Abstract
The pathogenic Fusarium oxysporum species complex (FOSC) causes significant yield losses in the family Solanaceae including on tomato, eggplant and pepper in the Aegean and Mediterranean regions of Turkey. Fusarium wilt of pepper, caused by Fusarium oxysporum f. sp. capsici (Foc), has emerged recently in Turkey, where its significance has not been investigated thoroughly. In the present study, disease prevalence was determined with surveys conducted in 67 pepper-growing greenhouses and 56 pepper fields in the Aegean and Mediterranean regions in 2015 and 2016. In both years, about 32% of the greenhouses and 55% of the fields were found to be infested with the wilting disease. It was observed in a pathogenicity experiment that 64 isolates were pathogenic to pepper. In cross-inoculation experiments, these isolates did not cause wilting symptoms on tomato and eggplant and therefore were designated as Foc. Phylogenetic analyses were carried out with TEF-1α and actA DNA regions of a selected subset of 40 Foc isolates, along with non-host formae of F. oxysporum and outgroup Fusarium species. Sequence data for the TEF-1α and actA DNA regions displayed limited variation among Foc isolates and failed to distinguish reliably several formae speciales of F. oxysporum. The phylogenetic tree successfully separated the outgroup isolates. All Foc isolates were placed in the same branch, suggesting a single monophyletic group in the dendrogram. This is the first comprehensive study for pathogenic and phylogenetic analysis of Foc isolates from peppers in Turkey.
Résumé
Dans les régions égéenne et méditerranéenne de la Turquie, le complexe d’espèces pathogènes Fusarium oxysporum cause d’importantes pertes de rendement chez les solanacées, y compris la tomate, l’aubergine et le poivron. La jaunisse fusarienne du poivron, causées par Fusarium oxysporum f. sp. capsici (Foc), est apparue récemment en Turquie où son importance n’a pas été étudiée de façon approfondie. Dans cette étude, menée en 2015 et 2016 dans les régions égéenne et méditerranéenne, la prévalence de la maladie a été déterminée par un examen effectué dans 67 serres et 56 champs où l’on cultive le poivron. Durant les deux années, l’examen a démontré que 32% des serres et 55% des champs étaient infestés par la jaunisse. Au cours d’un essai de pathogénicité, nous avons observé que 64 isolats se sont avérés pathogènes pour le poivron. Au cours d’expériences d’inoculation croisée, ces isolats n’ont causé aucun symptôme de la jaunisse ni chez la tomate ni chez l’aubergine et, en conséquence, ont été désignés comme Foc. Des analyses phylogénétiques ont été faites sur les régions du TEF-1α et l’actA de l’ADN d’un sous-ensemble sélectionné de 40 isolats de Foc, parallèlement à des formes spéciales d’espèces non-hôtes de F. oxysporum et à des espèces de Fusarium hors groupe. Les données de séquences pour les régions du TEF-1α et le l’actA de l’ADN ont affiché une faible variation chez les isolats de Foc et n’ont pas permis de distinguer avec certitude plusieurs formes spéciales de F. oxysporum. L’arbre phylogénétique a permis de différencier les isolats hors groupe. Tous les isolats de Foc ont été placés sur la même branche, indiquant un groupe monophylétique unique dans le dendrogramme. Il s’agit de la première étude complète quant à l’analyse des variations pathogéniques et phylogénétiques chez des isolats de Foc provenant du poivron en Turquie.
Introduction
Pepper (Capsicum annuum L.) is grown worldwide on over 4,265,483 ha with an annual production of 38,961,880 tons (FAO Citation2016). In Turkey, pepper is cultivated over an area of 80,517 ha with an annual production of 2,608,172 tons (TUIK Citation2017). Fusarium wilt is caused by the soil-borne fungus Fusarium oxysporum (Schlect.) emend. Synd. & Hans. f. sp. capsici Riv. (Foc), which has recently emerged in local pepper fields. This disease was first reported in New Mexico (Leonian Citation1919). Thereafter, it was reported from Avery Island (Black and Rivelli Citation1990), Java (Agrios Citation2005; Ferniah et al. Citation2014), Egypt, Pakistan (Bashir et al. Citation2018), and Mexico (Velarde-Félix et al. Citation2018). Fusarium wilt is a widespread disease of peppers throughout the world and several recent studies reported that root and basal stem rot disease occurred on this crop (Pérez-Hernández et al. Citation2014; Cerkauskas Citation2017). Wilting and root-rot diseases caused by Rhizoctonia solani, Macrophomina phaseolina, F. oxysporum, and F. solani have also been recently reported in pepper fields in the Cukurova plains and Southeastern Anatolia region of Turkey (Yücel et al. Citation2013; Güler Güney and Güldür Citation2018).
Present field observations revealed that the prevalence of fusarium wilt in pepper has increased in recent years, especially within the Mediterranean region of Turkey. In this study, surveys were conducted in 2015 and 2016 for wilt disease caused by Foc in pepper greenhouses and fields in the Mediterranean and Aegean regions of Turkey. The characteristic symptoms of fusarium wilt of pepper include wilting, stunting, leaf chlorosis, slight vein clearing on the outer leaflets, vascular necrosis, and ultimately death (Black and Rivelli Citation1990; Agrios Citation2005). Vegetative compatible group/groups (VCGs) and physiological race/races of this pathogen have not been reported in previous studies. Members of the F. oxysporum species complex (FOSC; Booth Citation1971) are the most common phytopathogenic Fusaria that cause wilt and root-rot. These species cause vascular wilts in many cultivated plant species, including tomato, potato, eggplant, cucumber, and pepper. FOSC consists of both non-pathogens and host-specific pathogens that are morphologically indistinguishable (Gordon and Martyn Citation1997). Pathogenic strains of F. oxysporum generally display high host specificity based on virulence on a single host or group of hosts and are classified into formae speciales (ff. spp). Control of fusarium wilt is difficult, because the pathogens are systemic and can remain dormant for a very long time in soil as chlamydospores until induced to germinate (Nelson et al. Citation1983). Conventional control of FOSC is inadequate in most cases. The presence and viability of pathogens in soil depend largely on their ability to survive in a suitable host. Wild plants and weeds are alternative hosts, and cultivated plants have been shown to be symptomless carriers of Fusarium spp. (Katan Citation1971; Postic et al. Citation2012; Altinok Citation2013). Plant residues can also serve as a reservoir for plant pathogens (Postic et al. Citation2012).
