Abstract
Cercospora leaf spot (CLS), caused by Cercospora beticola Sacc., is the dominant foliar disease affecting the profitability of table beet production in New York, USA. The disease is controlled primarily with fungicides, but sustainability is critically threatened by strobilurin resistance within the C. beticola population and a limited suite of alternatives. This study evaluated the temporal dynamics of strobilurin resistance within the C. beticola population over three years, and quantified sensitivity to selected demethylation inhibitors (DMIs; propiconazole and difenoconazole) and succinate dehydrogenase inhibitors (SDHIs; fluopyram, penthiopyrad, boscalid and benzovindiflupyr). Resistance to azoxystrobin (EC50 > 0.2 µg mL−1) was prevalent and detected in all fields sampled in 2015 and 2016, and six of 10 fields in 2017. Within fields, the incidence of azoxystrobin-resistant isolates ranged between 4.8% and 70%. No significant differences were detected in the frequency of azoxystrobin-resistant isolates within populations from fields with conventional and organic practices in 2016 and 2017. Reduced sensitivity to propiconazole and difenoconazole was detected in less than 1% of isolates. The EC50 values for the SDHIs were higher than the maximum concentrations (≥ 20 µg mL−1) evaluated. Two small-plot replicated field trials were also conducted over two years to evaluate alternatives to the commercial standards, propiconazole and azoxystrobin. Two applications of benzovindiflupyr + difenoconazole and pydiflumetofen + difenoconazole significantly reduced CLS severity and epidemic progress compared with plots that received propiconazole and non-treated controls.
Résumé
La cerscosporose, causée par Cercospora beticola Sacc., est la principale maladie foliaire qui compromet la rentabilité de la production de la betterave dans l’État de New York, aux États-Unis. La maladie se gère principalement avec des fongicides, mais la viabilité de la méthode est gravement compromise par la résistance de la population de C. beticola aux strobilurines ainsi que par une gamme limitée de solutions de rechange. Cette étude a évalué, pendant trois ans, la dynamique spatio-temporelle de la résistance aux strobilurines au sein de la population de C. beticola et a quantifié la sensibilité à des inhibiteurs choisis de la déméthylation (IDM: propiconazole et difénoconazole) et de la succinate-déshydrogénase (ISDH: fluopyram, penthiopyrade, boscalide et benzovindiflupyr). La résistance à l’azosxystrobine (EC50 > 0.2 µg mL−1) dominait et a été détectée dans tous les champs échantillonnés en 2015 et 2016 et, en 2017, dans 6 des 10 champs. Dans les champs, l’incidence d’isolats résistants à l’azosxystrobine variait de 4.8% à 70%. Aucune différence significative n’a été détectée quant à la fréquence des isolats résistants à l’azosxystrobine dans les populations des champs cultivés traditionnellement ou biologiquement en 2016 et 2017. Une sensibilité réduite au propiconazole et au difénoconazole a été détectée dans moins de 1% des isolats. Les valeurs d’EC50 pour les ISDH étaient plus élevées que les concentrations maximales (≥ 20 µg mL−1) évaluées. Deux essais répétés dans de petites parcelles ont également été menés pendant deux ans afin d’évaluer des solutions de rechange aux normes commerciales, soit au propiconazole et à l’azosxystrobine. Deux applications de benzovindiflupyr + difénoconazole et pydiflumetofen + difénoconazole ont significativement réduit la gravité de la cerscosporose et ralenti la progression de l’épidémie, et ce, comparativement aux parcelles traitées au propiconazole et aux parcelles témoins non traitées.
Introduction
Cercospora leaf spot (CLS), caused by Cercospora beticola Sacc., is the most significant foliar disease affecting table beet (Beta vulgaris L. ssp. vulgaris) production in New York (NY), USA (Jacobsen and Franc Citation2009; Pethybridge et al. Citation2018). The disease causes crop loss by reducing the quality of the foliage making it unsuitable for fresh market sales, and if epidemics occur early, the reduced green leaf area may impair the plant’s growth and ability to achieve adequate root sizes for sale (Pethybridge et al. Citation2018). If CLS is not controlled, defoliation also deleteriously affects mechanized harvest, which relies upon healthy foliage to pull roots from the ground (Pethybridge et al. Citation2017a). In NY, the inability to top-pull harvest may result in complete crop loss as digging is hampered by heavy and rocky mineral soils, and often wet conditions in autumn. CLS is also a substantial constraint worldwide to production of the related crop sugar beet, and causes losses through reduced photosynthetic area deleteriously affecting root growth and sugar content (Smith and Ruppel Citation1973; Smith and Martin Citation1978; Shane and Teng Citation1992; Weiland and Koch Citation2004; Jacobsen and Franc Citation2009; Tedford et al. Citation2019).
Symptoms of CLS on table beet begin as distinctive discrete light brown or tan spots that become grey with age and coalesce rapidly when conditions are conducive to infection and disease development (Koike et al. Citation2010). In red table beet cultivars, which are the most popular for broad-acre production for the processing market, CLS lesions are surrounded by a red halo. Diagnostic for CLS is the presence of signs of C. beticola within lesions including small, black pseudostromata and conidia (McKay and Pool Citation1918; Weiland and Koch Citation2004). The conidia are disseminated by wind and/or rain (Koike et al. Citation2010) and the latent period may range from 5 to 14 days dependent upon cultivar (Pethybridge, unpublished data).
Although there is a broad range of susceptibility to CLS in table beet cultivars (Pethybridge et al. Citation2017b), the ability to mitigate CLS risk through the selection of less susceptible cultivars is limited for broad-acre table beet production considering the processing market’s strict product standards for canning (USDA Citation2017). Cultivars grown for processing into value-added products such as juices and snack packs must also have desirable quality characteristics such as high sugar content and colour stability and these typically are highly susceptible to CLS (Pethybridge et al. Citation2017b).
The rapid spread of CLS from multiple infection cycles within the season results in a polycyclic epidemic (Pool and McKay Citation1916; Pethybridge et al. Citation2017a). The dominant tactic used for CLS management is three to four fungicide applications with the aim of reducing the rate of disease progress (Madden et al. Citation2007; Pethybridge et al. Citation2017a, Citation2018). However, the durability of this approach is critically threatened by strobilurin resistance within the local C. beticola population, reported in 41% of isolates collected from broad-acre table beet fields in 2014 (Vaghefi et al. Citation2016). Strobilurin resistance in the local C. beticola population was associated with G143A and F129L mutations within the cytochrome b gene (Vaghefi et al. Citation2016). This report followed field control failures despite repeated applications of azoxystrobin (Abawi et al. Citation2014). Resistance to strobilurin fungicides has also been reported in C. beticola populations associated with CLS in sugar beet across the USA (Kirk et al. Citation2012; Bolton et al. Citation2012a, Citation2013; Rosenzweig et al. Citation2015) and Ontario, Canada (Trueman et al. Citation2013). Early season disease control programmes also continue to rely upon azoxystrobin applied in-furrow and post-emergence for Rhizoctonia root rot caused by Rhizoctonia solani (Abawi et al. Citation1986; Gugino et al. Citation2008; Pethybridge et al. Citation2018). However, no information is available on the temporal dynamics of strobilurin resistance within the C. beticola population in NY.
