Abstract
Restrictions about the use of chemicals have limited the availability of control measures against plant-parasitic nematodes. The search for more sustainable approaches has focused the attention on biological control agents, such as Trichoderma species. In recent years, there has been a growing interest in the use of biopolymers for a wide range of applications. These polysaccharide-based compounds may be 20 good carriers of microbial agents or act as barriers against pathogens or pests for their ability to form coating films. In this study, we evaluated the combination of a biopolymer obtained from the leguminous plant Ceratonia siliqua and T. harzianum M10, T. atroviride P1 or T. longibrachiatum MK1, as root protector or adjuvant agents, for the management of the root-knot nematode Meloidogyne incognita. Coating tomato roots with the carob galactomannan biopolymer followed by soil application of selected Trichoderma strains reduced the root galling index caused by M. incognita and soil nematode population in comparison to untreated control under greenhouse conditions. 25 Scanning electron microscopy revealed that coated tomato roots were embedded within a polymeric material. The sedimentation test showed that the addition of this biopolymer retarded the tendency of Trichoderma spores to settle in the bottom of aqueous suspension. In conclusion, beneficial fungi combined or formulated with a biopolymer could represent a promising strategy to increase their activity in plant protection and enhance their proliferation or distribution into rhizosphere.
Résumé
Les restrictions relatives aux pesticides de synthése ont limité l’offre quant aux moyens de lutte contre les nématodes phytoparasitaires. La recherche pour des approches plus durables s’est focalisée sur des agents de lutte biologique tels que les espéces de Trichoderma. Récemment, il y a eu un intérêt croissant pour l’utilisation de biopolyméres, et ce, pour une grande variété d’applications. Ces composés à base de polysaccharides peuvent être de bons vecteurs d’agents microbiens ou agir comme barriéres contre les agents pathogénes ou les organismes nuisibles grâce à leur capacité à former des pellicules protectrices. Dans cette étude, nous avons évalué la combinaison d’un polymére obtenu de la légumineuse Ceratonia siliqua L. et de T. harzianum M10, T. atroviride P1 ou T. longibrachiatum MK1 comme protecteur de racine ou adjuvant afin de gérer le nématode cécidogéne Meloidogyne incognita. En serre, l’application d’un biopolymére issu du galactomanne de caroube sur des racines de tomate, suivie de l’inoculation du terreau avec des souches choisies de Trichoderma, a permis de réduire l’indice de galles racinaires causées par M. incognita ainsi que la population de nématodes terricoles, comparativement à un témoin non traité. La microscopie par balayage a montré que les racines de tomates enrobées étaient couvertes d’un polymére. Le test de sédimentation a démontré que l’ajout de ce biopolymére a retardé la tendance qu’ont les spores de Trichoderma de se déposer au fond d’une suspension aqueuse. Pour conclure, la combinaison de champignons bénéfiques avec un biopolymére, ou la composition d’un tel produit, pourraient se révéler une stratégie prometteuse pour accroître leur action protectrice et améliorer leur prolifération ou leur distribution dans la rhizosphére.
Introduction
Under Directive 2009/128/EC, the European Community has deeply restricted or revised the use of pesticides in plant protection focusing the attention on more sustainable approaches. The root-knot nematode (RKN) Meloidogyne incognita (Kofoid & White) Chitwood is the most widespread and damaging plant-parasitic nematode for a wide range of economically important crops in Italy, including tomato plants (d’Errico et al. Citation2014, Citation2016; Landi et al. Citation2018). Different methods have been developed and used to manage RKNs, but chemical control still represents the most efficient strategy under high infestation levels (Giacometti et al. Citation2010; d’Errico et al. Citation2011a, Citation2011b, Citation2017a, Citation2017b). However, this approach has become a source of public concern for several toxicological and environmental reasons, that necessitate reduced reliance on conventional pesticides (Lamichhane et al. Citation2016; d’Errico et al. Citation2018). Various non-chemical strategies, including crop rotation, soil solarization, plant resistance, biofumigation, organic amendments, cultural practices and application of biological control agents (BCAs), have shown a considerable potential to keep nematode population below damaging levels (Sharon et al. Citation2001, Citation2007; Zasada et al. Citation2010; Soppelsa et al. Citation2011; Mocali et al. Citation2015; Marra et al. Citation2018). Worldwide researches suggest that nematode control can be achieved using integrated measures (Zasada et al. Citation2010; Sokhandani et al. Citation2016; d’Errico et al. Citation2019). Among BCAs, fungi belonging to the Trichoderma genus are known as broad-spectrum plant protection agents and growth promoters (Lorito et al. Citation2010; Woo et al. Citation2014; Lombardi et al. Citation2018), acting with different mechanisms (Howell Citation2003; Harman et al. Citation2004; Vinale et al. Citation2008; Marra et al. Citation2019). However, the type of formulation greatly affects the distribution and the efficacy of spore-based bio-products, i.e. by improving the contact with the plant tissues and protecting them from micro-environmental factors (Schroer et al. Citation2005).
