Abstract
Leaf mottle and wilt (LM) of sunflower caused by Verticillium dahliae is the most important disease in Argentina. LM is a monocyclic disease where microsclerotia in the soil are the primary inoculum source and have an important impact on symptom expression (incidence and severity) and yield. The inoculum (microsclerotia/g of soil) and disease relationship is affected by several factors, such as temperature and soil moisture. The objective of this work was to determine the regression model that best fits the relationship between V. dahliae microsclerotia in soil and sunflower LM, over a wide environmental range. Sunflower plants were exposed to different microsclerotia densities in pasteurized and non-pasteurized soils in pots under greenhouse and field conditions. The microsclerotia in soil and LM relationship regression models were also tested in the field with natural soil borne microsclerotia infestation, in different sunflower cultivars and locations. After the flowering stage (± 8 days), disease severity was affected by inoculum density at every evaluation time (P-value≤0.0009). A 45% increase in the area under the disease progress curve was shown by soil pasteurization. Power regression model showed the highest concordance and Pearson correlation coefficients, parameter significance and no difference with the lowest Akaike index criterion value among all tested models in all experiments. This study provides a model (LM = 11.6*inoculum density^0.57) which describes the inoculum density and LM relationship. The use of this model might help farmers to predict an LM risk before sowing and to choose the appropriate field and the resistance level of the sunflower hybrid to be used.
Résumé
La verticilliose (V) du tournesol, causée par Verticillium dahliae, est la plus importante maladie en Argentine. La V est une maladie monocyclique pour laquelle les microsclérotes du sol constituent la source primaire d’inoculum et ont une influence majeure sur l’expression des symptômes (incidence et gravité) ainsi que sur le rendement. L’inoculum (microsclérotes/g de sol) et le lien avec la maladie sont influencés par divers facteurs tels que la température et l’humidité du sol. Le but de ces travaux était de déterminer le modèle de régression qui représente le mieux le lien entre les microsclérotes de V. dahliae dans le sol et la verticilliose du tournesol, et ce, pour une gamme étendue de conditions environnementales. En serre et au champ, des plants de tournesol en pots ont été exposés à différentes densités de microsclérotes dans des sols pasteurisés et non pasteurisés. Les modèles de régression décrivant le lien entre les microsclérotes du sol et la V ont également été testés au champ, avec différents cultivars et à divers sites, dans des sols naturellement infestés par des microsclérotes. Après la floraison (±8 jours), nous avons noté que la gravité de la maladie avait été influencée par la densité de l’inoculum, et ce, lors de chaque évaluation (P-value ≤ 0,0009). La pasteurisation du sol a affiché un accroissement de 45% de l’aire sous la courbe de progression de la maladie. Le modèle de régression a démontré la plus forte concordance et les coefficients de corrélation de Pearson, l’importance des paramètres et aucune différence avec le plus faible indice de la valeur du critère d’Akaike, et ce, parmi tous les modèles testés au cours de toutes les expériences. Cette étude fournit un modèle (V = 11,6 * densité de l’inoculum ∧ 0,57) qui décrit le lien entre la densité de l’inoculum et la verticilliose. Ce modèle pourrait aider les producteurs à prédire le risque de V avant de semer et à choisir le champ approprié ainsi que le degré de résistance de l’hybride de tournesol à utiliser.
Introduction
Leaf mottle and wilt (LM) on sunflowers caused by the fungus Verticillium dahliae Kleb. is one of the most important diseases in Argentina (Pereyra and Escande Citation1999) and is endemic in half of the cultivated area in this region. Microsclerotia (MS) allow survival of the fungus in the soil and plant debris for up to 13 years (Wilhelm and George Citation1955) and constitute the primary inoculum. Because LM is a monocyclic disease, microsclerotia play an important role in the expression of symptoms (incidence and severity) and subsequent yield losses (López-Escudero et al. Citation2003). In Argentina, production losses due to LM can reach up to 73% on susceptible genotypes in highly infested fields (Pereyra et al. Citation1999); hence disease management is based on the use of disease-resistant sunflower cultivars. As complementary preventive measures, no-tillage and crop rotations with non-host species such as barley and wheat (Mol and Van Riesen Citation1995) help to avoid an incremental increase in the amount of microsclerotia in soils with low levels of inoculum (Quiroz et al. Citation2008), since V. dahliae is a natural soil invader that increases in population by mono cropping or rotation with susceptible crops. The pathogen has a wide host range that includes 350 plant species in more than 160 families, and affects important crops like alfalfa (Medicago sativa L.), cauliflower (Brassica oleracea var. botrytis L.), cotton (Gossypium hirsutum L.), horseradish (Armoracia rusticana G. M. Sch.), olive (Olea europaea L.), pepper (Capsicum annuum L.), potato (Solanum tuberosum L.), sunflower (Helianthus annuus L.) and tomato (Solanum lycopersicum L.) (Pegg Citation1974).
