Abstract
Phytoplasmas are a major liming factor to the growth and production of many plant species worldwide. Most studies have used the conserved 16S rRNA gene for phytoplasma identification and classification. The efficiency of the tuf and secA genes length in the characterization of phytoplasmas was evaluated by designing universal primers to amplify the long and short tuf (740 bp and 414 bp) and secA (1232 bp and 536 bp) genes from the phytoplasma 16SrII-A and -D sub-groups, which are closely related phytoplasma strains. The neighbour joining (NJ) and the maximum likelihood (ML) methods were used to test the accuracy and stability of the phylogenetic trees. The NJ and ML phylogenetic trees from the long and short DNA sequences of the 16S rDNA, tuf, and secA genes produced two major clades (WB and AS). The NJ and ML phylogenetic trees showed high similarity in their differentiation between and within the 16S phytoplasma groups. This study indicates that the long DNA sequences of tuf and secA genes produced phylogenetic trees with higher resolution and separated very closely related phytoplasma groups. The accurate characterization of phytoplasmas is important for epidemiological studies and in disease management.
Résumé
Les phytoplasmes sont un facteur limitant important en ce qui a trait à la croissance et à la production de nombreuses plantes partout dans le monde. La majorité des études ont utilisé le gène conservé de l’ARNr 16S pour identifier et classifier les phytoplasmes. L’efficacité de la longueur des gènes tuf et secA quant à la caractérisation des phytoplasmes a été évaluée en concevant des amorces universelles pour amplifier les gènes tuf et secA longs et courts, (740 bp et 414 bp) et (1232 bp et 536 bp), respectivement, des sous-groupes 16SrII-A et -D du phytoplasme qui sont des souches de phytoplasmes étroitement apparentées. Les méthodes de neighbour joining (NJ) et de vraisemblance maximale (VL) ont été utilisées pour vérifier la précision et la stabilité des arbres phylogénétiques. Les arbres NJ et VL issus des séquences longues et courtes de l’ADN de l’ARNr 16S ainsi que des gènes tuf et secA ont produit deux principaux clades (WB et AS). Les arbres phylogénétiques NJ et VL ont affiché une grande similitude quant à leur différenciation entre et dans les groupes 16S de phytoplasmes. Cette étude indique que les séquences longues de l’ADN des gènes tuf et secA ont produit des arbres phylogénétiques de plus haute résolution qui ont permis de distinguer des groupes distincts de phytoplasmes étroitement apparentés. La caractérisation précise des phytoplasmes est importante sur le plan des études épidémiologiques et de la gestion des maladies.
Introduction
Phytoplasmas infect more than 700 species of plants worldwide (Rao and Kumar Citation2017; Rao et al. Citation2018; Bogoutdinov et al. Citation2019; Jurga and Zwolińska Citation2020). The identification and classification of phytoplasmas is challenging due to the difficulty of culturing phytoplasmas in vitro (Bertaccini et al. Citation2014). Characterization of phytoplasma diseases relies on the use of molecular methods, immuno-fluorescence tests, transmission electron microscopy, and serology and biological properties of the phytoplasmas (Bové et al. Citation1988; Nair et al. Citation2014; Wang et al. Citation2017).
In the 1990s, the conserved 16S rRNA gene was used for the identification and classification of phytoplasmas, which were classified into 43 phytoplasma Candidatus species (Girsova et al. Citation2017; Contaldo et al. Citation2018; Bogoutdinov et al. Citation2019). The 16S rRNA gene is a highly conserved and non-coding gene, so there is no clear-cut consensus in the variation of phytoplasma groups or subgroups within species (Martini et al. Citation2007; Cimerman et al. Citation2009). The use of conserved (16S rRNA) and less-conserved genes, such as the tuf, secA, rplV-rpsC, imp, secY, aceF, pnp, rpoB, and cpn60 genes allowed for a finer differentiation of closely related phytoplasma sub-groups, strains, and/or isolates (Arnaud et al., Citation2007; Valiunas et al. Citation2013; Dumonceaux et al. Citation2014; Cai et al. Citation2016; Cieślińska and Smolarek Citation2019; Mishra et al. Citation2019; Pilet et al. Citation2019; Plavec et al. Citation2019; Siampour et al. Citation2019). The 16SrXII-A ‘Candidatus Phytoplasma solani’ sub-group was divided into more than one strain by using tuf and vmp1 genes (Kostadinovska et al. Citation2014). The ribosomal protein operon (rp), secY, groEl, 16S–23S rRNA region and the tuf genes were all useful in the sub-division of ‘Ca. P. asteris’-related (16SrI) phytoplasmas (Mitrović et al. Citation2011). Many studies have used group-specific PCR primers that amplify long amplicons in order to study the taxonomic relationships among phytoplasmas within the 16Sr groups (Martini et al. Citation2007; Lee et al. Citation2010).