The use of plant-derived polymers in agriculture is attractive because they are biocompatible, biodegradable and renewable. However, the application of these polymers is mainly adopted in the pharmaceutical sector (Satturwar et al. Citation2003; Perepelkin Citation2005; Chaurasia et al. Citation2006; Malafaya et al. Citation2007; Chivate et al. Citation2008). Polymeric materials can be used as stabilizers, film-coating formers, thickeners or viscosity enhancers, delivery systems, suspending agents, bio-adhesives, etc. (Guo et al. Citation1998). Beneficial agents can be mixed with adhesive polymers, including methylcellulose, xanthan gum, chitosan, latex derivatives and synthetic adhesives to produce seed or root coatings (Brownbridge et al., Citation2012; Benhamou et al. Citation1998; Tefera and Vidal Citation2009; Colla et al. Citation2015; Ruiz-de-La-Cruz et al. Citation2017). In this study, the possible agricultural applications of a polymer obtained from the leguminous plant Ceratonia siliqua (L.), commonly known as carob tree and widely distributed in the Mediterranean basin, have been investigated. This natural product consists of a galactomannan polymer composed of 1,4-linked D-mannopyranosyl units and every fourth or fifth chain unit is substituted on C6 with a D-galactopyranosyl unit (Beneke et al. Citation2009). The mannose/galactose (M/G) ratio differ according to the species (Neukom Citation1989; Reid and Edwards Citation1995; Beneke et al. Citation2009). Galactomannans are water soluble hydrocolloids which form highly viscous, stable aqueous solutions weakly affected by pH changes (Glicksman Citation1963; Neukom Citation1989; Kök et al. Citation1999). To the best of our knowledge, combination between this specific galactomannan polymer and Trichoderma species has not been investigated.
Specifically, our work aims to evaluate the efficacy of Trichoderma spp. combined with the carob biopolymer to control RKNs infestation in tomato plants and evaluate the feasibility of their applications. Thus, the biopolymer was tested: (a) as a root coating for three Trichoderma species in M. incognita naturally infested soil; and (b) for effects on spore sedimentation and survival in laboratory tests, indicating potential for use as an adjuvant in fungal formulations.
Materials and methods
Biopolymer studies
Experimental procedure for the production of galactomannan
Brown beans collected from C. siliqua were processed by milling the endosperms to obtain a flour. The polysaccharide extraction was performed using ethanol and distilled water. Seeds were removed from the pods, cleaned and mechanically crushed in a blender. Then, the endosperm was suspended in ethanol (EtOH, purity 99.8%, Riedel-de Haën, Germany) in a proportion 1:3 (v/v, seeds: EtOH) at 70°C for 15 min. The ethanol was decanted and distilled water was added in a proportion of 1:5 (v/v, endosperm: water). The suspension was left to rest for approximately 24 hours. Subsequently, water was added (1:10 v/v, suspension: water) and the solution was mixed in a blender for 5 min.
Viscosity test
The viscosity of 1% galactomannan polymer solution (w/v) in water was tested at 25°C and pH 6.0 by a ‘Visco Star R’ (Opto-Lab, Modena, Italy) rotational viscometer. This tool allows the determination of the viscosity of products based on the rotational principle. It consists of immersing a rotor in the material to be tested and rotating it at a constant speed. Resistance generated by the sample on the viscometer rotor is directly proportional to its viscosity. Using a microprocessor, the viscometer determines viscosity directly in digital form, expressed in centipoises (cps; mPa * s). The apparent viscosity was calculated using the constants provided by the manufacturer and six different shear rates (rpm), and values were expressed as cps. On the basis of preliminary results, the minimum quantity of galactomannan polymer showing significant effect was selected for our experiments.
Spore sedimentation test
The biopolymer was also evaluated for the feasibility to retard sedimentation of T. harzianum strain M10 spores in water suspension (spore emulsifier). Sedimentation was recorded using the method described by Peters and Backes (Citation2003). Thus, the biopolymer was added at different concentrations (0, 0.1, 0.3 and 0.5%; v/v) to distilled water. Spore suspensions were applied to obtain an initial concentration of 1 × 108 spore mL−1 and stirred in a 13.0/13.5/6.5 cm (l/w/h) flask until spores were equally distributed in the mixture. The effect of the biopolymer on spore sedimentation was estimated by sampling 1 mL of the suspension from a depth of 2 cm at 0, 10, 15, 30 and 60 min after stopping the agitation. Fungal spore concentration was counted using a Bürker chamber (Knittel, Germany). This experiment was replicated four times and repeated twice.