The design of durable management programmes for CLS in NY table beet production based on rotation of fungicides with different site-specific modes of action is problematic due to the restricted number of registered products. Currently, azoxystrobin (as Quadris; Syngenta Corporation) and propiconazole (Tilt; Syngenta Corporation) are the only site-specific fungicides registered for CLS control in table beet in NY (Reiners et al. Citation2019). Propiconazole is a demethylation inhibitor that interrupts sterol 14α-demethylase activity and the C14-demethylation step during sterol formation (Köller and Scheinpflug Citation1987). Resistance to DMIs is also prevalent in C. beticola populations from sugar beet across Europe (Karaoglanidis et al. Citation2000, Citation2001, Citation2002, Citation2003; Karaoglanidis and Bardas Citation2006), the USA (Secor et al. Citation2010) and Canada (Trueman et al. Citation2017). Other products, such as triphenyltin hydroxide (Giannopolitis Citation1978; Giannopolitis and Chrysayi-Tokousbalides Citation1980) and benzimidazoles (Georgopoulos and Dovas Citation1973; Percich et al. Citation1986; Campbell et al. Citation1998; Weiland and Halloin Citation2001; Karaoglanidis and Ioannidis Citation2010; Vogel et al. Citation2018) that have been used for CLS management in sugar beet production are not available to table beet growers in NY. Moderate disease control has been reported from selected succinate dehydrogenase inhibitors (SDHIs) alone or in combination with members of other FRAC groups (Pethybridge et al. Citation2017a). SDHI fungicides act on ubiquinone binding sites within the mitochondrial succinate dehydrogenase leading to respiration inhibition (Sierotzki and Scalliet Citation2013) and belong to FRAC group 7 (FRAC Citation2017). A pre-mixed formulation of benzovindiflupyr (FRAC group 7) and difenoconazole (FRAC group 3) (as Aprovia Top; Syngenta Corporation) provided excellent disease control compared with the current commercial standards (Pethybridge et al. Citation2017a). However, neither of the active ingredients within this pre-mixed formulation were tested individually so it is difficult to determine whether the CLS control resulted from one or both. The only multi-site contact fungicides registered for CLS control in table beet in NY are copper-based products (FRAC group M5) which have moderate efficacy and are best used prior to the first appearance of symptoms (Pethybridge et al. Citation2017b, Citation2018). The current commercial recommendation for CLS control is to apply the first fungicide when an average of one CLS lesion per leaf is observed, with subsequent applications repeated based on the reapplication recommendations of specific products from the manufacturer. The presence of only two site-specific FRAC groups registered for CLS control means that fungicide resistance management through rotation of modes of action cannot be achieved.
The objective of this study was to quantify temporal changes in strobilurin resistance and evaluate the in vitro sensitivity to selected DMI (propiconazole) and SDHI fungicides within the C. beticola population in NY. A complementary objective was to evaluate the in-field performance of difenoconazole and selected SDHIs as potential additions to the limited suite of currently available products for CLS control. The status of sensitivity within C. beticola to difenoconazole and selected SDHI fungicides is important baseline information to monitor temporal changes that may result following introduction.
Materials and methods
Isolate collection, culture and storage
Cercospora beticola isolates were obtained from symptomatic table beet leaves collected arbitrarily along linear transects within rows in selected fields in western New York in 2015, 2016 and 2017. Leaves were stored at 4°C for 5–7 days before isolations were conducted. Samples were first rinsed in sterile distilled water and placed in glass Petri dishes in humid boxes at room temperature to promote sporulation. Isolations from plant tissue and single spore transfer were conducted according to the protocol described by Vaghefi et al. (Citation2016), maintained on potato dextrose agar (PDA; Hardy Diagnostics, Santa Maria, CA) plates, and stored at −20°C as colonized mycelial plugs.
In vitro fungicide sensitivity assays
Azoxystrobin. Sensitivity to azoxystrobin was assessed in a conidial germination assay using the methods described by Vaghefi et al. (Citation2016). Sensitivity to azoxystrobin of 452 C. beticola isolates from across NY collected in 2015 (n = 162), 2016 (n = 190) and 2017 (n = 100) was tested. Isolates collected in 2015 were from four table beet fields and within-field population sample sizes ranged between 38 and 42 isolates. Two of the fields were managed using conventional practices and hence for foliar disease management received at least two applications of conventional fungicides. The remainder of the fields were organic and did not receive any fungicides. In 2016, C. beticola populations were collected from three conventional and organic table beet fields (total of six fields), and in-field population sample sizes ranged between 29 and 35. In 2017, C. beticola populations were collected from five conventional and organic table beet fields (total of 10 fields) and 10 isolates were used from each field.
In brief, technical grade azoxystrobin (100 mg; Sigma-Aldrich, St. Louis, MO) was dissolved in warm acetone and made up to 5 mL to make a stock suspension (20 000 µg mL−1) and used to make a dilution series (10−1, 10−2, 10−3 and 10−4) in sterile distilled water resulting in four concentrations. Suitable aliquots from the dilution series were added to 3% (wt:vol) water agar (WA; Hardy Diagnostics) at 60°C, to achieve concentrations of 0.001, 0.01, 0.1, 1, 10, 25 and 100 µg mL−1. Salicylhydroxyamic acid (SHAM; Sigma-Aldrich) was added to all fungicide-amended media to inhibit the alternative respiration pathway at 60 µg mL−1. This concentration was the maximum to suppress the alternative respiration pathway and not affect conidial germination (Vaghefi et al. Citation2016). A SHAM only and non-amended control was used in each assay.
Conidia for azoxystrobin sensitivity assays were generated by the technique described in Vaghefi et al. (Citation2016). In brief, this involved culturing C. beticola isolates on clarified V8 agar (Miller Citation1955; Secor and Rivera Citation2012) for 14 days under 118 volt/60 hz white fluorescent lights with a 12 h photoperiod at 20°C. Plates were flooded with sterile distilled water, and conidia detached from mycelia using a sterile spatula. The resultant conidial suspension was then passed through four layers of sterile muslin cloth and a 50 µl aliquot placed onto 9 mm2 blocks of 3% WA mounted on microscope slides with three replicates for each fungicide concentration, SHAM only, and non-amended control per isolate. Blocks were covered by a glass coverslip and placed in a sealed container in the dark for 24 h at room temperature prior to evaluation of conidial germination at 200 to 400× magnification. Fifty conidia were evaluated from each slide and the number of germinated or non-germinated conidia was recorded. Conidia were considered germinated if any single germ tube was at least half the length of the conidium. The effective concentration required to cause 50% inhibition (EC50) of conidial germination in comparison to the non-amended control was calculated by probit analysis (PROC PROBIT; Statistical Analysis System (SAS) Version 9.4). The effect of SHAM only on conidial germination was quantified by analysis of variance (Genstat Version 18; Hemel Hempstead, UK).
A discriminatory dose associated with azoxystrobin-resistant and -sensitive phenotypes in the NY C. beticola population has been defined (Vaghefi et al. Citation2016). The presence of the G143A mutation was associated with a less sensitive phenotype (EC50 > 0.2 µg mL−1) and classified as resistant. Isolates with a sensitive phenotype (EC50 < 0.1 µg mL−1) did not have the G143A mutation and were classified as sensitive. A low frequency of isolates with an intermediate phenotype (0.1 < EC50 < 0.2 µg mL−1) had the F129L mutation (Vaghefi et al. Citation2016). For the 2016 and 2017 populations, the effect of conventional and organic production practices on the frequency of C. beticola isolates with azoxystrobin-resistant phenotypes was tested using an independent t-test assuming unequal sample variances (Genstat Version 18).
