Abstract
In addition to causing disease in wheat and other grain crops, Fusarium graminearum is associated with a wide range of forage, weedy and non-cultivated gramineous species.. The nature of these associations is often unclear, and interactions between F. graminearum and these hosts are not well characterized, despite their prevalence in and around cereal fields. We observed differences in the host suitability (competency) of spring wheat and six common grasses during three stages of the pathogen life cycle. Pathogen survival in artificially infested stem tissue was comparable between grasses and wheat over two years with the exception of one host. Sexual and asexual spore production on host stems under laboratory conditions varied significantly but exceeded 1 × 105 spores per dry gram of tissue for all grass species. A seedling root infection assay revealed the apparent resistance of two grasses to root rot and indicated that host preference could vary between pathogen isolates. These findings are relevant for how the role of wild grass hosts in disease epidemics of proximate crops and in pathogen evolution is viewed. Managing weedy grasses in field margins and avoiding gramineous pasture–wheat rotations may reduce local sources of F. graminearum inoculum. Changes in the relative pathogenicity of isolates depending on host species suggest that wild grasses could exert selective pressure on F. graminearum and requires further investigation.
Résumé
En plus de causer des maladies chez le blé et les autres céréales, Fusarium graminearum est associé à une vaste gamme de plantes fourragères ainsi qu’à des graminées adventices et non cultivées. La nature de ces associations est souvent floue et les interactions entre F.graminearum et ces hôtes ne sont pas bien caractérisées, malgré leur prévalence dans et autour des champs de céréales. Nous avons observé des différences quant à la pertinence de l’hôte (compétence) du blé de printemps et de six graminées courantes durant trois stades du cycle vital de l’agent pathogène. Sauf pour un hôte, la survie de l’agent pathogène dans les tissus de tiges infectés artificiellement était similaire chez les graminées et le blé pendant deux années. La production sexuée et asexuée de spores sur les tiges des hôtes en laboratoire avarié significativement, mais excédait 1 × 105 spores par gramme sec de tissu pour toutes les espèces de graminées. Un test d’infection racinaire mené sur des plantules arévélé la résistance apparente de deux graminées au pourridié et amontré que la préférence de l’hôte pouvait varier entre les isolats de l’agent pathogène. Ces conclusions sont pertinentes en ce qui atrait à la perception du rôle des graminées naturelles en tant qu’hôtes dans les épidémies qui se propagent dans les cultures situées à proximité et quant à l’évolution de l’agent pathogène. La gestion des graminées adventices sur le pourtour des champs et l’abstention d’intégrer un pâturage de graminées dans le plan de rotation du blé peuvent contribuer à réduire les sources locales d’inoculum de F.graminearum. Des changements dans la pathogénicité relative des isolats selon l’espèce de l’hôte suggèrent que les graminées naturelles pourraient exercer une pression de sélection chez F.graminearum, ce qui nécessite des études plus approfondies.
Introduction
Fusarium graminearum is a globally distributed pathogen of cereal crops responsible for lost revenue estimated at one billion dollars annually in the USA (Wilson et al. Citation2017). Known for causing head blight and crown rot of wheat and ear rot of corn, F. graminearum has been isolated from dozens of other gramineous hosts (Farr and Rossman Citation2018). Many of these are wild or weedy species covering large areas of land and can be found readily in pastures, grasslands and the margins of agricultural fields. Even in regions with intensive cereal production, the acreage of wild grasses and grassy pasture can be greater than that of susceptible field crops. For example, in 2017, the state of Kansas contained approximately 2.7 million ha of corn and 2.2 million ha of winter wheat but also 8.9 million ha of grass and mixed pasture (USDA Citation2018). Wild grass spikes and residues containing F. graminearum have been consistently reported from diverse locations (Inch and Gilbert Citation2003; Pereyra and Dill-Macky Citation2008; Landschoot et al. Citation2011; Turkington et al. Citation2011; Postic et al. Citation2012; Mourelos et al. Citation2014; Lofgren et al. Citation2018). Despite the distribution, extensive interface with crops and near universal ability of wild grass species to harbour F. graminearum, their interaction with the pathogen remains poorly understood. The host competency of these grasses, or how well they support the life cycle and successful spread of F. graminearum, has implications for disease epidemics and the evolution of the pathogen.