Determination of fungal colony-forming units
The survival of T. harzianum strain M10 spores in aqueous solutions containing galactomannan at different concentrations was determined. After one week in solution, an aliquot of solution was collected from each biopolymer concentration (0, 0.1, 0.3 and 0.5%; v/v). Detection and quantification of fungal colony-forming units (CFUs) was conducted using standard techniques by serial dilutions. The total number of fungal CFUs was estimated on Rose Bengal-Chloramphenicol agar (HiMedia Pvt. Ltd., Mumbai, India) supplemented with 0.1% (v/v) Igepal (Sigma-Aldrich, Milan, Italy). A 100 μL aliquot of each solution was spread on the surface of the solid media and plated in three replicates. The CFUs were counted after incubation for 3–7 days at 25°C. This experiment was repeated twice.
Greenhouse studies
Trichoderma strains and culture conditions
The antagonistic fungi T. harzianum Rifai strain M10, T. atroviride Karsten strain P1 and T. longibrachiatum Rifai strain MK1 were maintained on Potato Dextrose Agar (PDA, SIGMA, St Louis, MO) slants at 25°C and sub-cultured bimonthly. Conidia were collected by adding sterile distilled water (10 mL) to each Petri plate and scratching the agar surface with a sterile spreader. After removing the fungal biomass, spore concentration was counted using a Bürker chamber and adjusted to 1 × 108 spores mL−1. Conidial suspensions were stored at 4°C until use.
Chemical nematicide
A commercial preparation of fosthiazate 150 g L−1 (Nemathorin® 150 EC; Syngenta) was used as standard control and applied to the soil at the dose of 10.0 L ha−1 (recommended dose from the manufacturer).
Pot experiments
The biopolymer solution was applied as root dipping in order to coat and fix fungal spores to the plant tissue. The experiments were conducted in a greenhouse (located in Portici, Naples, south Italy) using pots (14 cm ø) filled with homogenously mixed sandy clay loam soil (pH 7.5) containing 1% organic matter (w/w). The initial nematode population from naturally infested soil was extracted by sieving on cotton-wool filters for 48 h at room temperature (Cobb Citation1918) and then second stage juveniles (J2s) of M. incognita were counted (40 J2s per 100 cm3 soil).
The roots of tomato (Lycopersicon lycopersicum L. cv. Roma) seedlings, at the two true-leaf stage, were immersed in the biopolymer solution (0.3% v/v) for 30 min and air-dried for 20 min before transplanting. Then, seedlings were transplanted into pots and soil inoculated with spore suspensions (50 mL of 1 × 107 spores mL−1). The experiment consisted of nine treatments: (1) T. harzianum strain M10; (2) T. atroviride strain P1; (3) T. longibrachiatum strain MK1; (4) T. harzianum strain M10 plus biopolymer root coating; (5) T. atroviride strain P1 plus biopolymer root coating; (6) T. longibrachiatum strain MK1 plus biopolymer root coating; (7) biopolymer root coating; (8) chemical control (nematicide); (9) untreated control (water). The experiment was carried out using a completely randomized block design with 16 replications per treatment and repeated twice. Nematode infestation parameters were calculated on each replication pot. Ordinary flood irrigation was provided according to the crop water requirements. After 60 days from transplanting time, plants were removed from the pots and the roots were washed to remove soil residuals. Root galling index (RGI) was evaluated for each plant using a 0–10 scale, where 0 = no galling visible, 1 = 10% of the root system galled, 2 = 20% of the roots galled, etc., and 10 = 100% galled roots. Final soil nematode population was determined as reported above.
Scanning electron microscopy
Six tomato seedlings from the same block of plants used for pot experiments were utilized for Scanning Electron Microscope (SEM) images. To investigate the ultrastructure of the upper surface of the biopolymer (0.3%) on roots, samples (c. 5 × 5 mm) were fixed with 2.5% glutaraldehyde in 0.1 M phosphate buffered saline (PBS, pH 7.4) at 0–4°C for 24 h. Each sample was rinsed three times in PBS and slowly transferred through a short alcohol series (70%, 85%, 95% and 100%), before drying with CO2 at the critical point in a critical-point dryer EMITECH K850 (Emitech, Ashford, UK). The dry tissues were then mounted on aluminium stubs and coated with gold/palladium in an agar sputter coater B7340 040 (Agar Scientific Ltd, Stansted, UK). Representative specimens were examined with a Zeiss EV040 SEM. Images of tomato roots are presented at a magnification of 310 × and 307 × (treated and untreated roots, respectively), accelerating voltage of 20.00 kV, current of 10 mA and focal length (15.00 mm < WDL < 18.00 mm). Tomato roots without biopolymer coating were used as controls.
Statistical analysis
Data were subjected to analysis of variance (ANOVA) followed by Student Newman Keuls multiple comparison (SNK) test using SPSS software (SPSS Statistics, v. 21 for Windows). Analyses of the pooled data were done only if there was no significant heterogeneity between the repeated experiments. Data were expressed as mean ± SD (standard deviation) and the level of significance was set at p < 0.05.