The disease cycle of LM starts when sunflower root exudates stimulates germination of microsclerotia in soil and the emerging hyphae penetrate at the root hair zone (Eynck et al. Citation2007) and invade the xylem. Around sunflower flowering R5 stage (Schneiter and Miller Citation1981), interveinal leaf necrosis surrounded with a yellow chlorosis are seen starting on the lowest leaves and progressing upwards (Markell et al. Citation2015). Close to physiological maturity (R9 stage) (Schneiter and Miller Citation1981), mycelia surround and/or disorganize the pith producing microsclerotia along the stem pith. Therefore, most of this new inoculum is produced in sunflower aerial stems, and is then incorporated into the soil by tillage, thus increasing the primary inoculum.
Many biotic and abiotic environmental processes are involved in disease expression affecting the relationship between microsclerotia and disease symptoms, such as the presence of antagonistic or competitor microorganisms in the rhizosphere (Berg et al. Citation2005), tillage or no-tillage (changes in physical and chemical soil composition) and sunflower cultivar resistance level (Quiroz et al. Citation2008). Previous work on V. dahliae has proposed different regression models to describe the inoculum density (microsclerotia/g of soil) and its relationship with the disease in many susceptible crops, including cauliflower, cotton, horseradish, olive and potato. These relationships were described by negative exponential, linear, natural logarithm, quadratic, power and sigmoid regression models (Pullman and DeVay Citation1982; Paplomatas et al. Citation1992; Nagtzaam et al. Citation1997; Xiao et al. Citation1998; Khan et al. Citation2000; López-Escudero and Blanco-López Citation2007). These studies were conducted in commercial fields, greenhouse or microplots. Understanding the relationship between inoculum density and LM may assist growers in assessing the risk of the disease, before sowing the crop in a particular field. The purpose of this work was to determine the regression function that best fits the relationship between V. dahliae microsclerotia in soil and sunflower LM, in a wide range of environments.
Materials and methods
Production of microsclerotia
To obtain microsclerotia, V. dahliae isolate America recovered from a sunflower commercial field in 2003 (35°28ʹ35.2”S 62°59ʹ35.9”W, America town, Buenos Aires province, Argentina) was used. Ten discs (10 mm diameter) of colonized potato dextrose agar (2% PDA, Sigma-Aldrich, St. Louis, Missouri, USA) were used to inoculate autoclaved (121ºC, 14.7 psi, 15 min) wetted rice (100 g rice; 45 mL distilled water), contained in 473 cm3 glass bottles. Bottles were sealed with a cotton plug wrapped in paper and incubated lying down in the dark for 20 days at 25 ± 2°C. Microsclerotia were harvested by adding 133 cm3 of sterilized distilled water to each bottle. After shaking for 45 s, suspension was filtered (0.2 mm gauze) and poured in 400 g of pre-washed (in 1M HCl) river sand (grain > 300 µm). Thin layers of infested sand were dried on lab plates for three days, and then sieved through 25, 45, 75, 150 and 300 µm diameter meshes using sieve shaker equipment (W.S. Tyler, Rotap RX-29–10, Ohio, USA). To relate the microsclerotia number with weight, 10 mg of microsclerotia was suspended in 1 mL of distilled water and homogenized with a tube shaker (Precytec, Model A-11M/180, Buenos Aires, Argentina) for 25 s. The suspension was diluted with distilled water (50 µL/1000 µL), a 50 µL aliquot was placed in a RODAC (Replicate Organism Detection and Counting) plate of 55 mm diameter, and microsclerotia were counted with 40X using a stereoscopic microscope (SMZ-10, Nikon, Konan, Minato-ku, Tokio, Japan). The number of microsclerotia was corrected given the viable microsclerotia (growing hyphae) in the RODAC plate after incubation for 48 hr at 25 ± 2ºC in the dark.