Fig. 1 Survival of Fusarium graminearum in grass stems over two winters. Bars show per cent pathogen recovery from stems after one and two years of overwintering in artificially infested debris deposited outside in autumn 2016. Whiskers represent standard deviation of three replicates, each containing 10 stem segments. Lolium perenne was significantly less likely to be colonized than other hosts (P ≤ 0.001), and by year two all the grasses had decreased to less than 40% infestation rates (P < 0.001).
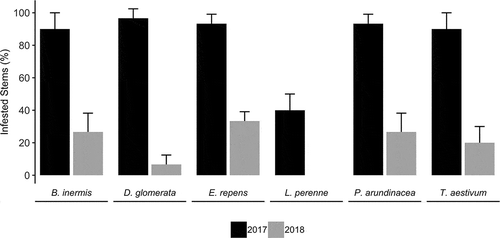
Fig. 2 Fusarium graminearum spore production on six different grasses. Bars are confidence intervals (95%) around least square means from a linear mixed model of (a) ascospore and (b) conidia production on grass stems averaged across 10 isolates of F. graminearum. The number of spores produced was significantly affected by host in both cases (F5,8 = 14.30, P < 0.001 and F5,8 = 43.45, P < 0.001, respectively).
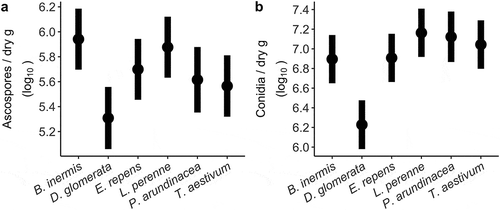
Fig. 3 Incidence of root rot caused by five isolates of Fusarium on the seedlings of six grass species. Host species had a significant effect on disease incidence (F5,419 = 91.04, P ≤ 0.001), and there was a significant interaction between host and isolate (F20,419 = 3.01, P ≤ 0.001). Sample sizes are included below each bar.
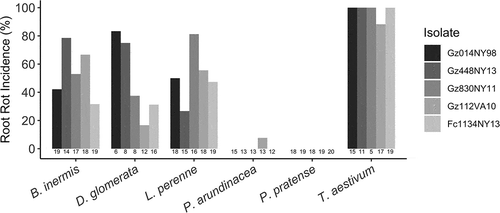
The role these wild grass hosts play in crop disease cycles and whether they are capable of contributing substantial amounts of inoculum to disease epidemics is unknown. Perithecia and sporodochia can be observed on senesced grass stems in the spring (Purss Citation1969), indicating that the pathogen may overwinter in wild grass residue. How much inoculum is produced on these grasses and their importance in the context of multiple inoculum sources originating from field crop residues and airborne propagules is not clear (Keller et al. Citation2014). The relative suitability of these grasses for pathogen overwintering and spore production also is unknown.
In addition to epidemiological considerations, wild grass hosts could be influencing pathogen evolution. Fusarium graminearum sensu lato is a phylogenetic species complex whose members produce an array of toxic secondary metabolites (Marasas et al. Citation1984; O’Donnell et al. Citation2000), and passing F. graminearum through different agricultural hosts is reported to alter pathogenic fitness (Akinsanmi et al. Citation2007). The presence of diverse gramineous hosts could be important to the maintenance of broad range pathogenicity or the emergence of new pathogen variants.
Previously observed interactions of F. graminearum with wild grass spikes indicate host-variable lifestyles and adaptations. Grass spikes are colonized asymptomatically under natural conditions (Inch and Gilbert Citation2003; Starkey et al. Citation2007; Mourelos et al. Citation2014; Lofgren et al. Citation2018), in contrast to the typical scab symptoms and ‘tombstone’ kernels that develop during the infection of small grains (McMullen et al. Citation1997). However, exceptions are known (Nyvall et al. Citation1999), and artificial inoculation of some grasses results in recognizable symptoms (MacInnes and Fogelman Citation1923; Lofgren et al. Citation2018). Further, little mycotoxin contamination is detected in grass hosts colonized by toxigenic Fusarium spp., indicating no clear role for these crop virulence factors and providing evidence of variable fungus–host interactions (Di Menna et al. Citation1987; Engels and Krämer Citation1996; Kononenko et al. Citation2015; Lofgren et al. Citation2018).
