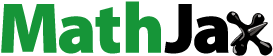
Abstract
Clubroot, caused by Plasmodiophora brassicae, is an important soilborne disease of canola (Brassica napus) in Alberta, Canada. As clubroot prefers acidic soils, the application of lime to increase soil pH may reduce disease. Replicated field plot experiments were conducted to evaluate the efficacy of hydrated lime for clubroot management. In 2017, moderate (11.4 t ha−1) to high rates (12.7 t ha−1) of hydrated lime reduced the index of disease (ID) by 35–91% in a susceptible canola cultivar 8 weeks after seeding. In contrast, no effect of lime treatment was observed in the 2018 field trials, due to several environmental factors. A greenhouse study compared the efficacy of hydrated lime and limestone in reducing ID in susceptible and resistant canola cultivars, at different application rates and inoculum concentrations. In treatments that did not receive lime, ID was very high (92–100%) in the susceptible and low (9–13%) in the resistant canola. The application of hydrated lime at 4.7, 8.1, 11.4 and 14.8 t ha−1 eliminated visible symptoms in both cultivars, whereas limestone reduced the ID only at the two lowest inoculum concentrations. Root tissues from the same study were analyzed by quantitative PCR to measure P. brassicae proliferation in planta. Inoculum concentration and the type and rate of lime significantly affected the amount of P. brassicae DNA present in the root tissue. Repeated trials with less virulent inoculum indicated similar trends. Hydrated lime may represent a more effective tool than limestone to manage P. brassicae on canola in highly infested soils.
Résumé
En Alberta, au Canada, la hernie, causée par Plasmodiophora brassicae, est une importante maladie terricole du canola (Brassica napus). Puisque la hernie préfère les sols acides, l’application de chaux pour accroître le pH du sol peut contribuer à atténuer la maladie. Des expériences répétées sur des parcelles en plein champ ont été menées afin d’évaluer l’efficacité de la chaux pour gérer la hernie. En 2017, des taux modérés (11,4 t ha−1) à élevés (12,7 t ha−1) de chaux on réduit l’indice de la maladie (IM) de 35 à 91% chez un cultivar sensible, 8 semaines après l’avoir semé. En revanche, aucun effet du traitement avec de la chaux n’a été observé en 2018 dans des essais en plein champ à cause de plusieurs facteurs environnementaux. Une étude menée en serre a permis de comparer l’efficacité de la chaux et du calcaire pour ce qui est de réduire l’IM chez des cultivars de canola sensibles et résistants, et ce, à différents taux d’application et pour diverses concentrations d’inoculum. Dans le cas des traitements sans chaux, l’IM était très élevé (92 à 100%) chez les cultivars sensibles et, faible (9 à 13%) chez les cultivars résistants. L’application de chaux aux taux de 4,7, 8,1, 11,4 et 14,8 t ha−1 a éliminé les symptômes visibles chez les deux types de cultivars, tandis que le calcaire a réduit l’IM aux deux plus faibles concentrations d’inoculum seulement. Des tissus racinaires provenant de la même étude ont été analysés par PCR afin de mesurer la prolifération de P. brassicae dans les plants. La concentration d’inoculum et le type ainsi que le taux d’application de chaux ont significativement influencé la quantité d’ADN de P. brassicae contenue dans les tissus racinaires. Des essais répétés avec des inoculums moins virulents ont indiqué des tendances similaires. La chaux peut être un outil plus efficace que le calcaire pour combattre P. brassicae chez le canola dans des champs gravement infestés.
Introduction
Clubroot, caused by Plasmodiophora brassicae Woronin, is a soilborne disease of the Brassicaceae family, including the economically important canola (oilseed rape; Brassica napus L.) crop. Infected plants develop distinct, club-shaped galls on the tap and lateral roots. The galls interfere with the plant’s ability to take up water and nutrients, leading to wilting, stunting and premature ripening. In severe cases, clubroot can cause up to 100% yield loss and decrease canola seed oil content by 5–6% (Pageau et al. Citation2006; Strelkov et al. Citation2007). Since 2003, clubroot has emerged as one of the most important diseases of canola in western Canada, and now occurs throughout most of the canola-producing districts of Alberta (Strelkov et al. Citation2021). It is also found with increasing frequency in Saskatchewan (Ziesman et al. Citation2019), Manitoba (Froese et al. Citation2019), Ontario (Al-Daoud et al. Citation2017) and North Dakota (Chittem et al. Citation2014), indicating that clubroot may become a major problem over a much wider area of canola production.
Few tools were available for the effective management of clubroot on canola until the first resistant cultivars were released in 2009/2010 (Strelkov et al. Citation2011). Clubroot-resistant (CR) canola is now grown widely throughout Alberta, but the erosion or loss of resistance has been observed in an increasing number of fields since 2013 (Strelkov et al. Citation2016, Citation2018; Hollman et al. Citation2020). This loss of resistance has been attributed to pathotype shifts in P. brassicae populations, resulting from the selection pressure imposed by the cultivation of resistant hosts (Strelkov et al. Citation2016, Citation2018). Given that pathotype shifts can occur quickly, strategies that reduce inoculum levels and/or disease pressure may contribute to resistance stewardship, wherein the durability of current resistance genes is maintained and prolonged (Peng et al. Citation2015). This is important, as the identification and introgression of new resistance genes into elite germplasm for cultivar development can take time.
Soil amendments, including lime, have long been used for the management of clubroot on vegetable Brassicas. Liming increases soil pH and calcium levels, making conditions less conducive for the development of the disease (Webster Citation1986; Murakami et al. Citation2002). Higher soil pH and calcium reduce the ability of P. brassicae to complete the primary stage of its life cycle, affecting resting spore germination, the production of primary zoospores and root hair infection (Bochow Citation1961; Niwa et al. Citation2008). An increase in soil pH is required 0–7 days after inoculation for a visible reduction in clubroot symptoms (reviewed in Dixon Citation2009). Treatments that delay infection for several weeks after seeding are also likely to reduce disease severity and spore production, and have a positive impact on seed yield in clubroot-susceptible canola grown in P. brassicae-infested soil (Hwang et al. Citation2011b). Lime formulations differ in the amount of calcium supplied and its subsequent rate of release (Campbell and Grethead Citation1989). Limestone (CaCO3) is the main source for agricultural application to neutralize soil pH and improve plant growth (Wellman Citation1930; Kenny and Oates Citation2000). Hwang et al. (Citation2011b) found that rates of 5.0 and 7.5 T ha−1 of limestone reduced clubroot disease severity at several sites in Alberta, but the results were inconsistent over site and year. High-calcium hydrated lime is a dry powder produced by combining quicklime (CaO) with water, resulting in the product Ca(OH)2 containing approximately 75% CaO and 25% H2O (Graymont Limited Citation2019). Once mixed with water, hydrated lime quickly dissolves, resulting in a highly alkaline solution (pH 12.4) (Graymont Limited Citation2019). In a series of laboratory and pot tests conducted by Wellman (Citation1930) on transplanted cabbage, hydrated lime completely inhibited clubroot infection, while there was no effect from the use of limestone.
With approximately 2.55 million hectares of soils with a pH ≤ 6.0 in western Canada (Lickacz Citation2002), liming could be an appropriate strategy to manage clubroot and improve soil health. Rapid increases in soil pH and calcium content prior to seeding appear to be vital for effective clubroot management, and the quicker activity of hydrated lime may be helpful in this respect (Webster Citation1986; Webster and Dixon Citation1991). As P. brassicae continues to spread across the Northern Great Plains of North America, and the number of fields with resistance issues continues to climb, the need for effective management options to supplement CR canola becomes more important. The objectives of this study were to (1) evaluate the potential of hydrated lime for reducing clubroot disease development under field conditions; (2) compare the efficacy of varying rates of hydrated lime and limestone for clubroot control at different inoculum levels under greenhouse conditions; and (3) measure the effect of different lime treatments on P. brassicae proliferation in host roots.
