Abstract
Leaf rust caused by Puccinia hordei is a major disease of barley and is best managed by deploying effective resistance genes. The effect of post-inoculation temperature [18 ± 2°C (low), 22 ± 2°C (mid), or 26 ± 2°C (high)] on the expression of a selection of known all stage resistance (ASR) Rph genes and their interaction in combination with the adult plant resistance (APR) gene Rph20 were investigated in barley. Rph1.a and Rph2.b expressed best at higher temperatures whereas Rph4.d lost its effectiveness at higher temperatures when tested with pathotype (pt) 200 P− (avirulent on these genes). The expression of the genes Rph3.c, Rph5.e, Rph6.f, Rph12 (9.z), Rph13.x and Rph15.ad was not affected by post-inoculation temperature. The interaction between Rph14.ab and pt 5457 P+ gave the lowest infection type at low post-inoculation temperature. Among a selection of lines carrying Rph20 (‘Flagship’), Rph23 (‘Yerong’) and Rph24 (‘ND24260ʹ), higher levels of resistance were observed in seedlings of ‘Flagship’ (Rph20) at lower temperatures, while seedlings of ‘Yerong’ (Rph23) and ‘ND24260ʹ (Rph24) showed high infection types at all three temperatures with pt 5457 P+. When RphASR genes were combined with the APR gene Rph20 an enhanced level of seedling resistance was observed in the six combinations: Rph1.a+Rph20, Rph2.b+Rph20, Rph4.d+Rph20, Rph9.i+Rph20, Rph5.e+Rph20 and Rph15.ad+Rph20. Seedlings carrying the Rph5.e+Rph20 combination remained resistant when challenged with the Rph5.e-virulent pt 220 P+ + Rph13, suggesting a residual effect of Rph5.e when present in combination with Rph20. This is the first study to demonstrate that several ASR Rph genes interact with the APR gene Rph20 in an additive manner, emphasizing the importance of deploying genes in combination rather than individually to achieve durable resistance.
Résumé
La rouille des feuilles, causée par Hordeum vulgare, est une grave maladie de l’orge et elle est plus facilement gérée en déployant des gènes de résistance efficaces. L’influence des températures post-inoculation [18 ±2°C (basse), 22 ±2°C (moyenne), ou 26 ±2°C (élevée)] sur l’expression d’une sélection de gènes de résistance Rph connus, capables de conférer une résistance à tous les stades (RTS), ainsi que leur interaction en association avec le gène de résistance des plants adultes (RPA) Rph20, a été étudié chez l’orge. Les gènes Rph1.a et Rph2.b se sont mieux exprimés à températures élevées, tandis que le gène Rph4.d a perdu de son efficacité à températures élevées lorsqu’il a été testé avec le pathotype (pt) 200 P− (avirulent à l’égard de ces gènes). L’expression des gènes Rph3.c, Rph5.e, Rph6.f, Rph12 (9.z), Rph13.x et Rph15.ad n’a pas été influencée par la température post-inoculation. L’interaction entre Rph14.ab et pt 5457 P+ a affiché le plus faible type d’infection à basse température post-inoculation. Parmi une sélection de lignées portant les gènes Rph20 (‘Flagship’), Rph23 (‘Yerong’) et Rph24 (‘ND24260ʹ), de plus forts degrés de résistance ont été observés à basse température chez les plantules de ‘Flagship’ (Rph20), tandis que les plantules de ‘Yerong’ (Rph23) et de ‘ND24260ʹ (Rph24) ont affiché des types substantiels d’infections avec pt 5457 P+, et ce, aux trois températures. Quand les gènes RphRTS ont été combinés au gène Rph20 de RPA, un degré amélioré de résistance chez les plantules a été observé dans les six combinaisons suivantes: Rph1.a+Rph20, Rph2.b+Rph20, Rph4.d+Rph20, Rph9.i+Rph20, Rph5.e+Rph20 et Rph15.ad+Rph20. Les plantules portant la combinaison Rph5.e+Rph20 ont conservé leur résistance lorsque soumises à Rph5.e-virulent pt 220 P+ + Rph13, ce qui suggère un effet résiduel de Rph5.e lorsque combiné à Rph20. Cette étude est la première à démontrer que plusieurs gènes Rph RTS interagissent additivement avec le gène Rph20 de RPA, ce qui met l’accent sur l’importance de déployer des gènes en association plutôt qu’individuellement afin d’obtenir une résistance durable.
