Jr J M, Minsavage GV, Dahlbeck D, Akhunova A, Almeida N, et al. 2015. Phylogenomics of Xanthomonas field strains infecting pepper and tomato reveals diversity in effector repertoires and identifies determinants of host specificity. Front Microbiol. 6:535–551.
- Singh D, Raghavendra B, Rathaur PS, Singh H, Raghuwanshi R, Singh R. 2014. Detection of black rot disease causing pathogen Xanthomonas campestris pv. campestris by bio-PCR from seeds and plant parts of cole crops. Seed Sci Technol. 42:36–46.
- Singh D, Rathaur P, Vicente J. 2016. Characterization, genetic diversity and distribution of Xanthomonas campestris pv. campestris races causing black rot disease in cruciferous crops of India. Plant Pathol. 65:1411–1418.
- Taylor J, Conway J, Roberts S, Astley D, Vicente JG. 2002. Sources and origin of resistance to Xanthomonas campestris pv. campestris in Brassica genomes. Phytopathology. 92:105–111.
- Trindade L, Marques E, Lopes DB, Ferreira M. 2007. Development of a molecular method for detection and identification of Xanthomonas campestris pv. viticola. Summa Phytopathol. 33:16–23.
- Tsygankova S, Ignatov A, Boulygina E, Kuznetsov B, Korotkov E. 2004. Genetic relationships among strains of Xanthomonas campestris pv. campestris revealed by novel rep-PCR primers. Eur J Plant Pathol. 110:845–853.
- Van den Mooter M, Swings J. 1990. Numerical analysis of 295 phenotypic features of 266 Xanthomonas strains and related strains and an improved taxonomy of the genus. Int J Syst Evol Micr. 40:348–369.
- Vauterin L, Hoste B, Kersters K, Swings J. 1995. Reclassification of Xanthomonas. Int J Syst Evol Microbiol. 45:472–489.
- Vauterin L, Rademaker J, Swings J. 2000. Synopsis on the taxonomy of the genus Xanthomonas. Phytopathology. 90:677–682.
- Vicente JG, Conway J, Roberts S, Taylor J. 2001. Identification and origin of Xanthomonas campestris pv. campestris races and related pathovars. Phytopathology. 91:492–499.
- Vicente JG, Everett B, Roberts S. 2006. Identification of isolates that cause a leaf spot disease of Brassicas as Xanthomonas campestris pv. raphani and pathogenic and genetic comparison with related pathovars. Phytopathology. 96:735–745.
- Vicente JG, Holub EB. 2013. Xanthomonas campestris pv. campestris (cause of black rot of crucifers) in the genomic era is still a worldwide threat to Brassica crops. Mol Plant Pathol. 14:2–18.
- Vicente JG, Rothwell S, Holub EB, Studholme DJ. 2017. Pathogenic, phenotypic and molecular characterisation of Xanthomonas nasturtii sp. nov. and Xanthomonas floridensis sp. nov., new species of Xanthomonas associated with watercress production in Florida. Int J Syst Evol Micr. 67:3645–3654.
- Vos M. 2011. A species concept for bacteria based on adaptive divergence. Trends Microbiol. 19:1–7.
- Williams PH. 1980. Black rot: A continuing threat to world crucifers. Plant Dis. 64:736–742.
- Wulff EG, Mguni CM, Mortensen CN, Keswani CL, Hockenhull J. 2002. Biological control of black rot (Xanthomonas campestris pv. campestris) of Brassicas with an antagonistic strain of Bacillus subtilis in Zimbabwe. Eur J Plant Pathol. 108:317–325.
- Zaccardelli M, Campanile F, Spasiano A, Merighi M. 2007. Detection and identification of the crucifer pathogen, Xanthomonas campestris pv. campestris, by PCR amplification of the conserved Hrp/type III secretion system gene hrcC. Eur J Plant Pathol. 118:299–306.