Materials and methods
Field trials
Replicated field trials were conducted in 2017 and 2018 to evaluate the effects of multiple rates of hydrated lime on clubroot disease and various growth parameters and yield. The trials were located at the Crop Diversification Centre North, Alberta Agriculture and Forestry, Edmonton, Alberta, in a black Chernozemic loam soil (Soil Classification Working Group Citation1998) naturally infested with P. brassicae. The predominant pathotype in this nursery was pathotype 3H, as defined on the Canadian Clubroot Differential (CCD) set (Strelkov et al. Citation2018), or pathotype 3 or P2 as classified on the systems of Williams (Citation1966) and Somé et al. (Citation1996), respectively. Within each experimental year, the trials were located on the east side of the 6 ha nursery (53° 38.8ʹ N, 113° 22ʹ 33”W), known as site 1, and on the west side of the nursery (53° 38.8ʹ N, 113° 22ʹ 49”W), known as site 2 (about 400 m from site 1). In the second year, the plots at each site were placed adjacent to the previous year’s plots, to avoid the residual effects of the lime treatment. Treatments were arranged in a randomized complete block design with four replicates. Each plot was 1.5 × 6 m with a 0.5 m buffer between plots and a 2 m buffer between replicates. To determine the pH, soil samples were collected with a trowel to a depth of 10 cm. Two subsamples of soil per plot were combined per replicate and mixed in a pail. The average pH of the four replicates was regarded as the starting or pre-treatment pH at each site. The soil samples were also used to determine the P. brassicae inoculum concentration (see below).
Hydrated lime (Ca(OH)2 (Graymont, Richmond, BC) rates were calculated based on the targeted pH relative to the pre-treatment pH of the soil in the plots in the first year (2017). A rate of 3.36 t lime ha−1 to increase the soil pH by 0.5 (Government of Alberta Citation2002) was used in these calculations. In 2017, the pre-treatment pH at sites 1 and 2 was 6.3 and 5.1, respectively. The target pH values at site 1 were 7.0 (4.7 t lime ha−1 required), 7.5 (8.0 t lime ha−1) and 8.0 (11.4 t lime ha−1). At site 2, the target pH values were 6.0 (6.0 t lime ha−1), 6.5 (9.4 t ha−1) and 7.0 (12.7 t ha−1). To maintain consistency, the same amounts of lime were applied to the trials in 2018, although the average pre-treatment pH values at sites 1 and 2 were 6.6 and 5.0, respectively. The lime treatments were spread across the plots manually, as evenly as possible, and incorporated immediately afterwards to a depth of 8–10 cm using a 2 m tiller attached to a tractor. The trials were seeded with the clubroot-susceptible canola cultivar ‘45H31ʹ (DuPont Pioneer, Mississauga, ON, Canada) 7–8 days after lime application and incorporation.
In 2017, the lime was applied and incorporated on June 8 at both sites and canola was seeded on June 16. During that week, the plots received approximately 19 mm of natural rainfall. In 2018, the lime was applied and incorporated on May 22 at both sites, and canola was seeded on May 29. During this week, natural rainfall was minimal, and therefore, the plots were irrigated with 30 L of water over each 9-m2 treatment plot. Due to the low moisture and cutworm damage, the trial was re-seeded on June 18 after being sprayed with Decis 5EC (Bayer, Calgary, AB) on June 14. Total rainfall for the plots reached 34 mm in addition to the water applied on May 22 by the second seeding date. A second insecticidal spray with Decis 5EC + Lorsban NT (Corteva, USA) was applied to control cutworms on June 28.
Seedling emergence was recorded 7, 14 and 21 days after seeding, while plants were rated for clubroot symptom development 8 weeks after seeding. Briefly, 10 plants per plot were dug out of the soil, the roots were washed with water, and each root was rated on a 0 to 3 scale where: 0 = no galling, 1 = a few small galls, 2 = moderate galling, and 3 = severe galling (Kuginuki et al. Citation1999). The individual ratings were used to calculate an index of disease (ID) for each plot, according to the formula of Horiuch and Hori (Citation1980) as modified by Strelkov et al. (Citation2006):
Where: = number of plants in a class;
= total number of plants in an experimental unit; and 0, 1, 2 and 3 = symptom severity classes.
Individual plant height, above-ground biomass and root weight were recorded for 10 plants per plot 8 weeks after seeding. Sites 1 and 2 were harvested with a small plot combine on October 6 in 2017 and on October 19 and 18, respectively, in 2018. The grain was dried and cleaned before weighing and calculating yield.
Greenhouse trials
The effects of multiple rates of hydrated lime and limestone on clubroot development were compared at various inoculum levels on two canola genotypes. A clubroot-susceptible cultivar ‘45H31ʹ (DuPont Pioneer) and a clubroot-resistant cultivar ‘9558C’ (DuPont Pioneer), both treated with Prosper FX (Bayer Crop Science, Calgary, AB), were grown in a potting medium consisting of a mixture of 10% peat moss and 90% soilless mix (Sungro Professional Growing Mix, Sungro Horticulture, Seba Beach, AB) to create an initial soil pH of 5.3. Soil aliquots of 25 L were prepared and represented one treatment of inoculum, lime product and rate, for both cultivars.
The potting medium was inoculated with different concentrations of P. brassicae resting spores. The inoculum consisted of resting spores collected from root galls (stored at −20°C) caused by pathotype 3H (Strelkov et al. Citation2018). The same pathotype was used for both experiments, but the collection of galls used to create the inoculum came from two different previous greenhouse experiments. To extract the P. brassicae resting spores, galls were ground in a blender with water, and then filtered through six layers of cheesecloth (American Fibre & Finishing Inc., Albemarle, NC). A stock concentration of resting spores was estimated with a haemocytometer (VWR, Mississauga, Ontario) to target an inoculation of 1 × 106 resting spores g−1 medium for a 25 L aliquot of medium. Serial dilutions were performed with the stock concentration to target the three remaining final potting medium concentrations of 1 × 103, 1 × 104 and 1 × 105 resting spores g−1 potting medium.
The dilutions of resting spore suspensions were added to 25 L aliquots of potting medium and mixed thoroughly by hand. Following soil inoculation with P. brassicae, the potting medium was treated with ‘Zero Grind’ limestone (Graymont) or hydrated lime (Graymont) and mixed thoroughly by hand again, at rates equivalent to 4.7, 8.1, 11.4 or 14.8 t ha−1 of lime, to adjust the pH to 6.0, 6.5, 7.0 or 7.5, respectively. Rates were calculated as per Alberta Agriculture and Forestry guidelines (Government of Alberta Citation2002) as described earlier for the field trials. Following the application of lime and inoculation with the corresponding concentration of P. brassicae resting spores, each 25 L aliquot of potting medium was mixed thoroughly with 1.8 L of water and left in the greenhouse for 1 week prior to being transferred into pots to prep for seeding.
The canola genotypes were sown 2 cm deep at a density of 16 seeds per 12 × 12 × 12 cm pot filled with 2.14 L of potting medium; treatments were thinned to five plants per pot 10 days after planting. The pots were maintained in a greenhouse at ca. 24°C with 30% relative humidity under natural light supplemented with artificial lighting (16 h day/8 h night). The pots were placed on water-filled trays for the first 2 weeks after seeding to ensure sufficient moisture for clubroot development, following which holes were poked in the trays and the plants were top-watered as needed with watering cans. Pots were fertilized with a 0.1% solution of 20:20:20 (N:P:K) once a week until harvest.
In summary, 80 treatments were examined in each repetition of the greenhouse experiment. These included all combinations of the four tested inoculum concentrations (1 × 103, 1 × 104, 1 × 105 and 1 × 106 resting spores g−1 medium), five rates of each lime product (limestone or hydrated lime at rates of 0.0, 4.7, 8.1, 11.4 or 14.8 T ha−1 of lime) and two canola cultivars (‘45H31ʹ and ‘9558C’). There were two control treatments for each inoculum concentration (example: 1 × 103 resting spores g−1 medium + no limestone and 1 × 103 resting spores g−1 medium + no hydrated lime) where the medium was inoculated but did not receive a lime treatment. Each treatment was replicated five times. The entire experiment was repeated, with the first trial conducted in 2017 and the second in 2018. In 2017, inoculation and liming took place on August 22, followed by seeding on August 30 and harvest on October 31. In 2018, inoculation and liming occurred on May 11, followed by seeding on May 18 and harvest on July 10. At harvest, the plants were dug out from the potting medium, and the roots were washed and scored for clubroot symptom severity on a 0 to 3 scale (Kuginuki et al. Citation1999). The individual severity ratings were used to calculate an ID for each replicate as described above for the field trials. Plant height was recorded for each plant and averaged per replicate. Additionally, dry-weights were recorded after the harvested plants were dried in the greenhouse for 1 week.
