Abstract
Resistance breeding is an effective strategy against wheat stripe (yellow) rust caused by Puccinia striiformis f. sp. tritici (Pst). To identify and map quantitative trait loci (QTL) associated with stripe rust resistance, a durum wheat doubled haploid population (n = 87) derived from ‘Strongfield/Blackbird’ was evaluated for disease severity near Toluca, Mexico (2017–2019) and Lethbridge, Canada (2016–2019). The population was genotyped with the wheat 90 K Illumina iSelect single nucleotide polymorphism (SNP) array and simple sequence repeat (SSR) markers, and QTL analysis was performed with MapQTL 6. We identified stripe rust-resistance QTL contributed by ‘Blackbird’ on chromosomes 3A (2 loci, designated QYr.spa-3A.1, QYr.spa-3A.2) and 5B (QYr.spa-5B), and ‘Strongfield’ on 2B (QYr.spa-2B). All seem to represent QTL not reported previously. The QYr.spa-3A.2 was most consistently effective against Pst races across the Lethbridge and Toluca nurseries. With a LOD value of 4.9, QYr.spa-3A.2 explained a maximum phenotypic variation of 22.7% observed at the Toluca 2019 nursery. The QYr.spa-2B from ‘Strongfield’ and QYr.spa-3A.1 from ‘Blackbird’ expressed in multiple years at Toluca but were not detected at Lethbridge. QYr.spa-5B was identified in the Lethbridge 2016 environment. The identified QTL should be valuable in diversifying resistance genes used in breeding durum wheat cultivars with stripe rust resistance. ‘Blackbird’ was particularly useful for introducing the new QTL QYr.spa-3A.2 resistance that is effective in Canada and Mexico into traditional durum wheat germplasm. SNP markers associated with QTL will have application in marker-assisted breeding of resistance to Pst in durum wheat.
Résumé
La sélection pour la résistance est une stratégie efficace contre la rouille (jaune) causée par Puccinia striiformis f. sp. tritici (Pst). Pour identifier et cartographier les locus à caractère quantitatif (QTL) associés à la résistance à la rouille, une population dihaploïde de blé dur (n = 87) dérivée de ‘Strongfield/Blackbird’ a été évaluée pour la gravité de la maladie près de Toluca, au Mexique (2017 à 2019), et de Lethbridge, au Canada (2016 à 2019). La population a été génotypée avec la puce Illumina iSelect 90K de polymorphisme à nucléotide simple (SNP) et des marqueurs à séquences répétitives simples (SSR), puis on a procédé à l’analyse QTL avec MapQTL 6. Nous avons identifié le QTL de résistance à la rouille fourni par ‘Blackbird’ sur les chromosomes 3A (2 locus, désignés QYr.spa-3A.1, QYr.spa-3A.2) et 5B (QYr.spa-5B), et par ‘Strongfield’ sur 2B (QYr.spa-2B). Tous semblent représenter des QTL jamais rapportés auparavant. Le locus QYr.spa-3A.2 était le plus constamment efficace contre les races de Pst dans les pépinières de Lethbridge et de Toluca. Avec un LOD score de 4,9, QYr.spa-3A.2 expliquait un maximum de 22,7% de variations génotypiques à la pépinière de Toluca en 2019. Le locus QYr.spa-2B de ‘Strongfield’ et le QYr.spa-3A.1 de ‘Blackbird’ se sont exprimés depuis plusieurs années à Toluca, mais n’ont pas été détectés à Lethbridge. QYr.spa-5B a été identifié dans l’environnement de Lethbridge de 2016. Le QTL identifié devrait être utile pour diversifier les gènes de résistance utilisés pour sélectionner les cultivars de blé dur résistants à la rouille. ‘Blackbird’ a été particulièrement utile pour introduire la nouvelle résistance basée sur le QTL QYr.spa-3A.2 qui est efficace au Canada et au Mexique dans les germoplasmes de blés durs traditionnels. Les marqueurs SNP associés au QTL serviront à la sélection assistée par marqueurs pour la résistance à Pst chez le blé dur.