Experiments with V. dahliae added inoculum
Experiment 1 (buried pots under field conditions)
The treatments were eight inoculum densities by adding 0, 0.1, 1, 3, 5, 15, 25 or 100 viable microsclerotia/g of pasteurized soil (steam-air, 60/71ºC, 30 min, twice). The soil was extracted from the A horizon of a typical argiudol [pH = 6.4; Soil Organic Matter (SOM) = 4.6%]. For each treatment, microsclerotia and soil were mixed with a concrete mixer (Deper, motor three-fourths Hp 220v, Buenos Aires, Argentina). A randomized complete block design, with three blocks and an experimental unit of seven pots, was used. Pots with 9 kg of soil each were buried in a field at Balcarce, Buenos Aires, Argentina (37°45ʹ28.2”S 58°17ʹ53.7”W), 5 cm above the ground. Five seeds of the susceptible sunflower cultivar ‘Cauquén’ (El Cencerro®, Coronel Suárez, Buenos Aires, Argentina) were sown (October 18, 2006) in each pot. One-week-old plants were thinned to one seedling per pot. Close to the sunflower reproductive stage R5.5 (flowering) (± 8 days) (Schneiter and Miller Citation1981), disease incidence (number of plants with LM/number of total plants) and severity (number of leaves with LM/number of total leaves per plant) were assessed on a weekly basis. Disease data underwent variance analysis (Infostat® Ver 2.0, Faculty of Agricultural Sciences, Córdoba National University, Córdoba, Argentina) to test the effects of inoculum density on these variables (LM incidence and severity). Regression analyzes were also conducted; incidence and severity mean data were related to inoculum density using linear, natural logarithm, negative exponential, power, quadratic and sigmoidal regression models (Sigma Plot®, Systat Software, Inc., San Jose, CA, USA). We also performed a regression analysis for the area under disease (incidence and severity) progress curve (AUDPC) calculated from all disease assessment times (Madden et al. Citation2007). The evaluated regression models were the most used models for V. dahliae inoculum and disease relationship in many susceptible crops (Pullman and DeVay Citation1982; Paplomatas et al. Citation1992; Nagtzaam et al. Citation1997; Xiao et al. Citation1998; Khan et al. Citation2000; López-Escudero and Blanco-López Citation2007). To test which regression model best suited the data, linear regression analysis of observed and predicted values for each model was performed. Concordance (ρc) (Lin Citation1989) and Pearson´s (ρ) (Pearson Citation1895) correlation coefficients were used to compare models deviation from a concordance line (1:1 line with a slope of 1 and an intercept of 0) (Infostat® Professional v.1.1, Córdoba, Argentina). For further regression model evaluation, Akaike Index Criterion (AIC) (Akaike Citation1974) was used.
Experiment 2 (buried pots under greenhouse conditions)
The same materials and methods described for Experiment 1 were used, with the following modifications: Five inoculum density treatments with 0, 1, 4, 15 and 100 microsclerotia/g of pasteurized soil (pH = 6.5, SOM% = 4.1) and non-pasteurized soil (pH = 6.4, SOM% = 6.5) under greenhouse conditions (26.7 ± 16ºC average) were included. The experimental unit consisted of three pots (= three plants) with a randomized complete block design, with three blocks. Sowing date (SD) was on September 30, 2009. Disease was assessed on a weekly basis, starting from the R5.5 sunflower reproductive stage until R7 stage (early grain filling).
Field experiments with a natural soilborne inoculum of V. dahliae
Data from six sunflower field trials from the Argentinian National Trial Network of Commercial Sunflower Hybrids (INTA-ASAGIR) were used for this experiment in 2005–2006 season (www.asagir.org.ar/acerca-de-evaluacion-de-cultivares-463). Field names and locations were: Balcarce (37°45ʹ17”S 58°18ʹ22”W) conventional tillage (BCT; SD: October 23, 2005) and without tillage (BWT; SD: October 19, 2005), Coronel Suárez (CS, 37°29ʹ33”S 61°52ʹ17”W; SD: October 31, 2005) without tillage, El Carretero (EC, 38°29ʹ34”S 59°48ʹ52”W; SD: November 01, 2005), Pieres (PI, 38°23ʹ56”S 58°39ʹ48”W; SD: November 01, 2005) and San Francisco de Bellocq (BQ, 38°41ʹ22”S 59°58ʹ57”W; SD: October31, 2005) with conventional tillage. Fields had between one (EC, BCT and BWT) or two (BQ, CS and PI,) sunflower crops in a four season rotation with wheat and maize. All fields had a previous history of LM