Demethylation inhibitors
Propiconazole. Mycelial growth assays were done to quantify the sensitivity of select C. beticola isolates to propiconazole to establish a discriminatory dose. Twenty-seven C. beticola isolates collected in 2017 (one per field) were used to estimate the effective concentration of technical grade propiconazole (Sigma-Aldrich) to reduce mycelial growth by 50% (EC50). Concentrations of 0.1, 1, 10 and 40 µg mL−1 were prepared within the fungicide-amended media. To evaluate the sensitivity of a broader range of C. beticola populations (n = 252) to propiconazole collected in 2016 (n = 152) and 2017 (n = 100), a discriminatory concentration of 20 µg mL−1 was selected. In this assay, 6 mm mycelial plugs from a 14-day-old culture of each isolate were placed in the centre of Petri dishes amended with the discriminatory dose of propiconazole.
Difenoconazole. Twenty-three of the same isolates from 2017 used for propiconazole sensitivity testing were also evaluated for sensitivity to technical grade difenoconazole (Sigma-Aldrich) using the same concentrations used for propiconazole (0.1, 1, 10 and 40 µg ml−1) within the fungicide-amended media, and a non-amended control, using methods described below. The base media for mycelial growth assays was PDA.
For propiconazole and difenoconazole sensitivity testing, the experimental design was completely randomized and for each isolate, three replicates of fungicide-amended and non-amended media were used. Colony diameter of each isolate was measured in two perpendicular directions and averaged. The per cent of mycelial growth inhibition was calculated for each fungicide as: [1− (colony diameter in fungicide-amended/colony diameter in non-amended control)] × 100. Isolates were insensitive if the per cent inhibition induced by each discriminatory concentration was less than 50% relative to the control, or otherwise sensitive. The association between EC50 values for propiconazole and difenoconazole across isolates was tested using Spearman’s rank correlation coefficient (Spearman Citation1904; Genstat Version 18).
Succinate dehydrogenase inhibitors. The sensitivity of 28 C. beticola isolates (7 from 2014 and 21 from 2016) to selected succinate dehydrogenase inhibitors (SDHIs) was also tested using mycelial growth assays. Technical grade aliquots of each fungicide (Sigma-Aldrich) were first dissolved in acetone. Stock concentrations of fluopyram (6400 µg mL−1), boscalid (6300 µg mL−1), penthiopyrad (2000 µg mL−1) and benzovindiflupyr (5000 µg mL−1) were used to achieve concentrations of 0.05, 0.5 and 5 µg mL−1 for all fungicides and a maximum concentration of 20 µg mL−1 for all fungicides, except fluopyram for which the maximum was 25 µg mL−1. Non-amended media including an equivalent amount of acetone was used as the control.
Mycelial plugs (6 mm diameter) were removed from a 14-day-old culture of each isolate and placed in the centre of Petri dishes containing each of the fungicide concentrations and non-amended controls. The colony diameter of each isolate was measured in two perpendicular directions after 5 days of incubation at 22°C in the dark. The experimental design was completely randomized and included three replicates of each concentration of fungicide and non-amended control. An experimental unit was defined as a Petri dish with a mycelial plug. The effective concentration required to cause 50% inhibition (EC50) of mycelial growth in comparison to the non-amended control was calculated by probit analysis (PROC PROBIT; Statistical Analysis System (SAS) Version 9.4).
Field trials
One small-plot, replicated trial was conducted in each cropping season of 2017 and 2018 at the Research North facility of Cornell AgriTech at The New York State Agricultural Experiment Station, Geneva, New York. Both trials were established by planting seed of the table beet cultivar Ruby Queen (Seneca Foods, Dayton, WA) which is the commercial standard used for processing crops in NY. The crops were planted on 9 June 2017 and 18 May 2018 with a precision Monosem planter (Monosem, Lenexa, KS) at an in-row plant density of 54 seeds m−1 and 0.76 m between rows. Sufficient nutrients for optimal plant growth was ensured by incorporating 392 kg ha−1 two days prior to planting by broadcast application followed by incorporation with a Coulter mulcher and 337 kg ha−1 in a band at planting. On both occasions, the fertilizer was 10 N: 10 P: 10 K + 2.2 kg ha−1 Boron (Phelps Supply Inc., Phelps, NY). Weeds were managed using the herbicides S-metolachlor (as Dual Magnum (0.78 L ha−1); Syngenta Corporation) + ethofumesate (as Nortron (2.2 L ha−1); Bayer CropScience) within 24 h of planting as a pre-emergence application. A post-emergence application of the herbicides, clopyralid (as Stinger (280.2 g ha−1); Dow AgroSciences) and phenmedipham (as Spin-Aid (1.1 L ha−1); Bayer CropScience) was applied 27–29 days after planting (DAP) when table beet plants had reached two to four true leaves. Trials received supplementary irrigation at 7- to 10-day intervals using overhead sprinklers for optimal plant growth and disease development.
Each plot was inoculated with a suspension of eight C. beticola isolates representative of the genotypes within the NY population in 2014 and used in other trials for comparative purposes (Tb14-047, Tb14-070, Tb14-081, Tb14-113, Tb14-116, Tb14-118, Tb14-146 and Tb14-154; Vaghefi et al. Citation2016) at 41 and 50 DAP (2017) or54 and 68 DAP (2018). Inoculum suspensions were prepared from liquid clarified V8 (CV8) juice broth (Pethybridge et al. Citation2017a). The concentration of the inoculum varied between 2.3 × 103 colony forming units (cfu) mL−1 and 1.2 × 105 cfu mL−1 (+ 0.01% (v/v) polysorbate-20) with a backpack sprayer at 130 L ha−1. Colony forming units were estimated by making serial dilutions of the final inoculum suspension to 10−5 in sterile distilled water and spreading 50 µL aliquots onto WA plates. Three to four replicate plates were used for each concentration and incubated at room temperatures under white fluorescent light with a 12 h photoperiod. The number of colonies on each plate was counted after four days and averaged between replicates within concentrations.
In the 2017 trial, treatments were Aprovia Top (950 mL ha−1; benzovindiflupyr + difenoconazole), Inspire (365.4 mL ha−1; difenoconazole only), Aprovia (511.5 mL ha−1; benzovindiflupyr only) and Tilt (292.3 mL ha−1; propiconazole conventional standard). Each fungicide was applied either one, two or three times at regular intervals (49, 65 and 72 DAP, respectively). In the 2018 trial, treatments were Aprovia Top (950 mL ha−1; benzovindiflupyr + difenoconazole), Inspire (365.4 mL ha−1; difenoconazole only), Miravis Top (1001.2 mL ha−1; pydiflumetofen + difenoconazole) and Tilt (292.3 mL ha−1; propiconazole conventional standard). Each fungicide was also applied either once, twice or three times (53, 69 and 83 DAP).