PCR analysis
Soil samples were collected 2 weeks before lime application in the field trials to determine the starting level of P. brassicae inoculum. In 2017, approximately 500 g of soil was collected across the trial replicates and mixed together, while in 2018 the soil samples were collected and processed separately for the individual plots. The soil samples were air-dried at room temperature and then ground to a powder in a mortar with a pestle. Total genomic DNA was extracted from the soil samples following Cao et al. (Citation2007) and stored at 4°C if they were to be analyzed within 1 week, or at −20°C for longer-term storage. In the greenhouse trials, root samples were collected to quantify colonization by P. brassicae. Briefly, 10-day old seedlings were removed carefully from the potting medium and washed in standing water; the root system was excised with a scalpel and stored in a small bag in a −20°C freezer until further processing. Total genomic DNA was extracted from these root samples with a NucleoSpin® Plant II DNA Isolation Kit (Macherey-Nagel GmbH & Co. KG, Germany) as per the manufacturer’s instructions. The DNA samples were stored at 4°C if they were to be analyzed within 1 week, or stored at −20°C for longer periods.
All samples were subjected to conventional PCR analysis to determine the presence or absence of P. brassicae DNA using primers TC1F and TC1R as described by Cao et al. (Citation2007). Those samples which tested positive for the presence of P. brassicae DNA were analyzed further by quantitative PCR (q-PCR) following Rennie et al. (Citation2011), with resting spore concentrations estimated on a five-point standard curve generated with standards of 1 × 102, 1 × 103, 1 × 104, 1 × 105 and 1 × 106 resting spores g−1 soil (Rennie et al. Citation2011) or g−1 root tissue (Cao et al. Citation2014). Pathogen levels in the field soil samples are reported as resting spores g−1 soil, while in the root tissue samples they are reported as resting spores g−1 root tissue.
Statistical analysis
All statistical analyses were performed using RStudio v. 1.1.463 (2009–2018 RStudio, Inc.). Regression analysis was conducted on field results from 2017 and 2018 when Pearson’s correlation coefficient was significant. If correlation was not significant, the data were subjected to a one-way analysis of variance based on the Tukey test. The plant parameters analyzed included canola germination, plant height, shoot weight, root weight, yield and clubroot ID to evaluate the efficacy of hydrated lime vs non-treated controls. A logarithmic transformation was applied to clubroot ID to normalize the data and a non-parametric test (Krustal-Wallis) was used to evaluate plant height. Non-transformed means are presented for consistency. For all analyses, differences were considered significant at P < 0.05.
An analysis of variance using the General Linear Model was used to analyze which factors significantly affected each parameter measured following a gamma distribution. Effects of cultivar, inoculum concentration, lime product and lime rate were assessed for the individual plant measurements of height and disease severity rating, and the net dry (five plants per replicate) root and shoot weight were subjected to a mean separation based on least-squares mean, Tukey test. These same factors were also evaluated for their effect on the quantity of P. brassicae DNA in 10-day-old root samples as determined by q-PCR analysis. In the greenhouse trials, ‘year’ was considered as a block because the trials were conducted in separate greenhouses using different inoculum sources, and as a result, the two trials are presented and analyzed separately. The means of each parameter are presented in this study. The data represent the means of five replicates in the greenhouse study and three replicates in the q-PCR analysis. Differences were considered significant at P < 0.05 unless otherwise specified.
Results
Field trials
In 2017, the high and moderate rates of lime reduced clubroot severity compared with the low rate and the control treatment at site 1. The average ID for the control treatment (pH 6.3) at site 1 was 47%, and the ID for the lowest rate of lime was 37.5% (). The moderate and high rates of lime (8.0 and 11.4 t ha−1) reduced ID to 6.7%, and 4%, respectively. The log-transformed ID and lime rate were positively correlated (R2 = 0.46, P = 0.0023, F(1,14) = 13.78). As determined by q-PCR, the average P. brassicae resting spore concentration in the soil at site 1 (2017) was 3.3 × 103 spores g−1 soil (range of 1.65 × 103 to 5.05 × 103 spores g−1 soil). The averages of the second site in 2017 are included for interest in , but since an error in staking resulted in only the first two replicates receiving the targeted rate of lime, a full statistical analysis could not be conducted.
Table 1. Effects of hydrated lime (Ca(OH)₂) on clubroot index of disease and plant growth parameters of the clubroot-susceptible canola ‘45H31ʹ under field conditions in Edmonton, Alberta, in 2017 and 2018.a
In 2018, there was no treatment effect observed at either site (). Site 1 developed no visible symptoms of clubroot in three of the replicates and only very mild symptoms in the fourth replicate. The ID in the control treatment was 3% and IDs varied from 1% to 4% across the various lime treatments. As was the case in 2017, clubroot disease pressure was higher at site 2. The average ID in the control treatment was 61%, which was not significantly different from the 67% to 68% observed across the various lime treatments. There were no significant correlations observed in 2018 between clubroot severity and lime application. The average P. brassicae resting spore concentration was estimated via q-PCR analysis and was 1.22 × 104 spores g−1 soil (range of 2.59 × 103 to 4.34 × 104 spores g−1 soil) at site 1 and 8.55 × 105 spores g−1 soil (range of 1.39 × 103 to 8.27 × 106 spores g−1 soil) at site 2 in 2018.
There were no significant differences observed in root or shoot weight, plant height or yield at site 1 in 2017. However, there was a correlation (R2 = 0.3513, P = 0.009, F(1,14) = 9.124) between the log-transformed ID and root weight. Above-ground biomass and lime rate also were correlated (R2 = 0.3319, P = 0.011, F(1,14) = 8.45). Similarly, seedling emergence was affected significantly by lime rate. The lowest rate of lime reduced the emergence count by 16%. The moderate to high rates of lime reduced emergence by 40% and 46%, respectively, compared with the control, but did not differ significantly from each other. Although there was a significant effect of lime rate on canola seedling emergence, yield remained unchanged or increased slightly while ID decreased ().
Greenhouse trials
In the first greenhouse trial conducted in 2017, clubroot severity was significantly affected by the canola cultivar grown (F(1,398) = 446.9, P < 2.2 × 10−16), lime product applied (F(2,396) = 376.6, P < 2.2 × 10−16), inoculum concentration (F(3,393) = 8.6, P = 1.56 × 10−5), and their interactions (cultivar*inoculum (P = 0.000275), cultivar*lime product (P = 5.06 × 10−5), lime*inoculum (P < 2.2 × 10−16), cultivar*lime*inoculum (P = 6.69 × 10−8)). The susceptible cultivar ‘45H31ʹ developed an ID of 92% to 100% across all resting spore concentrations in the absence of lime amendments. The application of hydrated lime at any of the rates evaluated resulted in a decrease in ID to 0% across all spore concentrations, with the exception of the rate 8.1 t ha−1 at 1 × 106 spores g−1 medium, which developed an ID of 18% (). On the resistant cultivar ‘9558C’, ID ranged from 9% to 13% in the absence of lime amendments; this declined to 0% across all resting spore concentrations when hydrated lime was applied at any of the rates examined. The reduction in ID was less pronounced with the application of limestone (). On the susceptible canola ‘45H31ʹ, significant reductions in ID were observed only at the lower resting spore concentrations (1 × 103 and 1 × 104 spores g−1 medium), and limestone treatment at any rate had no effect on ID at spore concentrations of 1 × 105 and 1 × 106 spores g−1 medium. Similar trends were observed with the canola cultivar ‘9558C’, except that ID values generally were lower. As was observed with ‘45H31ʹ, the application of limestone at any rate did not appear to have a consistent effect on ID on ‘9558C’, relative to the control treatments, at 1 × 105 and 1 × 106 spores g−1 medium.