The accuracy in the differentiation of very closely related groups and sub-groups of phytoplasmas is more affected by the length of gene sequences (Makarova et al. Citation2012). The shorter DNA sequence of the tuf gene showed limitation in discriminating phytoplasmas among the closely related 16SrII-A and D sub-groups (Makarova et al. Citation2012; Al-Subhi et al. Citation2018). Further, the phytoplasma phylogenetic tree of the secA gene (using about 480 bp) showed a difference in the branch lengths when compared with the phytoplasma 16S rRNA gene tree (Hodgetts et al. Citation2008).
In this study, long and short DNA sequences of the tuf and secA genes from different phytoplasma groups, specifically the closely related phytoplasma strains 16SrII-A and -D sub-groups, were used to determine whether the use of longer gene sequences of the tuf and secA genes can improve the accuracy of phytoplasma discrimination and phylogenetic tree resolution.
Materials and methods
Phytoplasma selection
A set of 19 tuf and 18 secA phytoplasma GenBank gene sequences (NCBI, Bethesda, MD, USA) were downloaded from the National Centre for Biotechnology (NCBI) database and used for construction of datasets. In addition, a dataset of 21 phytoplasma 16S rRNA GenBank gene sequences (conserved gene) was obtained from the NCBI, which were used as a reference to evaluate the effect of sequence length variation of tuf and secA genes on phytoplasma detection and classification accuracy ().
Table 1. Phytoplasma gene sequences used in this study
Sequence analysis
For each dataset of the 16S rDNA (~1.15 kb), tuf and secA genes, the DNA sequences were aligned and checked using CLUSTAL W (Thompson et al. Citation1994). The 740 bp and 414 bp sequences were used to construct long and short phylogenetic trees of the tuf gene, respectively. The 1232 bp (long) and 536 bp (short) sequences were used for the secA gene. Two common methods were employed to test the accuracy and stability of the phylogenetic trees, the neighbour joining (NJ) and the maximum likelihood (ML). This was carried out with the MEGA 6 software (Tamura et al. Citation2013). The reference sequence of Bacillus subtilis 16S rRNA, tuf, and secA genes, GenBank Accession numbers AB042061, GCA000789275, and D10279, respectively, were used as an outgroup to root the phylogenetic trees.
Results and discussion
The neighbour joining (NJ) phylogenetic trees based on the DNA sequences from 16S rDNA, tuf and secA genes () resulted in two major clades (WB and AS) (Wang et al. Citation2003), which also had a strong bootstrap support (with values of 85%–100%) within the 16Sr group phytoplasmas. However, the trees based on the tuf and secA gene analyses and the 16S rDNA gene trees are similar, but not identical, in distribution of the phytoplasma 16Sr groups between the two main clades. The long and short DNA sequences of tuf and secA gene phylogenic trees placed the 16SrX-A subgroup ‘Ca. P. mali’ phytoplasma closer to the WB clad (), although the 16S rRNA gene tree places the 16SrX-A phytoplasma closer to the AS clade (). This finding is similar to previous studies (Danet et al. Citation2007; Martini et al. Citation2007; Hodgetts et al. Citation2008).