disease, except for Balcarce. A randomized complete block design with three replicates was used. Three LM susceptible cultivars were used: DK3920 (Monsanto Company®, St. Louis, Missouri, USA), ‘Paihuén’ (El Cencerro®, Coronel Suárez, Buenos Aires, Argentina) and PAN7009 (Pannar ®, Venado Tuerto, Santa Fe, Argentina). The experimental unit was a plot of three 0.7 m width and 6 m long rows, with approximately 60 plants. To relate soil inoculum with leaf mottle symptoms, 10 soil sub-samples were extracted from the central row at 20 cm depth using a phosphorus sampler (MC 20 model, Meridiens Instrumental, Mar del Plata, Argentina). Sub-samples of approximately 1.5 kg g of soil were collected for every cultivar and its replications. All samples were air dried at 24 ± 2ºC under laboratory conditions over two months, leaving only viable resistance structures. Colony forming units (CFU) of V. dahliae in soil samples (1 CFU = 1 microsclerotia) were counted by the plate dispersion method (Gould and Termorshuizen Citation2003) using Soil Pectate Tergitol Agar media (Polygalacturonic acid P-3889; Sigma-Aldrich, St. Louis, Missouri, USA) as substrate (Mpofu & Hall Citation2003). Disease severity was estimated at the R7 phenological stage (early grain filling), and it was related to estimated inoculum densities through regression analysis, as indicated above. Disease severity and inoculum density data were analyzed by a two-way (location and hybrid) analysis of variance (ANOVA procedure, INFOSTAT Professional v.1.1, Córdoba, Argentina). Significance of the factors and their interactions was evaluated based on the F statistics and P-values (considered significant if ≤ 0.05). Means separation of treatments was performed using Fischer’s LSD test (α = 0.05).
Results
Experiments with V. dahliae added inoculum
Experiment 1 (buried pots under field conditions)
Incidence of leaf mottle increased between R4 and R7 phenological stages (8 to 17 days after flowering, DAF; ). Incidence reached 20% ± 10 and 100% ± 0 during this period, for 0 (control without microsclerotia added) and 100 microsclerotia/g of soil treatments, respectively. At all evaluation times, disease incidence showed an effect of inoculum density (P-value = 0.02). Control without microsclerotia added (0 microsclerotia/g of soil) had the smallest area under disease progress curve (AUDPC) for incidence, while inoculum densities of 5, 25 and 100 showed the highest values (P-value = 0.003; LSD Test, α = 0.05; inset). The rest of microsclerotia-added treatments (0.1, 1 and 3 microsclerotia/g of soil) showed intermediate AUDPC values for incidence (LSD Test, α = 0.05, inset).
Fig. 1 Effect of soil microsclerotia (MS) density of Verticillium dahliae on leaf mottle (LM) progress in two experiments in buried pots (Exp. 1 in the field: A and B; Exp. 2 in greenhouse: C, D, E and F) and two soil pasteurization treatments (pasteurized: (a– d); non-pasteurized: (e and f)). Microsclerotia densities: (●) 0, (○) 0.1, (▼) 1, (▽) 3, (▲) 4, (■) 5, (∆) 15, (□) 25 and (♦) 100 MS/g of soil. Leaf mottle variables: Incidence (number of plants with LM/number of total plants in percentage), severity (number of leaves with LM/number of total leaf assessed in diseased plants, in percentage) and the area under disease progress curve (AUDPC) in figure insets for incidence and severity. Bars indicate one area under diseases progress curve standard error.
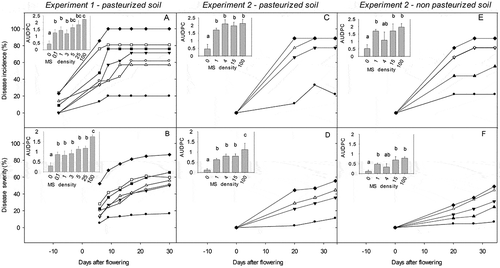
Leaf mottle severity increased from flowering to the end of grain filling (0 to 35 days after flowering; ). At all evaluation times, inoculum density had a significant (P-value<0.001) effect on disease severity. Control without microsclerotia added (0 microsclerotia/g of soil) showed the smallest AUDPC for disease severity, while the 100 microsclerotia/g of soil treatment showed the largest area (P-valueMSadded= 0.0043; LSD Fischer, α = 0.05). The remaining inoculum densities treatments (0.1, 1, 3, 5 and 25 microsclerotia/g of soil) showed intermediate AUDPC values for disease severity (LSD Test, α = 0.05) ( inset).
Relationships between inoculum density and LM were established. As LM incidence showed a similar pattern as found with severity, we used LM severity and AUDPC for disease severity to evaluate the regression models. The power regression model showed the highest ρ, ρc and no significant differences with lower AIC values (; ).