Introduction
Cultivated barley is an important cereal crop, ranked fourth after wheat, maize and rice. Barley is affected by various diseases that include four caused by rust fungi (crown rust, leaf/brown rust, stem rust and stripe/yellow rust), of which leaf rust (BLR) (caused by Puccinia hordei) is the most common and can lead to yield losses as high as 60% in very susceptible varieties (Cotterill et al. Citation1992; Griffey et al. Citation1994). Genetic resistance serves as the best measure to control the disease as it is economical and eco-sustainable and reduces reliance on fungicides. To date, 24 all stage resistance (ASR) genes (Rph1–Rph19, Rph21, Rph22, Rph25, Rph26–Rph27) have been mapped and catalogued (Park et al. Citation2015; Yu et al. Citation2018; Rothwell et al. Citation2020), many of which have been rendered ineffective by the evolution of new pathotypes (pts) of P. hordei (Park Citation2003). Three genes conferring adult plant resistance (APR) to leaf rust in barley have been designated to date, all of which have proved to be durable (viz. Rph20 (Hickey et al. Citation2011), Rph23 (Singh et al. Citation2015), Rph24 (Ziems et al. Citation2017)). Accumulating or pyramiding effective resistance genes into a single cultivar has proven to be one of the most effective strategies for achieving resistance durability (Ziems et al. Citation2017).
Interactions can occur between resistance genes when combined, resulting in a lowering of the disease level relative to that conferred by individual genes (Dyck Citation1977; Samborski and Dyck Citation1982). Rust resistance genes can interact in different ways, for example: (i) additive effects – when two effective genes are combined, a level of resistance higher than that of the individual genes alone is seen (Knott and Weller Citation1988; Singh et al. Citation2015; Ziems et al. Citation2017); (ii) modifying effect – when an effective gene is combined with an ineffective gene, the latter may lead to an improved level of resistance (Knott and Weller Citation1988); and (iii) residual or ghost effect – when two ineffective genes are combined, an increase in resistance may be observed due to small residual effects of the genes (Knott and Weller Citation1988). The focus on interactions between individual genes arose after the gene-for-gene relationship in host–parasite interactions was proposed by Flor (Citation1942).
Various gene combinations in wheat have been found to confer enhanced levels of resistance relative to genes that give intermediate levels of resistance individually. For instance, Samborski and Dyck (Citation1982) demonstrated that the combination of ASR genes Lr13+Lr16 (carried by North American wheat cultivar ‘Columbus’) conferred an enhanced level of resistance even when inoculated with an isolate of Puccinia triticina virulent on Lr16. The explanation behind lowering the disease level was that Lr13 had a ghost or residual effect on Lr16 (Samborski and Dyck Citation1982). No ghost or residual effect was observed when the two ASR genes were challenged with pathotypes that combined virulence for both Lr13 and Lr16 (Samborski and Dyck Citation1982). The wheat APR gene Lr34 is reported to be a highly interactive gene that provides an effective level of resistance in wheat singly, but when combined with other minor Lr genes confers higher levels of resistance. Various studies in wheat have also reported the interaction of the APR gene Lr34 with ASR genes such as Lr13, Lr16 and Lr23 (Oelke and Kolmer Citation2005), Lr37 (Kloppers and Pretorius Citation1997), and Lr3ka, Lr17a, Lr18 and Lr21 (German and Kolmer Citation1992).
APR genes such as Rph20 in barley and Lr34 and Sr2 in wheat have remained effective despite being used by breeders throughout the world over many years. In barley, the minor APR gene Rph23 confers very low levels of resistance but is highly interactive with other APR genes, such as Rph20 (conferring moderate resistance), resulting in high levels of resistance (Singh et al. Citation2015). Similarly, when Rph24 (conferring moderate resistance) was combined with Rph20 an enhanced level of resistance or additive effect was noticed as compared with the level of resistance conferred by these two genes individually (Hickey et al. Citation2011; Ziems et al. Citation2017). Although many studies on the interaction of various APR genes among each other are available, there are limited or no reports available on the interaction of APR with other ASR in barley. If APR in barley in fact interacts with ASR, then identification of the best gene combinations will be very useful for breeders trying to achieve durable resistance to leaf rust in barley.