Fig. 1 Phylogenetic tree of the 16S rRNA gene sequences from the 21 published phytoplasma strains using (a) Neighbour Joining method (NJ) and (b) Maximum Likelihood method (ML); the trees were rooted using Bacillus subtilis (AB042061)
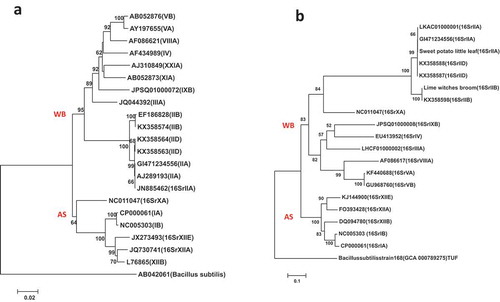
Fig. 2 Phylogenetic trees of tuf gene sequences from the previously published 19 phytoplasma strains () using the NJ method; (a) shows the tree based on the short DNA sequence (about 350 bp), (b) shows the tree based on the long DNA sequence (about 730 bp). The trees were rooted using Bacillus subtilis strain 168 (GCA:000789275)
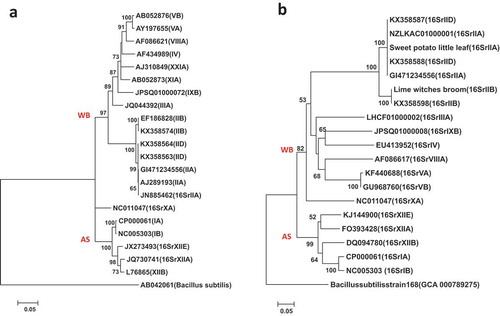
Fig. 3 Phylogenetic trees of secA gene sequences from the previously published 16 phytoplasma strains () using the NJ method; (a) shows the tree based on the short DNA sequence (about 530 bp), (b) shows the tree based on the long DNA sequence (about 1220 bp). The trees were rooted using Bacillus subtilis (D10279)
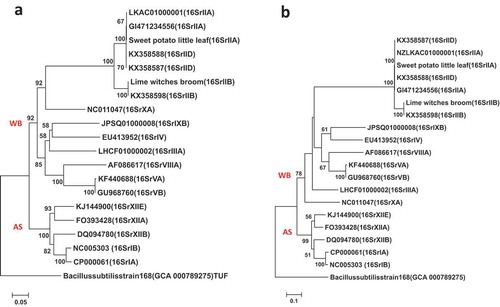
The tuf gene has been proposed for potential DNA barcoding and to study the genetic variability of phytoplasmas (Makarova et al. Citation2012; Kostadinovska et al. Citation2014; Al-Subhi et al. Citation2018). The phylogenetic tree of the short DNA sequence of tuf gene () placed the 16SrII-D sub-group (AlWB and SEWB phytoplasmas) and 16SrII-A sub-group (PnWB and SPLL phytoplasmas) in one sub-clade, supporting findings from earlier studies (Makarova et al. Citation2012; Kostadinovska et al. Citation2014). However, in the long tuf gene sequence (about 730 bp) of this study (), the phytoplasmas of the 16SrII-A and -D sub-groups clustered into two sub-clades, similar to the 16S rRNA gene-based tree () (Kostadinovska et al. Citation2014; Al-Subhi et al. Citation2018). The tuf gene has been used recently to detect and differentiate Ca. P. pini in pine phloem (Valiunas et al. Citation2019). These workers also generated a full-length sequence of the tuf gene for this phytoplasma strain (MK303568).
The short sequences of the secA gene were used for phytoplasma identification and placement into groups and sub-groups and also to improve the resolution of the candidate species ‘Candidatus Phytoplasma’ (Hodgetts et al. Citation2008; Al-Subhi et al. Citation2018). In this and previous studies, the phylogenetic trees of short secA gene DNA sequences placed the SPLL phytoplasma belonging to 16SrII-A subgroup in one sub-clade with the 16SrII-D sub-group phytoplasmas () (Hodgetts et al. Citation2008; Ramaswamy et al. Citation2013; Al-Subhi et al. Citation2018). On the other hand, the long secA gene (about 1220 bp) placed SPLL phytoplasma in a separate sub-clad away from the16SrII-D subgroup phytoplasmas ().
The results of the Maximum Likelihood method (ML) trees built from the 16S rRNA, tuf, and secA genes () supported the 16S phytoplasma groups of the Neighbour Joining method (NJ) phylogenetic trees (). They showed high similarity in their differentiation and classification both between and within the 16S phytoplasma groups.