Results
Biopolymer studies
Viscosity test
Viscosity values for 1% galactomannan polymer solution in water were dependent on the rate of share as seen in . In general, viscosity values were inversely proportional to share rate with a decrease in values (8 cps) from 10 to 20 rpm. The viscosity values decreased (9 to 23.5 cps) gradually from 20 rpm to 100 rpm.
Table 1. Viscosity values expressed in centipoises (cps; mPa*s) of 1% galactomannan polymer aqueous solution measured at 25°C and pH 6.0 according to share rates (rpm)
Spore sedimentation test
The galactomannan solution was tested to evaluate the effect on sedimentation of T. harzianum strain M10 spores in water suspensions. shows that the biopolymer used at the highest tested concentrations (0.3% and 0.5% v/v) retarded the sedimentation of fungal spores in water after 60 minutes. Conversely, the concentration of spores detected in water control or in the presence of 0.1% (v/v) biopolymer was significantly reduced (p < 0.05) after 60 minutes. As there were no significant differences (SNK’s test: p = 0.26) between repeated experiments, analyses were done on the pooled data.
Fig. 1 Effects of different concentrations of the biopolymer on Trichoderma harzianum strain M10 spore counts in water. Data were obtained at different time points (5, 10, 15, 30 and 60 min) after the agitation was complete. Values are means of triplicate measurements of spores taken from suspensions at a depth of 2 cm. Different letters indicate significant differences by the Student-Newman-Keuls test at p < 0.05
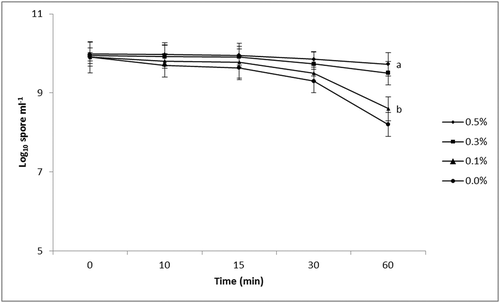
Determination of fungal colony-forming units
The survival of T. harzianum strain M10 spores in aqueous solutions containing different concentrations of galactomannan was determined after an incubation time of one week. On selective media, the CFUs/plate of Trichoderma inoculum amended with the biopolymer at 0.1% and 0.3% were not different from the control (Trichoderma inoculum only). The CFUs (except for the biopolymer solution at 0.5%) ranged from 1.3 × 104 to 2.9 × 104 among different solutions (). Interestingly, the highest number of fungal colonies was observed in the highest concentration (0.5%) of the galactomannan. The fungal concentration in biopolymer solution at 0.5% was 10-fold more than those observed in the less concentrated and water solutions, with counts ranging from 1.6 × 105 to 2.5 × 105 CFUs (). No significant differences (SNK’s test: p = 0.31) were observed between repeated experiments.
Table 2. Number of colony-forming units (CFUs) of Trichoderma harzianum strain M10 spores after an incubation period of one week in 0%, 0.1%, 0.3% and 0.5% galactomannan polymer aqueous solutions. Measurements are from two experiments with three replicates each. Different letters indicate significant differences by the Student-Newman-Keuls test with p < 0.05
Greenhouse studies
Pot experiments
The use of a galactomannan biopolymer as root coating in combination with soil applications of different Trichoderma species, applied singly, was evaluated in reducing M. incognita infestation on tomato plants. Generally, no differences among treatments with T. harzianum, T. atroviride and T. longibrachiatum were observed. These Trichoderma species, applied to coated or uncoated roots, significantly (p < 0.05) reduced by 28% to 38% the number of M. incognita J2s in soil in comparison to the untreated control (). Coated roots (without Trichoderma inoculum) caused no effect on soil nematode population, showing statistically similar results (p > 0.05) in comparison to the untreated control (water). Although, among treatments, the nematicide fosthiazate produced the maximum reduction in soil nematode population (up to 70%). Root coating plus Trichoderma inoculum significantly (p < 0.05) reduced the RGI by 40% (M10), 42% (P1) and 39% (MK1) as compared to the untreated control (). Uncoated roots showed decrements in RGI values of 27% (M10) and 30% (both P1 and MK1) in comparison to the water control. However, no statistical differences in RGI values were detected between coated and uncoated roots. Fosthiazate treatment showed the highest RGI reduction (up to 75%). Interestingly, root coating with the biopolymer alone decreased RGI values (up to 20%) in comparison to untreated plants (water). No significant differences (SNK’s test: p = 0.40) were observed between the two repeated experiments.