In order to better understand this variability and the potential role of wild grasses in crop disease epidemics, we compared the host competency of wheat and several grass species common to cereal production sites in New York state, USA. Host–pathogen interactions were characterized at three points in the pathogen life cycle: winter survival, reproduction and seedling infection.
Materials and methods
Grass and inoculum preparation
Senesced stems used in the winter survival and spore production assays were taken from plants grown in a greenhouse for two months. The spring wheat variety ‘Norm’ and five non-cereal grasses were included in these experiments. Four were grown starting with commercially available seed (Great Basin Seed, Ephraim, UT) (Bromus inermis Leyss. (smooth brome), Phalaris arundinacea L. (reed canarygrass), Dactylis glomerata L. (orchardgrass), Lolium perenne L. (perennial ryegrass)), and one was collected from mature, natural stands because seed was unavailable (Elymus repens (L.) Gould (quackgrass)). Stems were stripped of leaves and air dried for one week, trimmed into 5 cm long pieces containing one internode each, and autoclaved at 121°C for 20 min on two consecutive days before use. The seedling root rot assay included the above grasses with the exception of E. repens and the addition of Phleum pratense L. (timothy). The inocula for all three experiments were obtained by washing conidia from 2-week-old pathogen colonies grown on potato dextrose agar (PDA) under 12 h black light (F40/350BL, Sylvania, Wilmington, MA). All spore suspensions were adjusted to 1 × 104 conidia mL−1. Ten isolates of the pathogen previously collected from several hosts, locations and years were used in the winter survival and spore production assays to incorporate between isolate variability in estimates of host competency. A different set of isolates, including four of F. graminearum and one of F. cerealis, were used in the seedling root rot assay so several pathogen chemotypes would be represented and the reaction of grasses to a different Fusarium species would be included ().
Table 1. Fusarium isolates used in this study.
Overwintering
The ability of F. graminearum to overwinter on the senesced stems of wheat and five grass species was examined by artificially infesting sterile plant tissue and observing year-to-year survival rates. A random complete block trial with six replicates per grass was conducted under field conditions in Tompkins County, New York from September 2016 to March 2018. Stems were dip inoculated in an equal-parts mixture of all 10 isolates to simulate multiple infections that may occur in nature, wrapped in cheesecloth and incubated for two weeks in darkness at room temperature. Each replicate packet contained 10 tissue segments. Packets were staked to the soil surface and after overwintering under natural conditions, three bundles of each grass were collected in March 2017 and the remainder in March 2018. Recovered stems were surface sterilized in 1.65% NaOCl for 60 s, rinsed in sterile distilled water for 30 s, and left on PDA amended with streptomycin (5 g L−1) and neomycin (5 g L−1) for four days under 12 h white light. The resulting fungal colonies were identified morphologically, and the number of stems infested with F. graminearum was recorded (Leslie and Summerell Citation2007).
Reproduction
Spore production per dry gram of host tissue was quantified in a laboratory experiment adapted from Pereyra and Dill-Macky (Citation2008). Six grasses and each of the 10 isolates plus a sterile water control treatment were arranged in a random complete block design with three replicates per ‘isolate × grass’ combination. The moisture content of host tissue was determined with an OHAUS MB25 moisture meter (Parsippany, NJ) so spore counts could later be converted to a per dry gram basis. An equal wet mass of each grass (0.25–0.30 g) was placed into 60 mm glass Petri dishes containing 10 mL of sterile sand, which was included to maintain moist conditions. Dishes were inoculated with 5 mL of spore suspension from single isolates or sterile water and kept at room temperature under 12 h black light for two weeks, when the contents of each plate were moved to 15 mL tubes and mixed with 10 mL of sterile water and one drop of 0.01% (v/v) Tween 20. Samples were mixed well and left to release spores for 12 h. After being vortexed for 2 min, 1 mL subsamples were aliquoted from these tubes and both conidia and ascospores were counted on a hemocytometer. The experiment was performed twice.