Table 2. Effects of hydrated lime (Ca(OH)₂) on clubroot index of disease (ID), canola plant height, dry shoot weight and dry root weight under greenhouse conditions 8 weeks after planting, in 2017 and 2018.a
Table 3. Effects of limestone (CaCO₃) on clubroot index of disease (ID), canola plant height, dry shoot weight and dry root weight under greenhouse conditions 8 weeks after planting, in 2017 and 2018.a
When the greenhouse trial was repeated in 2018, the IDs in the control treatments were generally lower than in 2017 at the lower inoculum concentrations, but similar at the higher inoculum concentrations (). Overall, the trends in 2018 were consistent with those observed in 2017, and ID was significantly affected by cultivar (F(1,398) = 356.2, P < 2.2 × 10−16), lime product (F(2,396) = 247.7, P < 2.2 × 10−16), inoculum concentration (F(3,393) = 46.0, P < 2.2 × 10−16), and the interactions of cultivar*inoculum (P = 0.00069). On the clubroot-susceptible canola ‘45H31ʹ, ID in the non-limed control treatments increased from 10% at 1 × 103 resting spores g−1 medium to 100% at 1 × 106 spores g−1 medium. The application of any rate of hydrated lime resulted in no visible symptoms of clubroot (ID of 0%) at any of the resting spore concentrations evaluated (). On the resistant cultivar ‘9958C’, ID values in the controls ranged from 0% at 1 × 103 resting spores g−1 medium to 8% at 1 × 106 spores g−1 medium, and treatment with any rate of hydrated lime resulted in an ID of 0% across inoculum levels. As was observed in the first trial, the application of limestone reduced IDs mainly at the lower spore concentrations (). On ‘45H31ʹ, any rate of limestone reduced ID to 0% at the lowest inoculum concentration (1 ×103 spores g−1 medium); at 1 ×104 spores g−1 medium, all rates also significantly reduced ID, but ID was 0% only at the two highest rates. Clubroot development also decreased significantly with application of limestone at 1 ×104 spores g−1 medium to 1 ×106 spores g−1 medium; however, the lowest ID obtained increased with increasing inoculum concentration. On the resistant canola ‘9558C’, no clubroot symptoms developed on the control or any of the treatments at 1 × 103 spores g−1 medium, and no clear trends or significant differences were observed with limestone treatment at any of the higher spore concentrations. Indeed, at spore concentrations from 1 × 104 to 1 × 106 spores g−1 medium, IDs fluctuated in a narrow range from 0% to 12% across all limestone rates.
In the first greenhouse trial conducted in 2017, plant height was affected by cultivar (F(1,397) = 324.4, P < 2.2 × 10−16), lime product (F(2,395) = 77.6, P < 2.2 × 10−16), inoculum concentration (F(3,389) = 153.1, P < 2.2 × 10−16) and interactions (cultivar*lime (P < 2.2 × 10−16), inoculum*cultivar (P = 55.0 × 10−15), inoculum*lime (P < 2.2 × 10−16), rate*inoculum (P = 5.3 × 10−12), cultivar*inoculum*lime (P = 1.2 × 10−13), inoculum*rate*lime (P = 6.6 × 10−09), and cultivar*inoculum*rate*lime (P = 0.02785)). On the susceptible canola ‘45H31ʹ, the most pronounced effects on plant height were observed at the higher spore concentrations (1 × 105 to 1 × 106 spores g−1 medium), while plant heights fluctuated close to the height of the control treatments at the lower concentrations (). On the resistant cultivar ‘9558C’, no clear trend was discernible with respect to hydrated lime treatment and plant height. There were some rates that reduced plant height by 2 cm to 13 cm, with the largest reductions observed at the lower inoculum concentrations, and there were also rates that increased plant height by 2 cm to 8 cm with the tallest plants observed at the higher inoculum concentration; however, the differences were not significant compared with the control. All rates of limestone appeared to increase the height of ‘45H31ʹ, by as much as 22 cm relative to the control treatments, with most differences significant at inoculum concentrations of 1 × 105 spores g−1 medium or 1 × 106 spores g−1 medium (). On ‘9558C’, significant increases in plant height were observed with increasing rates of limestone at concentrations of 1 × 103 spores g−1 medium or 1 × 105 spores g−1 medium, and declined at the highest inoculum, compared with the control.
Dry shoot weight was affected by cultivar (F(1,398) = 96.0, P < 2.2 × 10−16), inoculum (F(3,395) = 26.8, P = 9.2 × 10−16), lime product (F(2,393) = 6.0, P = 0.0027) and lime rate (F(1,392) = 22.4, P = 3.1 × 10−06) with significant interactions of lime inoculum*lime (P = 1.0 × 10−06), and inoculum*rate (P = 5.1 × 10−07). All rates of hydrated lime increased dry shoot weight on ‘45H31ʹ relative to the control treatments, with these increases most significant at the higher inoculum levels (1 × 105 and 1 × 106 spores g−1 medium). In the case of the partially resistant cultivar ‘9558C’, however, the effect of rate resulted in significant reductions in dry shoot weight of 21% to 94% with increasing rates of hydrated lime at all inoculum levels except for 1 × 105 spores g−1 medium (). Limestone treatment resulted in significant increases in the shoot weight of ‘45H31ʹ at all inoculum levels except 1 × 106 spores g−1 medium (); on ‘9558C’, limestone increased dry shoot weight at the two lowest rates of limestone and two lowest inoculum concentrations. At the two higher inoculum concentrations, the effect of limestone on ‘9558C’ did not follow any trend.
Dry root weight was affected by cultivar (F(1,394) = 642.7, P < 2.2 × 10−16), lime product (F(2,392) = 79.6, P < 2.2 × 10−16), inoculum concentration (F(3,396) = 57.4, P < 2.2 × 10−16), rate (F(1,395) = 211.9, P < 2.2 × 10−16) and their interactions (cultivar*lime (P = 0.0027), inoculum*cultivar (P = 5.1 × 10−05), inoculum*lime (P < 2.2 × 10−16), rate*cultivar (P = 0.00095), inoculum*rate (P = 9.1 × 10−13), inoculum*rate*cultivar (P = 0.041), and inoculum*rate*lime (P = 0.0021)). Hydrated lime reduced the dry root weight of both ‘45H31ʹ and ‘9558C’, likely because of the reduction or elimination of root clubs. In the susceptible cultivar ‘45H31ʹ, the average root weight of the control treatments ranged from 3.15 g to 3.71 g, while in treatments receiving hydrated lime, the dry root weight significantly decreased and ranged from 0.26 g to 0.90 g due to the elimination of galling (). In the resistant cultivar ‘9558C’, the average root weight of the control treatments was 0.70 g to 0.93 g, but any rate of hydrated lime reduced this weight further at all inoculum concentrations. Similar trends were observed with the application of limestone, in a manner consistent with the decreases in clubroot severity observed for those treatments ().
The patterns observed when the greenhouse trial was repeated in 2018 resembled those in 2017. Plant height was significantly affected by cultivar (F(3,396) = 59.5, P < 2.2 × 10−16), lime product (F(1,395) = 59.3, P = 1.2 × 10−13) and inoculum concentration (F(2,393) = 26.7, P = 1.2 × 10−11) and interactions including cultivar*lime (P < 2.2 × 10−16), inoculum*lime (P = 1.3 × 10−09) and inoculum*cultivar*lime (P < 2.2 × 10−16). On ‘45H31ʹ, plant height increased significantly by 0.4 cm to 24.1 cm when subjected to hydrated lime treatments, with the largest changes occurring at the higher inoculum concentrations (). In the partially resistant cultivar ‘9558C’, the application of hydrated lime resulted in no significant changes in plant height at the three lower inoculum concentrations, regardless of rate, while a significant reduction in plant height was observed at some rates at the highest inoculum concentration. The application of limestone significantly increased the height of ‘45H31ʹ by 2.2 cm to 33.9 cm across all inoculum concentrations (). In the case of cultivar ‘9558C’, plant height following limestone application varied by −29.5 cm to +12.7 cm compared with the control across all inoculum concentrations, generating significantly taller plants at some rates at the three lowest inoculum concentrations and significantly shorter plants at the highest inoculum concentration.
Dry shoot weight in the 2018 trial was again affected by cultivar (F(1,394) = 41.1, P = 4.5 × 10−10), inoculum concentration (F(3,395) = 24.2, P = 2.8 × 10−14) and lime product (F(2,392) = 7.8, P = 0.00048), plus the interactions of inoculum*lime (P = 9.1 × 10−15), inoculum*cultivar (P = 6.0 × 10−15), cultivar*lime (P = 8.9 × 10−13), inoculum*lime*rate (P = 0.043), inoculum*cultivar*lime (P < 2.2 × 10−16) and cultivar*lime*rate (P = 0.021). On ‘45H31ʹ, hydrated lime generally increased dry shoot weight significantly at all inoculum levels except 1 × 103 spores g−1 medium, relative to the control treatments; the largest increases were observed at the highest inoculum level (). On ‘9558C’, treatment with hydrated lime produced no clear significant differences at the two lowest inoculum concentrations. At 1 × 106 spores g−1 medium, however, almost all rates of hydrated lime significantly reduced dry shoot weight. Limestone application resulted in increased dry shoot weight in ‘45H31ʹ at the three highest inoculum levels, but was associated with small decreases (1 to 6%) in dry shoot weight at the lowest inoculum concentration (). On ‘9558C’, the limestone treatments increased dry shoot weight only at 1 × 105 spores g−1 medium and decreased it at 1 × 106 spores g−1 medium, compared with the control, regardless of rate.