Introduction
Canada is a major durum (Triticum turgidum spp. durum (Desf) Husn.) wheat-producing country with about three times the combined production of the United States and Mexico (Sopiwnyk Citation2018). For the period 2016–2020 inclusive, Canada produced an average of 6.4 MMT (Statistics Canada Citation2021). Global durum production for the same period is about 40 million metric tons (International Grains Council [IGC] Citation2020). Stripe (yellow) rust, caused by Puccinia striiformis Westend. f. sp. tritici Eriks. (Pst) is a fungal pathogen that threatens production of durum wheat and bread wheat (Triticum aestivum L.) globally (Line Citation2002; Milus et al. Citation2009; Lyon and Broders Citation2017). Stripe rust was first reported in North America in 1915 (Carleton Citation1915; Line Citation2002). Until 2000, Pst was confined to the Pacific Northwest (PNW) regions in the USA, British Columbia, and southern Alberta (Aboukhaddour et al. Citation2020). Severe epidemics occurred in the 1960s followed by the most widespread epidemic in 2000, and thereafter (Chen et al. Citation2002; Line Citation2002; Chen Citation2007).
Pst is adapted to cool environmental conditions and may survive under mild winter conditions by reproducing asexually (Lin et al. Citation2018). However, epidemics that have occurred since 2000 in North America, Australia and Europe were mainly due to change in adaptation by the fungus allowing the incursions of more aggressive Pst lineages from cooler and wetter areas to the warmer and drier regions where it was previously infrequent or absent (Wellings Citation2007; Hovmøller et al. Citation2008, Citation2011; Milus et al. Citation2009; Chen et al. Citation2014; Beddow et al. Citation2015; Ali et al. Citation2017; Lyon and Broders Citation2017). The newer, more aggressive Pst pathogen genotypes can also infect previously resistant wheat cultivars, leading to rapid pathogen migration across and between continents (Hovmøller et al. Citation2008; Schwessinger Citation2017). For example, the two dominant Pst strains PstS1 and PstS2 have become adapted to high temperatures (Hovmøller et al. Citation2008; Brar et al. Citation2018; De Vallavieille-pope et al. Citation2018). PstS1 was predominant in North America, PstS2 in West Asia and North Africa, and both PstS1 and PstS2 in East Africa (Ali et al. Citation2017; Brar et al. Citation2018). PstS1 and PstS1-related races are dominant in western Canada (Brar et al. Citation2019). Similarly, the geographic range of Pst has expanded in the United States, and the old population of races was replaced by a new population (Milus et al. Citation2006; Markell and Milus Citation2008). The new races are better adapted and, thus, more aggressive at warmer temperatures than the old isolates (Milus et al. Citation2006; Brar et al. Citation2019) posing severe threats to current wheat production.
In Canada, stripe rust was mainly a problem in the western provinces of British Columbia and southern Alberta before 2000, but it later expanded to Saskatchewan, and the eastern provinces of Manitoba and Ontario (Chen Citation2005; Fetch et al. Citation2011; Brar et al. Citation2019). Up to about 2000, stripe rust infections were occasionally observed after mid-August in spring planted winter wheat cultivars ‘Norstar’ (Grant Citation1980) and ‘CDC Kestrel’ (Fowler Citation1997) in irrigated disease nurseries near Swift Current, SK. About the year 2002, stripe rust symptoms were observed in juvenile wheat plants on both irrigated and dryland by mid-July. In 2005, stripe rust symptoms observed in late June on dryland wheat in the semiarid region was really startling. The source of these infections in Canada was unknown, but it is possible to be from the ‘Puccinia pathway’ along the Pacific coast starting from north-western Mexico or the great central plains pathway starting from north central Mexico or from overwintering on winter wheat or foxtail barley (Hordeum jubatum L.) (Brar et al. Citation2019).
Eighty-three Yr genes have been formally documented to date (McIntosh et al. Citation2018a; Li et al. Citation2020; McIntosh et al. Citation2020). The majority of the genes belong to all-stage (seedling) resistance (ASR) and a few to adult-plant resistance (APR) (Chen Citation2007; Schwessinger Citation2017; Jamil et al. Citation2020). ASR genes are characterized by a strong to moderate immune response that fully curtails fungal infection and sporulation at all developmental stages (Schwessinger Citation2017). Unlike ASR, APR genes express at the adult plant stage and provide more durable resistance (Chen et al. Citation2014; Rinaldo et al. Citation2017; Schwessinger Citation2017). APR Yr genes are generally effective against multiple races of Pst. Yr genes such as Yr7 (Zhang et al. Citation2009), Yr15 (Klymiuk et al. Citation2018), Yr24/Yr26 (McIntosh et al. Citation2018b), Yr30/Sr2 (Randhawa et al. Citation2018) and Yr36 (Uauy et al. Citation2005) originate from tetraploid wheat. Yr36 is known to confer high-temperature adult-plant (HTAP) resistance (Uauy et al. Citation2005). Furthermore, QTL conferring stripe rust resistance in durum wheat have been reported (Singh et al. Citation2013; Lin et al. Citation2018).