Several previous studies have demonstrated that post-inoculation temperature plays a key role in modifying the expression of resistance genes or combinations of genes (Pretorius et al. Citation1984; Singh et al. Citation2013). In other words, temperature can be utilized by breeders to select a gene of interest by inactivating another (Luig and Rajaram Citation1972). For instance, single ASR genes conferring resistance to leaf rust in wheat such as Lr13 are expressed more strongly at higher post-inoculation temperatures. Genes Lr11, Lr14a, Lr15, Lr16, Lr18 and Lr30 (Dyck and Johnson Citation1983) are effective at lower post-inoculation temperatures, and the APR gene Lr34 is best expressed at very low post-inoculation temperatures (7°C) (Drijepondt et al. Citation1991). In wheat genotypes containing Lr34, it was observed that an increase in temperature shortened the latent period and resulted in larger uredinia, leading to higher severities of leaf rust (Pretorius et al. Citation1993). In addition, manipulating temperature can facilitate rapid phenotyping of APR (Rothwell et al. Citation2019). For example, postulation of the APR gene Lr34 is possible at seedling stages in the greenhouse under low temperatures (Dyck and Johnson Citation1983; Singh and Gupta Citation1992; Singh et al. Citation2007). Similarly, in barley, lines carrying Rph20 (second week), Rph23 (fourth week) and Rph24 (fourth week) showed resistance at 18°C in the greenhouse (Singh et al. Citation2013; Rothwell et al. Citation2019).
Considering the importance of temperature and gene interaction in modifying the expression of resistance, the studies reported here were designed with three objectives: (i) to identify the optimal post-inoculation temperature for the expression of 12 known ASR Rph genes; (ii) to assess whether or not selected ASR Rph genes act in an additive manner when combined with the APR gene Rph20; and (iii) to assess the effectiveness of various gene combinations with pathotypes virulent on each ASR gene.
Material and methods
Host material
Genotypes ‘Bowman’ and ‘Bowman’ near-isogenic lines (NILs) carrying 12 different known ASR Rph genes (originally developed by J.D. Franckowiak and colleagues at North Dakota State University; Chicaiza Citation1996; Caffarel Citation2005), ‘Flagship’ (Australian cultivar carrying Rph20), ‘Yerong’ (Rph23) and ‘ND24260ʹ (Rph24) () were investigated in this study. A BLR differential set (Park et al. Citation2015) regularly purified and maintained at the Plant Breeding Institute Cobbitty (PBIC) was included in all experiments.
Table 1. List of barley ‘Bowman’ near-isogenic lines (NILs)* and genotypes screened for response to Puccinia hordei at three different temperature regimes in the greenhouse
Development of plant material
The Bowman NILs were crossed with ‘Flagship’ and 12 gene combinations (BC2F3 or BC3F3) were developed using the theoretical model outlined in . Based on this model, all F3 families of a particular BCn for a specific gene combination (e.g. Flagship/Bowman+Rph12 (9.z) BC3F3 combination) should carry Rph20 in a homozygous state (AA), and the ASR gene involved will be either non-segregating (BB = 1/4 or bb = 1/4) or segregating (Bb = 2/4). The non-segregating resistant lines represent homozygous genetic stocks (pure lines) for Rph20+RphASR (e.g. Rph20+Rph12 (9.z)). Five of these pure lines were selected from each combination to assess resistance expression.
Pathogen material
The pathotypes of P. hordei used in this study were sourced from the cereal rust collection maintained at PBIC. For the temperature studies experiment two pts, 200 P− (culture no. = 518) and 5457 P+ (= 612), were used. Pathotype 200 P− is avirulent on all the genes investigated in this study () and pt 5457 P+ is virulent on all these genes in the ‘Bowman’ background except Rph5.e, Rph6.f, Rph13.x, Rph14.ab and Rph15.ad at temperature 22 ± 2°C. To determine the resistance expression of gene combinations, BCnF3 families were tested with pt 200 P− excluding Rph5.e+Rph20, Rph13.x+Rph20 and Rph15.ad+Rph20 gene combinations, which were screened with pt 5457 P+. To determine potential residual effects of defeated ASR genes, BCnF3 families were tested with pathotypes virulent on the ASR gene involved (either pt 5457 P+ or 220 P+ +Rph13). Because we did not have pathotypes virulent on Rph14.ab and Rph15.ad, the combinations involving these genes were excluded from the assessment of residual effects.