Table 1. Regression models evaluation. The second-order Akaike Index Criterion (AICc) and linear regression analysis of observed vs predicted values between microsclerotia density of Verticillium dahliae in soil and sunflower leaf mottle (severity on grain filling stage and area under severity progress curve, AUDPC).
Fig. 2 Power regression model for relationship between soil microsclerotia (MS) densities of Verticillium dahliae and leaf mottle severity on R7 stage (early grain filling) or area under disease progress curve (severity) in two experiments in buried pots (Exp. 1 in the field: (a) and (b); Exp. 2 in greenhouse: (c) and (d)). Leaf mottle disease (LM) variables: Severity and the area under the disease progress curve (AUDPC) for severity. Bars indicate one standard error (n = 3 in Exp 1 and n = 2 in Exp 2). Dashed lines show LM in non-pasteurized soil.
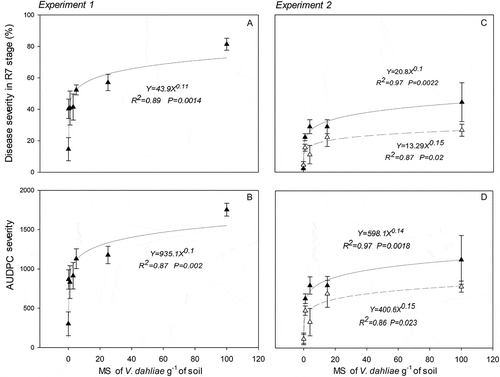
Experiment 2 (buried pots under greenhouse conditions)
Incidence of LM increased between R5 and R7 phenological stages (0 to 27 days after flowering; c and e) for both soil pasteurization treatments (pt). Incidence at R7 stage reached 22 ± 11% and 89 ± 11%, during this period, for control with 0 and 100 microsclerotia/g of soil treatments, respectively. After R5 (0 days after flowering) the effect of inoculum on disease incidence was observed (P-value≤0.02) at all evaluation times. Soil pasteurization did not affect disease incidence at any evaluation time (AUDPC for disease incidence, P-valuept= 0.17 and P-valueMSadded x pt =0.4). Nevertheless, control without microsclerotia added (0 microsclerotia/g of soil) had the lowest AUDPC for disease incidence, while the other inoculum density treatments showed the same high AUDPC level (P-value = 0.0007; LSD Fischer, α = 0.05; and inset).
LM severity increased from flowering to end of grain filling (0 to 34 days after flowering; and ). During this period, severity increased to 7 ± 4% for control without microsclerotia added (0 microsclerotia/g of soil), and 56 ± 14% for 100 microsclerotia/g of soil treatment. After flowering, disease severity showed an effect of inoculum density (P-value≤0.0009) at all assessment times. The 0 microsclerotia/g of soil treatment showed the smallest AUDPC for disease severity, while 15 and 100 microsclerotia/g of soil treatments showed the highest AUDPC values (P-value = 0.0002; LSD Fischer, α = 0.05). The others inoculum density treatments (1 and 4 microsclerotia/g of soil) showed AUDPC for disease severity values between 0 and 100 microsclerotia/g of soil (LSD Test, α = 0.05). Plants grown in pasteurized soil showed 45% more AUDPC for disease severity than non-pasteurized soil, and this effect was not significantly affected by inoculum density (P-valuept= 0.02 and P-valueMSadded x pt =0.47).
Among all regression models tested, power regression showed the highest ρ, ρc and no significant differences with lower AIC values (; ) independently of the pasteurization treatment.
Field experiments with natural soil borne inoculum of V. dahliae
Inoculum density of V. dahliae varied between 2 and 311 CFU/g of soil (= microsclerotia/g of soil) (P-valuelocation= 0.0004) among locations, whereas disease severity ranged from 7% to 73% (P-valuelocation= 0.017, P-valuehybrid= 0.001). No effects of hybrid were detected (P-valuehybrid= 0.58). Locations BCT, BNT and EC presented the lower inoculum density values with 20 ± 9, 15 ± 11 and 64 ± 11 microsclerotia/g of soil, respectively (LSD Test, α = 0.05). CS showed the highest inoculum density number with 235 ± 48 microsclerotia/g of soil, whereas PI y BQ recorded intermediate values with 125 ± 21 and 124 ± 8 microsclerotia/g of soil, respectively (LSD Test, α = 0.05). Disease severity showed a similar variation among locations. BCT, BNT and EC also showed the lowest values with 20 ± 6, 18 ± 7 and 43 ± 3 LM severity, respectively (LSD Test, α = 0.05). PI showed the highest LM severity, 56 ± 5%, BQ 54 ± 7% and CS 52 ± 3% (LSD Test, α = 0.05). Leaf mottle increased with inoculum density increments (0 to 300 microsclerotia/g of soil) (). When LM severity ranged between 40% and 60%, inoculum density fluctuated from 70 to 260 microsclerotia/g of soil. Power regression model showed a high ρ (0.76), ρc (0.73) and no significant differences with lower AIC values (). This model accounted for 57% of the observed variation in the field (). Exponential model showed similar correlation coefficients (ρ = 0.78, ρc = 0.77) but only in this experiment ().