Control of Pst can be achieved with fungicides and/or genetic resistance. Genetic resistance is the most economically and environmentally sustainable strategy for controlling wheat stripe rust. The stripe rust pathogen has a rather high mutation rate from avirulence to virulence (Hovmøller and Justesen Citation2007), and variation can arise through asexual and sexual recombination (Jin et al. Citation2010; Ali et al. Citation2014); therefore, there is a need for prophylactic measures.
The ‘Strongfield’ was the most widely grown durum cultivar from 2007 to 2015 due to its combination of high grain yield, protein concentration, low cadmium uptake, and improved semolina and pasta quality. Consequently, ‘Strongfield’ became a major parent of modern cultivars of Canada Western Amber Durum (CWAD) market-class. However, Canadian durum wheat production was threatened when reports that ‘Strongfield’ and the majority of Canadian durum germplasm were susceptible to stripe rust in the seedling stage (Lin et al. Citation2018). The tetraploid T. carthlicum Nevski wheat accession, ‘Blackbird’, had been observed to express resistance to stripe rust in spontaneous epidemic conditions in field nurseries near Swift Current, Saskatchewan. Determining if the ‘Blackbird’ resistance was different from that of ‘Strongfield’ would potentially reveal additional resistance that could be characterized and exploited in breeding. The present study sought to determine how similar genetically the resistance was between the durum wheat genotypes, ‘Strongfield’ and ‘Blackbird’. If different, the intention was to determine the genomic regions controlling stripe rust response and to identify molecular markers associated with the resistance genes.
Materials and methods
Plant materials
A doubled haploid (DH) population comprising 87 lines was developed from the cross ‘Strongfield’/‘Blackbird’ following the maize pollen method described by Humphreys and Knox (Citation2015). ‘Strongfield’ has high yield, high grain protein concentration, low grain cadmium concentration, and is adapted to the durum production area of the southern Canadian prairies (Clarke et al. Citation2005). ‘Blackbird’ is a T. carthlicum (Nevski) accession with genome AABB, black awns, and glumes (Somers et al. Citation2006).
Stripe rust disease assessment
The parents and DH lines were evaluated in response to stripe rust under field conditions at nurseries near Toluca, Mexico, from 2017 to 2019, and Lethbridge, Ab, Canada from 2016 to 2019. Both at Toluca and Lethbridge, parents and DH lines were grown in unreplicated single row plots. Being a biparental population, each allele at each locus under study is replicated in about half of the 87 lines. Therefore, the precision gained from additional experimental replication was considered not worth the cost. In Lethbridge, nurseries were artificially inoculated using Pst spores collected during the previous growing season. At Toluca, nurseries were planted as single rows of 1.5 m. Susceptible spreader plants included in the nurseries were inoculated with a Pst isolate MX16.04, which is virulent on lines carrying Yr24/Yr26. Isolate MEX16.04 belongs to the aggressive race group first identified in North America in 2000 and which became predominant in the subsequent year (Huerta-Espino and Singh Citation2017). The rationale behind using two different approaches for disease pressure on DH population that involved a natural mix of races at Lethbridge and a specific race at Toluca was to take advantage of the collaborating institution systems in place for evaluating germplasm. Disease severity was recorded as per cent of leaf area infected by stripe rust using the modified Cobb Scale (Peterson et al. Citation1948) except in 2018 and 2019 at Lethbridge when a 0 to 9 disease scale was used. The Lethbridge 2018 and 2019 data were converted to percentage for QTL analysis. In order to convert the 0 to 9 scale into a percentage, we divided each datapoint by 9 and then multiplied it by 100 as [(0–9 scale datapoint)/9]/100. Readings were done thrice every 7 days at Toluca and once at Lethbridge. Pearson correlation coefficients between locations and seasons were calculated to determine the consistency of disease severity across the environments.
Genotyping, linkage mapping and QTL analysis
The genotyping and linkage mapping of the ‘Strongfield’/‘Blackbird’ population were described by Pei (Citation2019). Briefly, the DH lines and their parents-were genotyped with a combination of simple sequence repeat (SSR) and single nucleotide polymorphism (SNP) markers. The SNP markers were genotyped in the population using the Illumina 90 K iSelect Infinium array and scored in GenomeStudio software (Illumina Inc., San Diego, CA). After discarding severely distorted loci, a total of 12 014 markers composed of 11 681 SNPs and 333 SSRs were mapped by linkage analysis resulting in 16 linkage groups corresponding to the 14 chromosomes of tetraploid wheat. The linkage map was 2782 cM in length with 1068 linkage bins. The average marker density was 4.3 loci/cM or 0.23 cM/locus.
