Abstract
Symptoms of ringspots and vein-clearing were observed on wasabi (Wasabia japonica (Miq) Matsum) plants in three greenhouses in British Columbia during 2017. Ten indicator plant species, including four Nicotiana species, were inoculated with sap extracts from symptomatic leaves; after 4–11 days, necrotic lesions developed on all plants. Transmission electron microscopy revealed rod-shaped virions, 250–300 nm in length, in leaves of N. occidentalis and N. clevelandii. Total RNA from symptomatic wasabi tissues was used in RT-PCR with universal primers corresponding to five virus genera and specific primers for turnip ringspot virus and alfalfa mosaic virus. Amplicons ~400 bp in size were obtained with tobamovirus primer set TobN up3/TobN do4 and amplicons of ~300 and ~600 bp were obtained with ilarvirus primer set Ilar1F5/Ilar1R7. Sequencing and MegaBLAST (NCBI) query of the Ilar amplicons showed 99% identity to wasabi mottle virus (WMoV), a member of the genus Tobamovirus. Next-generation Sequencing confirmed WMoV as the only virus present in diseased plants. BC isolates (GenBank accession no. MK431779) showed 99.43% sequence identity to isolate ‘Alishan’ (GenBank accession no. KJ207375.1) and 98.62% identity to ‘Tochigi’ strain (GenBank accession no. AB017504.1). Mechanical inoculation of wasabi cultivars ‘Green Thumb’ and ‘Daruma’ produced ringspots and vein-clearing symptoms after 22–23 days on the former while the latter was asymptomatic, but WMoV was detected in all plants by RT-PCR. WMoV may have been introduced into Canada on imported infected ‘Green Thumb’ plants and subsequently spread through commercial vegetative propagation. The effects on yield or rhizome quality are yet unknown.
Résumé
En 2017, des symptômes de tache annulaire et d’éclaircissement des nervures ont été observés sur des plants de wasabi (Wasabia japonica [Miq] Matsum) dans trois serres en Colombie-Britannique (C.-B.). Dix plantes indicatrices, dont quatre espèces de Nicotiana, ont été inoculées avec des extraits de sève provenant de feuilles symptomatiques. Au bout de 4 à 11 jours, des lésions nécrotiques sont apparues sur tous les plants. La microscopie électronique à transmission a révélé des virions de forme allongée de 250 à 300 nm de longueur dans les feuilles de N. occidentalis et de N. clevelandii. L’ARN total provenant des tissus symptomatiques de wasabi a été utilisé pour une analyse par PCR en temps réel avec des amorces universelles correspondant à cinq genres de virus et d’amorces spécifiques du virus de la tache annulaire du navet ainsi que du virus de la mosaïque de la luzerne. Des amplicons d’environ 400 bp ont été obtenus avec le jeu d’amorces du tobamovirus, TobN up3/TobN do4, et des amplicons d’environ 300 bp et 600 bp ont été obtenus avec le jeu d’amorces de l’ilarvirus, Ilar1F5/Ilar1R7. Le séquençage et la requête MegaBLAST (NCBI) des amplicons Ilar ont montré 99% de similitude avec le virus de la marbrure du wasabi (VMaW), un membre du genre Tobamovirus. Le séquençage de nouvelle génération a confirmé le VMaW en tant que seul virus trouvé dans les plants infectés. Les isolats de la C.-B. (no d’obtention de la GenBank: MK431779) ont montré une identité de séquence de 99,43% par rapport à l’isolat ‘Alishan’ (no d’obtention de la GenBank: KJ207375.1) et de 98,62% par rapport à la souche ‘Tochigi’ (no d’obtention de la GenBank: AB017504.1). L’inoculation mécanique des cultivars de wasabi ‘Green Thumb’ et ‘Daruma’ a produit les symptômes de la tache annulaire et de l’éclaircissement des nervures sur le premier au bout de 22 à 23 jours, tandis que le second était asymptomatique, mais le VMaW y a quand même été détecté dans tous les plans par PCR en temps réel. Le VMaW a probablement été introduit au Canada sur des feuilles importées de ‘Green Thumb’ infectées et, par la suite, disséminé par multiplication végétative commerciale. Les effets du virus sur le rendement ou la qualité des rhizomes ne sont pas encore connus.
Introduction
Wasabia japonica (Miq.) Matsumura (syn. Eutrema japonicum Matsum.), or wasabi, is a member of the Brassicaceae family and is native to Japan, where it has been cultivated since the 10th century (Hodge Citation1974). Although wasabi can grow as a perennial plant, for commercial production it is harvested after 12–18 months. The valuable rhizome is typically used to flavour traditional Japanese dishes (Chadwick et al. Citation1993). Although wasabi is primarily cultivated in Japan and Taiwan (Hodge Citation1974; Chadwick et al. Citation1993), production has increased in Korea (Park et al. Citation2016), New Zealand (Douglas and Follett Citation1992), the United States (Chadwick et al. Citation1993), Australia (Wilson Citation1998), and Canada (Rodríguez and Punja Citation2007).