Fig. 4 Phylogenetic trees of tuf gene sequences from the previously published 19 phytoplasma strains () using the ML method; (a) shows the tree based on the short DNA sequence (about 350 bp), (b) shows the tree based on the long DNA sequence (about 730 bp). The trees were rooted using Bacillus subtilis strain 168 (GCA:000789275)
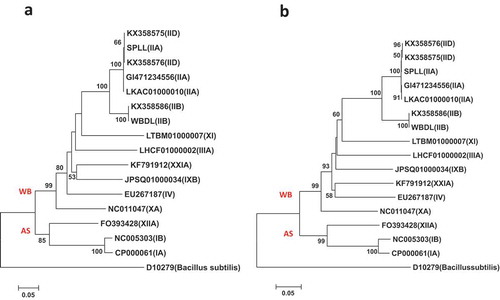
Fig. 5 Phylogenetic trees of secA gene sequences from the previously published 16 phytoplasma strains () using the ML method; (a) shows the tree based on the short DNA sequence (about 530 bp), (b) shows the tree based on the long DNA sequence (about 1220 bp). The trees were rooted using Bacillus subtilis (D10279)
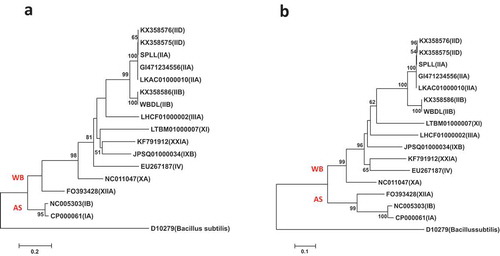
The examination of the 16S rDNA sequences allows for differentiation between phytoplasmas at the 16S group and sub-group levels, whereas for finer strain differentiation, including very close sub-grouped phytoplasmas, the 16S rRNA gene is insufficient and additional molecular markers such as the tuf and secA genes are necessary (Danet et al. Citation2011; Davis et al. Citation2013; Makarova et al. Citation2013). Overall, the short DNA sequences of the tuf and secA genes can still differentiate all the 16S group phytoplasmas and are used in barcoding and classification. This study shows that the long sequences produce phylogenetic trees with higher resolution that can separate very closely related phytoplasma groups. Besides taxonomic differentiation, the precise characterization of phytoplasmas is necessary in epidemic studies and disease control (Duduk and Bertaccini Citation2011; Bertaccini et al. Citation2014).
Acknowledgements
We thank SQU for facilitating our study.
Additional information
Funding
References
- Al-Subhi AM, Hogenhout SA, Al-Yahyai RA, Al-Sadi AM. 2018. Detection, identification, and molecular characterization of the 16SrII-D phytoplasmas infecting vegetable and field crops in Oman. Plant Dis. 102:576–588. doi:10.1094/PDIS-07-17-0935-RE.
- Arnaud G, Malembic-Maher S, Salar P, Bonnet P, Maixner M, Marcone C, Boudon-Padieu E, Foissac X. 2007. Multilocus sequence typing confirms the close genetic interrelatedness of three distinct flavescence dorée phytoplasma strain clusters and group 16SrV phytoplasmas infecting grapevine and alder in Europe. Appl Environ Microbiol. 73:4001–4010. doi:10.1128/AEM.02323-06.
- Bertaccini A, Duduk B, Paltrinieri S, Contaldo N. 2014. Phytoplasmas and phytoplasma diseases: a severe threat to agriculture. Am J Plant Sci. 5:1763–1788. doi:10.4236/ajps.2014.512191.
- Bogoutdinov DZ, Kastalyeva TB, Girsova NV, Samsonova LN. 2019. Phytoplasma diseases: a review of 50 year history and current advances. Selskokhozyaistvennaya Biol. 54:3–18. doi:10.15389/agrobiology.2019.1.3eng.
- Bové JM, Garnier M, Mjeni AM, Khayrallah A Witches’ broom disease of small-fruited acid lime trees in Oman: first MLO disease of citrus. Proceedings of the 10th Conference of the International Organization of Citrus Virologists (IOCV); 1988; Riverside.
- Cai H, Wang L, Mu W, Wan Q, Wei W, Davis RE, Chen H, Zhao Y. 2016. Multilocus genotyping of a ‘Candidatus Phytoplasma aurantifolia’-related strain associated with cauliflower phyllody disease in China. Ann Appl Biol. 169:64–74.
- Cieślińska M, Smolarek T. 2019. Multilocus sequence analysis of phytoplasmas detected in cherry trees in Poland. Zemdirbyste. 106:73–80. doi:10.13080/z-a.2019.106.010.
- Cimerman A, Pacifico D, Salar P, Marzachi C, Foissac X. 2009. Striking diversity of vmp1, a variable gene encoding a putative membrane protein of the stolbur phytoplasma. Appl Environ Microbiol. 75:2951–2957. doi:10.1128/AEM.02613-08.
- Contaldo N, Paltrinieri S, Bellardi MG, Lesi F, Satta E, Bertaccinia A. 2018. Rapid screening for phytoplasma presence in flower crops using tuf gene barcode. Acta Hortic. 1193:63–67. doi:10.17660/ActaHortic.2018.1193.9.