Fig. 2 Effects of root-coating with the biopolymer and inoculation of Trichoderma spores on second stage juveniles (J2s) of Meloidogyne incognita in 100 cm3 of soil. Values are means of combined results from two experiments with 16 replicates each. Different letters indicate significant differences by the Student-Newman-Keuls test at p < 0.05
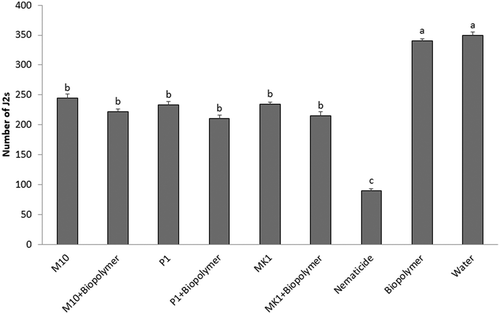
Fig. 3 Effects of root-coating with biopolymer and inoculation of Trichoderma spores on root galling index (RGI) caused by Meloidogyne incognita on tomato plants. RGI was evaluated using the 0–10 scale where, 0 = no galling visible, 1 = 10% of the root system galled, 2 = 20% of the roots galled, etc., and 10 = 100% galled roots. Values are means of combined results from two experiments with 16 replicates each. Different letters indicate significant differences by the Student-Newman-Keuls test at p < 0.05
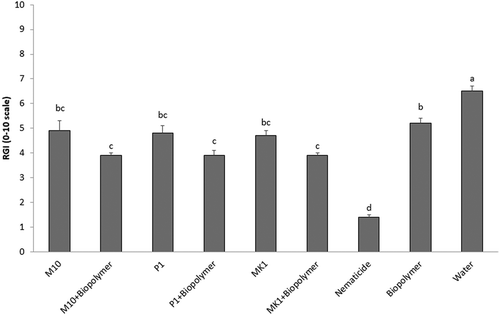
Scanning electron microscopy
SEM analyses was used to display biofilm formation on tomato root surface. Images of uncoated roots in comparison to those coated revealed an entirely different appearance of root surface (). The presence of the biopolymer resulted in a structure covering the surface of the later roots ().
Discussion
Galactomannan obtained from C. siliqua supported the performance of Trichoderma spp. in reducing root galling severity caused by the root-knot nematode M. incognita on tomato plants. In our experiments, Trichoderma species and strains were selected for their well-known activity as antagonists, plant growth promoters and secondary metabolite producers (Spiegel and Chet Citation1998; Woo et al. Citation1999; Dababat and Sikora Citation2007; Ruocco et al. Citation2009; Vinale et al. Citation2013). Results indicate that these Trichoderma species showed some potential in reducing root galling index and nematode density parameters as previously reported (Braithwaite et al. Citation2016). Soil nematode population was not influenced when the biopolymer was used alone, conversely RGI was significantly affected by root coating in comparison to the untreated control. Likely, reductions in RGI values may be attributed to an inhibition of M. incognita J2s to penetrate into roots and successfully reproduce caused by the biopolymer film formation. We suppose that root coating allowed functionality and retention of the Trichoderma spores on the root surface. This observation could be in accordance to previous reports regarding the film-forming properties of various plant polysaccharides such as pectin, inulin and rosin (Beneke et al. Citation2009). Additionally, it is feasible that the root protection conferred by the biopolymer film formation was probably useful to overcome the initial inactivity of the fungal spores. However, further studies are required to investigate the presence of minor metabolites from seeds of C. siliqua and their effect on M. incognita.
SEM confirmed that the surfaces of coated tomato roots were morphologically different from those of uncoated roots. In fact, root coating formed a continuous translucent film showing a dusty appearance when dry. This feature may confer root tissue adherence for beneficial spore treatments. However, additional micro-plot and field experiments are necessary to recommend the use of these compounds in integrated nematode management systems.
Furthermore, our results also support the use of the galactomannan polymer as carrier for Trichoderma-based bioformulations, as the activity of the beneficial fungus towards M. incognita was not affected. Likely, the biopolymer may improve the rhizosphere colonization by plant growth-promoting beneficial microbes as reported for some organic soil amendments (Pérez-Piqueres et al. Citation2006). Interestingly, the number of CFUs was higher on plates amended with galactomannan at 0.5%, whereas at lower concentrations of galactomannan fungal growth was not affected by the biopolymer. Our results demonstrated that the Trichoderma growth process was improved when the galactomannan was implemented. This biopolymer, used as adjuvant and/or additive in formulation, may have the function of immobilizing the beneficial spores around the rizosphere and create suitable environmental conditions for their development. In addition, we found that the survival of fungal spores after incubation in an aqueous solution of galactomannan was preserved. These characteristics make this galactomannan polymer a valid formulation compound in order to reduce the fungal application density as previously observed for entomopathogenic nematodes (Ehlers Citation1996).
Additionally, the biopolymer retarded the sedimentation of fungal spores over time increasing the viscosity of water. The viscosity of the recovered galactomannan is typical of a high molecular weight polymer (Robinson et al. Citation1982). This physical parameter seems to be strongly correlated with the prevention of spore sedimentation, provision of ideal conditions for host invasion and, consequently, efficacy of the formulation. However, the slowest spore sedimentation allows a more homogeneous distribution of spores under field conditions.