Treatments were arranged in a randomized complete block design with four replications, including a non-treated control. Individual plots were 3.1 m sections of two adjacent rows, with a non-treated buffer of 1.5 m between plots within rows. One non-treated and non-inoculated row separated adjacent plots. Fungicides were applied using a CO2-pressurized backpack sprayer with output volumes of 193 and 233 L ha−1 in 2017 and 2018, respectively. The sprayer was fitted with three TJ 8002VS flat fan nozzles spaced 48.2 cm apart along a 96.5 cm long boom.
The homogeneity of the crop stand was evaluated by counting in-row plant populations in each of two arbitrarily placed 0.5 m sections (one per row in each plot) following full emergence and prior to treatment application at 29 DAP (2017) and 39 DAP (2018). Disease severity was quantified by counting lesions on each of 20 arbitrarily selected leaves within each plot (10 per row) at regular intervals (43, 52, 55, 63, 69 and 76 DAP in 2017; and 51, 75, 83, 89 and 93 DAP in 2018). These data was used to calculate epidemic progress in each plot (area under the disease progress stairs; AUDPS; Simko and Piepho Citation2012).
Canopy reflectance in the near infrared range (830 nm) was measured using a hand-held multispectral radiometer (MSR5; CropScan Inc., Rochester, MN) at 77 and 93 DAP in 2017 and 2018, respectively. Canopy reflectance at 830 nm has been demonstrated to be a good non-invasive and objective measurement of green leaf area and hence plant health, inversely correlated with disease intensity (Nilsson Citation1995; Pethybridge et al. Citation2017a). Measurements were taken between 1200 and 1400 h (Eastern Time) when incoming radiation was at least 400 W m−2. The radiometer was placed within the middle of each plot 2 m above the soil surface. Canopy reflectance was calculated as a percentage of the voltage for the reflected radiation divided by the voltage for the incident radiation. Two readings were averaged from each plot.
At harvest (80 and 94 DAP in 2017 and 2018, respectively), the efficacy of fungicides was assessed on above and belowground yield components. Foliar biomass was quantified by clipping foliage from the roots within a single 1 m section of each plot and recording weight after drying at 60ºC for 24 h. Roots were dug by hand and the number of roots within the 1 m section was counted and fresh weight recorded. The shoulder diameter of 20 arbitrarily selected roots from each plot was also measured using digital calipers (Mitutoyo 500-196-30; Mitutoyo USA, Aurora, IL) and averaged per plot.
The effect of fungicides on disease severity measured as the average number of CLS lesions per leaf at the final evaluation and AUDPS, canopy reflectance at 830 nm, and yield components (root number at harvest, dry weight of foliage, fresh root weight and average root shoulder diameter) was analysed using a generalized linear model. Data from each trial were analysed separately due to differences in treatments. Treatment and replication were evaluated as fixed and random effects, respectively. Means were separated by a Fisher’s protected least significant difference test at P = 0.05. Linear regression analysis quantified the relationships between table beet yield components within trials (Genstat Version 18).
Results
In vitro azoxystrobin sensitivity
In 2015, 3 of 42 (7.1%), 2 of 42 (7.1%), 24 of 40 (60%) and 14 of 38 (36.8%) C. beticola isolates within each of the table beet fields were classified as resistant (EC50 > 0.2 µg azoxystrobin mL−1 (Fig. 1a). In 2016, the frequency of azoxystrobin-resistant isolates obtained from conventional fields was 12 of 30 (40%), 16 of 35 (45.8%) and 13 of 32 (40.6%); and in organic fields was 21 of 30 (70%), 22 of 34 (64.7%) and 2 of 29 (6.9%) (). In 2017, in one of the five conventional fields, all C. beticola isolates were sensitive to azoxystrobin but in the remaining four fields, the frequency of resistant isolates ranged between five and six of 10 isolates (). In three of the organic production fields, all isolates were sensitive to azoxystrobin, but in the remaining two, the frequency of resistant isolates was 1 and 6 of 10 isolates, respectively. In both of the latter years, there was no significant difference (P = 0.785 in 2016; P = 0.101 in 2017) in the proportion of azoxystrobin-resistant isolates from table beet fields grown using organic and conventional production practices.
In vitro propiconazole and difenoconazole sensitivity
For the sub-set of C. beticola isolates used to establish a propiconazole discriminatory dose, EC50 values ranged between 5.29 to 19.43 µg mL−1. For two isolates collected in 2016 from broad-acre, conventional fields, the EC50 value was greater than the highest concentration tested (40 µg propiconazole mL−1). Using a discriminatory dose of 20 µg propiconazole mL−1 to evaluate the populations collected in 2016 and 2017, all additional isolates tested were classified as sensitive. EC50 values for difenoconazole for 21 of the 23 isolates collected in 2016 were less than the lowest concentration tested (0.1 µg mL−1) and for the remaining two isolates was greater than the highest concentration tested (40 µg mL−1). The two isolates that were less sensitive to propiconazole were also less sensitive to difenoconazole. There was a significant association between the EC50 values calculated for difenoconazole and propiconazole across isolates (ρ = 0.645; P < 0.001).
Succinate dehydrogenase inhibitor sensitivity
The EC50 values of all C. beticola isolates tested against the SDHI fungicides were above the maximum concentrations tested (20 µg mL−1 for boscalid, penthiopyrad and benzovindiflupyr; and 25 µg mL−1 for fluopyram).
Field trials – 2017
No significant differences (P = 0.559) in plant density were detected between plots prior to application of treatments. Fungicides and application frequency had a significant effect on disease severity and AUDPS (; Fig. 2). Benzovindiflupyr, difenoconazole, propiconazole and benzovindiflupyr + difenoconazole significantly reduced CLS severity and epidemic progress compared with the non-treated control. Benzovindiflupyr + difenoconazole provided the best control and there was no significant benefit in disease control from repeating the application two or three times compared with a single application. Benzovindiflupyr only provided moderate disease control irrespective of application number, and AUDPS values were significantly higher than in plots receiving difenoconazole only. Two or three applications of difenoconazole provided disease control that was not significantly different than plots receiving benzovindiflupyr + difenoconazole. Propiconazole provided moderate disease control and AUDPS was significantly reduced by 21–27.6% from two applications compared with a single application, but was not significantly improved by a third application (; ). The effects of fungicides on disease control were also observed in canopy reflectance at 830 nm at 77 DAP, with the exception of no significant differences between benzovindiflupyr applications, a single propiconazole application, and non-treated plots. There was also a significant 16.1–25.6% increase in canopy reflectance at 830 nm in plots receiving repeated applications of benzovindiflupyr + difenoconazole compared with a single application (). The improvements in CLS control from fungicides were also reflected in effects on the dry weight of foliage (). For example, a single and three applications of benzovindiflupyr + difenoconazole increased the dry weight of foliage compared with non-treated plots by 168.8% and 265%, respectively. Propiconazole also significantly increased the dry weight of foliage compared with non-treated plots by an average of 120.3% and no further benefit was gained from increasing application number. Treatment had no significant effect on the number of roots per metre and average root shoulder diameter, however several treatments that resulted in a significant increase in the dry weight of foliage also increased the fresh weight of roots (; Fig. S1).