- Danet JL, Balakishiyeva G, Cimerman A, Sauvion N, Marie-Jeanne V, Labonne G, Laviňa A, Batlle A, Križanac I, Škorić D, et al. 2011. Multilocus sequence analysis reveals the genetic diversity of European fruit tree phytoplasmas and supports the existence of inter-species recombination. Microbiology. 157(Pt2):438–450. doi:10.1099/mic.0.043547-0.
- Danet JL, Bonnet P, Jarausch W, Carraro L, Skoric D, Labonne G, Foissac X. 2007. Imp and secY, two new markers for MLST (multilocus sequence typing) in the 16SrX phytoplasma taxonomie group. Bull Insectol. 60:339–340.
- Davis RE, Zhao Y, Dally EL, Lee IM, Jomantiene R, Douglas SM. 2013. ‘Candidatus Phytoplasma pruni’, a novel taxon associated with X-disease of stone fruits, Prunus spp.: multilocus characterization based on 16S rRNA, secY, and ribosomal protein genes. Int J Syst Evol Microbiol. 63:766–776. doi:10.1099/ijs.0.041202-0.
- Duduk B, Bertaccini A. 2011. Phytoplasma classification: taxonomy based on 16S ribosomal gene, is it enough? Phytopathogenic Mollicutes. 1:3–13. doi:10.5958/j.2249-4669.1.1.001.
- Dumonceaux TJ, Green M, Hammond C, Perez E, Olivier C. 2014. Molecular diagnostic tools for detection and differentiation of phytoplasmas based on chaperonin-60 reveal differences in host plant infection patterns. PLoS ONE. 9(12):e116039. doi:10.1371/journal.pone.0116039.
- Girsova NV, Bottner-Parker KD, Bogoutdinov DZ, Kastalyeva TB, Meshkov YI, Mozhaeva KA, Lee IM. 2017. Diverse phytoplasmas associated with leguminous crops in Russia. Eur J Plant Pathol. 149:599–610. doi:10.1007/s10658-017-1209-6.
- Hodgetts J, Boonham N, Mumford R, Harrison N, Dickinson M. 2008. Phytoplasma phylogenetics based on analysis of secA and 23S rRNA gene sequences for improved resolution of candidate species of ‘Candidatus Phytoplasma’. Int J Syst Evol Microbiol. 58:1826–1837. doi:10.1099/ijs.0.65668-0.
- Jurga M, Zwolińska A. 2020. Phytoplasmas in Poaceae species: a threat to the most important cereal crops in Europe. J Plant Pathol. 102:287–297. doi:10.1007/s42161-019-00481-6.
- Kostadinovska E, Quaglino F, Mitrev S, Casati P, Bulgari D, Bianco PA. 2014. Multiple gene analyses identify distinct “bois noir” phytoplasma genotypes in the Republic of Macedonia. Phytopathol Mediterr. 53:491–501.
- Lee IM, Bottner-Parker KD, Zhao Y, Davis RE, Harrison NA. 2010. Phylogenetic analysis and delineation of phytoplasmas based on secY gene sequences. Int J Syst Evol Microbiol. 60:2887–2897. doi:10.1099/ijs.0.019695-0.
- Makarova O, Contaldo N, Paltrinieri S, Bertaccini A, Nyskjold H, Nicolaisen M. 2013. DNA bar-coding for phytoplasma identification. Methods Mol Biol. 938:301–317.
- Makarova O, Contaldo N, Paltrinieri S, Kawube G, Bertaccini A, Nicolaisen M. 2012. DNA Barcoding for Identification of ‘Candidatus Phytoplasmas’ using a fragment of the elongation factor Tu Gene. PLoS ONE. 7:e52092. doi:10.1371/journal.pone.0052092.
- Martini M, Lee I-M, Bottner KD, Zhao Y, Botti S, Bertaccini A, Harrison NA, Carraro L, Marcone C, Khan AJ, et al. 2007. Ribosomal protein gene-based phylogeny for finer differentiation and classification of phytoplasmas. Int J Syst Evol Microbiol. 57:2037–2051. doi:10.1099/ijs.0.65013-0.
- Mishra S, Mitra S, Radhika NS, Yadav A, Rao GP. 2019. Multilocus gene-specific characterization of ‘Candidatus Phytoplasma australasia’ associated with shoot proliferation disease of small cardamom in India. 3 Biotech. 9(11):420. doi:10.1007/s13205-019-1944-9.