DNA-based diagnostic tools have allowed researchers to comprehend the genetic diversity and phylogeny of Fusarium species. Many methods have been used to characterize the genetic diversity and evolutionary origin of FOSC, including vegetative compatibility grouping (VCG) (Correll Citation1991), restriction fragment length polymorphisms (RFLPs) (Appel and Gordon Citation1995), random amplified polymorphic DNA markers (RAPDs) (Assigbetse et al. Citation1994), amplified fragment length polymorphisms (AFLPs) (Baayen et al. Citation2000), simple sequence repeats (SSRs, also known as microsatellites) (Jarne and Lagoda Citation1996), and comparisons of DNA sequences from conserved genomic regions (O’Donnell et al. Citation1998). The protein-coding regions of nuclear DNA also have been analyzed extensively in FOSC phylogenetic studies for high-resolution comparisons. DNA barcoding is a taxonomic method used in different fungal groups. The most commonly and successfully used sequence-based markers in FOSC include housekeeping genes. Sequence analysis of the mitochondrial small subunit (mtSSU), translation elongation factor 1-α (TEF-1α), β-tubulin, histone, actin, chitin synthase, and calmodulin genes, nuclear ribosomal DNA (rDNA), and nuclear rDNA intergenic spacer (IGS) have been used to determine the genus Fusarium (Jiménez-Gasco et al. Citation2002; Schmitt et al. Citation2009). Elongation factor-1α and actin genes involved in basic cellular functions encode highly conserved proteins identified in fungi (Schmitt et al. Citation2009; Altinok et al. Citation2018). These techniques are able to detect polymorphisms in the DNA sequence of a genome. Nuclear protein-coding genes could be utilized via sequencing, BLAST search, and phylogenetic analysis to provide a reliable guide to the species-level identification (Raja et al. Citation2017).
This study was conducted to identify the Foc isolates from the Mediterranean and Aegean regions of Turkey, by pathogenicity at the forma specialis level. In addition, the DNA polymorphisms among Foc isolates in transcription elongation factor (TEF-1α) and actin (actA) gene sequences were analyzed with the hierarchical maximum likelihood clustering method. To the best of our knowledge, this is the first detailed study to report F. oxysporum f. sp. capsici (Foc), causal agent of fusarium wilt of pepper in Turkey.
Materials and methods
Plant materials
In 2015, 42 greenhouses and 38 fields, and in 2016, 25 greenhouses and 18 fields were surveyed to collect pepper samples exhibiting fusarium wilt symptoms from the major pepper-producing provinces located in the Mediterranean (Antalya, Mersin, Adana, and Hatay) and Aegean regions (Aydin, Izmir, Mugla, and Manisa). Sampling locations are presented in . Depending on field size, 5–10 plants were sampled per greenhouse or field by walking diagonally. Samples from each greenhouse/field were transferred to the laboratory in labelled paper bags. Sampling was performed between June and August of both years on the common pepper cultivars, namely green pepper ‘Carliston-Long’, bell pepper, and red pepper ‘Capia’. In addition to sampling, the greenhouse/field geographic coordinates were recorded with a GPS (Global Positioning System) unit. These survey areas are the most representative sites with respect to greenhouse/field pepper production systems of the relevant regions.
Fig. 1 (Colour online) Fusarium oxysporum f. sp. capsici (Foc) isolate collection across the provinces of Turkey in 2015 (a) and 2016 (b). Pie charts show percent distribution of three virulence groups (based on disease severity values) of the Foc isolates collected from pepper plants per province (see and ).
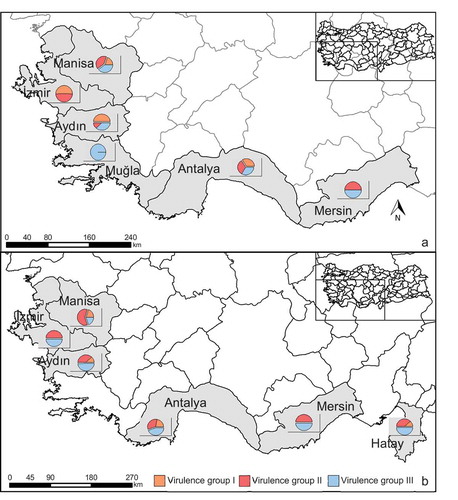
In the pathogenicity experiments, seedlings of C. annuum (‘Atalante F1’) (susceptible to wilting) were used. Tomato (‘H-2274ʹ) and eggplant (‘Kemer’) seedlings were used in cross-inoculation experiments.