Table 1. Effect of fungicides (benzovindiflupyr, difenoconazole, benzovindiflupyr + difenoconazole, and propiconazole [conventional fungicide standard]) and frequency of applications on Cercospora leaf spot (CLS) disease severity and epidemic progress, canopy reflectance at 830 nm, and table beet yield components in a small-plot, replicated trial at Geneva, New York in 2017.
Fig. 2 Temporal changes in the average number of cercospora leaf spot (CLS) lesions per leaf from 43 to 76 days after planting (DAP) in plots receiving up to three applications (number of applications in parentheses) of benzovindiflupyr (a), benzovindiflupyr + difenoconazole (b), difenoconazole (c), and propiconazole (d) in a small-plot, replicated trial conducted at Geneva, New York in 2017.
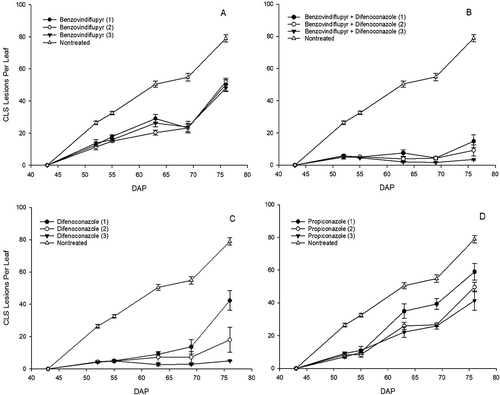
Field trials – 2018
No significant differences (P = 0.5) in plant density were detected between plots prior to treatments. Fungicide and application frequency had a significant effect on CLS severity and epidemic progress (; Fig. 3). When receiving only one application, pydiflumetofen + difenoconazole was the most efficacious product and reduced the number of CLS lesions per leaf and AUDPS by 73.6% and 77.4%, respectively. Additional significant reductions in CLS lesions per leaf and AUDPS resulted from a second application of pydiflumetofen + difenoconazole but no further reduction in disease severity resulted from a third application, resulting in an average reduction of CLS lesions per leaf of 96.4%. For difenoconazole, three applications provided disease control that was not significantly different from two applications of pydiflumetofen + difenoconazole. Although one application of benzovindiflupyr + difenoconazole resulted in a significantly higher number of CLS lesions per leaf and AUDPS compared with the equivalent of pydiflumetofen +difenoconazole, repeated applications resulted in significantly less disease severity that was not significantly different from two applications of pydiflumetofen + difenoconazole. Moreover, there was no benefit in disease control from a third application of benzovindiflupyr + difenoconazole compared with two. For propiconazole (the conventional fungicide standard), one application provided relatively poor disease control and reduced the number of CLS lesions per leaf only by 15.5% compared with non-treated plots. Significant reductions in the number of CLS lesions per leaf and AUDPS were obtained from another application of propiconazole but no additional benefit resulted from a third application (; ). Canopy reflectance at 830 nm also showed the improvements in disease control obtained from fungicide application and hence differences in green leaf area. For example, canopy reflectance at 830 nm was significantly increased by 59.8% in plots receiving two applications of pydiflumetofen + difenoconazole compared with non-treated plots, and by 15.9% over plots receiving only one application (). All treatments significantly increased the dry weight of foliage compared with non-treated plots. However, increasing application frequency did not increase the dry weight of foliage for any of the fungicides. Treatment had no significant effect on the number of roots, fresh weight of roots, and average root shoulder diameter ().
Table 2. Effect of fungicides (benzovindiflupyr + difenoconazole, difenoconazole, pydiflumetofen + difenoconazole, and propiconazole) and the number of applications on cercospora leaf spot (CLS) disease severity and epidemic progress, canopy reflectance at 830 nm, and table beet yield components in a small-plot, replicated trial at Geneva, New York in 2018.
Fig. 3 Temporal changes in the average number of cercospora leaf spot (CLS) lesions per leaf from 51 to 93 days after planting (DAP) in plots receiving up to three applications (number of applications in parentheses) of benzovindiflupyr + difenoconazole (a), pydiflumetofen + difenoconazole (b), difenoconazole (c), and propiconazole (d) in a small-plot, replicated trial conducted at Geneva, New York in 2018.
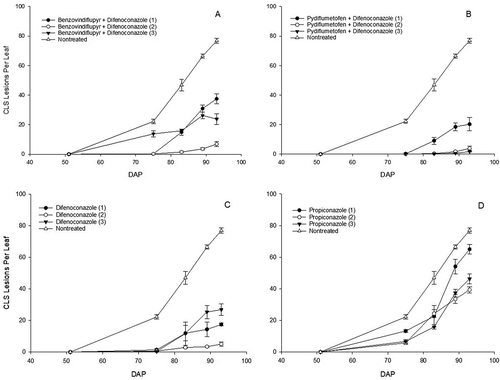
Discussion
The high prevalence and incidence of azoxystrobin-resistant C. beticola isolates associated with CLS on table beet in NY found in each of three years is suggestive of persistence of this trait in the population or the annual introduction of isolates with this phenotype. Evidence supporting the relative contributions of a broad range of potential primary inoculum sources for CLS epidemics in table beet is mixed. For example, Knight et al. (Citation2018) did not find evidence for shared multilocus genotypes in C. beticola populations associated with CLS epidemics in three fields planted with table beet between seasons in successive years. The most likely dominant inoculum sources were external to the field of interest and may include infested seed and an unknown sexual morph producing spores able to be disseminated long distances between fields (Knight et al. Citation2018). In contrast, alternative hosts that could be responsible for local inoculum sources while acting as green bridges between seasons have also been documented (Knight et al. Citation2019a). Cercospora beticola isolates from NY are pathogenic to several weed species, including Brassica kaber, Chenopodium album, Carthamus tinctorius, Rumex obtusifolius and Spinacia oleacera but surveys of CLS-like lesions on weeds in table beet fields have found C. beticola only at low frequencies. For example, of 71 CLS-like lesions observed on field populations of Ch. album, 51 were associated with C. chenopodii and only two with C. beticola. However, this study also found C. beticola could sporulate on necrotic and senescent tissue, which suggests saprophytic survival on non-hosts may also contribute to the perpetuation of inoculum between seasons (Knight et al. Citation2019a) and hence contribute to the perpetuation of specific phenotypes within the population, such as azoxystrobin resistance.
The high frequency of azoxystrobin-resistant isolates in C. beticola populations also highlights the critical need to reduce the reliance of disease management programmes on strobilurins. Azoxystrobin is currently the only registered product for early-season and root disease control primarily targeting Rhizoctonia root rot (Abawi et al. Citation1986; Gugino et al. Citation2008; Pethybridge et al. Citation2018), so there is little potential to eliminate its use from table beet production to prevent continued exposure to both R. solani and C. beticola populations. The lack of alternatives to strobilurins for CLS control means that growers have little choice and their use persists in rotation with propiconazole, even in the wake of this knowledge.