Table 2. Responses of barley near-isogenic lines (NILs) against pathotypes 200 P− and 5457 P+ of Puccinia hordei at three post-inoculation temperatures in the greenhouse under natural lighting
Greenhouse screening
Sowing and plant maintenance
Seeds of the Bowman NILs and the BCnF3 lines of each gene combination population were sown in 90 mm plastic pots containing a mixture of pine bark and coarse sand in a 4:1 ratio. The NILs were sown as clumps in a clockwise direction with three lines per pot, 15 seeds per line, and BCnF3 lines were sown as one line per pot, 20 seeds per line. The post-inoculation temperature studies experiment was replicated three times with two sets per replicate. The pots were fertilized with the soluble nitrogenous fertilizer Aquasol® (Hortico Pty Ltd, Australia) at a rate of 30 g in 10 L of water for 200 pots, prior to sowing. After sowing, the pots were kept in a growth room maintained at 18°C–20°C where they were regularly drip irrigated and fertilized again 1 day prior to inoculation.
Inoculation and disease assessment
Ten to 12-day-old seedlings were inoculated in an inoculation room with the required pathotype. Urediniospores were mixed with mineral oil (Shellsol®, Mobil Oil) in a tube and misted with an atomizer over the top of the seedlings. After each inoculation, the spray equipment and tube were kept in ethanol to prevent cross-contamination. The inoculated seedlings were transferred to an incubation room (without light) for 18 to 24 h at ambient temperatures, in which artificial mist was generated by an ultrasonic humidifier. The incubated seedlings were then transferred to greenhouse microclimates maintained at 18 ± 2°C (low post-inoculation temperature), 22 ± 2°C (mid post-inoculation temperature) or 26 ± 2°C (high post-inoculation temperature) depending upon the experimental objective. Disease response was assessed 10–12 days post-inoculation using a ‘0’–‘4’ scale (Park and Karakousis Citation2002).
Results
Resistance response of Rph genes under different temperatures
The recurrent parent of the NILs, ‘Bowman’, was susceptible, showing high infection types (ITs) (‘3+’) to both pathotypes (200 P− and 5457 P+) at all three temperatures (). The NILs carrying Rph13.x, Rph14.ab or Rph15.ad showed low ITs to all pathotypes. The IT of Rph13.x was relatively lower with pt 200 P− compared with pt 5457 P+, and for Rph14.ab the IT was lower with pt 5457 P+. The NIL carrying Rph15.ad exhibited a very low IT (;+CN) across all pathotypes and temperature regimes. There were very minor IT differences for Rph13.x under the three different temperature regimes. Gene Rph14.ab expressed notably better at 18 ± 2°C in comparison with the other two temperatures (22 ± 2°C and 26 ± 2°C) with pt 5457 P+ (). As expected, NILs carrying the ASR genes Rph1.a, Rph2.b, Rph3.c, Rph4.d and Rph11.p were susceptible (IT ‘3+’) to pt 5457 P+ (virulent on all these genes) at all three temperatures, although lines carrying the ASR genes Rph1.a, Rph2.b and Rph11.p had ITs marginally lower (‘3=’ to ‘33+’) with this pathotype at 26 ± 2°C. With pt 200 P−, avirulent on these genes, Rph1.a and Rph2.b best expressed at 26°C, while the effects of Rph4.d could not be seen at 26°C. For NILs carrying other ASR Rph genes (Rph3.c, Rph5.e, Rph6.f, Rph12 (9.z)), differences observed between ITs against the two pathotypes in the three temperature regimes were very minor and essentially negligible, indicating that they were not temperature sensitive. The NILs carrying Rph5.e or Rph6.f behaved identically to both pathotypes at all three temperatures (). Three lines carrying the APR genes Rph20 (‘Flagship’), Rph23 (‘Yerong’) and Rph24 (‘ND24260ʹ) singly were also included in these seedling tests. At lower temperatures (18°C), ‘Flagship’ (Rph20) displayed low IT responses with both pathotypes. ‘ND24260ʹ (Rph24) was susceptible to both pathotypes at all three temperatures, and ‘Yerong’ (Rph23) showed a high IT response with pt 5457 P+ and a low IT (;12 to 2C) response with pt 200 P− with best expression at the high temperature. The low IT of ‘Yerong’ with pt 200 P− is attributed to Rph2 as this pathotype is avirulent on this ASR gene (Derevnina et al. Citation2013).