Fig. 3 Power regression model for relationship between microsclerotia (MS) density of Verticillium dahliae in soil and leaf mottle severity in R7 stage (early grain filling). Symbol´s shape identifies field experiment location: triangle up, Balcarce Conventional Tillage; triangle upside down, Balcarce No Tillage; diamond, S. F. Bellocq; hexagonal, Coronel Suárez; circle, El Carretero and square, Pieres. Symbol´s color identifies hybrids: black, Paihuén; grey, DK3920 and white, PAN7009. The location of each symbol is the mean of three replications.
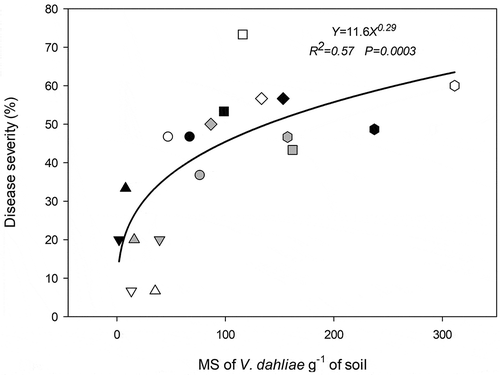
Discussion
Results from this study showed that LM incidence depends on microsclerotia density in soil and the time or phenological stages (). This demonstrates that time is a factor to be considered in order to understand the relationship between inoculum density of V. dahliae in soil and LM. The effect of inoculum density and time was mentioned by Xiao et al. (Citation1998) in field experiments with cauliflower.
Despite the heterogeneous conditions across all experiments, the power regression model seems to be the model that best explains the data and describes the relationship between the inoculum of V. dahliae and sunflower LM. Many studies have found other regression models to describe the relationship between V. dahliae inoculum and disease in susceptible hosts. Khan et al. (Citation2000) found that disease incidence in three greenhouse trials in horseradish was described by quadratic, exponential negative and linear functions. They also found in microplot trials that this relationship was explained by linear (log transformed) and negative exponential regression models. In the present study, we tested these regression models and found lower or no fit to the data than the power regression model (). To relate the inoculum in soil with the disease, we chose severity given that it is highly associated with the amount of infection sites in the roots and with the time at which they occur. These two factors, as suggested by Xiao et al. (Citation1998), are closely related to soil inoculum densities.
Although the same regression model explained the relationship between microsclerotia and LM throughout all our experiments, we observed many differences between experiments that are worth mentioning. Using similar levels of added inoculum, in experiment 1 (pasteurized soil in pots buried in the field), we observed significantly higher levels of disease severity than in experiment 2 (same type of soil but under greenhouse conditions) (up to 80% and 40%, respectively; and ). Similar incidence values and lower LM severity in experiment 2 were possibly due to the high temperature in the greenhouse (19 days with maximum temperatures above 40ºC), which could affect symptom expression (Xiao et al. Citation1998; Khan et al. Citation2000). Lucena et al. (Citation2013) observed a decrease in disease severity associated with temperatures above 28ºC in olive trees. We also observed higher LM severity in pasteurized soil than in non-pasteurized soil for all inoculum densities (). It is known that the pasteurization process reduces the presence of microorganisms, including those that could compete or antagonize with V. dahliae (Berg et al. Citation2005). It is possible that in the non-pasteurized soil used in our trials some microbial action could have decreased microsclerotia viability or its infection ability.