Wasabi production in British Columbia (BC) occurs in the southern coastal region in polyethylene or glass greenhouses. Plants are grown using hydroponic or semi-hydroponic systems in river rock substrate, and usually with overhead misters to maintain humid conditions. Typically, plants are initiated from vegetative offshoots collected from mother (stock) plants to reduce production costs; however, plantlets derived from tissue-cultured plant materials are also commercially available. The three cultivars currently grown in BC are ‘Mazuma’, ‘Daruma’, and ‘Green Thumb’; the latter of which, introduced from Taiwan, has been present in BC since at least 2008. ‘Green Thumb’ has gained popularity despite its susceptibility to a number of recently reported fungal pathogens (MacDonald and Punja Citation2017).
Fungal diseases are a major concern for wasabi producers due to the humid-growing conditions and the use of vegetatively propagated planting materials that can harbour and spread pathogens (Rodríguez and Punja Citation2007, Citation2009; MacDonald and Punja Citation2017; Punja et al. Citation2017). Surveys of wasabi greenhouses in BC have shown the widespread occurrence of several diseases caused by fungi and oomycetes (Betz et al. Citation2016). Several viruses have also been reported to infect wasabi crops in New Zealand (Fletcher Citation1989), Japan (Chadwick et al. Citation1993), Australia (Wilson Citation1998), Korea (Kim and Lee Citation1999), and Taiwan (Deng et al. Citation2016). These previously recognized viruses include alfalfa mosaic virus (AMV) (Fletcher Citation1989), turnip mosaic virus (TuMV) (Chadwick et al. Citation1993), tobacco mosaic virus (TMV) (Chadwick et al. Citation1993; Kim and Lee Citation1999), cucumber mosaic virus (CMV) (Wilson Citation1998), and wasabi mottle virus (WMoV) (Deng et al. Citation2016). Although the aphid species that have the potential to act as virus vectors have been identified on wasabi in BC (MacDonald et al. Citation2017), no viruses have been identified on wasabi in North America prior to the preliminary report associated with this study (MacDonald et al. Citation2019).
Isolates of the species Wasabi mottle virus (family: Virgaviridae; genus: Tobamovirus) have recently been reported to infect wasabi plants, causing symptoms of rugose and yellow mosaic in Taiwan (Deng et al. Citation2016). This virus was previously known as the wasabi strain of TMV or the wasabi strain of crucifer tobamovirus, and is part of the ‘Ribgrass mosaic virus’ subgroup 3, along with the species Ribgrass mosaic virus (RMV), Turnip vein clearing virus (TVCV), and Youcai mosaic virus (YoMV) (Heinze et al. Citation2006). Members of the Tobamovirus genus have a single stranded (+) sense genomic RNA in the range of 6.3–6.6 kb in length that typically encodes at least four separate proteins. Tobacco mosaic virus, the type member, has two proteins involved in replication of the virus that are produced directly from the genomic RNA, the larger of which is produced by a read through of the amber stop codon (UAG) that normally terminates the smaller replication-associated protein. Two other viral proteins are generated from individual 3’ co-terminal subgenomic RNAs, giving rise to the non-structural movement protein (MP) and the viral coat protein (CP) (Zaitlin Citation1999).
In 2017, symptoms of putative virus infection observed as vein-clearing and ringspots () were observed on wasabi plants grown in a production greenhouse located in the Fraser Valley of BC. This study was conducted to: (i) identify the possible virus(es) present in symptomatic plants through transmission studies, electron microscopy, PCR-based detection, and next-generation sequencing; (ii) develop a PCR-based diagnostic method to identify infected plants; and (iii) conduct a survey of the distribution of the disease in different wasabi growing regions in BC.
Materials and methods
Disease symptoms and sample collection
In July 2017, a commercial wasabi production facility in southern coastal BC was observed to have ‘Green Thumb’ plants that showed striking symptoms of ringspots and vein-clearing (). Representative symptomatic leaf samples from four plants were collected for further study.
Transmission studies
A mechanical transmission study to select indicator host plants was conducted by sap inoculations using symptomatic wasabi tissue. Ten indicator species were inoculated: Nicotiana benthamiana Domin (Australian tobacco), N. clevelandii A. Gray (Cleveland’s tobacco), N. glutinosa L. (Peruvian tobacco), N. occidentalis H.-M. Wheeler (native tobacco), N. tabacum L. ‘Samsun’ (cultivated tobacco), Chenopodium quinoa Willd. (quinoa), C. amaranticolor (Coste & A.Reyn.) Coste & A.Reyn. (goosefoot), Gomphrena globosa L. (globe amaranth), Brassica rapa var. perviridis L.H. Bailey, 1930 (‘Tender Green’) and Tetragonia tetragonioides (Pall.) Kuntze, 1891 (New Zealand spinach). Leaves of all plants were dusted with 320 grit carborundum (Thermo Fisher Scientific, Waltham, MA, USA) and rubbed with a leaf slurry prepared by grinding 0.5–1 g of tissue in 1–2 mL 50 mM sodium phosphate buffer (PO4) pH 7.5. Inoculated and control plants (n = 3–5 for each set of inoculated or control plants per species) were maintained in a greenhouse compartment, and observed daily for symptoms.
To establish the transmission of the putative virus to wasabi plants, tissue-cultured ‘Daruma’ and ‘Green Thumb’, presumed to be free of virus and obtained from a local nursery, were inoculated as described above. For each cultivar, five plants were inoculated and five were left as the non-inoculated control and maintained in the greenhouse compartment. Plants were assessed regularly (every 1–3 days) for symptoms. Inoculation experiments were repeated twice with ‘Green Thumb’, and once for ‘Daruma’ using freshly collected symptomatic wasabi leaves.