Assessment and isolation of gene combination lines
BC2F3 and BC3F3 lines derived for each gene combination were tested with specific avirulent pathotypes in the greenhouse and classified as homozygous resistant (HR), segregating (Seg), or homozygous susceptible (HS). As predicted according to the theoretical model (), the F3 families of all combinations (except Rph11.p and Rph14.ab) segregated for the genetic ratio expected for the inheritance of a single gene (1HR:2Seg:1HS; ), thereby confirming that each of these gene combinations carries a specific single ASR Rph gene. The BCnF3 families derived from crosses B+Rph11.p/Flagship and B+Rph14.ab/Flagship did not segregate (probably there was an accidental self-pollination instead of a cross) and were therefore incorrect and hence not studied further. The HR lines from each combination were selected for further studies because they represent putative homozygous gene combination stocks of each ASR gene in combination with the APR gene Rph20 (based on selection of BC1F2 individuals using a codominant marker linked to Rph20 (Dracatos et al. Citation2021); ). The identified HR lines are combination stocks of Rphseedling+Rph20 (AABB).
Table 3. Frequency of segregation (HR, Seg and HS)* observed among BCnF3 families of barley for each gene combination when inoculated with pathotypes 200 P− or 5457 P+ of Puccinia hordei.
Identification of the best gene combinations with improved resistance
Five randomly selected HR lines from each gene combination were tested with a pathotype avirulent on the ASR gene present, and the responses of the combinations were compared with the single gene carrying parents, i.e. Bowman NIL (carrying RphASR) and ‘Flagship’ (Rph20). Improved resistance (at least one sporulation scale less on the 1–4 assessment scale) over that observed for each parent was noticed for the combinations Rph1.a+Rph20, Rph2.b+Rph20, Rph4.d+Rph20, Rph5.e+Rph20, Rph9.i+Rph20 and Rph15.ad+Rph20. Among these combinations, Rph2.b+Rph20, Rph4.d+Rph20 and Rph15.ad+Rph20 were deemed the best for providing levels of resistance higher than those of the two individual parents ().
Table 4. Infection type (IT) response of barley parents and gene combinations using avirulent pathotypes (200 P− or 5457 P+) of Puccinia hordei.
Assessment of residual resistance effect in gene combinations
HR lines from each population were tested with pathotypes virulent on each of the ASR genes involved to assess the effectiveness of each gene combination. Except for one combination (i.e. Rph5.e+Rph20), all lines with gene combinations showed high IT responses (3+) when challenged with a pathotype virulent for the ASR gene present. In the combination Rph5.e+Rph20, an incompatible IT ‘;1-CN’ was observed in tests with the Rph5.e-virulent pathotype 220 P+ +Rph13, to which the parents, B+Rph5.e and Flagship were susceptible (ITs ‘3+’ and ‘3+’, respectively) (). The incompatible IT response observed may be a residual effect as a consequence of the interaction between the ASR gene Rph5.e and the APR gene Rph20.
Fig. 2 (Colour online) Rph5.e+Rph20 combination lines of barley showing improved resistance in contrast to parental line Bowman+Rph5.e and ‘Flagship’ (Rph20) when tested with avirulent pathotype 5457P+ (A) and virulent pathotype 220 P+ +Rph13 (B) of Puccinia hordei. ‘Bowman’ was used as a susceptible control
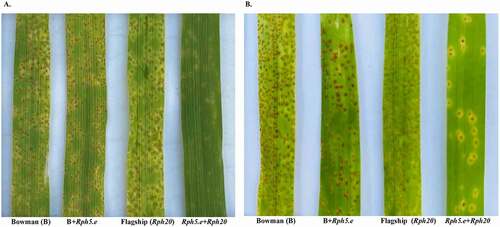
Discussion
Resistance to P. hordei is available, but identifying resistance that is genetically diverse, easy to select and effective for successful disease management is challenging (Niks et al. Citation2000; Park et al. Citation2015). Understanding the effectiveness and expression of resistance genes in combination is important for gene stewardship as well as ensuring diversity in the resistances that are deployed to achieve durable resistance. While many such studies on understanding the expression of resistance genes and their combinations have been conducted in wheat (Kloppers and Pretorius Citation1997; Oelke and Kolmer Citation2005), knowledge of Rph gene combinations in barley is very limited. To narrow this gap, this research was designed to understand the expression of Rph genes in barley both singly and in combination and to assess their interactions.