Fungal isolation and identification
Stem tissues of around 15 cm in length were sliced into small pieces (approximately, 0.3 cm) from the margin of xylem showing dark-brown necrosis and surface-disinfested with 2.0% NaOCl (1 min), followed by a rinse for 1 min in sterile water. After drying, the plant tissues were placed into 90 mm-diameter Petri plates, containing potato dextrose agar (PDA; Merck, Darmstadt, Germany) and incubated for 7 days in the dark at 25°C. Pure cultures of a total of 129 F. oxysporum isolates were obtained by the single-spore technique and identified based on morphologic characteristics. For single-spore isolation, a drop of conidial suspension from aerial mycelia (approx. 5 µL) was transferred to a predefined location on the water agar in a Petri plate. The drop was streaked across the agar surface with an inoculation needle and then incubated for 12–16 h at 24°C. Germinating conidia was located under low-magnification with a microscope and then transferred with the underlying water agar block to PDA medium for incubation (Nelson et al. Citation1983; Leslie and Summerell Citation2006). Fungal cultures were stored in 20% glycerol at −80°C until used. Molecular identification of morphologically characterized isolates was conducted using the universal F. oxysporum identification primers (uni-f ‘ATCATCTTGTGCCAACTTCAG’ & uni-r ‘GTTTGTGATCTTTGAGTTGCCA’ 670 bp for F. oxysporum) (Hirano and Arie Citation2006).
Pathogenicity screening
The pathogenicity of 129 F. oxysporum isolates on C. annuum ‘Atalante F1ʹ seedlings at the six-leaf stage was tested using the root-dip inoculation method. Pepper seedlings with wounded roots were submerged into a conidial suspension (106 conidia per ml water) for 10 min, while control plants were dipped in sterile distilled water. The inoculated seedlings were transplanted into pots (8.5 cm diameter) and kept in growth chambers (27°C, 80–90% RH, 12-h photoperiod, 60 Klux/m2). All of the F. oxysporum isolates pathogenic on pepper were inoculated individually on tomato and eggplant seedlings in cross-inoculation tests. All experiments were conducted in a completely randomized design with three replicates (three pots per replicate, with each pot containing three seedlings), and repeated twice for all isolates with similar results. Pepper seedlings were also inoculated with the same Foc isolates in the cross-inoculation tests. Data from the first pathogenicity experiment are presented in this paper.
The development of disease symptoms was recorded at 10, 15, 20, and 25 days after inoculation (DAI) using the fusarium wilt rating scale of Altinok and Can (Citation2010) with minor modifications. The disease severity was assessed based on the scale values obtained on the 25th DAI, according to the formula of Townsend and Heuberger (Citation1943). The data were subjected to Levene’s homogeneity of variance test followed by Tukey’s HSD test (P ≤ 0.05) in JMP v9.0 (SAS Institute Inc., Carry, NC, USA). The area under the disease progress curve (AUDPC) was calculated following Campbell and Madden (Citation1990) for all Foc isolates; the Pearson correlation coefficient between disease severity and AUDPC also was calculated (Altinok and Can Citation2010). Disease severity (DS) values were further classified into three groups by recursive partition analysis (RPA) in JMP v9.0, for easier interpretation of the DS caused by several isolates.
DNA extraction and PCR assays
Forty purified monoconidial Foc isolates representing various geographical locations were selected as a subset from the Foc isolates and further characterized using PCR analysis. A subset of 40 Foc isolates, along with non-host f. sp. isolates (F. oxysporum f. sp. lycopersici, Fol; F. oxysporum f. sp. radicis-lycopersici, Forl; F. oxysporum f. sp. melongenae, Fomg), was selected for phylogenetic analyses based on the TEF-1α and actA gene sequences. Non-host isolates were obtained from the culture collection of the Mycology Laboratory, Plant Protection Department, Erciyes University, Turkey. TEF-1α sequences of F. oxysporum pathogenic on eggplant (GenBank accessions numbers KT780441.1, KF928931.1, HG916993.1) were included. As outgroups, F. proliferatum (PKMI01000011.1) and F. solani (KY486673.1) sequences from GenBank were also incorporated into the analysis. There were no records found in GenBank for the actA gene region of Foc, but actA sequences of F. oxysporum f. sp. dianthi (LT841214) were included in the analysis. As outgroups, F. proliferatum (KM231217) and F. subglutinans (KU603821) actA sequences from GenBank were used.
For DNA extraction, the method of Altinok et al. (Citation2018) was used. Mycelial tissue plugs from a 7-day-old culture were transferred aseptically to 50 mL potato dextrose broth (PDB; Difco, Detroit, USA). Cultures were grown on an orbital shaker (∼120 rpm) at 28°C for 7 days. Mycelium was collected by vacuum filtration with a Buchner funnel with sterile filter paper. Approximately 200 mg tissue was ground in liquid nitrogen, placed in 400 μL lysis buffer for 45 min (Tissue Lysis Buffer, ExiPrep™ Tissue Genomic DNA Kit, Bioneer, USA), and incubated at 60°C for 1 h. After incubation, the suspensions were centrifuged at 4°C for 5 min at 16 700 × g. After decanting the supernatant, 200 μL was loaded into wells and DNA was extracted using ExiPrep™ 16 (Fully Automated Nucleic Acid Extraction System, Bioneer, USA), with the fungal DNA extraction preset. The DNA concentration and 260/280 ratios were determined using a NanoDrop ND-1000 spectrophotometer (NanoDrop Products, USA).