Transmission electron microscopy
A sap sample from leaf tissues from each of N. clevelandii and N. occidentalis was collected 5–10 days after mechanical sap inoculation and freeze-dried. As well, symptomatic W. japonica plant material collected from a commercial greenhouse was included. Brandes’ dip (Brandes Citation1957) and preparation method for crude leaf extracts was performed as per Hitchborn and Hills (Citation1965).
The prepared sample was observed with a Hitachi H-7100 Transmission Electron Microscope (TEM) (100 kv) and imaged in Gatan Digital Micrograph software (v. 2.31.734.0; Gatan Inc., Pleasanton, CA, USA). Virions were searched for systematically from top to bottom, from left to right. Preliminary searches were done at 5,000–6,000 X magnification, and increased to 30,000–40,000 X magnification for imaging and measurement of individual virions. Fifty virions were imaged from each host sample, and the length and diameter of each was measured in the Gatan Digital Micrograph software.
Molecular detection and sequencing
Symptomatic wasabi leaf tissue was used for total RNA extraction with an RNeasy Plant Mini Kit (Qiagen Sciences, Germantown, MD, USA). A small portion of extracted RNA was converted to cDNA using the two-step RT-PCR system, iScriptTM Select cDNA Synthesis Kit (Bio-Rad Laboratories Ltd., Mississauga, ON, Canada). PCR was conducted on the cDNA samples generated using degenerate broad-spectrum primer sets (Supplementary Table 1) designed to detect the presence of viruses in the genera Tobamovirus, Nepovirus, Potyvirus, Ilarvirus, and Cucumovirus, and the species Turnip ringspot virus and Alfalfa mosaic virus. The amplified PCR products were purified with the MinElute PCR Purification Kit (Qiagen Sciences, Germantown, MD, USA), and plasmid clones were constructed using the Qiagen PCR Cloning Kit (Qiagen Sciences, Germantown, MD, USA) following the manufacturer’s recommended protocol. The recombinant plasmids were transformed into Invitrogen DH5α competent E. coli as per the manufacturer’s recommended conditions utilizing heat shock methodologies, followed by spread plating two dilutions of sample onto LB agar plates containing ampicillin and X-gal. To pre-select for transformed colonies, LB agar plates were used that contained both 0.1 mg/mL ampicillin, and overlaid with 40 uL of 40 mg/mL X-Gal to allow for blue/white screening with the pDrive cloning vector (Qiagen Sciences, Germantown, MD, USA). Ten single white colonies from each isolate plate were selected the next day to be cultured in 4.5 mL of liquid LB with 0.1 mg/mL ampicillin, and incubated in a shaker at 37°C for ~18 hr. In addition, selected colonies were PCR screened for inserts by dipping the pipet tip used to inoculate each culture tube into 25 uL of a PCR reaction mix containing universal primers (T7 and SP6) and DreamTaq (Invitrogen, Waltham, MA, USA).
The plasmid DNA from cultures was purified using Qiagen’s QIAprep Spin Miniprep Kit following the manufacturer’s recommended protocol. Sample concentrations were estimated by measuring absorbance at 260 nm using spectrophotometry. For each host sample (naturally infected W. japonica, mechanically infected B. rapa var. perviridis ‘Tendergreen’, and mechanically infected N. occidentalis), 3–5 clones were miniprepped and processed. Plasmid preps were sent to the University of British Columbia – Nucleic Acid Protein Service (UBC-NAPS; UBC, Vancouver, BC, Canada) for sequencing, and the sequence data were then subjected to a MegaBLAST (NCBI; https://blast.ncbi.nlm.nih.gov/) search for analysis.
Next-generation sequencing
Approximately one gram of leaf tissue was collected for sequencing of the viral genome from a naturally infected W. japonica leaf sample. Extraction of viral double-stranded RNA (dsRNA) intermediates, removal of genomic DNA and single-stranded RNA, and construction of cDNA library were done as described by Su et al. (Citation2015). The cDNA library was paired-end sequenced on a HiSeq 2500 platform (Illumina) by the Centre for Applied Genomics, the Hospital for Sick Children (Toronto, ON, Canada). Sequence reads were trimmed to remove low quality and adaptor sequences and assembled using the de novo assembly algorithm of CLC Genomics Workbench 11 (Qiagen Sciences, Germantown, MD, USA).
Development of a PCR-based diagnostic test for virus detection
Using the full-length sequence data from this study as well as that for isolate ‘Alishan’ from Taiwan available in GenBank (KJ207375.1), which were 99.43% similar, a primer set was designed to detect the virus with RT-PCR. Wasabi virus primer wv-F1(aacaccatcatcatcgctgc) and wv-R1(ttaggcttgtacgacacggt) were selected by Primer 3 (available from https://github.com/primer3-org) at positions 4380 bp and 4887 bp. Symptomatic leaves from wasabi inoculation studies were collected and subjected to RT-PCR analysis using the conditions described below to confirm the presence/absence of the virus in inoculated/control tissues.
Three commercial wasabi facilities were sampled in BC during 2017–2018 to determine the presence of diseased plants based on the symptoms shown in . At least 7–9 plants were collected at each facility. In addition, in one facility cultivating ‘Green Thumb’, leaves with mild chlorotic areas () or that were asymptomatic were sampled randomly by removing one leaf from each plant. A total of 75 plants located in five adjacent rows were sampled. All samples were subjected to RNA extraction and RT-PCR analysis.