In these trials, propiconazole provided only moderate CLS control. Moreover, a tendency to apply propiconazole several times within a season because of the lack of alternatives imposes additional selection pressure on a pathogen population that is highly genotypically diverse (Vaghefi et al. Citation2016, Citation2017a, Citation2017b; Knight et al. Citation2019b) and at a high risk of resistance development to additional single site mode of action fungicides. Resistance to DMIs is prevalent in C. beticola populations affecting sugar beet (e.g. Karaoglanidis et al. Citation2000, Citation2001, Citation2002, Citation2003; Bolton et al. Citation2012b). In this study, the low frequency (0.7%) of C. beticola isolates with reduced sensitivity to propiconazole and difenoconazole is encouraging because the majority of isolates are still highly sensitive to FRAC group 3. This information is an important reference point for the evaluation of temporal changes in DMI sensitivity in the future. If this phenotype becomes more prevalent, further investigation into the molecular basis of resistance within the local C. beticola population affecting table beet will become important, and a basis for the development of assays for detection and monitoring. Point mutations within the CYP51A1 gene have been associated with resistance to another DMI, tetraconazole, within C. beticola populations affecting sugar beet in the USA (Bolton et al. Citation2012b), and have led to reduced binding affinity in other plant-pathogenic fungi (Asai et al. Citation1999; Stammler et al. Citation2009). Overexpression of ABC and MFS transporters (Hayashi et al. Citation2002; Hulvey et al. Citation2012), and overexpression of the CYP51A1 gene leading to increased copy number (Hamamoto et al. Citation2000; Schnabel and Jones Citation2001; Villani et al. Citation2016) have also been reported as the molecular basis of DMI resistance.
The superior CLS control in each of the small-plot, replicated trials from benzovindiflupyr + difenoconazole, and pydiflumetofen + difenoconazole in 2018 suggests the potential for improved in-field performance of fungicides for foliar disease control compared with propiconazole and azoxystrobin. However, registration of either of these products for CLS control on table beet still does not offer the ability to improve the best management practices for strobilurin resistance management by rotation to other site-specific modes of action due to the pre-mixed formulations both containing another FRAC group 3 (difenoconazole) (Reddy Citation2012). In both trials, difenoconazole provided improved disease control compared with propiconazole irrespective of the number of applications. The range of EC50 values for difenoconazole was also several magnitudes lower than for propiconazole which suggests the improvements in CLS control may be caused by a high toxicity which resulted in differential sensitivity to the DMIs within the C. beticola population. Differential sensitivity to the DMIs has also been reported in C. beticola isolates on sugar beet in Ontario, Canada (Trueman et al. Citation2017). In the 2017 trial, difenoconazole significantly reduced CLS severity compared with the equivalent number of benzovindiflupyr applications when applied two or three times, but when applied in the pre-mixed formulation (benzovindiflupyr + difenoconazole) significantly greater CLS control was realized. In the 2018 trial, the two pre-mixed formulations were included because pydiflumetofen + difenoconazole was more likely than benzovindiflupyr + difenoconazole to be registered for use in speciality crops, and registration of difenoconazole as a sole active ingredient (as Inspire) appeared unlikely. The significant reduction in CLS severity from pydiflumetofen + difenoconazole compared with propiconazole remains encouraging as an alternative to the conventional standard. For pydiflumetofen + difenoconazole, two applications provided significant reduction in CLS severity compared with a single application, but there was no significant benefit of a third. Two applications of pydiflumetofen + difenoconazole significantly reduced CLS severity by 94.9% and increased the dry weight of the foliage by 165.7% at 93 and 94 DAP, compared with non-treated control plots. For difenoconazole only, there were significant reductions in CLS severity with each additional application.
The moderate disease control obtained when benzovindiflupyr was applied alone in the 2017 field trial is similar to discouraging findings towards insensitivity of the selected SDHIs, including benzovindiflupyr, within the in vitro assays. SDHIs act on ubiquinone binding sites within the mitochondrial succinate dehydrogenase and lead to respiration inhibition (Kuhn Citation1984; Sierotzki and Scalliet Citation2013). The EC50 values for each of these SDHIs was estimated to be above the highest concentrations tested for fluopyram (25 µg mL−1), and boscalid, benzovindiflupyr and penthiopyrad (all 20 µg mL−1). Resistance to this fungicide group in other plant-pathogenic fungi is relatively complex and diverse, including amino acid exchanges within the SdhB, SdhC or SdhD proteins (e.g. Avenot et al. Citation2008, Citation2012; Avenot and Michailides Citation2010; Mallik et al. Citation2014). Relatively poor CLS control in sugar beet has been reported with penthiopyrad in Canada (Trueman Citation2014) and in an earlier trial on table beet (Pethybridge et al. Citation2017a). Further research is needed to investigate whether EC50 value estimation in C. beticola is sensitive to assay factors such as the selection of base medium. Significant differences in SDHI sensitivity have been noted within Monilinia fructicola isolates when mycelial growth was evaluated on media of varying nutrient status (Hu et al. Citation2011). Differences in SDHI sensitivity estimation within isolates have also been described between mycelial growth and conidial germination assays (Schnabel et al. Citation2010).
Given the recalcitrant nature of azoxystrobin resistance within the NY C. beticola population, care should be taken to reduce the selection pressure from other single site mode of action FRAC groups which threatens the sustainability of a fungicide-based management strategy for CLS in table beet. Further research should evaluate the potential to schedule fungicide applications in periods of highest risk. In the CLS-sugar beet pathosystem, fungicides are applied based on risk predictions from a forecasting model that integrates the effects of temperature and relative humidity (Windels et al. Citation1998; Khan et al. Citation2007; Kaiser et al. Citation2010). A forecasting system for CLS has not been optimized for table beet production in the north-eastern USA, and hence prophylactic applications are made in practice because growers are risk averse as a direct function of the high crop losses and absence of information on cost-effective action thresholds. Informed fungicide timing and initiation, together with improved foundational knowledge about the dominant inoculum sources will enhance the sustainability of the current fungicide-based management for CLS.
Supplemental Material
Download MS Word (85.4 KB)Acknowledgements
We thank Carol Bowden, Audrey Klein, Sean Murphy, Nicolette Nault and Alex Silva (listed alphabetically by surname) for excellent technical support.
Supplementary material
Supplemental data for this article can be accessed online here: https://doi.org/10.1080/07060661.2019.1690048.
Additional information
Funding
References
- Abawi GS, Crosier DC, Cobb AC, Becker RF. 1986. Root rot of table beets in New York State. N.Y. Food Life Sci. Bull. 115. Geneva (NY): New York State Agricultural Experiment Station. 8 p.
- Abawi GS, Kikkert JR, Moktan K, Lange HW, Smart CD. 2014. First report of resistance to Quadris among populations of Cercospora beticola causing leaf spot of table beet in New York State. (Abstr) Phytopathology. 104:S1.1.
- Asai K, Tsuchimori N, Okonongi K, Perfect JR, Gotoh O, Yoshida Y. 1999. Formation of azole-resistant Candida albicans by mutation of sterol 14-demethylase P450. Antimicrob Agents Chemother. 43:1163–1169.
- Avenot HF, Michailides TJ. 2010. Progress in understanding molecular mechanisms and evolution of resistance to succinate dehydrogenase inhibiting (SDHI) fungicides in phytopathogenic fungi. Crop Prot. 29:643–651.
- Avenot HF, Sellam A, Karaoglanidis G, Michailides TJ. 2008. Characterization of mutations in the iron sulfur subunit of succinate dehydrogenase correlating with boscalid resistance in Alternaria alternata from California pistachio. Phytopathology. 98:736–742.