TEF-1α and actA amplification, sequencing and alignment
The PCR reactions were performed in a total volume of 20 μL containing 10x PCR buffer, 2 mM of dNTPs, 10 mM of each primer, 1 U of Taq DNA Polymerase (Thermo Scientific), and 25 ng of genomic DNA. A negative control, with sterile double-distilled water instead of DNA, was included in each experiment. Amplification of the TEF-1α (TEF 2 elongation factor 1-728F and TEF 4 elongation factor 1-986R) and actin (ACT-512F and ACT-783R genes) gene regions was performed with the following reaction conditions: 5 min of denaturation at 94°C; 35 cycles of 30 s at 94°C, 30 s at 55°C and 1 min at 72°C; and a final extension for 10 min at 72°C. The sequences of the TEF-1α and actA primers and PCR conditions used are given in . Amplification products were electrophoresed on a 1.5% agarose gel in 0.5X Tris-borate EDTA buffer and stained with ethidium bromide. Images were acquired with a gel documentation system (DNR Bio-Imaging System) under UV light. The primers were obtained from Operon Technologies GmbH (Cologne, Germany). PCR reactions were purified with the Qiagen QIAquick 96 PCR Purification Kit (Germany) according to the manufacturer’s instructions. The products were sequenced by Ref-Gen Biotechnology (Ankara, Turkey). Representative sequences of TEF-1α (accession no. MK001024) and actA (accession no. MK001023) were deposited in the GenBank database.
Table 1. Primer codes and sequences used for the analysis of the translation elongation factor-1α (TEF-1α) and actin (actA) genes of the Fusarium oxysporum species complex in this study.
Molecular statistical analyses
TEF-1α and actA consensus sequences were created using Geneious v10.0.5 (Biomatters, NZ, USA). Multiple alignments of consensus sequences were made with ClustalW and inspected manually for single-nucleotide changes (Larkin et al. Citation2007). The Megablast algorithm (Morgulis et al. Citation2008) was used to perform similarity searches with the F. oxysporum sequences in GenBank (Hall Citation1999). A dendrogram of the consensus sequences was created with MEGA 7 (Huelsenbeck and Ronquist Citation2001; Tamura et al. Citation2013), using the hierarchical maximum likelihood clustering method, with 2000 bootstrap repetitions (Sneath and Sokal Citation1973).
Results
Greenhouse and field surveys
The F. oxysporum isolates were collected from peppers with wilting symptoms in different cultivation areas of Turkey. Disease prevalence was determined with surveys in 67 pepper-growing greenhouses and 56 fields in 2015 and 2016. In the Mersin and Antalya provinces of the Mediterranean region and in the Mugla, Aydin, Izmir, and Manisa provinces of the Aegean region, 29% of the greenhouses and 51% of the fields investigated were found to be infested with wilting disease in 2015 (). A total of 502 pepper plants displaying disease symptoms were sampled, 148 Fusarium isolates were obtained after isolations from these plants, and 69 isolates were identified as F. oxysporum. In 2016, Adana, Hatay, Mersin and Antalya provinces in the Mediterranean region, and Mugla, Aydin, Izmir and Manisa provinces in the Aegean region were surveyed; 35% of the greenhouses and 59% of the fields examined were found to be infested with wilting disease. Two hundred and eleven plants displaying wilting symptoms were sampled, 106 Fusarium isolates were obtained by isolations from these plant materials, and 60 isolates were identified as F. oxysporum. Identifications made by macroscopic and microscopic characterization () were confirmed by PCR with universal F. oxysporum identification primers. The geographic origin of the F. oxysporum isolates recovered from Turkish pepper greenhouse and field crops in 2015 and 2016 is given in .
Fig. 2 (Colour online) Symptoms of fusarium wilt of pepper, along with culture and conidial characteristics, under light microscopy. Symptoms on plant (a), Foc-infected pepper seedling (right) in pathogenicity experiment (b), leaf and vascular bundle symptoms (c,d), colony morphology of isolate Foc-ANSR-40/2 (e), micro- and macroconidia stained with lactophenol cotton-blue, at 30 µm bar scale (f–h).
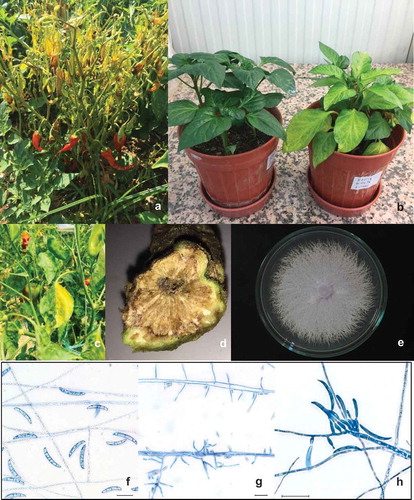
Pathogenicity experiments
Sixty-four of the 129 F. oxysporum isolates tested were pathogenic on the pepper ‘Atalante F1ʹ and designated as F. oxysporum f. sp. capsici (Foc), fulfilling Koch’s postulates. The non-inoculated seedlings exhibited no symptoms. In this experiment, the initial symptoms were observed 10 days after inoculation as slight chlorosis of the older leaves. The wilting symptoms progressed systemically until the 25th day, and some infected seedlings eventually collapsed. The symptoms exhibited in inoculated plants () were similar to those observed in the field, including leaf chlorosis, necrosis, dropping of the leaves, and browning of the vascular bundles. None of the 64 isolates of F. oxysporum pathogenic on pepper caused any wilting symptoms on tomato and eggplant in the cross-inoculation tests, confirming their designation as F. oxysporum f. sp. capsici (Foc).