Fig. 1 Early symptoms of wasabi mottle virus development on wasabi leaves from natural infection. (a) Small chlorotic spots. (b, c) Chlorotic streaks developing along leaf veins. (d) Ringspot
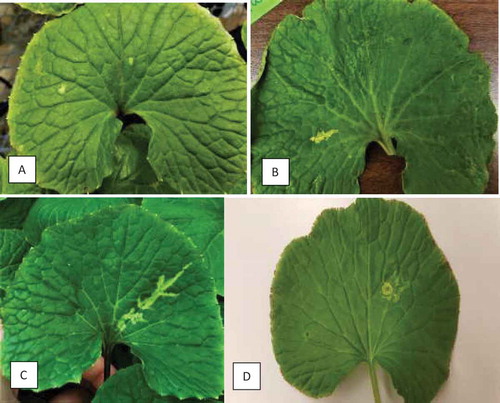
Total RNA was extracted from wasabi leaves using the QIAGEN RNA extraction kit (RNeasy Plant Mini Kit ref: 74903). For RT-PCR, the iScript cDNA synthesis kit (Bio-Red cat. no. 1708891) was used to obtain cDNA. The PCR utilized 2 uL cDNA in a total 25 uL reaction using QIAGEN Taq DNA polymerase (cat. no. 201205). Cycling conditions were 94°C for 3 min, 94°C for 30 sec, 55°C for 45 s, 72°C for 2 min (40 cycles), then 72°C for 7 min. Phylogenetic analysis was conducted using MEGA 7.0 software. A neighbour-joining tree was constructed with a 480 bp alignment of the sequences generated from two different samples of wasabi leaves and compared to those of wasabi mottle virus and other related viruses deposited in GenBank (). The tree represents 1,000 bootstrap values.
Results
Symptom development
Symptoms observed on naturally infected W. japonica in a commercial greenhouse included ringspots, small necrotic spots, and vein-clearing (). Typically, the progression of symptoms began with small bleached spots, and progressed to small areas of localized vein-clearing (), and finally to ringspots and to more extensive vein-clearing (). On rare occasions, necrotic symptoms were observed on severely diseased leaves (). In general, symptoms were more pronounced on larger, mature leaves and rarely seen on young actively growing leaves. Symptom development was noted during sampling conducted from June to August, and was less commonly found in May and September or at other times during the year.
Fig. 2 Advanced stages of natural infection by wasabi mottle virus on wasabi leaves. (a, b) Patterns of ringspots. (c) Ringspots and necrotic spots developing on leaves. (d) Extensive chlorotic streaks and vein-clearing. (e) Necrotic ringspots, vein clearing and extensive chlorosis. (f) Healthy leaf
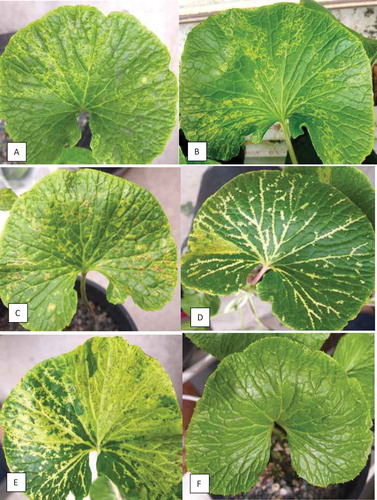
Transmission studies
On mechanically inoculated W. japonica plants, weak ringspot symptoms were first observed 22 days after inoculation on cultivar ‘Green Thumb’ only. Chlorotic spots () and ringspots () developed on these plants, but not in the non-inoculated controls. One inoculated ‘Green Thumb’ plant died and was excluded from the experiment. Of the remaining four inoculated plants, 66% of leaves exhibited chlorotic spots and 58% had ringspots to some degree at 39 days post inoculation (dpi); symptoms were minor and not as visually striking as those observed on plants found in commercial greenhouses. Ringspots were noted on a single ‘Daruma’ leaf at 43 dpi, but symptoms did not develop further. In the second inoculation trial on ‘Green Thumb’, chlorotic spots and ringspots developed 23 dpi. Mechanical inoculations using tissues from inoculated symptomatic W. japonica onto N. tabacum ‘Samsun’ resulted in rapidly forming, coalescing lesions and necrosis that was much more severe compared to the initial inoculations made onto N. tabacum ‘Samsun’ using naturally infected tissues. No control plants showed symptoms.
Fig. 3 Symptoms following artificial inoculation of wasabi mottle virus on wasabi leaves. (a) Chlorotic spot. (b) Ringspot. (c) Necrotic ringspot 22 days after mechanical inoculation
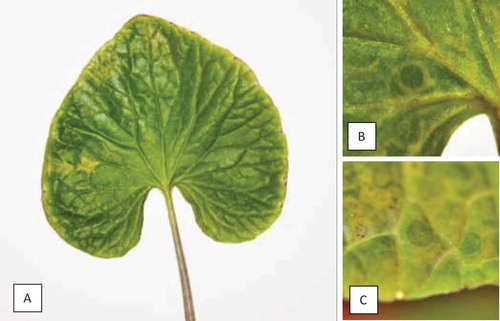
Nicotiana clevelandii, N. occidentalis, N. glutinosa, and N. tabacum ‘Samsun’ exhibited localized lesions, 2–3 mm in diameter, four days after inoculation. In N. occidentalis, lesions coalesced, and by 11 days after inoculation, necrosis along main veins was apparent and leaves were necrotic and/or dead (). Nicotiana benthamiana showed mild leaf curling abaxially, and puckering of leaves. Gomphrena globose (), T. tetragonioides (), C. quinoa () and C. amaranticolor showed localized lesions after four days; C. amaranticolor tended to have many more lesions than C. quinoa. B. rapa var. perviridis ‘Tendergreen’ developed vein banding, chlorosis and mild puckering after four days.