Conclusions
Plants and their derivatives represent outstanding sources of natural compounds displaying multiple properties, i.e. film forming, stabilization of emulsions, etc. Here we provide evidence that a carob-derived biopolymer, mainly constituted by galactomannans, may be utilized as an excipient in agricultural bioformulations (i.e. biopesticides or biofertilizers) for the effective delivery of beneficial microbes.
Additional information
Funding
References
- Beneke CE, Viljoen AM, Hamman JH. 2009. Polymeric plant-derived excipients in drug delivery. Molecules. 14(7):2602–2620. doi:10.3390/molecules14072602.
- Benhamou N, Kloepper JW, Tuzun S. 1998. Induction of resistance against Fusarium wilt of tomato by combination of chitosan with an endophytic bacterial strain: ultrastructure and cytochemistry of the host response. Planta. 204(2):153–168. doi:10.1007/s004250050242.
- Braithwaite M, Clouston A, Minchin R, Yardley J, Nieto-Jacobo MF, Mendoza-Mendoza A, Steyaert J, Hill R, Marshall J, Stewart A. 2016. The density-dependent effect of initial nematode population levels on the efficacy of Trichoderma as a bio-nematicide against Meloidogyne hapla on tomato. Australas Plant Path. 45(5):473–479. doi:10.1007/s13313-016-0432-5
- Brownbridge M, Reay SD, Nelson TL, Glare TR. 2012. Persistence of Beauveria bassiana (Ascomycota: hypocreales) as an endophyte following inoculation of radiata pine seed and seedlings. Biol Control. 61:194–200. doi:10.1016/j.biocontrol.2012.01.002.
- Chaurasia M, Chourasia MK, Jain NK, Jain A, Soni V, Gupta Y, Jain SK. 2006. Cross-linked Guar Gum microspheres: A viable approach for improved delivery of anticancer drugs for the treatment of colorectal cancer. AAPS Pharm Schi Tech. 7:E1–E9.
- Chivate AA, Poddar SS, Abdul S, Savant G. 2008. Evaluation of Sterculia foetida gum as controlled release excipient. AAPS Pharm Sci Tech. 9:197–204. doi:10.1208/s12249-008-9039-7.
- Cobb NA. 1918. Estimating the nema population of the soil. Agric Tech Circ Bur Pl Ind U S Dep Agric. No.1:48.
- Colla G, Rouphael Y, Bonini P, Cardarelli M. 2015. Coating seeds with endophytic fungi enhance growth, nutrient uptake, yield, and grain quality of winter wheat. Int J Plant Prot. 9:171–189.
- d’Errico G, Crescenzi A, Landi S. 2014. First Report of the Southern Root-Knot Nematode Meloidogyne incognita on the Invasive Weed Araujia sericifera in Italy. Plant Dis. 98(11):1593. doi:10.1094/PDIS-06-14-0584-PDN.
- d’Errico G, d’Errico FP, Greco N. 2011a. Efficacy of the available soil fumigants for the control of the root-knot nematode, Meloidogyne incognita, in tomato in plastic-house. Acta Hortic. 914:237–241. doi:10.17660/ActaHortic.2011.914.43.
- d’Errico G, Giacometti R, Roversi PF, d’Errico FP, Woo SL. 2017a. Mode of Action and Efficacy of Iprodione against the Root-Knot Nematode Meloidogyne incognita. Ann App Biol. 171:506–510. doi:10.1111/aab.12397.
- d’Errico G, Giacometti R, Roversi PF, Prasad L, Woo SL. 2016. Root knot disease caused by Meloidogyne incognita on tomato grown in soil-less culture in Italy. Redia. 99:25–28.
- d’Errico G, Giacometti R, Soppelsa O, D’Alessio M. 2011b. Effectivennes of plant-derived formulations against the root-knot nematode Meloidogyne incognita (Kofoid et White) Chitw. In a Protected Tomato Crop Redia. 44:167–169.
- d’Errico G, Marra R, Crescenzi A, Davino SW, Fanigliulo A, Woo SL, Lorito M. 2019. Integrated management strategies of Meloidogyne incognita and Pseudopyrenochaeta lycopersici on tomato using a Bacillus firmus-based product and two synthetic nematicides in two consecutive crop cycles in greenhouse. Crop Prot. 122:159–164. doi:10.1016/j.cropro.2019.05.004.
- d’Errico G, Marra R, Vinale F, Landi S, Roversi PF, Woo SL. 2017b. Nematicidal efficacy of new abamectin-based products used alone and in combination with indolebutyric acid against the Root-Knot Nematode Meloidogyne incognita. Redia. 100:95–101.
- d’Errico G, Woo SL, Lombardi N, Manganiello G, Roversi PF. 2018. Activity of chestnut tannins against the southern root-knot nematode Meloidogyne incognita. Redia. 101:53–59.
- Dababat AA, Sikora RA. 2007. Use of Trichoderma harzianum and Trichoderma viride for the biological control of Meloidogyne incognita on tomato. Jordan J Agricultural Sciences. 3(3):297–309.