Disease progress was expressed as disease severity and the AUDPC rates as percentages of the maximum AUDPC values ( and ). A strong correlation was observed between disease severity (on the 25th DAI) and AUDPC for all isolates for both 2015 (r = 0.87) and 2016 (r = 0.86). In 2015, based on percent disease severity, three main virulence groups were determined in the 38 Foc isolates from Turkey: 14 isolates were low-virulent (virulence group I; DS (Disease Severity) 28–42%), 13 were moderate-virulent (virulence group II; DS 43–52%), and 11 isolates were classified as virulent (virulence group III; DS 53–72%). The isolate ANSR-39/3 (Antalya, Serik) was found to be the most virulent based on disease severity (71.67%) on the 25th DAI, with an AUDPC value of 85.71%. This was followed by isolates ANLY-29/4, MSSR-86/7 and ANSR-40/2 (DS ranging between 66% and 70%). In 2016, three virulence groups were again identified in the 26 Foc isolates: three isolates were low-virulent (virulence group I; DS 40–48%), 11 were moderate-virulent (virulence group II; DS 57–60%), and 12 isolates were classified as virulent (virulence group III; DS 67–75%). Among these, the isolate HTKR-21/50 (Hatay, Kirikhan) was found to be the most virulent based on disease severity (75%) on the 25th DAI, with an AUDPC value of 100%. The next most virulent was the isolates HTKR-21/50, ANSR-39/3, ANKS-10/50 and ANLY-29/4 (DS ranging between 70% and 75%). The percent distribution of the three virulence groups is presented in for each province. Wilting symptoms in the field were generally at the level of moderate virulence in both years, and no strong wilting symptoms were encountered. These observations are consistent with the virulence of the Foc isolates in the pathogenicity tests.
Table 2. Geographical origin of Fusarium oxysporum isolates recovered from pepper greenhouses and fields in Turkey in 2015 and 2016.
Table 3. Virulence grouping and the area under the disease progress curve (AUDPC) of the Fusarium oxysporum f. sp. capsici (Foc) isolates obtained from pepper greenhouses and fields in 2015 in Turkey.
Table 4. Virulence grouping and the area under the disease progress curve (AUDPC) of Fusarium oxysporum f. sp. capsici (Foc) isolates obtained from pepper greenhouses and fields in 2016 in Turkey.
Phylogenetic analysis of Fusarium oxysporum f. sp. capsici (Foc) isolates
The Foc isolates yielded a single amplicon of 290 bp for TEF-1α and 300 bp for actA in conventional PCR. A molecular phylogenetic dendrogram was generated for the TEF-1α () and actA () DNA regions, based on sequences of the PCR products. There were three major clusters in the TEF-1α dendrogram. Two of the clusters contained the outgroup isolates (F. solani and F. proliferatum), while the other cluster contained a whole subset of Foc isolates, pathogenic F. oxysporum (other F. oxysporum obtained from pepper in different countries, sourced from GenBank) and non-host formae of F. oxysporum (tomato and eggplant, from our culture collection) (). The TEF-1α gene region was found to be inadequate to provide an intraspecific differentiation for the FOSC.
Fig. 3 Phylogenetic tree constructed using the hierarchical maximum likelihood clustering method with 2000 bootstrap replicates, based on sequences of the translation elongation factor 1-α (TEF-1α) gene region of each organism. It contains 40 Fusarium oxysporum f. sp. capsici (Foc) isolates along with non-host f. sp. isolates. As outgroups, F. proliferatum (PKMI01000011.1) and F. solani (KY486673.1) sequences from GenBank were included. *Tomato: F. oxysporum f. sp. lycopersici-Fol; 07AAK52/15, 07AKK2.2/15-1 and F. oxysporum f. sp. radicis-lycopersici-Forl; 07AAK10/15, 07AAK26/15-a. **Eggplant: F. oxysporum f. sp. melongenae-Fomg; Fomg140, UBR-24. ***Pepper: Pathogenic F. oxysporum (GenBank accessions KT780441.1, KF928931.1, HG916993.1).
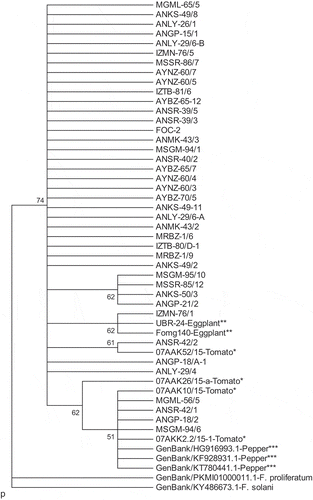
Fig. 4 Phylogenetic tree constructed using the hierarchical maximum likelihood clustering method with 2000 bootstrap replicates, based on sequences of actin (ActA) gene regions of each organism. It contains 40 Fusarium oxysporum f. sp. capsici (Foc) isolates along with non-host f. sp. isolates. As outgroups, F. proliferatum (KM231217) and F. subglutinans (KU603821) sequences from GenBank were included. *Tomato: F. oxysporum f. sp. lycopersici-Fol; 07AKK3.1/15-1, 07AAK52/15 and F. oxysporum f. sp. radicis-lycopersici-Forl; 07AAK26/15-a, 07AAK10/15. **Eggplant: F. oxysporum f. sp. melongenae-Fomg; Fomg236, Fomg219. ***Non-host: F. oxysporum f. sp. dianthi (GenBank accession LT841214).
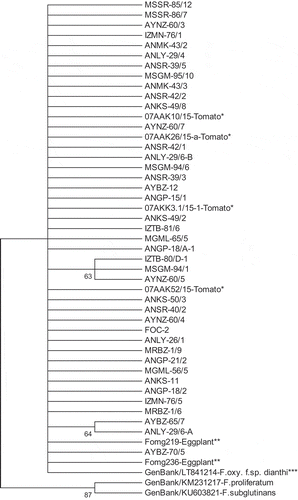
ActA gene region sequences yielded a highly similar dendrogram with three main clusters, as in TEF-1α. Outgroup isolates (F. solani and F. proliferatum) again formed two individual clusters. All of the FOSC grouped together and there was no significant discrimination among non-host F. oxysporum isolates (F. oxysporum f. sp. melongenae, F. oxysporum f. sp. lycopersici, F. oxysporum f. sp. radicis-lycopersici) and F. oxysporum f. sp. dianthi obtained from GenBank (). The sequence similarity for the TEF-1α and actA DNA regions was not related to the virulence or geographical origin of the Foc isolates.