QTL associated with stripe rust resistance were detected by carrying out QTL analysis to stripe rust reaction and genotypic data within MapQTL.6 ® (Van Ooijen Citation2009). A permutation test option of 1000 within MapQTL was used to determine the significance threshold for the logarithm of odds (LOD). Chromosome-wide threshold levels were used to declare significant QTL at the 5% level of significance. Automatic co-factor detection based on backward elimination to identify the cofactor markers as well-manual cofactor selection was performed for Multiple QTL Mapping (MQM) in MapQTL. MapChart v. 2.2 (Voorrips Citation2002) was used as a tool for visual presentation of the chromosomal locations of QTL. The detected QTL were assigned chromosomal arms based on the position of QTL associated SNP markers on the hexaploid wheat high-density consensus map by Wang et al. (Citation2014). To investigate the combined QTL effects on disease severity, an analysis of variance was carried out by SAS (SAS institute, Cary, NC). The analysis was conducted on disease severity data from environments at which the main effects of QTL were detected. For the analysis, lines of the ‘Strongfield’/‘Blackbird’ population were classified based on marker haplotypes at each locus.
Results
The T. carthlicum accession ‘Blackbird’ was relatively more resistant to stripe rust than the durum cultivar ‘Strongfield’ at Lethbridge except in 2016 (). In contrast, ‘Strongfield’ was relatively more resistant than ‘Blackbird’ at Toluca. The frequency distribution of disease severity of the DH lines was continuous with pronounced skewness towards susceptible reactions with some degree of variation in distribution among environments (). Significant correlations were observed between phenotypic data across environments with some exceptions (). Lethbridge 2019 did not correlate with any Toluca environments nor with Lethbridge 2017 and 2018 environments. The correlation between Lethbridge nurseries was generally weak or non-significant compared to among Toluca nurseries. Toluca 2018 and 2019 showed reasonably strong correlations with Lethbridge 2016 and 2018.
Table 1. Mean of parents and mean, standard deviation, and range of stripe rust severity for the ‘Strongfield’/‘Blackbird’ durum wheat population tested in different environments near Lethbridge, Canada and Toluca, Mexico
Table 2. Pearson correlation between stripe rust severity (%) of ‘Strongfield’/‘Blackbird’ durum wheat population tested in different environments near Lethbridge, Canada and Toluca, Mexico
Fig. 1 Frequency distribution of stripe rust severities of the ‘Strongfield’/‘Blackbird’ population evaluated in the field nurseries near Toluca, Mexico from 2017–2019 and Lethbridge, Canada from 2016–2019. Mean stripe rust severities of ‘Blackbird’ and ‘Strongfield’ are indicated with dark arrows
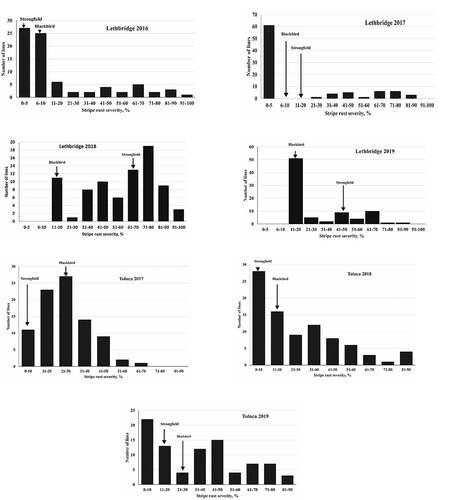
Four QTL associated with resistance to Pst were identified on chromosomes 2B, 3A (2 loci) and 5B designated as QYr.spa-2B, QYr.spa-3A.1, QYr.spa-3A.2 and QYr.spa-5B, respectively. depicts the QTL identified in the ‘Strongfield’/‘Blackbird’ population with associated markers, LOD value, parent allele mean value, additive value and phenotypic variation explained (PVE). shows a partial linkage map and position of the QTL identified. Derived from ‘Strongfield’, the QYr.spa-2B was detected in all 3 years at Toluca, but was not detected at Lethbridge. With peak SNP marker wsnp_Ra_c4321_7860456 and flanked by markers RAC875_c87052_193 and Ku_c55088_455, QYr.spa-2B spanned 9.4 cM in the genetic map of the ‘Strongfield’/‘Blackbird’ population (). SNP markers associated with the QTL were mapped on chromosome arm 2BS in a high-density consensus map by Wang et al. (Citation2014). Among the environments in which QYr.spa-2B expressed its highest peak LOD value of 3.5 and PVE of 17% was observed at Toluca in 2018.