Root rot assay
Susceptibility to Fusarium root rot was assayed at the seedling growth stage. Seeds of each host were surface sterilized in 1.65% NaOCl for 60 s, rinsed in sterile water for 30 s, and soaked in the inoculum from one of five isolates for 15 min. Sterile water served as a control treatment and 10 seeds, split into two replicates, were germinated for each of the ‘host × isolate’ combinations. Inoculated seeds were incubated in moist paper towels at room temperature under 12 h white light in partially closed plastic bags for two weeks. The number of seeds germinated and number of seedlings exhibiting symptoms of Fusarium root rot, browning of root tissue or water soaking at the root-shoot juncture, were recorded. The roots of five healthy and five symptomatic seedlings from each grass were then randomly selected, surface sterilized as described above and incubated on PDA to test for F. graminearum colonization. The experiment was performed twice.
Data analysis
All analyses were performed in Rstudio (Version 1.1.453; RStudio Team Citation2015) using linear models. Experimental repeats and blocks were included in all models as fixed effects. The recovery of overwintered F. graminearum from debris was analysed with a binomial model using grass and year of recovery as fixed effects. Model estimates (log-odds ratios) were compared with Tukey adjusted pairwise contrasts to compare grasses (Lenth Citation2017). Spore counts were converted to a dry gram basis and log10 transformed to fit the assumption of a normal distribution before analysis. These data were modelled with a fixed effect for grass and a random effect for isolate (Bates et al. Citation2015). Least square means and 95% confidence intervals were then produced to compare hosts. The effect of Fusarium inoculum on germination in the seedling assay was modelled on a binomial distribution using fixed effects for grass species and a factor with two levels, inoculum and water control. Since pathogen inoculum did not reduce germination rate and mock inoculated seeds did not display disease symptoms, data points for non-germinated plants and water controls were removed before further analysis. The presence or absence of disease symptoms on seedlings was used in a binomial model and subjected to a two-way analysis of variance using grass species and isolate as interacting terms.
Results and discussion
Overwintering
Artificially infested grass debris only retained F. graminearum at a high rate (25–100%) in the first year after colonization (). A significant effect on overwintering was detected for grass species (χ5 = 57.05, P < 0.001), and L. perenne was less likely to be infested than all the other grasses (P ≤ 0.001). After a second year, pathogen retention dropped significantly to between 0–40% (χ1 = 190.51, P < 0.001), coinciding with the physical deterioration of host tissues. The rate of pathogen recovery over two years confirms previous findings on wheat (Khonga and Sutton Citation1988; Pereyra et al. Citation2004) but adds to our understanding of how well wild host species might enable F. graminearum to persist in field margins or fallow fields between cropping cycles.
Reproduction
Inoculum production was compared on gramineous species under controlled conditions in two experiments. The number of both ascospores and conidia produced on grass stem tissues were significantly affected by host species (F5,8 = 14.30, P < 0.001 and F5,8 = 43.45, P < 0.001, respectively). Dactylis glomerata was the least suitable grass for spore production, though all grasses supported over 1 × 105 ascospores or conidia per dry gram of tissue (). The variation in spore production recorded across hosts may be related to nutrient composition or physiological structure. The ratio of C:N has been suggested as a relevant factor (Khonga and Sutton Citation1988; Leplat et al. Citation2013; Manstretta and Rossi Citation2016), as have silica-containing cells (Guenther and Trail Citation2005; Imboden et al. Citation2017).
Root rot assay
Inoculation did not have a significant effect on germination rate for any grass (F1,717 = 2.20, P = 0.138), and seeds soaked in sterile water exhibited no symptoms of disease. Wheat was the most susceptible host while P. pratense (no observed infections) and P. arundinacea (a single observed infection) were the least susceptible (). Grass species was a significant predictor of disease incidence (F5,419 = 91.04, P ≤ 0.001), and the ‘grass × isolate’ interaction was significant (F20,419 = 3.01, P < 0.001). No F. graminearum was recovered from asymptomatic or water control treated plants, and symptomatic plants contained no fungi other than F. graminearum. Fusarium graminearum has been recovered from the root or crown tissues of many grasses under natural conditions (Purss Citation1969; Postic et al. Citation2012; Scauflaire et al. Citation2013; Kiecana et al. Citation2014). Because root and crown rot infections may lead to the colonization of grass residues and eventual inoculum production, grasses not readily colonized in this manner may be less competent as sites for pathogen survival and reproduction. The variable interactions seen between grass species and isolate indicates that fitness may be optimized for one host over another.