Dry root weight was affected by cultivar (F(1,394) = 400.7, P < 2.2 × 10−16), inoculum concentration (F(3,395) = 107.4, P < 2.2 × 10−16) and lime product (F(2,392) = 206.2, P < 2.2 × 10−16), as well as the interactions of inoculum*lime (P < 2.2 × 10−16), inoculum*cultivar (P =0.022), cultivar*lime (P = 6.0 × 10−08), inoculum*cultivar*rate (P = 0.0015), inoculum*cultivar*lime (P = 9.4 × 10−07) and inoculum*cultivar*lime*rate (P = 0.012). Hydrated lime significantly reduced dry shoot weight at the three highest inoculum concentrations for ‘45H31ʹ, and at 1 × 106 spores g−1 medium for ‘9558C’ (). This is likely due to the elimination or reduction of root galling as noted earlier. Similarly, the application of limestone reduced the dry root weight in ‘45H31ʹ relative to the control at the three highest inoculum concentrations, but not to the same extent as hydrated lime. A significant reduction in root weight was observed for ‘9558C’ following limestone application at an inoculum concentration of 1 × 106 spores g−1 medium ().
Quantification of P. brassicae in host roots
In the first greenhouse trial conducted in 2017, the ability of P. brassicae to colonize host root tissues was significantly affected by lime product (F(2,231) = 99.4, P < 2.2 × 10−16), rate (F(3,228) = 9.0, P = 1.5 × 10−05) and inoculum concentration (F(3,225) = 301.1, P < 2.2 × 10−16). All interactions including those with cultivar were significant (from P = 0.001 to P < 2.2 × 10−16). The amount of pathogen DNA in the roots of the seedlings, expressed as resting spores g−1 root tissue, decreased with increasing rate of hydrated lime in both the susceptible canola ‘45H31ʹ and the resistant ‘9558C’ (). No pathogen was detected in the roots of either cultivar at the lowest inoculum concentration (1 × 103 spores g−1 medium) following treatment with any rate of hydrated lime. When the inoculum concentration increased to 1 × 104 spores g−1 medium, reduced levels of P. brassicae were measured in the roots of both cultivars at 4.7 t ha−1 hydrated lime, but rates of ≥ 8.1 t ha−1 were sufficient to eliminate any detectable infection. Similarly, at inoculum concentrations of 1 × 105 and 1 × 106 spores g−1 medium, rates of ≥ 11.4 or 14.8 t ha−1 hydrated lime, respectively, were required to eliminate any detectable levels of P. brassicae in the roots, although lower rates of lime did decrease the amount of the pathogen relative to the controls. Significant differences in the amount of the pathogen were detected only at the highest inoculum concentration for hydrated lime when the cultivar ‘45H31ʹ was grown. The application of limestone generally resulted in one- to two-fold reductions in the amount of P. brassicae in the roots of both ‘45H31ʹ and ‘9558C’, but significant differences in the amount of pathogen present were only observed at the highest inoculum concentration when limestone was applied and the cultivar ‘45H31ʹ was grown ().
Fig. 1 The effects of hydrated lime in 2017 on the amount of Plasmodiophora brassicae in the roots of 10-day-old seedlings of the canola cultivars (a) ‘45H31ʹand (b) ‘9558C’ as determined by quantitative PCR analysis. The seedlings were grown under greenhouse conditions in potting medium inoculated with 1 × 103, 1 × 104, 1 × 105 and 1 × 106 resting spores g−1 potting medium and treated with 4.7, 8.1, 11.4 or 14.8 T ha−1 of hydrated lime, which targeted a pH of 6.0, 6.5, 7.0 or 7.5, respectively. Controls did not receive any lime (pH 5.3). Means with no letters do not differ; means differences are denoted by different letters at P < 0.05.
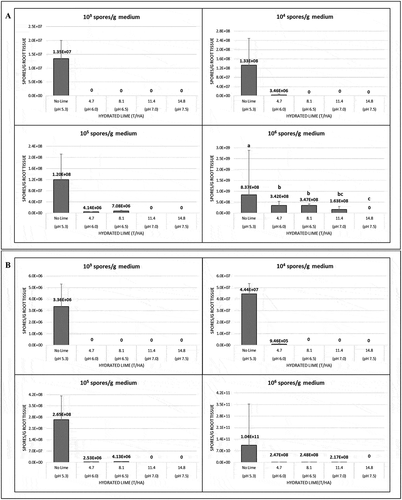
Fig. 2 The effects of limestone in 2017 on the amount of Plasmodiophora brassicae in the roots of 10-day-old seedlings of the canola cultivars (a) ‘45H31ʹ and (b) ‘9558C’ as determined by quantitative PCR analysis. The seedlings were grown under greenhouse conditions in potting medium inoculated with 1 × 103, 1 × 104, 1 × 105 and 1 × 106 resting spores g−1 potting medium and treated with 4.7, 8.1, 11.4 or 14.8 T ha−1 of limestone, which targeted a pH of 6.0, 6.5, 7.0 or 7.5, respectively. Controls did not receive any lime (pH 5.3). Means with no letters do not differ; means differences are denoted by different letters at P < 0.05.
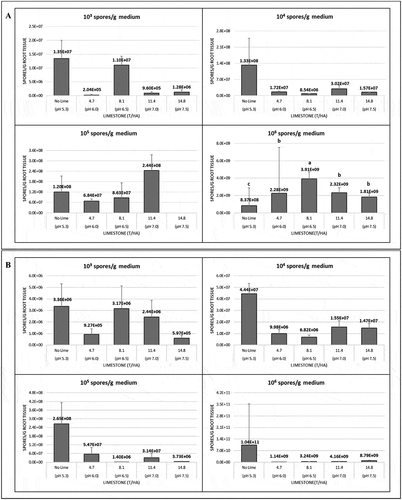
In the second greenhouse trial conducted in 2018, all factors, cultivar (F(1,234) = 21.7, P = 6.6 × 10−06), lime product (F(2,232) = 105.9, P < 2.2 × 10−16), lime rate (F(3,229) = 3.7, P = 0.013) and inoculum concentration (F(3,226) = 84.3, P < 2.2 × 10−16), as well as all interactions significantly (ranging from P = 0.012 to P < 2.2 × 10−16) affected the amount of P. brassicae detected in the roots. The pathogen was not detectable in roots of the cultivar ‘45H31ʹ following the application of hydrated lime to soil that had been inoculated with 1 × 103, 1 × 104 and 1 × 105 spores g−1 medium (). At the highest inoculum level (1 × 106 spores g−1 medium), a rate of ≥ 8.1 t ha−1 hydrated lime was required to eliminate pathogen infection. In ‘9558C’, any rate of hydrated lime was sufficient to prevent detection of P. brassicae in the roots of seedlings grown in inoculum concentrations of 1 × 103 and 1 × 105 spores g−1 medium 1. The lowest rate of hydrated lime reduced the amount of pathogen detected in the tissues, when grown at inoculum concentrations of 1 × 104 and 1 × 106 spores g−1 medium, but a rate ≥ 8.1 t ha−1 was needed to eliminate infection. However, significant differences in the amount of pathogen present were found only at the highest inoculum concentration for both cultivars when hydrated lime was applied. When limestone was incorporated in the soil, detectable P. brassicae in the roots was eliminated only at the lowest inoculum concentration. In ‘45H31ʹ, detectable infection was eliminated at limestone rates of 4.7, 11.4 and 14.8 t ha−1, but was only reduced at 8.1 t ha−1. In ‘9558C’, all rates of limestone eliminated detectable infection by the pathogen at the lowest inoculum concentration, but no significant differences were observed compared with the control at any of the other rates. Significant differences in the amount of pathogen detected in the roots were observed only at inoculum concentrations of 1 × 104 and 1 × 106 spores g−1 medium in both cultivars when limestone was applied ().
Fig. 3 The effects of hydrated lime in 2018 on the amount of Plasmodiophora brassicae in the roots of 10-day-old seedlings of the canola cultivars (a) ‘45H31ʹ and (b) ‘9558C’ as determined by quantitative PCR analysis. The seedlings were grown under greenhouse conditions in potting medium inoculated with 1 × 103, 1 × 104, 1 × 105 and 1 × 106 resting spores g−1 potting medium and treated with 4.7, 8.1, 11.4 or 14.8 T ha−1 of hydrated lime, which targeted a pH of 6.0, 6.5, 7.0 or 7.5, respectively. Controls did not receive any lime (pH 5.3). Means with no letters do not differ; means differences are denoted by different letters at P < 0.05.