Transmission electron microscopy
Transmission electron microscopy of inoculated N. occidentalis and N. clevelandii leaves revealed the presence of rod-shaped virions bisected by a canal (). On average, particles measured 257 nm (56–438 nm) × 21 nm (13–35 nm). No other virus-like particles were present.
Molecular detection and sequencing
The broad-spectrum primer set for Ilarvirus, Ilar1F5/Ilar1R7 (Untiveros et al. Citation2010), amplified two distinct amplicons, approximately 300 bp and 600 bp, in test samples. The two amplicons were present in each of the naturally infected W. japonica plants, as well as the artificially inoculated N. occidentalis, and B. rapa var. perviridis (‘Tender Green’) samples. Sequencing of these two amplicons revealed that the smaller fragment was completely nested within the larger one; Ilar1F5, was able to anneal in two locations and generate the two amplicons (specifically 533 bp and 261 bp). The sequence data of the 533 bp amplicons derived from the three different plant species was virtually identical. Using MegaBLAST (NCBI) to search the NCBI database with the sequence data obtained from the 533 bp amplicons, these Ilarvirus primer amplicons actually corresponded to a Tobamovirus, wasabi mottle virus. Over this amplified region, the British Columbia (BC) isolate shared 99.44% sequence identity to WMoV isolate ‘Alishan’ (GenBank Accession no. KJ207375.1), 99.25% sequence identity to WMoV isolate ‘Shizuoka’ (GenBank Accession no. AB017503.1), and 97.94% identity to WMoV isolate ‘Tochigi’ (GenBank Accession no. AB017504.1).
Next-generation sequencing
Next-generation sequencing revealed that WMoV was the only plant virus present in the sample. The genome sequence of WMoV isolate SFU2 was determined to be 6297 bp in length, excluding the 3’ poly-A tail (GenBank accession no. MK431779). The nucleotide sequence showed high similarity to the genome of WMoV isolates deposited in the GenBank, including 99.7% identical to isolate ‘pLX-WMoV6.3’ from Taiwan (GenBank accession no. MH200607), 99.4% to isolate ‘Alishan’, (Taiwan, KJ207375), 99.4% to isolate ‘Shizuoka’ (Japan, AB017503), and 98.6% to isolate ‘Tochigi’ (Japan, AB017504). The viral RNA genome organization closely resembles tobamoviruses, encoding four proteins (King et al. Citation2011). The 5’ proximal open reading frame (ORF) is from nucleotide (nt) 66 to 3377, encoding a peptide of 1103 amino acids (aa) with a calculated molecular mass of 124.6 kDa. The peptide contains a viral methyltransferase domain (pfam01660, aa 50–435, E-value 5.42E-56) and a viral RNA helicase domain (pfam01443, aa 820–1072, E-value 1.65E-60). A read through of the amber stop codon (UGA) of this ORF produces a protein with 1597 aa residues (181.3 kDa), serving as the viral RNA polymerase. In addition to the viral methyltransferase domain and RNA helicase domain, as described in the 124.6 kDa, the 3’ end of the 181.3 kDa protein contains the conserved viral RNA-dependent RNA polymerase (RdRp) superfamily 2 domain (pfam00978, aa 1155–1595, E-value 0E+00). Both 124.6 kDa and 181.3 kDa proteins are involved in RNA replication. The third ORF (nt 4862–5665) encodes the viral movement protein (267 aa, 29.9 kDa), containing TMV “30K”-like cell-to-cell MP domain (pfam01107, aa 10–175, E-value 7.70E-24). The fourth ORF (nt 5589–6062) encodes a peptide of 17.6 kDa (157 aa), which is the sub-unit of the viral coat protein, containing TMV-like coat protein domain (TMV_coat, pfam00721, aa 14–146, E-value 3.12E-10).
Development of a PCR diagnostic test
Utilizing the primer set designed from full-length sequences, inoculated and symptomatic ‘Green Thumb’ plants consistently tested positive and showed a 545 bp size band when subjected to RT-PCR (). With one exception, non-inoculated control plants remained asymptomatic and were negative for WMoV; one ‘Green Thumb’ control plant was positive. All inoculated ‘Daruma’ plants were positive for WMoV and remained asymptomatic except for one leaf on a single plant that showed a single ringspot.