For the same inoculum densities, LM severity was lower in the experiment with natural soilborne inoculum (Experiment 3) than in those experiments with added microsclerotia. Xiao et al. (Citation1998) obtained similar results, suggesting that many variables cannot be controlled in field experiments and that they might affect the relationship between inoculum density and disease. Cultural management, interaction with other microorganisms, physiology of the plant, soil moisture, soil pH, soil type, spatial distribution of pathogens in the soil, strain of the fungus and temperature are amongst the most important factors reported (Berbegal et al. Citation2007; Hamdollah-Zadeh Citation1993; Xiao et al. Citation1998; Quiroz et al. Citation2008; Rodríguez et al. Citation2008; Clemente et al. Citation2017). In further research, these factors could be used as covariables to test how they affect the relationship between microsclerotia in soil and LM.
Due to space restrictions, roots in potted plants tend to explore all the soil, increasing the probability of finding microsclerotia, hence increasing the probability of infection and disease expression. It is important to point out that the natural soilborne inoculum densities were estimated with the semi-selective medium SPT (see previous methods). According to Mpofu and Hall (Citation2003), this is the most accurate technique although it did not detect 100% of the microsclerotia in soil, even at a low inoculum density. If this was the case in our experiments, soilborne inoculum densities should be 55% higher than the estimated ones.
The models developed in this study suggest that there was a progressive decline in microsclerotia capacity to infect and generate symptoms with higher inoculum densities values. Differences in disease levels were higher at low inoculum densities (between 0.1 and 10 microsclerotia/g of soil) than in the high ones. It is likely that in high inoculum densities treatments, competition occurs among microsclerotia to germinate and infect roots. Relationship between potential root infection sites and inoculum density in the soil is a factor to consider because V. dahliae mainly infects near the tip of the root (Gerik and Huisman Citation1988). Data analysis of the experiments performed in different environments in the south of the Buenos Aires province allowed us to identify and develop a robust model that adequately explains the relationship between microsclerotia and LM. We postulate the power regression model with the parameter values found in experiment 3 (LM = 11.6*inoculum density^0.57) as the definitive model to be used to predict disease risk for a given farm before sowing. In further research, this model could be validated in different farms where sunflower will be sown. Knowing the amount of microsclerotia in the soil and its relationship with LM symptoms can be mainly useful as a preventive tool to avoid excessive accumulation of inoculum in the soil. Using this model to recognize a predicted LM risk before sowing will help farmers choose the appropriate field lot and the required resistance level of the sunflower hybrid to be used.
Acknowledgements
This study was possible thanks to the institutions and persons listed below: 1) Faculty of Natural Sciences and Faculty of Agronomy, National University of Mar del Plata, Argentina; National Institute of Agriculture Technology -INTA (fellowship and project grants) and National Foundation for Science and Technology, National Ministry of Science and Technology, Argentina; 2) Gerpe, O., Giuliano, S.; Antonelli, C.; Zabaleta, M; Montoya, M. and Cerrudo, I.
References
- Akaike H. 1974. A new look at the statistical model identification. IEEE Trans Automat Contr. 19:716–723.
- Berbegal M, Ortega A, Garcia-Jiménez J, Armengol J. 2007. Inoculum density-disease development relationship in Verticillium wilt of artichoke caused by Verticillium dahliae. Plant Dis. 91:1131–1136.
- Berg G, Krechel A, Ditz M, Sikora RA, Ulrich A, Hallmann J. 2005. Endophytic and ectophytic potato-associated bacterial communities differ in structure and antagonistic function against plant pathogenic fungi. FEMS Microbiol Ecol. 51:215–229.
- Clemente GE, Bazzalo ME, Escande AR. 2017. New variants of Verticillium dahliae causing sunflower leaf mottle and wilt in Argentina. J Plant Pathol. 99:19.
- Eynck C, Koopmann B, Grunewaldt-Stoecker G, Tiedemann A. 2007. Differential interactions of Verticillium longisporum and V. dahliae with Brassica napus detected with molecular and histological techniques. Eur J Plant Pathol. 118:259–274.
- Gerik JS, Huisman OC. 1988. Study of field-grown cotton roots infected with Verticillium dahliae using an immunoenzymatic staining technique. Phytopathology. 78:1174–1178.
- Gould JC, Termorshuizen AJ. 2003. Quality of methods to quantify microsclerotia of Verticillium dahliae in soil. Eur J Plant Pathol. 109:523–534.
- Hamdollah-Zadeh A. 1993. Properties of defoliant and non-defoliant strains of Verticillium dahliae, the causal agent of cotton wilt in northern Iran. Iran J Plant Pathol. 29:53–54.
- Khan A, Atibalentja N, Eastburn DM. 2000. Influence of inoculum density of Verticillium dahliae on root discoloration of horseradish. Plant Dis. 84:309–315.