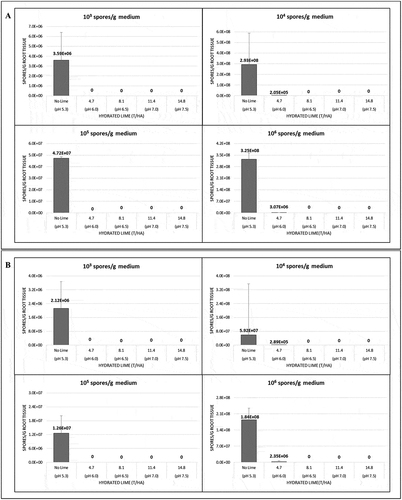
Fig. 4 The effects of limestone in 2018 on the amount of Plasmodiophora brassicae in the roots of 10-day-old seedlings of the canola cultivars (a) ‘45H31ʹ and (b) ‘9558C’ as determined by quantitative PCR analysis. The seedlings were grown under greenhouse conditions in potting medium inoculated with 1 × 103, 1 × 104, 1 × 105 and 1 × 106 resting spores g−1 potting medium and treated with 4.7, 8.1, 11.4 or 14.8 T ha−1 of limestone, which targeted a pH of 6.0, 6.5, 7.0 or 7.5, respectively. Controls did not receive any lime (pH 5.3). Means with no letters do not differ; means differences are denoted by different letters at P < 0.05.
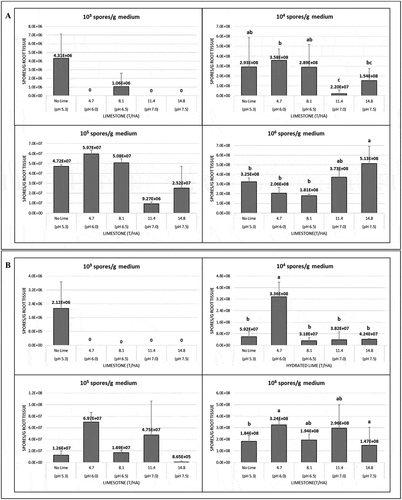
Discussion
Based on the results of the field trials, there may be some promise for the use of hydrated lime as a management tool for clubroot on canola. In 2017, all rates of hydrated lime reduced clubroot severity compared with the control treatment. At site 1, ID was reduced by about 90% at the highest (11.4 t ha−1) and moderate (8.0 t ha−1) rates of lime. Despite these significant decreases in clubroot severity, the yields remained similar. This may reflect that canola seedling emergence decreased significantly at the two highest rates of lime, so that any gains in yield resulting from reduced clubroot may have been offset by decreased plant density. Nonetheless, a reduction in clubroot severity could contribute to inoculum management, by reducing the number of new P. brassicae resting spores added to the soil (Shinoda et al. Citation2003). Murakami et al. (Citation2002) reported a reduction in resting spores resulting from the application of lime. Similar results were observed at site 2 in 2017, but unfortunately, two replicates were lost due to a staking error. Nonetheless, visually the plants appeared much healthier in the lime treatments versus the controls, with reduced ID and numerical increases in plant height and yield recorded with increasing rates of lime. The effects of liming have been reported to be greater on those soils with the lowest pH (Li et al. Citation2018), which may explain why site 2 (pH 5.1) showed a yield improvement although the reduction in clubroot severity was less than at site 1 (pH 6.3). Moreover, at this level of acidity, micronutrient availability is reduced and concentrations of Al+3 become toxic (Bhering et al. Citation2017), which could have caused deleterious effects on plant health in the controls and enhanced the effect of liming in the treated plots.
In contrast to the results in 2017, hydrated lime did not reduce clubroot severity when the field trial was repeated in 2018. At site 1, this may have reflected much lower disease pressure in 2018. Nonetheless, mean shoot weight, plant height and yield improved slightly with increasing lime rate, while the germination rate decreased significantly only at the highest rate of lime. The improvement in plant growth can likely be attributed to the increase in pH to a level where soil conditions were more favourable for plant growth (Bhering et al. Citation2017; Li et al. Citation2018). At site 2 in 2018, clubroot was severe in all plots (mean IDs of 60–67%), yet there was no effect of liming on clubroot severity. This was likely due to the length of the interval between liming and sowing in 2018 vs 2017. In 2017, canola was seeded 1 week after lime application, and the plots received a significant amount of rainfall 2 days after application, allowing the lime to dissolve, alter pH and increase calcium, all of which are known to create an adverse environment for P. brassicae (Webster and Dixon Citation1991). In contrast, in 2018, the canola was re-seeded 4 weeks after lime application due to cutworm damage, and no significant rainfall occurred. This likely contributed to a reduced response in emergence, disease severity and yield to the lime application, as not all the lime had dissolved even at harvest. Murakami et al. (Citation2002) found that the interval between liming and sowing is critical for the efficacy of lime treatments, with greater decreases in clubroot severity observed when soils were limed 2 weeks instead of 4 weeks prior to sowing of the crop. Other studies have reported similar results, which led Dixon (Citation2009) in a review of the literature to conclude that a high pH and calcium influx must be present within the first 3–7 days of inoculation for observable declines in clubroot. Others have reported inconsistency in treatments over sites and years, due to the many soil and environmental factors that can affect the efficacy of soil amendments, such as hydrated lime (Hwang et al. Citation2011b; Li et al. Citation2018).
Although these experiments were not designed to evaluate the effect of timing on the efficacy of hydrated lime for clubroot control, the results confirm earlier studies indicating the importance of targeting the appropriate period between lime application and seeding. Based on these results and comparison with previously published literature, in 2017 the timing between sowing and lime application likely occurred within the optimal range; and while the environmental conditions were more conducive for clubroot development in 2017, they were also favourable for lime activation, thus resulting in significant decreases in disease severity. In 2018, however, significant rainfall did not occur to dissolve the lime, plus the trial had to be re-seeded weeks later; therefore, sowing of the canola occurred outside of the optimal period following lime application. This suggests that under ideal conditions (timely rainfall and planting), liming could be an effective tool to supplement genetic resistance and manage resting spore production by the pathogen.
In the greenhouse experiments, all rates of hydrated lime reduced ID to 0% on both the susceptible and resistant canola cultivars. The small pot size and the controlled conditions may have improved the efficacy of the treatments relative to the field study (Li et al. Citation2018). Treatment with lime resulted in increased plant height and shoot weight in the susceptible canola cultivar ‘45H31ʹ, likely reflecting the elimination of clubroot symptom development. In contrast, in the resistant ‘9558C’, plant height and shoot weight remained similar or decreased slightly, possibly because even the control plants of this cultivar developed only mild clubroot symptoms, and the hydrated lime may have had some phytotoxic effects. The effects of limestone (calcium carbonate) on clubroot reduction were less pronounced than the effects of hydrated lime. This may reflect, at least in part, that the same rates (t ha−1) of the two products were compared; given that the calcium carbonate equivalent (CCE) of hydrated lime is 135%, it can neutralize 1.35× as much acid as the same amount of limestone (Havalin et al. Citation2004). As such, the latter would have been less effective at reducing the acidity of the potting medium (Donald and Porter Citation2009). Clubroot severity declined with increasing rate of limestone only at the two lowest inoculum levels, while no disease suppression occurred above 1 × 105 spores g−1 medium. Consequently, the impact on other growth parameters, such as plant height and shoot weight were affected similarly. In the resistant ‘9558C’, no general trend in height and only slight increases in shoot weight were observed following limestone treatment. The amount of clubroot control that limestone can provide appears to be limited and seems restricted to situations where the P. brassicae inoculum concentration is low (<1 × 104 spores g−1 medium). This may help to explain why little benefit was observed from limestone application in earlier studies conducted in Alberta (Hwang et al. Citation2011b), since P. brassicae infestations ≥1 × 108 spores g−1 soil have been reported in some fields in this province. The greenhouse experiments further underscored the importance of factors such as cultivar, lime product and inoculum concentration on the effectiveness of liming. While hydrated lime gave better control relative to limestone, phytotoxic effects may be observed on canola plants depending on the cultivar used. Webster and Dixon (Citation1991) were able to eliminate clubroot development under high pH and calcium concentrations, but also found phytotoxic effects on the host plants.