Fig. 6 Detection of wasabi mottle virus with RT-PCR using primers designed from the full-length sequence. (a) Lanes 1–13 are from symptomatic naturally infected plants; C = control, L = molecular weight ladder. (b) Lanes A to G are from mechanically inoculated plants; C = control, L = molecular weight ladder. (c) Samples originating from 14 asymptomatic ‘Green Thumb’ plants show presence of the virus; L = molecular weight ladder. (d) Samples originating from 13 ‘Daruma’ plants show presence of the virus; C = control, L = molecular weight ladder
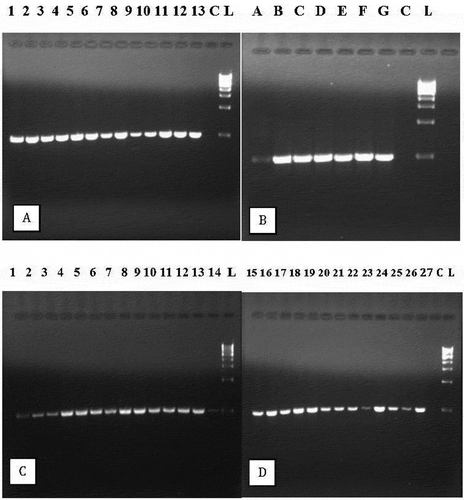
All three wasabi greenhouses sampled during 2017–2018 showed presence of WMoV by RT-PCR. In two facilities, 2 out of 7 and 3 out of 9 plants were positive. In a third facility, leaves with mild chlorotic areas or that were asymptomatic were sampled from 75 plants of ‘Green Thumb’ and tested by RT-PCR (). Among these, 38% of the plants were found to be positive for the virus; the remainder tested negative.
Phylogenetic analysis confirmed the close relationship of the WMoV BC isolates to isolate ‘Alishan’ (GenBank Accession no. KJ207375.1) and isolate ‘Tochigi’ (GenBank Accession no. AB017504.1), confirming the results from sequence analysis ().
Fig. 7 Phylogenetic tree of wasabi mottle virus sequences from two different leaf samples in BC compared with sequences from GenBank. Number of bootstrap support values ≥50% based on 1000 replicates. Abbreviations are: WmoV = Wasabi mottle virus; Ctbv = Crucifer tobamovirus; Ribgrass msV = Ribgrass mosaic virus; TvcV = Turnip vein-clearing virus; YmsV = Youcai mosaic virus. The outgroup was Tobacco mosaic virus.
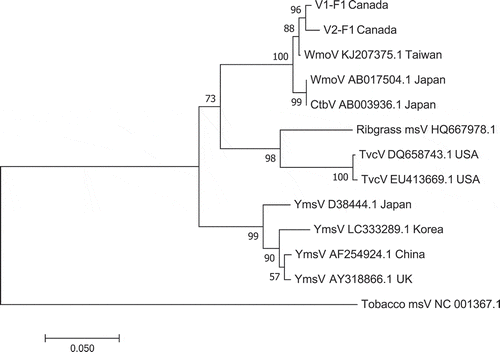
Discussion
The wasabi mottle virus was first identified on wasabi plants in Taiwan (Deng et al. Citation2016), where diseased plants of an unreported cultivar showed rugose and yellow mosaic symptoms. In our study, symptomatic plants in wasabi greenhouses showed marked symptoms of ringspots and vein-clearing. These differences in symptomology may be due to the wasabi cultivar, environmental factors such as temperature, or potentially variation in aggressiveness or titre of strains of the virus. The most susceptible cultivar on which predominant symptoms were observed in this study was the Taiwanese cultivar, ‘Green Thumb’. The high sequence similarity between the BC strain of WMoV and strains from Taiwan and Japan suggests that imported plant material may have contained WMoV; however, further investigation is required in order to determine the source.
The presence of WMoV on wasabi plants in three greenhouses in BC suggests it may have been present for some time and remained undiagnosed. Rod-shaped particles that are typical of tobamoviruses were observed using transmission electron microscopy in diseased tissues, supporting RT-PCR and NGS results. Incidence of disease symptoms was lower on the cultivar ‘Daruma’ when compared to ‘Green Thumb’. The cultivar ‘Daruma’, despite testing positive for the virus by RT-PCR, did not display symptoms except for one plant, and harboured a high titre of the virus. This could potentially also have provided a source of inoculum for spread onto ‘Green Thumb’ plants, as tobamoviruses are readily transmitted through mechanical means (Adams et al. Citation2017), and wasabi cultivation involves constant pruning and removal of leaves in addition to vegetative propagation. The high frequency of distribution (38%) of infected plants within one commercial facility suggests that mechanical spread is likely taking place. After inoculation, symptom development required at least three weeks to manifest, demonstrating that infected plants can remain asymptomatic for a period of time. The most striking symptoms were observed in samples obtained during June–August, suggesting that higher-ambient temperatures (sometimes exceeding 35°C) could be more conducive to symptom expression, as is observed with other viruses (Llamas-Llamas et al. Citation1998). Wasabi growth is also inhibited at these temperatures, and the plant is generally weaker. Further research is required to establish the effects of temperature, time of inoculation and plant age on symptom expression.
Tobamoviruses, as a group, show a range of symptoms on a range of host plants, which include mottling of leaves, mosaic, spots, ringspots or necrotic lesions on leaves and/or fruit, curling, vein-clearing or otherwise misshaping of the leaves, stunting of entire plants, misshapen, discoloured or dropping of fruit, and, rarely, death of the entire plant. The degree of the symptoms depends on many factors, including host cultivar, plant age, virulence of the particular virus strain, and environmental factors such as temperature, light intensity, and length of the day (Lartey et al. Citation1996; Stobbe et al. Citation2012; Gibbs et al. Citation2015). Transmission often occurs through contact between plants, and sometimes through seed, but is not known to occur via insect vectors (Adams et al. Citation2017). Mechanical transmission by crop manipulation is an effective method of transmission.