- Ehlers RU. 1996. Current and future use of nematodes in biocontrol: practice and commercial aspects with regard to regulatory policy issues. Biocontrol Sci Technol. 6(3):303–316. doi:10.1080/09583159631299.
- Giacometti R, d’Errico G, d’Errico FP. 2010. In vitro Nematocidal activity of the experimental formulation Tequil against Meloidogyne incognita and Heterodera daverti. Nematropica. 40:263–268.
- Glicksman M. 1963. Utilization of natural polysaccharide gums in the food industry. Adv Food Res. 11:109–200.
- Guo J, Skinner GW, Harcum WW, Barnum PE. 1998. Pharmaceutical applications of naturally occurring water-soluble polymers. PSTT. 1:254–261.
- Harman GE, Howell CR, Viterbo A, Chet I, Lorito M. 2004. Trichoderma species-opportunistic, avirulent plant symbionts. Nat Rev Microbial. 2:43–56. doi:10.1038/nrmicro797.
- Howell CR. 2003. Mechanisms employed by Trichoderma species in the biological control of plant diseases: the history and evolution of current concepts. Plant Dis. 87(1):4–10. doi:10.1094/PDIS.2003.87.1.4.
- Kök MS, Hill SE, Mitchell JR. 1999. Viscosity of galactomannans during high temperature processing: influence of degradation and solubilisation. Food Hydrocolloid. 13:535–542. doi:10.1016/S0268-005X(99)00040-5.
- Lamichhane JR, Dachbrodt-Saaydeh S, Kudsk P, Messéan A. 2016. Toward a reduced reliance on conventional pesticides in European agriculture. Plant Dis. 100(1):10–24. doi:10.1094/PDIS-05-15-0574-FE.
- Landi S, d’Errico G, Roversi PF, d’Errico FP. 2018. Management of the root-knot nematode Meloidogyne incognita on tomato with different combinations of nematicides and a resistant rootstock: preliminary data. Redia. 101:47–52.
- Lombardi N, Vitale S, Turrà D, Reverberi M, Fanelli C, Vinale F, Marra R, Ruocco M, Pascale A, d’Errico G, et al. 2018. Root exudates of stressed plants stimulate and attract Trichoderma soil fungi. MPMI. 31(10):982–994. doi:10.1094/MPMI-12-17-0310-R.
- Lorito M, Woo SL, Harman GE, Monte E. 2010. Translational research on Trichoderma: from ‘omics to the field. Annu Rev Phytopathol. 48:395–417. doi:10.1146/annurev-phyto-073009-114314.
- Malafaya PB, Silva GA, Reis RL. 2007. Natural-origin polymers as carriers and scaffolds for biomolecules and cell delivery in tissue engineering applications. Adv Drug Deliv. 59:207–233. doi:10.1016/j.addr.2007.03.012.
- Marra R, Lombardi N, d’Errico G, Troisi J, Scala G, Vinale F, Woo SL, Bonanomi G, Lorito M. 2019. Application of Trichoderma strains and metabolites enhances soybean productivity and nutrient content. J Agric Food Chem. 67(7):1814–1822. doi:10.1021/acs.jafc.8b06503.
- Marra R, Vinale F, Cesarano G, Lombardi N, d’Errico G, Crasto A, Mazzei P, Piccolo A, Incerti G, Woo SL, et al. 2018. Biochars from olive mill waste have contrasting effects on plants, fungi and phytoparasitic nematodes. PloS One. 13(6):e0198728. doi:10.1371/journal.pone.0198728.
- Mocali S, Landi S, Curto G, DellaValle E, Infantino A, Colzi C, d’Errico G, Roversi P, D’Avino L, Lazzeri L. 2015. Resilience of soil microbial and nematode communities after biofumigant treatment with defatted seed meals. Ind Crops Prod. 75(A):79–90. doi:10.1016/j.indcrop.2015.04.031.
- Neukom H. 1989. Galactomannans: properties and applications. In: Neukom H, editor. Lebensmmitel Wissenschaft und Technologie. Vol. 22. Oxford (UK): Elsevier; p. 41–45.
- Perepelkin KE. 2005. Polymeric materials of the future based on renewable plant resources and biotechnologies: fibres, films, plastics. Fibre Chem. 37:417–430. doi:10.1007/s10692-006-0014-3.
- Pérez-Piqueres A, Edel-Hermann V, Alabouvette C, Steinberg C. 2006. Response of soil microbial communities to compost amendments. Soil Biol Biochem. 38(3):460–470. doi:10.1016/j.soilbio.2005.05.025.
- Peters A, Backes J. 2003. Impact of substrate conditions and application method on the efficacy of Steinernema feltiae. IOBC WPRS Bull. 26(1):151–158.
- Reid JSG, Edwards ME. 1995. Galactomannans and other cell wall storage polysaccharides in seeds. In: Stephen AM, editor. Food polysaccharides and their application. New York: Marcel Dekker Inc; p. 155–186.