Discussion
The present study found F. oxysporum f. sp. capsici (Foc) as the causal agent of fusarium wilt of pepper in Turkey, along with genetic relatedness among F. oxysporum specific to Solanaceae hosts. Conventional control of the FOSC is notoriously difficult, since the chlamydospores can survive for long periods in the soil. Management strategies for the FOSC have shown limited success, mainly due to the emergence of new pathogenic races (Silva and Bettiol Citation2005). New and emerging strains of these pathogens, such as F. oxysporum f. sp. lactucae, are becoming more widespread each passing year (Gilardi et al. Citation2017, Citation2018). Therefore, it is important to have up-to-date information about the prevalence and phylogenetic properties of emerging FOSC, for the implementation of appropriate disease management strategies.
Pepper plants were generally found to have moderate symptoms in the greenhouse and field surveys. This was consistent with the virulence of the Foc isolates in the pathogenicity tests. Large-scale surveys in the Mediterranean and Aegean Regions revealed that the disease was not prevalent and devastating. Pathogenicity tests on a susceptible pepper cultivar showed that the initial symptoms appeared 10 days after inoculation as yellowing of the bottom leaves, with wilting spreading to the younger ones. While the wilting progressed systemically, in general, the number of plants that had died was limited, even by the last day of the experiment. In the stems of infected plants, a brown discolouration was observed in the vascular bundles.
The morphological identification of the Foc isolates from pepper plants was confirmed by BLAST analysis of the TEF-1α DNA sequences (no records for the ActA gene region of Foc were found in the GenBank database). These regions are commonly used in fungal molecular characterization and taxonomic classification (Schmitt et al. Citation2009; Ma et al. Citation2010; Altinok et al. Citation2018). PCR-based detection techniques have provided adequate information and can represent an alternative to the morphological identification of Fusarium species (O’Donnell et al. Citation1998). In this study, protein-coding gene regions (TEF-1α and actA) failed to discriminate Foc from non-host formae of F. oxysporum isolates. These regions may not be suitable for host-based discrimination in F. oxysporum. In the TEF-1α region of F. oxysporum f. sp. ciceris, little genetic diversity was observed between races of this fungus, although there were significant phenotypic differences between races of this forma specialis (Demers et al. Citation2014). Dubey et al. (Citation2014) used TEF-1α to compare F. oxysporum f. sp. ciceris from chickpea and reported no relationship with race specificity. Pathogenic and nonpathogenic isolates may not be distinguished based on sequence variation in the ‘house-keeping’ genes of the core genome (Ma et al. Citation2010). In present phylogenetic analyses, both the TEF1-α and actin gene regions enabled interspecies discrimination for the tested outgroups but were found to be inadequate at the intraspecies level. These results are in line with the findings of other studies carried out on various F. oxysporum formae speciales (Baayen et al. Citation2000; Bao et al. Citation2002; Bogale et al. Citation2006). Forma specialis is a phenotypic character and is defined by pathogenicity, which is influenced by several factors that may not be correlated with phylogenetic analyses (Leslie and Summerell Citation2006).
Host-specificity is associated with the presence of accessory or lineage-specific (LS) chromosomes, which contain effector genes associated with pathogenicity. Pathogenic effector proteins are important for virulence and interact or interfere with host proteins (De Wit et al. Citation2009). Horizontal transfer of accessory chromosomes is a major force driving the evolution of new virulent pathotypes of the FOSC (Ma et al. Citation2010). While the FOSC shares highly similar core chromosomes, the variation in the accessory genome increases host-specificity and virulence. Determination of F. oxysporum formae speciales requires identification of host specificity factors, which may be revealed with a range of methods, such as the characterization of the pathosystem, study of genotype-specific interactions of host-specific strains, and comparative genomics, transcriptomics and proteomics (Borah et al. Citation2018). Formae speciales of F. oxysporum are often polyphyletic within the species complex (Baayen et al. Citation2000), while F. oxysporum f. sp. ciceris formed a monophyletic structure in an analysis of sequences of the TEF-1α region, despite its pathotypic diversity (Jiménez-Gasco et al. Citation2002).
This study showed that the Foc isolates collected from symptomatic pepper grown in Turkey exhibited possible monophyletic ontogeny, and there was no significant variation in phylogenetic analyses of the TEF-1α and actA DNA regions to form genetically different groups. It is possible that the nature of the host–pathogen interaction, along with ecological and epidemiological factors, could have restricted the genetic differentiation of the Foc isolates in Turkey. Basic factors that may play a role in genetic diversification of a fungus include pathogenic ability, alternative host availability and saprophytic ability, as well as resistance to unsuitable environmental conditions for extended periods (Parker and Gilbert Citation2004).
Physiological races may not develop or take extended periods to emerge if there is no resistant cultivar available to cause selection pressure on the fungal population. Fusarium oxysporum f. sp. melongenae (Fomg) may be considered as an example of this, since there are no known cultivars with strong resistance to Fomg, and studies have recorded no races for this formae speciales, either (Altinok et al. Citation2013, Citation2014). In contrast, Zhan et al. (Citation2012) reported a positive and significant correlation between variation in the virulence of Rhynchosporium secalis and barley cultivar diversity over time. Currently, diversity and genetic information about Foc are quite limited. This work may serve as a foundation for future genetic variation studies on Foc. The uniform genetic structure of the Foc population indicated by the current findings could be considered as an advantage in breeding efforts to generate resistant pepper cultivars.