- Avenot HF, Thomas A, Gitaitis RD, Langston DB, Stevenson K. 2012. Molecular characterization of boscalid- and penthiopyrad-resistant isolates of Didymella bryoniae and assessment of their sensitivity to fluopyram. Pest Manag Sci. 68:645–651.
- Bolton MD, Birla K, Rivera-Varas V, Rudolph KD, Secor GA. 2012b. Characterization of CbCyp51 from field isolates of Cercospora beticola. Phytopathology. 102:298–305.
- Bolton MD, Rivera V, Secor G. 2012a. Identification of the G143A mutation associated with QoI resistance in Cercospora beticola field isolates from Michigan, United States. Pest Manage Sci. 69:35–39.
- Bolton MD, Rivera-Varas V, Secor GA, Cattanach A, Metzger M. 2013. Identification of the G143A mutation in cytochrome b associated with QoI resistance in Cercospora beticola isolates from the red river valley. Plant Health Prog. 14:1. doi:10.1094/PHP-2013-0812-02-RS.
- Campbell LG, Smith GA, Lamey HA, Cattach AW. 1998. Cercospora beticola tolerant to triphenyltin hydroxide and resistant to thiophanate-methyl in North Dakota and Minnesota. J Sugar Beet Res. 35:29–41.
- Fungicide Resistance Action Committee. 2017. List of plant pathogenic organisms resistant to disease control agents. [accessed 2018 Sep 15]. http://www.frac.info/what-s-new/2017/03/03/publication-of-the-frac-code-list-2017.
- Georgopoulos SG, Dovas C. 1973. Occurrence of Cercospora beticola strains resistant to benzimidazole fungicides in Northern Greece. Plant Dis Rep. 57:321–324.
- Giannopolitis CN. 1978. Occurrence of strains of Cercospora beticola resistant to triphenyltin fungicides in Greece. Plant Dis Rep. 62:205–208.
- Giannopolitis CN, Chrysayi-Tokousbalides M. 1980. Biology of triphenyltin-resistant strains of Cercospora beticola from sugar beet. Plant Dis. 64:940–942.
- Gugino BK, Ludwig JW, Abawi GS. 2008. Evaluation of fungicides for the control of pocket rot and leaf spot of table beet, 2007. Plant Dis Manag Rep. 2:V156.
- Hamamoto H, Hasegawa K, Nakaune R, Young JL, Makizumi Y, Akutsu K, Hibi T. 2000. Tandem repeat of a transcriptional enhancer upstream of the sterol 14α-demethylase gene (CYP51) in Penicillium digitatum. Appl Environ Microbiol. 66:3421–3426.
- Hayashi K, Schoonbeek HJ, De Waard MA. 2002. Expression of the ABC transporter BcatrD from Botrytis cinerea reduces sensitivity to sterol demethylation inhibitor fungicides. Pestic Biochem Physiol. 73:110–121.
- Hu M-J, Luo C-X, Grabke A, Schnabel G. 2011. Selection of a suitable medium to determine sensitivity of Monilinia fructicola mycelium to SDHI fungicides. J Phytopathol. 159:616–620.
- Hulvey J, Popko JT, Sang H, Berg A, Jung G. 2012. Overexpression of ShCPY51B and ShatrD in Sclerotinia homoeocarpa isolates exhibiting practical field resistance to a demethylation inhibitor fungicide. Appl Environ Microbiol. 78:6674–6682.
- Jacobsen BJ, Franc GD. 2009. Cercospora leaf spot. In: Harveson RM, Hanson LE, Hein GL, editors. Compendium of beet diseases and pests. St. Paul (MN): APS Press; p. 7–10.
- Kaiser U, Kluth C, Märländer B. 2010. Variety-specific epidemiology of Cercospora beticola Sacc. And consequences for threshold-based timing of fungicide application in sugar beet. J Phytopathol. 158:296–306.
- Karaoglanidis GS, Bardas G. 2006. Control of benzimidazole- and DMI-resistant strains of Cercospora beticola with strobilurin fungicides. Plant Dis. 90:419–424.
- Karaoglanidis GS, Ioannidis PM. 2010. Fungicide resistance of Cercospora beticola in Europe. In: Lartey RT, Leiland JW, Panella L, Crous PW, Windels CE, editors. Cercospora leaf spot of sugar beet and related species. MN (USA): APS Press; p. 189–211.
- Karaoglanidis GS, Ioannidis PM, Thanassoulopoulos CC. 2000. Reduced sensitivity of Cercospora beticola isolates to sterol-demethylation-inhibiting fungicides. Plant Pathol. 49:567–572.
- Karaoglanidis GS, Ioannidis PM, Thanassoulopoulos CC. 2001. Influence of fungicide spray schedules on the sensitivity to the sterol demethylation-inhibiting fungicides in Cercospora beticola. Crop Prot. 20:941–947.
- Karaoglanidis GS, Karadimos DA, Ioannidis PM. 2003. Sensitivity of Cercospora beticola populations to fentin-acetate, benomyl and flutriafol in Greece. Crop Prot. 22:735–740.
- Karaoglanidis GS, Konnidis PM, Thanassoulopoulos CC. 2002. Changes in sensitivity of Cercospora beticola populations to sterol-demethylation-inhibiting fungicides during a 4-year period in northern Greece. Plant Pathol. 51:55–62.
- Khan J, Del Rio LE, Nelson R, Khan MFR. 2007. Improving the Cercospora leaf spot management model for sugar beet in Minnesota and North Dakota. Plant Dis. 91:1105–1108.
- Kirk WW, Hanson LE, Franc GD, Stump WL, Gachango E, Clark G, Stewart J. 2012. First report of strobilurin resistance in Cercospora beticola in sugar beet (Beta vulgaris) in Michigan and Nebraska, USA. New Dis Rep. 26:3. doi:10.5197/j.2044-0588.2012.026.003.
- Knight NL, Vaghefi N, Hansen ZR, Kikkert JR, Pethybridge SJ. 2018. Temporal differentiation and shifts in genetic structure of Cercospora beticola populations in table beet fields of New York. Plant Dis. 102:2074–2082.
- Knight NL, Vaghefi N, Kikkert JR, Bolton MD, Secor GA, Hanson LE, Nelson SC, Pethybridge SJ. 2019b. Genetic diversity and structure in regional Cercospora beticola populations from Beta vulgaris ssp. vulgaris suggest two clusters of separate origin. Phytopathology. 109:1280–1292.
- Knight NL, Vaghefi N, Kikkert JR, Pethybridge SJ. 2019a. Alternative hosts of Cercospora beticola in field surveys and inoculation trials. Plant Dis. 103:1983–1990.
- Koike ST, Du Toit LJ, Abawi GS. 2010. Cercospora leaf spot of vegetable crops in the Chenopodiaceae. In: Lartey RT, Weiland JJ, Panella L, Crous PW, Windels CE, editors. Cercospora leaf spot of sugar beet and related species. St. Paul (MN): American Phytopathological Society; p. 91–96.
- Köller W, Scheinpflug H. 1987. Fungal resistance to sterol biosynthesis inhibitors: a new challenge. Plant Dis. 71:1066–1074.
- Kuhn PJ. 1984. Mode of action of carboxamides. Symp Ser Br Mycol Soc. 9:155–183.