- Mitrović J, Kakizawa S, Duduk B, Oshima K, Namba S, Bertaccini A. 2011. The groEL gene as an additional marker for finer differentiation of ‘Candidatus Phytoplasma asteris’-related strains. Ann Appl Biol. 159:41–48. doi:10.1111/j.1744-7348.2011.00472.x.
- Nair S, Roshna OM, Soumya VP, Hegde V, Suresh Kumar M, Manimekalai R, Thomas GV. 2014. Real-time PCR technique for detection of arecanut yellow leaf disease phytoplasma. Australas Plant Pathol. 63(5):527–529. doi:10.1007/s13313-014-0278-7.
- Pilet F, Quaicoe RN, Osagie IJ, Freire M, Foissac X. 2019. Multilocus sequence analysis reveals three distinct populations of ‘Candidatus Phytoplasma palmicola’ with a specific geographical distribution on the African continent. Appl Environ Microbiol. 85:e02716–02718. doi:10.1128/AEM.02716-18.
- Plavec J, Budinšćak Ž, Križanac I, Škorić D, Foissac X, Šeruga Musić M. 2019. Multilocus sequence typing reveals the presence of three distinct flavescence dorée phytoplasma genetic clusters in Croatian vineyards. Plant Pathology. 68:18–30. doi:10.1111/ppa.12934.
- Ramaswamy M, Nair S, Soumya VP, Thomas GV. 2013. Phylogenetic analysis identifies a ‘Candidatus Phytoplasma oryzae’-related strain associated with yellow leaf disease of areca palm (Areca catechu L.) in India. Int J Syst Evol Microbiol. 63:1376–1382. doi:10.1099/ijs.0.043315-0.
- Rao GP, Kumar M. 2017. World status of phytoplasma diseases associated with eggplant. Crop Prot. 96:22–29. doi:10.1016/j.cropro.2017.01.005.
- Rao GP, Marcone C, Bellardi MG. 2018. Phytoplasma diseases of medicinal crops. In: Rao GP, Bertaccini A, Fiore N, Liefting LW, editors. Phytoplasmas: plant pathogenic bacteria - I: characterisation and epidemiology of phytoplasma —associated diseases. Singapore: Springer; p. 235–266.
- Siampour M, Izadpanah K, Martini M, Salehi M. 2019. Multilocus sequence analysis of phytoplasma strains of 16SrII group in Iran and their comparison with related strains. Ann Appl Biol. 175:83–97. doi:10.1111/aab.12513.
- Tamura K, Stecher G, Peterson D, Filipski A, Kumar S. 2013. MEGA6: molecular evolutionary genetics analysis version 6.0. Mol Biol Evol. 30:2725–2729. doi:10.1093/molbev/mst197.
- Thompson JD, Higgins DG, Gibson TJ. 1994. CLUSTAL W: improving the sensitivity of progressive multiple sequence alignment through sequence weighting, position-specific gap penalties and weight matrix choice. Nucleic Acids Res. 22:4673–4680. doi:10.1093/nar/22.22.4673.
- Valiunas D, Jomantiene R, Davis RE. 2013. Evaluation of the DNA-dependent RNA polymerase β-subunit gene (rpoB) for phytoplasma classification and phylogeny. Int J Syst Evol Microbiol. 63:3904–3914. doi:10.1099/ijs.0.051912-0.
- Valiunas D, Jomantiene R, Ivanauskas A, Sneideris D, Zizyte-Eidetiene M, Shao J, Yan Z, Costanzo S, Davis RE. 2019. Rapid detection and identification of ‘Candidatus Phytoplasma pini’-related strains based on genomic markers present in 16S rRNA and tuf genes. Forest Pathology. 49(6). doi:10.1111/efp.12553.
- Wang J, Gao R, Yu XM, An M, Ai CX. 2017. Morphological and molecular detection of phytoplasma associated with persimmon fasciation disease. Zhiwu Shengli Xuebao/Plant Physiol J. 53:219–226.
- Wang K, Hiruki C, Yeh F. 2003. Molecular evolution of phytoplasmas based on polymorphisms in the 16S rRNA genes and the 16/23S spacer regions. Proc Jpn Acad Ser B Phys Biol Sci. 79:155–162. doi:10.2183/pjab.79B.155.