The extent to which liming affected the ability of P. brassicae to proliferate in the host roots under greenhouse conditions was evaluated by q-PCR analysis. As was the case with reductions in clubroot symptom development, hydrated lime was more effective than limestone at reducing the amount of the pathogen in the roots. Root hair infection has been reported to be linearly proportional to inoculum concentration (Webster and Dixon Citation1991), and this study showed that the amount of lime needed to eliminate root hair infection increased with increasing inoculum concentration. Moreover, resting spore age, maturity and the relative prevalence of non-viable spores can alter the slope of the linear relationship between root hair infection and spore concentration (MacFarlane Citation1952; Dixon Citation2009; Peng et al. Citation2015). Webster and Dixon (Citation1991) also investigated the effects of pH on primary (root hair) infection and clubroot development in the absence and presence of calcium and observed both independent and synergistic effects of pH and calcium on the lifecycle of P. brassicae (Webster Citation1986). An increase in soil pH and calcium has been hypothesized to inhibit resting spore germination, but has also been suggested to affect the ability of P. brassicae to respond to root exudates in the rhizosphere, or to cause changes in the quality and quantity of the exudates themselves (Niwa et al. Citation2008). The increase in soil pH may also affect the rate of production of primary zoospores in infected host tissue (Bochow Citation1961), and suppress invasion by zoospores, zoosporangial maturation and clubroot symptom formation (Niwa et al. Citation2008).
The importance of pH and calcium in clubroot development underscores the potential benefits of liming as a clubroot management strategy. With over 400 000 hectares of strongly acidic (pH of 5.1 to 5.5) soil and 1.8 million hectares of acidic (pH of 5.6 to 6.0) soil farmed in Alberta (Government of Alberta Citation2002), liming should already be incorporated into many farm management plans to improve plant growing conditions. While lime treatments hold some promise for clubroot mitigation on canola, the type of lime selected is important, and the extent of control afforded may be influenced by the timing of application as well as numerous environmental and soil factors. This study highlighted the complex interactions of lime product and initial soil pH with pathogen resting spore concentration and virulence, as well as the need for an appropriate amount of timely rainfall after application and prior to seeding. Nonetheless, this study also demonstrated that the application of lime treatments could significantly reduce clubroot severity and the proliferation of P. brassicae in the root tissues. Such effects may not only serve to improve plant health, but may also contribute to long-term clubroot management by reducing selection pressure on pathogen populations and controlling soil inoculum loads. While proliferation of P. brassicae is reduced in CR cultivars, infection of these cultivars can still contribute new resting spores to the soil, resulting in increases in the spore concentration, presumably enriched for pathotypes capable of overcoming resistance (Hwang et al. Citation2011a; Ernst et al. Citation2019). While hydrated lime appears to provide superior clubroot control, at a cost of about $320 CAD tonne−1 it is considerably more expensive than limestone ($54 CAD tonne−1). In fields that are infested only mildly with P. brassicae, the application of limestone may be sufficient as a tool to manage the pathogen. Liming an entire field may never be economical (Donald and Porter Citation2009; Hwang et al. Citation2011b), but the application of lime to P. brassicae-infested patches in a field, such as hot spots and field entrances, could be an important strategy for clubroot management in canola. Integrated clubroot management strategies that reduce or at least maintain resting spores at low levels will reduce inoculum pressure and infection, and hence also reduce the chances for pathotype shifts, contributing to the stewardship of resistant cultivars (Diederichsen et al. Citation2003; Deora et al. Citation2011; Peng et al. Citation2011, Citation2013, Citation2015; Hwang et al. Citation2012).
Future studies need to consider multiple seeding dates after the same and different lime product applications, since the efficacy of treatments may vary depending on the lime product used and amount of rain received. The effect of lime on root colonization in the greenhouse could be used as a baseline for similar comparisons under field conditions. The monitoring of resting spore concentrations in the soil would also provide important information on the impact of liming on soil inoculum loads. Time-courses examining proliferation of P. brassicae in infected roots at multiple points following lime treatment may also help to understand their impact on disease development. Given the variability observed when using different inoculum sources (i.e., less virulent collections), it may be worthwhile to directly evaluate the effect of resting spore viability/virulence on the effectiveness of lime.
Acknowledgements
The authors wish to thank the undergraduate summer students and graduate students at the University of Alberta who assisted with setting up the experiments and data collection, as well as Ileana Strelkov and Tiesen Cao for helpful discussions.
Additional information
Funding
References
- Al-Daoud F, Megan M, Gossen BD, McDonald MR. 2017. First report of clubroot (Plasmodiophora brassicae) on canola in Ontario. Can J Plant Pathol. 40(1):96–99. doi:https://doi.org/10.1080/07060661.2017.1393696.
- Bhering ADS, do Carmo MG, Matos TDS, Lima ES, do Amaral Sobrinho NM. 2017. Soil factors related to the severity of clubroot in Rio de Janeiro, Brazil. Plant Dis. 101(8):1345–1353. doi:https://doi.org/10.1094/PDIS-07-16-1024-SR.
- Bochow H. 1961. Uber die Beeinflessung von Plasmodiophora brassicae Wor. durch Mineralsalze [The influence of Plasmodiophora brassicae Wor. through mineral salts]. Phytopathol. 40::420–421. doi:https://doi.org/10.1111/j.1439-0434.1960.tb01935.x.
- Campbell RN, Grethead AS. 1989. Control of clubroot of brassicas by liming. In: Engelhard AW, editor. Soilborne plant pathogens: management of diseases with macro- and microelements. St. Paul (MN): APS Press; p. 90–101.
- Cao T, Rennie DC, Manolii VP, Hwang SF, Falak I, Strelkov SE. 2014. Quantifying resistance to Plasmodiophora brassicae in Brassica hosts. Plant Pathol. 63:715–726. doi:https://doi.org/10.1111/ppa.12113.
- Cao T, Tewari J, Strelkov SE. 2007. Molecular detection of Plasmodiophora brassicae, causal agent of clubroot of crucifers, in plant and soil. Plant Dis. 91(1):80–87. doi:https://doi.org/10.1094/PD-91-0080.
- Chittem K, Mansouripour SM, del Río Mendoza LE. 2014. First report of clubroot on canola caused by Plasmodiophora brassicae in North Dakota. Plant Dis. 98(10):1438. doi:https://doi.org/10.1094/PDIS-04-14-0430-PDN.
- Deora A, Gossen BD, Walley F, McDonald MR. 2011. Boron reduces development of clubroot in canola. Can J Plant Pathol. 33(4):475–484. doi:https://doi.org/10.1080/07060661.2011.620630.
- Diederichsen E, Deppe U, Sacristán MD. 2003. Characterization of clubroot resistance in recent winter oilseed rape material. Proceedings of the 11th International Rapeseed Congress; July 6; Copenhagen. p. 68–70.
- Dixon GR. 2009. Plasmodiophora brassicae in its environment. J Plant Growth Regul. 28(3):212–228. doi:https://doi.org/10.1007/s00344-009-9098-3.
- Donald C, Porter I. 2009. Integrated control of clubroot. J Plant Growth Regul. 28:194–202. doi:https://doi.org/10.1007/s00344-009-9094-7.
- Ernst TW, KherS Stanton D, Rennie DC, Hwang SF, Strelkov SE. 2019. Plasmodiophora brassicae resting spore dynamics in clubroot resistant canola (Brassica napus) cropping systems. Plant Pathol. 68:399–408. doi:https://doi.org/10.1111/ppa.12949.
- Froese RD, Derksen H, Guo X, McLaren DL. 2019. Monitoring and occurrence of clubroot in Manitoba in 2018. The Canadian phytopathological Society Canadian Plant Disease Survey: Disease Highlights 2019. Can J Plant Pathol. 41(S1):179.
- Government of Alberta. 2002. Agri-facts: liming acid soils. Agdex 534–1. [ Internet]. [Accessed 2017 June 7]. https://www.alberta.ca/soil-ph.aspx.
- Graymont Limited. 2019. Graymont products: high calcium hydrated lime and high calcium limestone. [ Internet]. [Accessed 2018 Dec 18]. http://www.graymont.com/en/products.
- Havalin JL, Tisdale SL, Nelson WL, Beaton JD. 2004. Soil fertility and fertilizers: an introduction to nutrient management. 7th ed. Upper Saddle River (NJ): Pearson Prentice Hall.
- Hollman KB, Hwang SF, Manolii VP, Strelkov SE. 2020. Pathotypes of Plasmodiophora brassicae collected from clubroot resistant canola (Brassica napus L.) cultivars in Western Canada in 2017–2018. Can J Plant Pathol. in press. doi:https://doi.org/10.1080/07060661.2020.1851893.