Additional information
Funding
References
- Agrios GN. 2005. Plant pathology. 5th ed. London: Elsevier Academic Press; p. 952.
- Altinok HH. 2013. Fusarium species isolated from common weeds in eggplant fields and symptomless hosts of Fusarium oxysporum f. sp. melongenae in Turkey. J Phytopathol. 161:335–340.
- Altinok HH, Can C. 2010. Characterization of Fusarium oxysporum f. sp. melongenae isolates from eggplant in Turkey by pathogenicity, VCG and RAPD analysis. Phytoparasitica. 38:149–157.
- Altinok HH, Can C, Altinok MA. 2018. Characterization of Fusarium oxysporum f. sp. melongenae isolates from Turkey with ISSR markers and DNA sequence analyses. Eur J Plant Pathol. 150:609–621.
- Altinok HH, Can C, Boyaci HF, Topcu V. 2014. Genetic variability among breeding lines and cultivars of eggplant against Fusarium oxysporum f. sp. melongenae from Turkey. Phytoparasitica. 42:75–84.
- Altinok HH, Can C, Çolak H. 2013. Vegetative compatibility, pathogenicity and virulence diversity of Fusarium oxysporum f. sp. melongenae recovered from eggplant. J Phytopathol. 161:651–660.
- Appel DJ, Gordon TR. 1995. Intraspecific variation within populations of Fusarium oxysporum based on RFLP analysis of the intergenic spacer region of the rDNA. Exp Mycol. 19:120–128.
- Assigbetse KB, Fernandez D, Dubois MP, Geiger JP. 1994. Differentiation of Fusarium oxysporum f. sp. vasinfectum races on cotton by random amplified polymorphic DNA (RAPD) analysis. Phytopathology. 84:622–626.
- Baayen RP, O’Donnell K, Bonants PJM, Cigelnik E, Kroon LPNM, Roebroeck KEJA, Waalwijk C. 2000. Gene genealogies and AFLP analyses in the Fusarium oxysporum complex identify monophyletic and nonmonophyletic formae speciales causing wilt and rot disease. Phytopathology. 90:891–900.
- Bao JR, Fravel DR, O’Neill NR, Lazarovits G, van Berkum P. 2002. Genetic analysis of pathogenic and non-pathogenic Fusarium oxysporum from tomato plant. Can J Bot. 80:271–279.
- Bashir MR, Atiq M, Sajid M, Mohsan M, Abbas W, Alam MW, Bashair M. 2018. Antifungal exploitation of fungicides against Fusarium oxysporum f. sp. capsici causing Fusarium wilt of chilli pepper in Pakistan. Environ Sci Pollut Res. 25(7):6797–6801.
- Black LL, Rivelli V 1990. Fusarium wilt of pepper in Louisiana. Proceedings of 101t1 National Pepper Conference; June 25–27; Wilmington, Delaware.
- Bogale M, Wingfield BD, Wingfield MJ, Steenkamp ET. 2006. Characterization of Fusarium oxysporum isolates from Ethiopia using AFLP, SSR and DNA sequence analyses. Fungal Divers. 23:51–66.
- Booth C. 1971. The genus Fusarium. Kew (Surrey, England): Commonwealth Mycological Institute; p. 237.
- Borah N, Albarouki E, Schirawski J. 2018. Comparative methods for molecular determination of host-specificity factors in plant-pathogenic fungi. Int J Mol Sci. 19(3):863.
- Campbell CL, Madden LV. 1990. Introduction to plant disease epidemiology. New York (NY): John Wiley & Sons.
- Cerkauskas RF. 2017. Etiology and management of Fusarium crown and root rot (Fusarium oxysporum) on greenhouses pepper in Ontario, Canada. Can J Plant Pathol. 39:121–132.
- Correll C. 1991. The relationship between formae speciales, races and vegetative compatibility groups in Fusarium oxysporum. Phytopathology. 81:1061–1064.
- De Wit PJ, Mehrabi R, Van Den Burg HA, Stergiopoulos I. 2009. Fungal effector proteins: past, present and future. Mol Plant Pathol. 10:735–747.
- Demers JE, Garzón CD, Jiménez-Gasco MM. 2014. Striking genetic similarity between races of Fusarium oxysporum f. sp. ciceris confirms a monophyletic origin and clonal evolution of the chickpea vascular wilt pathogen. Eur J Plant Pathol. 139:303–318.
- Dubey SC, Priyanka K, Upadhyay BK. 2014. Development of molecular markers and probes based on TEF-1α, β-tubulin and ITS gene sequences for quantitative detection of Fusarium oxysporum f. sp. ciceris by using real-time PCR. Phytoparasitica. 42:355–366.
- Ferniah RS, Daryono BS, Kasiamdari RS, Priyatmojo A. 2014. Characterization and pathogenicity of Fusarium oxysporum as the causal agent of Fusarium wilt in Chili (Capsicum annuum L.). Microbiology. 8(3):21–126.
- Food and Agriculture Organization of the United Nations. Statistics Division. 2016. [accessed 2018 Aug 10]. http://www.fao.org/faostat/en/#data/QC.
- Gilardi G, Gullino ML, Garibaldi A. 2018. Emerging foliar and soil-borne pathogens of leafy vegetable crops: a possible threat to Europe. EPPO Bull. 48(1):116–127.
- Gilardi G, Pons C, Gard B, Franco-Ortega S, Gullino ML. 2017. Presence of Fusarium Wilt, Incited by Fusarium oxysporum f. sp. lactucae, on Lettuce in France. Plant Dis. 101(6):1053.
- Gordon TR, Martyn RD. 1997. The evolutionary biology of Fusarium oxysporum. Annu Rev Phytopathol. 35:111–128.