Temperature can play an important role in the interaction between hosts and pathogens causing cereal rust disease. For example, at high temperatures the wheat leaf rust ASR genes Lr16, Lr17a and Lr23 showed better expression, whereas Lr18 was completely ineffective (Dyck and Johnson Citation1983), and in barley, genes such as Rph20 and Rph21 were reported to be temperature sensitive (Singh et al. Citation2013; Sandhu et al. Citation2014). To expand our knowledge of the expression of ASR Rph genes, 12 Bowman NILs were tested with pathotypes avirulent or virulent over a range of temperatures under natural light in the greenhouse. These studies demonstrated that phenotypic expression of many of the Rph genes is temperature sensitive. At higher temperatures with an avirulent pathotype (200 P−), the ASR genes Rph1.a and Rph2.b expressed best, whereas the effects of Rph4.d could not be seen. Genes Rph3.c, Rph5.e, Rph6.f, Rph12 (9.z), Rph13.x and Rph15.ad exhibited high levels of resistance in response to pt 200 P− under all three temperature regimes, showing that these genes are not temperature sensitive. Variations in IT responses on NILs carrying Rph14.ab were observed depending on the temperature and pathotype used, with the gene being more effective at lower temperatures with pt 5457 P+. Among the three lines carrying single APR genes, only ‘Flagship’ (Rph20) showed variability with temperature, with a stronger expression at lower temperatures, concurring with the findings of Singh et al. (Citation2013). The low IT recorded on ‘Yerong’ (Rph23) with pt 200 P− was due to the effects of the ASR gene Rph2 (Derevnina et al. Citation2013).
The results reported here underline the importance of temperature sensitivity in the detection of ASR Rph genes in the greenhouse. The detection of resistance conferred by APR genes such as Rph20, as demonstrated in this study and in previous studies (Singh et al. Citation2013), could also explain the role that environment plays in its expression in the field and in different regions, with implications for disease progression and epidemiology. The low-temperature resistance conferred by Rph20 in the greenhouse suggests that this gene may provide greater protection at earlier growth stages when deployed in relatively cooler barley-growing regions, or that it could provide stronger protection during cooler winter months and in this way reduce inoculum pressure in the following spring.
Gene interaction is of considerable interest as it provides higher levels of protection, and potentially greater durability than single-gene deployment. Previous studies have shown additive interactions between Rph20 and other APR genes, such as Rph23 and Rph24 (Singh et al. Citation2015; Ziems et al. Citation2017). In wheat, the gene combination Sr2+Sr9d was shown to provide additive resistance, with the level of resistance detected exceeding the genes individually because Sr2 has a modifying effect on Sr9d (Knott and Weller Citation1988). Interestingly, an enhancement in resistance was also observed by Samborski and Dyck (Citation1982) even when two ineffective genes were combined due to a ghost effect of one gene on another. The study reported here is the first to examine interactions between seedling resistance genes and adult plant resistance genes in barley.
Enhancement of resistance conditioned due to the interactive nature of Rph20 with ASR genes was observed in the combinations Rph2.b+Rph20, Rph4.d+Rph20, Rph5.e+Rph20, Rph9.i+Rph20 and Rph15.ad+Rph20 when using a pathotype avirulent for the ASR gene. Nevertheless, there could also be other factors in the genetic backgrounds besides the two known resistance genes that enhance the resistance. When the lines of combination Rph5.e+Rph20 were tested with pt 220 P+ +Rph13, a compatible response at the seedling stage was expected because Rph5.e is ineffective in this pathotype and Rph20 is an APR gene. However, a lower IT was observed, probably due to the ghost or residual effect of Rph5.e in the presence of Rph20. Similar effects were also noticed by Samborski and Dyck (Citation1982) when seedlings of wheat cultivar ‘Columbus’ (Lr13 and Lr16) were inoculated with a pathotype virulent on Lr13; the IT produced was much lower than that produced by seedlings carrying Lr13 singly. The low infection in the combination could also be caused by an unknown APR in Bowman+Rph5.e NIL (used for developing the combination) and that APR is interactive with Rph20. To check this hypothesis, the Rph5.e NIL was tested at adult plant growth stages with pathotype 220 P+ +Rph13 in the greenhouse, and it was found that the B+Rph5.e line was susceptible and hence did not carry additional APR.