Acknowledgements
We thank the anonymous reviewers who helped improve this work through their thoughtful comments and suggestions.
Additional information
Funding
References
- Akinsanmi OA, Chakraborty S, Backhouse D, Simpfendorfer S. 2007. Passage through alternative hosts changes the fitness of Fusarium graminearum and Fusarium pseudograminearum. Environ Microbiol. 9(2):512–520.
- Bates D, Maechler M, Bolker B, Walker S. 2015. Fitting linear mixed-effects models using lme4. J Statist Software. 67(1):1–48.
- Di Menna ME, Lauren DR, Poole PR, Mortimer PH, Hill RA, Agnew MP. 1987. Zearalenone in New Zealand pasture herbage and the mycotoxin-producing potential of Fusarium species from pasture. N Z J Agr Res. 30(4):499–504.
- Engels R, Krämer J. 1996. Incidence of Fusaria and occurrence of selected Fusarium mycotoxins on Lolium spp. in Germany. Mycotoxin Res. 12(1):31–40.
- Farr DF, Rossman AY. 2018. Fungal databases, U.S. National Fungus Collections, ARS, USDA. [accessed 2018 December]. https://nt.ars-grin.gov/fungaldatabases.
- Guenther JC, Trail F. 2005. The development and differentiation of Gibberella zeae (anamorph: Fusarium graminearum) during colonization of wheat. Mycologia. 97(1):229–237.
- Imboden L, Afton D, Trail F. 2017. Surface interactions of Fusarium graminearum on barley. Mol Plant Pathol. 19(6):1332–1342.
- Inch S, Gilbert J. 2003. The incidence of Fusarium species recovered from inflorescences of wild grasses in southern Manitoba. Can J Plant Pathol. 25(4):379–383.
- Keller MD, Bergstrom GC, Shields EJ. 2014. The aerobiology of Fusarium graminearum. Aerobiologia. 30(2):123–136.
- Khonga EB, Sutton JC. 1988. Inoculum production and survival of Gibberella zeae in maize and wheat residues. Can J Plant Pathol. 10(3):232–239.
- Kiecana I, Cegielko M, Mielniczuk E, Pastucha A. 2014. Fungi infecting ornamental grasses and the pathogenicity of Fusarium culmorum (W.G.Sm.) and Fusarium equiseti (Corda) Sacc. to selected species. Acta Sci Pol-Hort. 13(5):61–75.
- Kononenko GP, Burkin AA, Gavrilova OP, Gagkaeva TY. 2015. Fungal species and multiple mycotoxin contamination of cultivated grasses and legumes crops. Agr Food Sci. 24(4):323–330.
- Landschoot S, Audenaert K, Waegeman W, Pycke B, Bekaert B, De Baets B, Haesaert G. 2011. Connection between primary Fusarium inoculum on gramineous weeds, crop residues and soil samples and the final population on wheat ears in Flanders, Belgium. Crop Prot. 30(10):1297–1305.
- Lenth R 2017. Emmeans: estimated marginal means, aka least-squares means. R package version 0.9.1. https://CRAN.R-project.org/package=emmeans.
- Leplat J, Friberg H, Abid M, Steinberg C. 2013. Survival of Fusarium graminearum, the causal agent of Fusarium head blight. Agron Sustain Dev. 33(1):97–111.
- Leslie JF, Summerell BA. 2007. The Fusarium laboratory manual. Hoboken (NJ): Wiley-Blackwell.
- Liang, JM, Xayamongkhon, X, Broz, K, Dong, YH, McCormick, SP, Abramova, S, Ward, TJ, Kistler, HC. 2014. Temporal dynamics and population genetic structure of Fusarium graminearum in the upper Midwestern United States. Fungal Genet. Biol. 73:83–92.
- Lofgren LA, LeBlanc NR, Certano AK, Nachtigall J, LaBine KM, Riddle J, Broz K, Dong Y, Bethan B, Kafer CW, et al. 2018. Fusarium graminearum: pathogen or endophyte of North American grasses? New Phytol. 217(3):1203–1212.