- Güler Güney İ, Güldür ME. 2018. Inoculation techniques for assessing pathogenicity of Rhizoctonia solani, Macrophomina phaseolina, Fusarium oxysporum and Fusarium solani on pepper seedlings. Turk J Agric Res. 5(1):1–8.
- Hall TA. 1999. BioEdit: a user-friendly biological sequence alignment editor and analysis program for Windows 95/98/NT. Nucleic Acids Symp Ser. 41:95–98.
- Hirano Y, Arie T. 2006. PCR-based differentiation of Fusarium oxysporum ff. sp. lycopersici and radicis-lycopersici and races of F. oxysporum f. sp. lycopersici. J Gen Plant Pathol. 72:273–283.
- Huelsenbeck JP, Ronquist F. 2001. MRBAYES: bayesian inference of phylogenetic trees. Bioinformatics. 17:754–755.
- Jarne P, Lagoda PJL. 1996. Microsatellites, from molecules to populations and back. Trends Ecol Evol. 11:424–429.
- Jiménez-Gasco MM, Milgroom MG, Jiménez-Díaz RM. 2002. Gene genealogies support Fusarium oxysporum f. sp. ciceris as a monophyletic group. Plant Pathol. 51:72–77.
- Katan J. 1971. Symptomless carriers of the tomato Fusarium wilt pathogen. Phytopathology. 61:1213–1217.
- Larkin MA, Blackshields G, Brown NP, Chenna R, McGettigan PA, McWilliam H, Valentin F, Wallace IM, Wilm A, Lopez R, et al. 2007. ClustalW and ClustalX version 2. Bioinformatics. 23:2947–2948.
- Leonian LH 1919. Fusarium wilt of chile pepper. New Mexico Agricultural Experimental Station Technical Bulletin. No:121.
- Leslie JF, Summerell BA. 2006. The Fusarium laboratory manual. Ames (Iowa): Blackwell Publishing.
- Ma LJ, van der Does HC, Borkovich KA, Coleman JJ, Daboussi MJ, Di Pietro A, Dufresne M, Freitag M, Grabherr M, Henrissat B, et al. 2010. Comparative genomics reveals mobile pathogenicity chromosomes in Fusarium. Nature. 464:367–373.
- Morgulis A, Coulouris G, Raytselis Y, Madden TL, Agarwala R, Schäffer AA. 2008. Database indexing for production MegaBLAST searches. Bioinformatics. 24:1757–1764.
- Nelson PE, Toussoun TA, Marasas WFO. 1983. Fusarium species: an illustrated manual for identification. University Park: Pennsylvania StateUniversity Press.
- O’Donnell K, Kistler HC, Cigelnik E, Ploetz RC. 1998. Multiple evolutionary origins of the fungus causing Panama disease of banana: concordant evidence from nuclear and mitochondrial gene genealogies. Proc Natl Acad Sci U S A. 95:2044–2049.
- Parker IM, Gilbert GS. 2004. The evolutionary ecology of novel plant-pathogen interactions. Annu Rev Ecol Evol Syst. 35:675–700.
- Pérez-Hernández A, Serrano-Alonso Y, Aguilar-Pérez ML, Gómez-Uroz R, Gómez-Vázquez J. 2014. Damping-off and root rot of pepper caused by Fusarium oxysporum in Almeria province, Spain. Plant Dis. 98:1159.
- Postic J, Cosic J, Vrandecic K, Jurkovic D, Saleh AA, Leslie JF. 2012. Diversity of Fusarium species isolated from weeds and plant debris in Croatia. J Phytopathol. 160:76–81.
- Raja HA, Miller AN, Pearce CJ, Oberlies NH. 2017. Fungal identification using molecular tools: a primer for the Natural Products Research Community. J Nat Prod. 80(3):756–770.
- Schmitt I, Crespo A, Divakar PK, Fankhauser JD, Herman-Sackett E, Kalb K, Nelsen MP, Nelson NA, Rivas-Plata E, Shimp AD, et al. 2009. New primers for promising single-copy genes in fungal phylogenetics and systematics. Persoonia. 23:35–40.
- Silva JC, Bettiol W. 2005. Potential of non-pathogenic Fusarium oxysporum isolates for control of Fusarium wilt of tomato. Fitopatol Bras. 30:409–412.
- Sneath PHA, Sokal RR. 1973. Numerical taxonomy; the principles and practice of numerical classification. San Francisco: Freeman; p. 573.
- Tamura K, Stecher G, Peterson D, Filipski A, Kumar S. 2013. MEGA6: molecular evolutionary genetics analysis version 6.0. Mol Biol Evol. 30:2725–2729.
- Townsend GR, Heuberger JW. 1943. Methods for estimating losses caused by diseases in fungicides experiments. Plant Dis Rep. 27:340–343.
- [TUIK] Turkey Statistical Institute. 2017. [accessed 2018 Aug 10]. https://biruni.tuik.gov.tr/medas/?locale=tr.
- Velarde-Félix S, Garzón-Tiznado JA, Hernández-Verdugo S, López-Orona CA, Retes-Manjarrez JE. 2018. Occurrence of Fusarium oxysporum causing wilt on pepper in Mexico. Can J Plant Pathol. 40(2):238–247.
- Yücel S, Günaçtı H, Sezen SM. 2013. Salçalık biber yetiştiriciliğinde farklı sulama yöntemlerinin toprak kökenli hastalık çıkışı ve verime etkileri. Derim. 30(2):11–21.
- Zhan J, Yang L, Zhu W, Shang L, Newton AC. 2012. Pathogen populations evolve to greater race complexity in agricultural systems-evidence from analysis of Rhynchosporium secalis virulence data. PLoS One. 7(6):e38611.