In summary, this study has shown that the expression of most ASR Rph genes depends on the pathotype and post-inoculation temperature used. While previous studies had established that the APR genes Rph20, Rph23 and Rph24 interact and produce enhanced levels of resistance, the results of this study have demonstrated that ASR and APR genes also interact and result in improved leaf rust resistance in barley. These results have important implications for plant breeding in achieving durability and also emphasize the value of deploying genes in combination rather than individually.
Acknowledgements
The authors thank Matthew Williams and Gary Standen for providing technical support, and Judith and David Coffey and their families for supporting the research.
Additional information
Funding
References
- Caffarel JC. 2005. Leaf rust studies in barley using Bowman backcross-derived lines [MS Thesis]. Fargo (USA): North Dakota State University.
- Chicaiza O. 1996. Genetic control of leaf rust [PhD Thesis]. Fargo: North Dakota State University.
- Cotterill PJ, Rees RG, Platz GJ, Dill-Macky R. 1992. Effects of leaf rust on selected Australian barleys. Aust J Exp Agric. 32:747–226. doi:https://doi.org/10.1071/EA9920747.
- Derevnina L, Singh D, Park RF. 2013. Identification and characterization of seedling and adult plant resistance to Puccinia hordei in Chinese barley germplasm. Plant Breed. 132:571–579. doi:https://doi.org/10.1111/pbr.12082.
- Dracatos PM, Park RF, Singh D. 2021. Validating molecular markers for barley leaf rust resistance genes Rph20 and Rph24. Plant Dis. 105(4):743–747. doi:https://doi.org/10.1094/PDIS-08-20-1735-SC.
- Drijepondt SC, Pretorius ZA, Rijkenberg FHJ. 1991. Expression of two wheat leaf rust resistance gene combinations involving Lr34. Plant Dis. 75(5):526–528. doi:https://doi.org/10.1094/PD-75-0526.
- Dyck PL. 1977. Genetics of leaf rust reaction in three introductions of common wheat. Can J Genet Cytol. 19:711–716. doi:https://doi.org/10.1139/g77-077.
- Dyck PL, Johnson R. 1983. Temperature sensitivity of genes for resistance in wheat to Puccinia recondita. Can J Plant Pathol. 5(4):229–234. doi:https://doi.org/10.1080/07060668309501601.
- Flor HH. 1942. Inheritance of pathogenicity in Melampsora lini. Phytopathology. 32:653–659.
- German SE, Kolmer JA. 1992. Effect of gene Lr34 in the enhancement of resistance to leaf rust of wheat. Theor Appl Genet. 84:97–105. doi:https://doi.org/10.1007/BF00223987.
- Griffey CA, Das MK, Baldwin RE, Waldenmaier CM. 1994. Yield losses in winter barley resulting from a new race of Puccinia hordei in North America. Plant Dis. 78:256–260. doi:https://doi.org/10.1094/PD-78-0256.
- Hickey LT, Lawson W, Platz GJ, Dieters M, Arief VN, Germán S, Fletcher S, Park RF, Singh D, Pereyra S, et al. 2011. Mapping Rph20: a gene conferring adult plant resistance to Puccinia hordei in barley. Theor Appl Genet. 123:55–68. doi:https://doi.org/10.1007/s00122-011-1566-z.
- Kloppers FJ, Pretorius ZA. 1997. Effects of combinations amongst genes Lr13, Lr34 and Lr37 on components of resistance in wheat to leaf rust. Plant Pathol. 46(5):737–750. doi:https://doi.org/10.1046/j.1365-3059.1997.d01-58.x.
- Knott DR, Weller J. 1988. Genetic interactions among four genes for resistance to stem rust in bread wheat. Genome. 30:182–185. doi:https://doi.org/10.1139/g88-031.
- Luig NH, Rajaram S. 1972. The effect of temperature and genetic background on host gene expression and interaction to Puccinia graminis tritici. Phytopathology. 62:1171–1174. doi:https://doi.org/10.1094/Phyto-62-1171.
- Niks RE, Walther U, Jaiser H, Martinez F, Rubiales D. 2000. Resistance against barley leaf rust (Puccinia hordei) in West-European spring barley germplasm. Agronomie. 20:769–782. doi:https://doi.org/10.1051/agro:2000174.
- Oelke LM, Kolmer JA. 2005. Genetics of leaf rust resistance in spring wheat cultivars Alsen and Norm. Phytopathology. 95(7):773–778. doi:https://doi.org/10.1094/PHYTO-95-0773.