- MacInnes J, Fogelman R. 1923. Wheat scab in Minnesota. St. Paul (MN): the University of Minnesota agricultural experiment station. Technical Bulletin. 18:3–43.
- Manstretta V, Rossi V. 2016. Effects of temperature and moisture on development of Fusarium graminearum perithecia in maize stalk residues. Appl Environ Microbiol. 82(1):184–191.
- Marasas WFO, Nelson PE, Tousser TA. 1984. Toxigenic Fusarium species: identification and mycotoxicology. University Park (PA): The Pennsylvania State University Press.
- McMullen M, Jones R, Gallenberg D. 1997. Scab of wheat and barley: A re-emerging disease of devastating impact. Plant Dis. 81(12):1340–1348.
- Mourelos CA, Malbran I, Balatti PA, Ghiringhelli PD, Lori GA. 2014. Gramineous and non-gramineous weed species as alternative hosts of Fusarium graminearum, causal agent of Fusarium head blight of wheat, in Argentina. Crop Prot. 65:100–104.
- Nyvall RF, Percich JA, Mirocha CJ. 1999. Fusarium head blight of cultivated and natural wild rice (Zizania palustris) in Minnesota caused by Fusarium graminearum and associated Fusarium spp. Plant Dis. 83(2):159–164.
- O’Donnell K, Kistler HC, Tacke BK, Casper HH. 2000. Gene genealogies reveal global phylogeographic structure and reproductive isolation among lineages of Fusarium graminearum, the fungus causing wheat scab. Proc Natl Acad Sci USA. 97(14):7905–7910.
- Pereyra SA, Dill-Macky R. 2008. Colonization of the residues of diverse plant species by Gibberella zeae and their contribution to Fusarium head blight inoculum. Plant Dis. 92(5):800–807.
- Pereyra SA, Dill-Macky R, Sims AL. 2004. Survival and inoculum production of Gibberella zeae in wheat residue. Plant Dis. 88(7):724–730.
- Postic J, Cosic J, Vrandecic K, Jurkovic D, Saleh AA, Leslie JF. 2012. Diversity of Fusarium species isolated from weeds and plant debris in Croatia. J Phytopathol. 160(2):76–81.
- Purss GS. 1969. The relationship between strains of Fusarium graminearum schwabe causing crown rot of various gramineous hosts and stalk rot of maize in Queensland. Austr J Agricul Res. 20(2):257–264.
- RStudio Team. 2015. RStudio. Boston (MA): Integrated Development for RStudio, Inc. http://www.rstudio.com/.
- Scauflaire J, Gourgue M, Foucart G, Renard F, Vandeputte F, Munaut F. 2013. Fusarium miscanthi and other Fusarium species as causal agents of Miscanthus x giganteus rhizome rot. Eur J Plant Pathol. 137(1):1–3.
- Starkey DE, Ward TJ, Aoki T, Gale LR, Kistler HC, Geiser DM, Suga H, Toth B, Varga J, O‘Donnell K. 2007. Global molecular surveillance reveals novel Fusarium head blight species and trichothecene toxin diversity. Fungal Genet Biol. 44(11):1191–1204.
- Turkington TK, Clear RM, Demeke T, Lange R, Xi K, Kumar K. 2011. Isolation of Fusarium graminearum from cereal, grass and corn residues from Alberta, 2001-2003. Can J Plant Pathol. 33(2):179–186.
- United States Department of Agriculture. 2018. National agricultural statistics service cropland data layer. Published crop-specific data layer. [accessed 2018 December]. https://nassgeodata.gmu.edu/CropScape/.
- Ward TJ, Clear RM, Rooney AP, O‘Donnell K, Gaba D, Patrick S, Starkey DE, Gilbert J, Geiser DM, Nowicki TW. 2008. An adaptive evolutionary shift in Fusarium head blight pathogen populations is driving the rapid spread of more toxigenic Fusarium graminearum in North America. Fungal Genet Biol. 45(4):473–484.
- Wilson WW, McKee G, Nganje W, Dahl B, Bangsund D. 2017. Economic impact of USWBSI’s Scab Initiative to reduce FHB. Fargo (ND): Department of Agricultural Economics, North Dakota State University. Agribusiness Appl Econ. No 774.