- Horiuchim S, Hori M. 1980. A simple greenhouse technique for obtaining high levels of clubroot incidence. Bull Chugoku Nat Agric Exp Stn Ser E. 17:33–35.
- Hwang SF, Ahmed HU, Strelkov SE, Gossen BD, Turnbull GD, Peng G, Howard RJ. 2011a. Seedling age and inoculum density affect clubroot severity and seed yield in canola. Can J Plant Sci. 91:183–190. doi:https://doi.org/10.4141/cjps10066.
- Hwang SF, Ahmed HU, Zhou Q, Strelkov SE, Gossen BD, Peng G, Turnbull GD. 2012. Assessment of the impact of resistant and susceptible canola on Plasmodiophora brassicae inoculum potential. Plant Pathol. 61:945–952. doi:https://doi.org/10.1111/j.1365-3059.2011.02582.x.
- Hwang SF, Strelkov SE, Gossen BD, Turnbull GD, Ahmed HU, Manolii VP. 2011b. Soil treatments and amendments for amelioration of clubroot of canola. Can J Plant Sci. 91:999–1010. doi:https://doi.org/10.4141/cjps2011-028.
- Kenny M, Oates T. 2000. Ullmann’s encyclopedia of industrial chemistry: lime and limestone. [place unknown]: Wiley-VCH.
- Kuginuki Y, Hiroaki Y, Hirai M. 1999. Variation in virulence of Plasmodiophora brassicae in Japan tested with clubroot-resistant cultivars of Chinese cabbage (Brassica rapa L. ssp. pekinensis). Eur J Plant Pathol. 105::327–332. doi:https://doi.org/10.1023/A:1008705413127.
- Li Y, Cui S, Chang SX, Zhang Q. 2018. Liming effects on soil pH and crop yield depend on lime material type, application method and rate, and crop species: a global meta-analysis. J Soils Sediments. 19(3):1393–1406. doi:https://doi.org/10.1007/s11368-018-2120-2.
- Lickacz J. 2002. Wood ash: an alternative liming material for agricultural soils. Alberta Agric Food Rural Dev. Agdex 534-2. https://open.alberta.ca/publications/2616494
- MacFarlane I. 1952. Factors affecting the survival of Plasmodiophora brassicae Wor. in the soil and its assessment by a host test. Ann Appl Biol. 39:239–256. doi:https://doi.org/10.1111/j.1744-7348.1952.tb00903.x.
- Murakami H, Tsushima S, Kuroyanagi Y, Shishido Y. 2002. Reduction of resting spore density of Plasmodiophora brassicae and clubroot disease severity by liming. Soil Sci Plant Nutr. 48(5):685–691. doi:https://doi.org/10.1080/00380768.2002.10409258.
- Niwa R, Nomura Y, Osaki M, Ezawa T. 2008. Suppression of clubroot disease under neutral pH caused by inhibition of spore germination of Plasmodiophora brassicae in the rhizosphere. Plant Pathol. 57(3):445–452. doi:https://doi.org/10.1111/j.1365-3059.2007.01817.x.
- Pageau D, Lajeunesse J, Lafond J. 2006. Impact of clubroot (Plasmodiophora brassicae) on the yield and quality of canola. Can J Plant Sci. 28:137–143.
- Peng G, Lahlali R, Hwang SF, Hynes RK, McDonald MR, Pageau D, Gossen BD, Strelkov SE, McGregor L. 2011. Control of clubroot on canola using the biofungicide Serenade plus cultivar resistance. Proceedings of the 13th International Rapeseed Congress; June 5; Prague (Czech Republic). p. 1134–1137.
- Peng G, Lahlali R, Hwang SF, Pageau D, Hynes RK, McDonald MR, Gossen BD, Strelkov SE. 2013. Crop rotation, cultivar resistance, and fungicides/biofungicides for managing clubroot (Plasmodiophora brassicae) on canola. Can J Plant Pathol. 36(1):99–112. doi:https://doi.org/10.1080/07060661.2013.860398.
- Peng G, Pageau D, Strelkov SE, Gossen BD, Hwang SF, Lahlali R. 2015. A> 2-year crop rotation reduces resting spores of Plasmodiophora brassicae in soil and the impact of clubroot on canola. Eur J Agron. 70:78–84. doi:https://doi.org/10.1016/j.eja.2015.07.007.
- Rennie DC, Manolii VP, Cao T, Hwang SF, Howard RJ, Strelkov SE. 2011. Direct evidence of surface infestation of seeds and tubers by Plasmodiophora brassicae and quantification of spore loads. Plant Pathol. 60:811–819. doi:https://doi.org/10.1111/j.1365-3059.2011.02449.x.
- Shinoda H, Murakami K, Goto I. 2003. An improved method for measurement of the resting spore density of Plasmodiophora brassicae from infested soils. Jpn J Soil Sci and Plant Nutr. 74(3):287 191.
- Soil Classification Working Group. 1998. The Canadian system of soil classification. 3rd ed. Agriculture and Agri-Food Canada Publication. [accessed 2021 Feb 7]. https://sis.agr.gc.ca/cansis/publications/manuals/1998-cssc-ed3/cssc3_manual.pdf.
- Somé A, Manzanares MJ, Laurens F, Baron F, Thomas G, Rouxel F. 1996. Variation for virulence on Brassica napus L. amongst Plasmodiophora brassicae collections from France and derived single-spore isolates. Plant Pathol. 45::432–439. doi:https://doi.org/10.1046/j.1365-3059.1996.d01-155.x.
- Strelkov SE, Hwang SF, Manolii VP, Cao T, Feindel D. 2016. Emergence of new virulence phenotypes of Plasmodiophora brassicae on canola (Brassica napus) in Alberta, Canada. Eur J Plant Pathol. 145(3):517–529. doi:https://doi.org/10.1007/s10658-016-0888-8.
- Strelkov SE, Hwang SF, Manolii VP, Cao T, Fredua-Agyeman R, Harding MW, Peng G, Gossen BD, Mcdonald MR, Feindel D. 2018. Virulence and pathotype classification of Plasmodiophora brassicae populations collected from clubroot resistant canola (Brassica napus) in Canada. Can J Plant Pathol. 40(2):284–298. doi:https://doi.org/10.1080/07060661.2018.1459851.
- Strelkov SE, Manolii VP, Aigu Y, Harding MW, Hwang SF, Daniels GC. 2021. The occurrence and spread of clubroot on canola in Alberta in 2020. Canadian Plant Disease Survey 2021 Vol. 101: Disease Highlights 2020. Can J Plant Pathol. 43(Supplement 1). Accepted 8 Jan 2021.
- Strelkov SE, Manolii VP, Cao T, Xue S, Hwang SF. 2007. Pathotype classification of Plasmodiophora brassicae and its occurrence in Brassica napus in Alberta, Canada. J Phytopathol. 155:706–712. doi:https://doi.org/10.1111/j.1439-0434.2007.01303.x.
- Strelkov SE, Manolii VP, Rennie DC, Xiao Q, Cui D, Hwang SF. 2011. The occurrence of clubroot on canola in Alberta in 2010. Can Plant Dis Surv. 91:109–111.
- Strelkov SE, Tewari JP, Smith-Degenhardt E. 2006. Characterization of Plasmodiophora brassicae populations from Alberta, Canada. Can J of Plant Pathol. 28:467–474. doi:https://doi.org/10.1080/07060660609507321.
- Webster MA. 1986. pH and nutritional effects on infection by Plasmodiophora brassicae Wor. and on clubroot symptoms [Dissertation]. University of Aberdeen.
- Webster MA, Dixon GR. 1991. Calcium, pH and inoculum concentration influencing colonization by Plasmodiophora brassicae. Mycol Res. 95:64–73. doi:https://doi.org/10.1016/S0953-7562(09)81362-2.
- Wellman FL 1930. Clubroot of crucifers. Washington (DC): Technical Bulletin 181 U.S. Department of Agriculture; [accessed 2021 Jan 21] https://naldc.nal.usda.gov/download/CAT86200175/PDF.
- Williams PH. 1966. A system for the determination of races of Plasmodiophora brassicae that infect cabbage and rutabaga. Phytopathology. 56:624–626.
- Ziesman B, Avila R, Ursu K, Kwasnicki J, Roszell L, Baraniecki C, Johnson B, Fennig C, Makohoniuk K, Junek S, et al. 2019. 2018 clubroot survey and known occurrence of clubroot in Saskatchewan. The Canadian Phytopathological Society Canadian Plant Disease Survey: Disease Highlights 2019. Can J Plant Pathol. 41(S1):159–161.