While PCR amplification was observed using the primer set TobN up3/TobN do4 and Ilar1F5/Ilar1R7, sequencing of the TobN amplicon was not pursued for this study as NGS sequencing was in progress. The Ilar primer set had been designed to detect the RNA1 of ilarviruses, and was found to have specificity within the larger family Bromoviridae (Untiveros et al. Citation2010). The members within the family Bromoviridae (such as the genus Ilarvirus) are members of the ‘alpha-like’ super group, sharing sequence similarity in their 1a protein domain of the methytransferase and helicase activities with members of other genera, including the genus Tobamovirus (van Regenmortel et al. Citation2000; van der Heijden and Bol Citation2002). The Ilar primer set is highly degenerate, which may account for the successful amplification of the wasabi tobamovirus. Analysis of the 533 bp amplicon (2287nt-2819nt of MK431779.1) has it spanning from the end of the methytransferase domain into most of the helicase domain. The smaller amplicon 261bp amplicon (2558nt-2819nt of MK431779.1) would be in the helicase domain.
Tobamoviruses assemble into virions that are typically rod-shaped particles, 18 nm in diameter and around 300–310 nm in length, similar to those seen in this study, with some shorter subgenomic sized particles as a minor component in the population (Klug Citation1999). Tobamoviruses are found throughout the world, and generally have a relatively narrow host range in nature; however, many have quite a broad experimental host range, and virus can generally be found in all parts of the infected host plants (Adams et al. Citation2017).
Management of WMoV on wasabi is likely to be challenging given it is already present in at least three wasabi production greenhouses in BC and vegetative propagation of plants that could be asymptomatic could increase the problem. The utility of meristem tip culture (Rodríguez and Punja Citation2009) to propagate virus-free plants should be investigated further. The effects of WMoV infection of wasabi yield and quality of the rhizomes are unknown; however, as with many viruses, sustained virus infection in a host over a period of 18 months is likely to cause negative effects. Improved sanitation practices and development of virus-free mother plant material will be of crucial importance in managing WMoV in wasabi production greenhouses.
Supplemental Material
Download MS Word (26.4 KB)Acknowledgements
We thank the wasabi growers who participated in the disease survey. Funding was provided by Growing Forward 2 Project No. URAGF2-406 and in-kind support was provided by Agriculture and Agri-Food Canada and Simon Fraser University. We thank Mr. Michael Weis (Summerland Research and Development Centre) for providing training and assistance with transmission electron microscopy and imaging, and Mr. David Nield and David Weir (Summerland Research and Development Centre) for providing space, time and material support. Wasabi mosaic virus isolate SFU2 (CPVC accession no. 836) is maintained in Agriculture and Agri-Food Canada’s Canadian Plant Virus Collection (Summerland, BC). Lastly, we appreciate the support of Mike Bernardy, former curator of the Canadian Plant Virus Collection.
Supplementary material
Supplemental data for this article can be accessed here.
References
- Adams MJ, Adkins S, Bragard C, Gilmer D, Li D, MacFarlane SA, Wong S-M, Melcher U, Ratti C, Ryu KH, ICTV Report Consortium. 2017. ICTV virus taxonomy profile: Virgaviridae. J Gen Virol. 98:1999–2000. doi:10.1099/jgv.0.000884.
- Betz EC, MacDonald JL, Punja ZK. 2016. New and recurring pathogens of wasabi in British Columbia. Can J Plant Pathol. 38:533–534 (abstr.).
- Brandes J. 1957. Eine elektronenmikroskopische Schnellmethode zum Nachweis faden-und stäbchenförmiger Viren, insbesondere in Kartoffeldunkelkeimen. Narachrbl Deut Pflanzenschutzdienst (Braunschweig). 9:151–152.
- Chadwick CI, Lumpkin TA, Elberson LR. 1993. The botany, uses and production of Wasabia japonica (Miq.) (Cruciferae) Matsum. Econ Bot. 47:113–135. doi:10.1007/BF02862015.
- Deng T-C, Tsai C-H, Ning F-Y. 2016. Identification of Wasabi mottle virus infecting wasabi in Taiwan. J Taiwan Agri Res. 65:103–108.
- Douglas JA, Follett JM. 1992. Initial research on the production of water-grown wasabi in the Waikato. Proc Agron Soc NZ. 22:57–60.
- Fletcher JD. 1989. Additional hosts of Alfalfa mosaic virus, Cucumber mosaic virus, and Tobacco mosaic virus in New Zealand. NZ J Crop Hort Sci. 17:361–362. doi:10.1080/01140671.1989.10428057.
- Gibbs AJ, Wood J, Garcia-Arenal F, Ohshima K, Armstrong JS. 2015. Tobamoviruses have probably co-diverged with their eudicotyledonous hosts for at least 110 million years. Virus Evol. 1:vev019. eCollection 2015. doi:10.1093/ve/vev019.
- Heinze C, Lesemann D-E, Ilmberger N, Willingmann P, Adam G. 2006. The phylogenetic structure of the cluster of Tobamovirus species serologically related to Ribgrass mosaic virus (RMV) and the sequence of Streptocarpus flower break virus (SFBV). Arch Virol. 151:763–774. doi:10.1007/s00705-005-0640-8.