- Lin LI. 1989. A concordance correlation coefficient to evaluate reproducibility. Biometrics. 45:255–268.
- López-Escudero FJ, Blanco-López MA. 2007. Relationship between inoculum density of Verticillium dahliae and the progress of Verticillium wilt of olive. Plant Dis. 91:1372–1378.
- López-Escudero FJ, Núñez-Santos D, Blanco-López MA. 2003. Aislamiento de Verticillium dahliae de suelo y caracterización morfológica de sus microesclerocios. [Isolation of Verticillium dahliae from soil and morphological characterization of its microsclerotia.]. Bol San Veg Plagas. 29:613–626.
- Lucena C, Trapero-Casas JL, Remesal E, Navas-Cortés JA 2013. Verticillium dahliae pathotypes and olive cultivars determine geographic distribution and development of Verticillium wilt under current and future climate change scenarios in Southern Spain. Program and Abstracts of the 11th International Verticillium Symposium. May; Göttingen, Germany: Georg-August-Universität, p. 5–8.
- Madden LV, Hughes G, van den Bosch F. 2007. The study of plant disease epidemics. St. Paul (MN): APS Press; p. 451.
- Markell, S., Harveson, R., Block, C.C., Gulya, T. 2015. In: Martínez-Force, E., Dunford, N. T., Salas, J. J., editors. Sunflower: Chemistry, Production, Processing and Utilization. Urbana, IL: American Oil Chemists' Society Press; p. 93–128.
- Mol L, Van Riesen HW. 1995. Effect of plant roots on the germination of microsclerotia of Verticillium dahliae. I. Use of root observation boxes to assess differences among crops. Eur J Plant Pathol. 101:673–678.
- Mpofu SI, Hall R. 2003. Accuracy and precision of population estimates of Verticillium dahliae on growth media in quantitative soil assays. Can J Bot. 81:294–306.
- Nagtzaam MPM, Termorshuizen AJ, Bollen GJ. 1997. The relationship between soil inoculum density and plant infection as a basis for a quantitative bioassay of Verticillium dahliae. Eur J Plant Pathol. 103:597–605.
- Paplomatas EJ, Bassett DM, Broome JC, DeVay JE. 1992. Incidence of Verticillium wilt and yield losses of cotton cultivars (Gossypium hirsutum) based on soil inoculum density of Verticillium dahliae. Phytopathology. 82:1417–1420.
- Pearson K. 1895. Notes on regression and inheritance in the case of two parents. Proceed Royal Soc London. 58:240–242.
- Pegg GF. 1974. Verticillium diseases. Rev Plant Pathol. 53:157–182.
- Pereyra V, Escande A. 1999. Enfermedades del girasol. Unidad Integrada Balcarce, Argentina. Cuaderno de actualización técnica. [Sunflower diseases. Integrated Unit Balcarce, Argentina. Technical update handbook]. Vol. 62. Buenos Aires (Argentina): INTA; p. 85–87.
- Pereyra V, Quiroz F, Agüero ME, Escande A. 1999. Relación del rendimiento de girasol con la intensidad de síntomas provocados por Verticillium dahliae. X Jornadas Fitosanitarias Argentinas, Jujuy, Argentina. [Relationship between sunflower yield symptoms intensity caused by Verticillium dahliae. X Argentinian phytosanitary meeting, Jujuy, Argentina.]. Jujuy (Argentina); p. 35.
- Pullman GS, DeVay JE. 1982. Effect of soil flooding paddy rice culture on the survival of Verticillium dahliae and incidence of Verticillium wilt in cotton. Phytopathology. 72:1285–1289.
- Quiroz F, Corro Molas A, Rojo R, Pérez Fernández J, Escande A. 2008. Effects of no tillage and genetic resistance on sunflower wilt by Verticillium dahliae. Soil Tillage Res. 99:66–75.
- Rodríguez E, García-Garrido JM, García PA, Campos M. 2008. Agricultural factors affecting Verticillium wilt in olive orchards in Spain. Eur J Plant Pathol. 122:287–295.
- Schneiter AA, Miller JF. 1981. Description of sunflower growth stages. Crop Sci. 21:901–903.
- Wilhelm S, George A. 1955. Incidence of Verticillium wilt in cotton not affected by root-knot nematodes. Plant Dis. 39:226–227.
- Xiao CL, Subbarao KV, Schulbach KF, Koike ST. 1998. Effects of crop rotation and irrigation of microsclerotia in soil and wilt in cauliflower. Phytopathology. 88:1046–1055.