- Park RF. 2003. Pathogenic specialisation and pathotype distribution of Puccinia hordei Otth. in Australia, 1992-2001. Plant Dis. 87(11):1311–1316. doi:https://doi.org/10.1094/PDIS.2003.87.11.1311.
- Park RF, Golegaonkar PG, Derevnina L, Sandhu KS, Karaoglu H, Elmansour HM, Dracatos PM, Singh D. 2015. Leaf rust of cultivated barley: pathology and Control. Annu Rev Phytopathol. 53:565–589. doi:https://doi.org/10.1146/annurev-phyto-080614-120324.
- Park RF, Karakousis A. 2002. Characterization and mapping of gene Rph19 conferring resistance to Puccinia hordei in the cultivar ‘Reka 1ʹ and several Australian barleys. Plant Breed. 121(3):232–236. doi:https://doi.org/10.1046/j.1439-0523.2002.00717.x.
- Pretorius ZA, Kloppers FJ, Drijepondt SC. 1993. Effects of inoculum density and temperature on three components of leaf rust resistance controlled by Lr34 in wheat. Euphytica. 74:91–96. doi:https://doi.org/10.1007/BF00033772.
- Pretorius ZA, Wilcoxson RD, Long DL, Schafer JF. 1984. Detecting wheat leaf rust resistance gene Lr13 in seedlings. Plant Dis. 68(7):585–586. doi:https://doi.org/10.1094/PD-69-585.
- Rothwell CT, Singh D, Dracatos PM, Park RF. 2020. Inheritance and characterization of Rph27: a third race-specific resistance gene in the barley cultivar Quinn. Phytopathology. 110(5):1067–1073. doi:https://doi.org/10.1094/PHYTO-12-19-0470-R.
- Rothwell CT, Singh D, Ogtrop FV, Sørensen C, Fowler R, Germán S, Park RF, Dracatos P. 2019. Rapid phenotyping of adult plant resistance in barley (Hordeum vulgare) to leaf rust under controlled conditions. Plant Breed. 138(1):51–61. doi:https://doi.org/10.1111/pbr.12660.
- Samborski DJ, Dyck PL. 1982. Enhancement of resistance to Puccinia recondita by interactions of resistance genes in wheat. Can J Plant Pathol. 4(2):152–156. doi:https://doi.org/10.1080/07060668209501317.
- Sandhu KS, Singh D, Park RF. 2014. Characterising seedling and adult plant resistance to Puccinia hordei in Hordeum vulgare. Ann Appl Biol. 165(1):117–129. doi:https://doi.org/10.1111/aab.12122.
- Singh D, Dracatos P, Derevnina L, Zhou M, Park RF. 2015. Rph23: a new designated additive adult plant resistance gene to leaf rust in barley on chromosome 7H. Plant Breed. 134(1):62–69. doi:https://doi.org/10.1111/pbr.12229.
- Singh D, Macaigne N, Park RF. 2013. Rph20: adult plant resistance gene to barley leaf rust can be detected at early growth stages. Eur J Plant Pathol. 137:719–725. doi:https://doi.org/10.1007/s10658-013-0282-8.
- Singh D, Park RF, Mcintosh RA. 2007. Characterisation of wheat leaf rust resistance gene Lr34 in Australian wheats using components of resistance and the linked molecular marker csLV34. Aust J Agric Res. 58(11):1106–1114. doi:https://doi.org/10.1071/AR07002.
- Singh RP, Gupta AK. 1992. Expression of wheat leaf rust resistance gene Lr34 in seedlings and adult plants. Plant Dis. 76(5):489–491. doi:https://doi.org/10.1094/PD-76-0489.
- Yu X, Kong HY, Meiyalaghan V, Casonato S, Chng S, Jones EE, Butler RC, Pickering R, Johnston PA. 2018. Genetic mapping of a barley leaf rust resistance gene Rph26 introgressed from Hordeum bulbosum. Theoret Appl Genet. 131:2567–2580. doi:https://doi.org/10.1007/s00122-018-3173-8.
- Ziems LA, Hickey LT, Platz GJ, Franckowiak JD, Dracatos PM, Singh D, Park RF. 2017. Characterization of Rph24: a gene conferring adult plant resistance to Puccinia hordei in barley. Phytopathology. 107:834–841. doi:https://doi.org/10.1094/PHYTO-08-16-0295-R.