- Hitchborn JH, Hills GJ. 1965. Use of negative staining in electron microscopic examination of plant viruses in crude extracts. Virology. 27:528–540. doi:10.1016/0042-6822(65)90178-9.
- Hodge WH. 1974. Wasabi — native condiment plant of Japan. Econ Bot. 28:118–129. doi:10.1007/BF02861977.
- Kim H-M, Lee K-J. 1999. Characteristics of Tobacco mosaic virus isolated from wasabi (Eutrema wasabi) in Korea. Plant Pathol J. 15:247–250.
- King A, Lefkowitz E, Adams MJ, Carstens EB, editors. 2011. Virus taxonomy: ninth report of the International Committee on Taxonomy of Viruses. 1st ed. Amsterdam (Netherlands): Elsevier; p. 1338.
- Klug A. 1999. The Tobacco mosaic virus particle: structure and assembly. Phil Trans R Soc Lond B Biol Sci. 354:531–535. doi:10.1098/rstb.1999.0404.
- Lartey RT, Voss TC, Melcher U. 1996. Tobamovirus evolution: gene overlaps, recombination, and taxonomic implications. Mol Biol Evol. 13:1327–1338. doi:10.1093/oxfordjournals.molbev.a025579.
- Llamas-Llamas ME, Zavaleta-Mejia E, Gonzalez-Hernandez VA, Cervantes-Diaz L, Santizo-Rincon JA, Ochoa-Martinez DL. 1998. Effect of temperature on symptom expression and accumulation of tomato spotted wilt virus in different host species. Plant Pathol. 47:341–347. doi:10.1046/j.1365-3059.1998.00249.x.
- MacDonald JL, Betz EC, Li YQ, Punja ZK, Bouthillier MJ, DeYoung RM, Bernardy MG. 2019. First report of ringspot and vein-clearing symptoms on Wasabia japonica plants associated with Wasabi mottle virus in North America. Can J Plant Pathol. 41:509 (abstr.).
- MacDonald JL, Maw E, Clarke P. 2017. First identifications of aphid and diamondback moth populations on wasabi in British Columbia. J Entomol Soc BC. 114:93–96.
- MacDonald JL, Punja ZK. 2017. Occurrence of botrytis leaf blight, anthracnose leaf spot, and white blister rust on Wasabia japonica in British Columbia. Can J Plant Pathol. 39:60–71. doi:10.1080/07060661.2017.1304021.
- Park YH, Moon YG, Cho SE, Shin HD. 2016. First report of powdery mildew caused by Erysiphe cruciferarum on wasabi (Wasabia japonica) in Korea. Plant Dis. 100:530. doi:10.1094/PDIS-08-15-0877-PDN.
- Punja ZK, Chandanie WA, Chen X, Rodríguez G. 2017. Phoma leaf spot of wasabi (Wasabia japonica) caused by Leptosphaeria biglobosa. Plant Pathol. 66:480–489. doi:10.1111/ppa.12589.
- Rodríguez G, Punja ZK. 2007. Root infection of wasabi (Wasabia japonica) by Pythium species. Can J Plant Pathol. 29:79–83. doi:10.1080/07060660709507440.
- Rodríguez G, Punja ZK. 2009. Vascular blackening of wasabi rhizomes caused by Pectobacterium carotovorum subsp. carotovorum. Eur J Plant Pathol. 124:483–493. doi:10.1007/s10658-009-9435-1.
- Stobbe AH, Melcher U, Pamer MW, Roossinck MJ, Shen G. 2012. Co-divergence and host-switching in the evolution of Tobamoviruses. J Gen Virol. 93:408–418. doi:10.1099/vir.0.034280-0.
- Su L, Li ZN, Bernardy MG, Wiersma PA, Cheng ZH, Xiang Y. 2015. The complete nucleotide sequence and genome organization of Pea streak virus (genus Carlavirus). Arch Virol. 160:2651–2654. doi:10.1007/s00705-015-2467-2.
- Untiveros M, Perez-Egusquiza Z, Clover G. 2010. PCR assays for the detection of members of the genus Ilarvirus and family Bromoviridae. J Virol Meth. 165:97–104. doi:10.1016/j.jviromet.2010.01.011.
- van der Heijden MW, Bol JF. 2002. Composition of alphavirus-like replication complexes: involvement of virus and host encoded proteins. Arch Virol. 147:875–898. doi:10.1007/s00705-001-0773-3.
- van Regenmortel MHV, Fauquet CM, Bishop DHL, Carstens EB, Estes MK, Lemon SM, Maniloff J, Mayo MA, McGeoch DJ, Pringle CR, et al. 2000. Chapter, Family Bromoviridae. In: International Committee on Taxonomy of Viruses, Van Regenmortel MHV, editors. Virus taxonomy: seventh report of the International Committee on Taxonomy of Viruses. 1st ed. San Diego (USA): Academic Press; p. 923–935.
- Wilson CR. 1998. First report of Cucumber mosaic cucumovirus on wasabi in Australia. Plant Dis. 82:590–590. doi:10.1094/PDIS.1998.82.5.590A.
- Zaitlin M. 1999. Elucidation of the genome organization of Tobacco mosaic virus. Phil Trans R Soc Lond B Biol Sci. 354:587–591. doi:10.1098/rstb.1999.0410.