- Madden LV, Hughes G, Van den Bosch F. 2007. The study of plant disease epidemics. St. Paul (MN): APS Press.
- Mallik I, Arabiat S, Pasche JS, Bolton MD, Patel JS, Gudmestad NC. 2014. Molecular characterization and detection of mutations associated with resistance to succinate dehydrogenase-inhibiting fungicides in Alternaria solani. Phytopathology. 104:40–49.
- McKay MB, Pool VW. 1918. Field studies of Cercospora beticola. Phytopathology. 8:119–136.
- Miller PM. 1955. V-8 juice agar as a general purpose medium for fungi and bacteria. Phytopathology. 45:461–462.
- Nilsson H. 1995. Remote sensing and image analysis in plant pathology. Can J Plant Pathol. 17:154–166.
- Percich JA, Hotchkiss MW, Nickelson LJ. 1986. Survey and screening of benomyl-resistant strains of Cercospora beticola in Minnesota and North Dakota. J Sugar Beet Res. 23:148–153.
- Pethybridge SJ, Kikkert JR, Hanson L, Nelson SC. 2018. Challenges and prospects for building resilient disease management strategies and tactics for the New York table beet industry. Agronomy. 8:112.
- Pethybridge SJ, Kikkert JR, Vaghefi NV. 2017b. Horticultural characteristics and susceptibility of table beet cultivars to Cercospora leaf spot in New York. Hort Technol. 27:539–543.
- Pethybridge SJ, Vaghefi N, Kikkert JR. 2017a. Management of Cercospora leaf spot in conventional and organic table beet production. Plant Dis. 101:1642–1651.
- Pool VW, McKay MB. 1916. Climatic conditions as related to Cercospora beticola. J Agric Res. 6:21–60.
- Reddy PP. 2012. Recent Advances in Crop Protection. New Delhi (India): Springer. Chapter 12, Strobilurin fungicides; p. 185–200.
- Reiners S, Curtis P, Helms M, McGrath M, Nault B, Seaman A. 2019. Cornell integrated crop and pest management guidelines for commercial vegetable production. Ithaca (NY): Cornell Cooperative Extension. Chapter 14, Beets; p. 113–120.
- Rosenzweig N, Hanson LE, Clark G, Franc GD, Stump WL, Jiang QW, Stewart J, Kirk WW. 2015. Use of PCR-RFLP analysis to monitor fungicide resistance in Cercospora beticola populations from sugar beet (Beta vulgaris) in Michigan, United States. Plant Dis. 99:355–362.
- Schnabel G, Amiri A, Brannen P. 2010. Sustainable brown rot management of peaches in the southeastern United States. Outlooks Pest Manage. 21:208–211.
- Schnabel G, Jones AL. 2001. The 14α-demethylase (CYP51A1) gene is overexpressed in Venturia inaequalis strains resistant to myclobutanil. Phytopathology. 91:102–110.
- Secor GA, Rivera VV. 2012. Fungicide resistance assays for fungal plant pathogens. In: Bolton MD, Thomma BPHJ, editors. Plant fungal pathogens: methods and protocols. NY USA: Humana Press; p. 385–392.
- Secor GA, Rivera VV, Khan MFR, Gudmestad NC. 2010. Monitoring fungicide sensitivity of Cercopsora beticola of sugar beet for disease management decisions. Plant Dis. 94:1272–1282.
- Shane WW, Teng PS. 1992. Impact of Cercospora leaf spot on root weight, sugar yield and purity of Beta vulgaris. Plant Dis. 76:812–820.
- Sierotzki H, Scalliet G. 2013. A review of current knowledge of resistance aspects for the next-generation succinate dehydrogenase inhibitor fungicides. Phytopathology. 103:880–887.
- Simko I, Piepho HP. 2012. The area under the disease progress stairs: calculation, advantage, and application. Phytopathology. 102:381–389.
- Smith GA, Martin SS. 1978. Differential response of sugar beet cultivars to Cercospora leaf spot. Crop Sci. 18:39–42.
- Smith GA, Ruppel EG. 1973. Association of Cercospora leaf spot, gross sugar, percentage sucrose, and root weight in Sugarbeet. Can J Plant Sci. 53:695–696.
- Spearman C. 1904. The proof and measurement of association between two things. Amer J Psychol. 15:72–101.
- Stammler G, Cordero J, Koch A, Semar M, Schlehuber S. 2009. Role of the Y134F mutation in cyp51 and overexpression of cyp51 in the sensitivity response of Puccinia triticina to epoxiconazole. Crop Prot. 28:891–897.
- Tedford SL, Burlakoti RR, Schaafsma AW, Trueman CL. 2019. Optimizing management of cercospora leaf spot (Cercospora beticola) of sugarbeet in the wake of fungicide resistance. Can J Plant Pathol. 41:35–46.
- Trueman CL. 2014. Evaluation of fungicides for management of Cercospora leaf spot in sugar beet, 2013. Plant Dis Manage Rep. 8:FC172.
- Trueman CL, Hanson LE, Rosenzweig N, Jiang QW, Kirk WW. 2013. First report of QoI insensitive Cercospora beticola on sugar beet in Ontario, Canada. Plant Dis. 97:1255.
- Trueman CL, Hanson LE, Somohano P, Rosenzweig N. 2017. First report of DMI-insensitive Cercospora beticola on sugar beet in Ontario, Canada. New Dis Rep. 36:20.
- United States Department of Agriculture – Agricultural Marketing Service. 2017. United States standards for grades of Canned Beets. [ accessed 2017 Jan 20]. https://www.ams.usda.gov/sites/default/files/media/CannedBeetsStandard.pdf
- Vaghefi N, Hay FS, Kikkert JR, Pethybridge SJ. 2016. Genotypic diversity and resistance to azoxystrobin of Cercospora beticola on processing table beet in New York. Plant Dis. 100:1466–1473.
- Vaghefi N, Kikkert JR, Bolton MD, Hanson LE, Secor GA, Nelson SC, Pethybridge SJ. 2017a. Global genotype flow in Cercospora beticola populations confirmed through genotyping-by-sequencing. PLoS One. 12:e0186488.
- Vaghefi N, Nelson SC, Kikkert JR, Pethybridge SJ. 2017b. Genetic structure of Cercospora beticola populations on Beta vulgaris in New York and Hawaii. Sci Rep. 7:1726.
- Villani SM, Hulvey J, Hily J-M, Cox KD. 2016. Overexpression of the CYP51A1 gene and repeated elements are associated with differential sensitivity to DMI fungicides in Venturia inaequalis. Phytopathology. 106:562–571.
- Vogel J, Kenter C, Holst C, Märländer B. 2018. New generation of resistant sugar beet varieties for advanced integrated management of Cercospora leaf spot in central Europe. Front Plant Sci. 9:222.
- Weiland J, Halloin JM. 2001. Benzimidazole resistance in Cercospora beticola sampled from sugar beet fields in Michigan, U.S.A. Can J Plant Pathol. 23:78–82.
- Weiland J, Koch G. 2004. Sugarbeet leaf spot disease (Cercospora beticola Sacc.). Mol Plant Pathol. 5:157–166.
- Windels CE, Lamey HA, Hilde D, Widner J, Knudsen T. 1998. A Cercospora leaf spot model for sugar beet in practice by an industry. Plant Dis. 82:716–726.