- Robinson G, Simon BR, Edwin RM. 1982. Viscosity-molecular weight relationships, intrinsic chain flexibility, and dynamic solution properties of guar galactomannan. Carbohyd Res. 107:17–32. doi:10.1016/S0008-6215(00)80772-7.
- Ruiz-de-La-Cruz G, Aguirre-Mancilla CL, Godínez-Garrido NA, Osornio-Flores NM, Torres-Castillo JA. 2017. Chitosan mixed with beneficial fungal conidia or fungicide for bean (phaseolus vulgaris l.) seed coating. Interciencia. 42(5):307–312.
- Ruocco M, Lanzuise S, Vinale F, Marra R, Turrà D, Woo SL, Lorito M. 2009. Identification of a new biocontrol gene in Trichoderma atroviride: the role of an ABC transporter membrane pump in the interaction with different plant-pathogenic fungi. Mol Plant Microbe Inter. 22(3):291–301. doi:10.1094/MPMI-22-3-0291.
- Satturwar PM, Fulzele SV, Dorle AK. 2003. Biodegradation and in vivo biocompatibility of rosin: a natural film-forming polymer. AAPS Pharm Sci Tech. 4:1–6. doi:10.1208/pt040455.
- Schroer S, Ziermann D, Ehlers RU. 2005. Mode of action of a surfactant/polymer formulation to support performance of the entomopathogenic nematode Steinernema carpocapsae for control of diamondback moth larvae (Plutella xylostella). Biocon Sci Technol. 15(6):601–613. doi:10.1080/09583150500088694.
- Sharon E, Bar-Eyal M, Chet I, Herrera-Estrella A, Kleifeld O, Spiegel Y. 2001. Biological control of the root-knot nematode Meloidogyne javanica by Trichoderma harzianum. Phytopathol. 91:687–693. doi:10.1094/PHYTO.2001.91.7.687.
- Sharon E, Chet I, Viterbo A, Bar-Eyal M, Nagan H, Samuels GJ, Spiegel Y. 2007. Parasitism of Trichoderma on Meloidogyne javanica and role of the gelatinous matrix. Eur J Plant Pathol. 118(3):247–258. doi:10.1007/s10658-007-9140-x.
- Sokhandani Z, Moosavi MR, Basirnia T. 2016. Optimum concentrations of Trichoderma longibrachiatum and cadusafos for controlling Meloidogyne javanica on Zucchini plants.. J Nematol. 48(1):54. doi:10.21307/jofnem-2017-009.
- Soppelsa O, Giacometti R, d’Errico G, D’Alessio M. 2011. Effectiveness of soil solarization combined with a plant-derived formulation for the control of the root-knot nematode Meloidogyne incognita (Kofoid et White) Chitw. in greenhouse tomato. Redia. 44:163–166.
- Spiegel Y, Chet I. 1998. Evaluation of Trichoderma spp. as a biocontrol agent against soilborne fungi and plant-parasitic nematodes in Israel. Integrated Pest Manag Rev. 3(3):169–175. doi:10.1023/A:1009625831128.
- Tefera T, Vidal S. 2009. Effect of inoculation method and plant growth medium on endophytic colonization of sorghum by the entomopathogenic fungus Beauveria bassiana. BioControl. 54:663–669. doi:10.1007/s10526-009-9216-y.
- Vinale F, Nigro M, Sivasithamparam K, Flematti G, Ghisalberti EL, Ruocco M, Varlese R, Marra R, Lanzuise S, Eid A, et al. 2013. Harzianic acid: a novel siderophore from Trichoderma harzianum. FEMS Microbiol Let. 347(2):123–129.
- Vinale F, Sivasithamparam K, Ghisalberti EL, Marra R, Woo SL, Lorito M. 2008. Trichoderma-plant-pathogen interactions. Soil Biol Biochem. 40(1):1–10. doi:10.1016/j.soilbio.2007.07.002.
- Woo SL, Donzelli B, Scala F, Mach R, Harman GE, Kubicek CP, Del Sorbo G, Lorito M. 1999. Disruption of the ech42 (endochitinase-encoding) gene affects biocontrol activity in Trichoderma harzianum P1. Mol Plant Microbe Inter. 12(5):419–429. doi:10.1094/MPMI.1999.12.5.419.
- Woo SL, Ruocco M, Vinale F, Nigro M, Marra R, Lombardi N, Pascale A, Lanzuise S, Manganiello G, Lorito M. 2014. Trichoderma-based products and their widespread use in agriculture. Open Mycol J. 8(1):71–126. doi:10.2174/1874437001408010071.
- Zasada IA, Halbrendt JM, Kokalis-Burelle N, LaMondia J, McKenry MV, Noling JW. 2010. Managing nematodes without methyl bromide. Annu Rev Phytopathol. 48:311–328. doi:10.1146/annurev-phyto-073009-114425.