Table 3. Associated chromosome, position on the chromosome, marker associated with the peak LOD value, LOD value, mean severity associated with each parental allele, percent phenotypic variation explained, additive effects, and favourable allele source for stripe rust resistance QTL identified in the ‘Strongfield’/’Blackbird’ population evaluated at Toluca, Mexico from 2017 to 2019 and Lethbridge, AB, Canada from 2016 to 2019
Contributed by ‘Blackbird’, QYr.spa-3A.1 was identified in all 3 years at Toluca (). This QTL was also not detected at Lethbridge. Based on the SNP markers associated with the QTL, QYr.spa-3A.1 is located in the centromeric region of chromosome 3A. QYr.spa-3A.1’s highest LOD was associated with marker JD_c1187_1398 in 2018 and 2019, and with marker BS00021981_51 in 2017 with the latter LOD from year 2017 of 3.1 being the highest of the three Toluca environments. The highest LOD corresponds to a maximum PVE of 13.8%. The QYr.spa-3A.1 stripe rust severity PVE was slightly lower than QYr.spa-2B, although both QTL had similar LOD values. Flanked by BS00021981_51 and JD_s1187_1398, QYr.spa-3A.1 spanned about 17.3 cM ().
As with QYr.spa-3A.1, the desirable allele for the second QTL on chromosome 3A, QYr.spa-3A.2 was derived from ‘Blackbird’. This QTL was the most stable QTL identified in the present study expressing in three out of 4 years at Lethbridge and two out of 3 years at Toluca. Located between BS00023337_51 and RAC875_c52805_182 on chromosome arm 3AL (Wang et al. Citation2014), QYr.spa-3A.2 spanned about 8.2 cM of the 3A genome on the genetic map of the ‘Strongfield’/‘Blackbird’ population. With a peak marker, Tdurum_contig51339_447, QYr.spa-3A.2 was associated with LOD values that ranged from 2.1 to 4.0 at Lethbridge, and 4.2 to 4.9 at Toluca. The maximum explained variation for stripe rust severity across environments was 16.1% (LOD, 3.3) at Lethbridge and 22.7% (LOD, 4.2) at Toluca.
A QTL on chromosome 5B, QYr.spa-5B, was detected at Lethbridge in a 2016 test environment. With the desirable allele contributed by ‘Blackbird’, this QTL was the only QTL specific to Canada unlike the two QTL QYr.spa-2B and QYr.spa-3A.1, the expression of which was confined to Mexico. QYr.spa-3A.2 expressed in both countries. The marker associated with the peak of QYr.spa-5B was BS00062762_51 which mapped on chromosome arm 5BL (Wang et al. Citation2014). Associated with a LOD of 2.9, QYr.spa-5B explained 15% PVE of the stripe rust severity in the Lethbridge 2016 environment.
The statistical analysis of gene combinations indicated that there were significant additive effects between the main effect QTL identified in this study (). A combination of QYr.spa-2B with QYr.spa-3A.1, QYr.spa-2B with QYr.spa-3A.2 and QYr.spa-3A.2 with QYr.spa-5B resulted in elevated disease resistance compared with individual QTL effects over environments.
Fig. 2 Linkage map of chromosomes 2B, 3A, and 5B showing positions of stripe rust resistance QTL identified in the ‘Strongfield’/’Blackbird’ population. ‘Strongfield’ contributed the QTL on chromosome 2B detected in Canada. ‘Blackbird’ contributed the QTL on chromosome 3A, 3A.1 (QYr.spa-3A.1) detected in Mexico and 3A.2 (QYr.spa-3A.2) detected in Canada and Mexico, and 5B detected in Mexico. Numbers on the left side of the graphs represent map position given in cM values
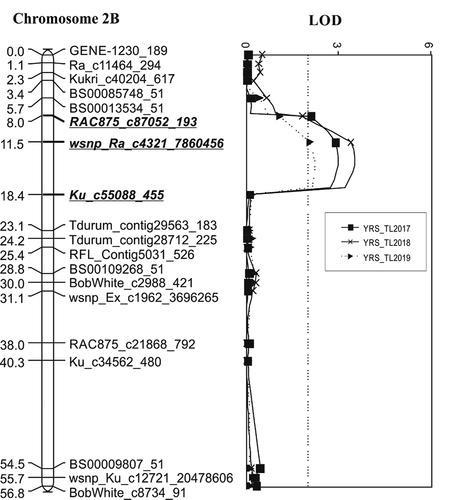
Fig. 3 The difference in the effect of QTL combination compared with individual QTL on stripe rust severity in the ‘Strongfield’/‘Blackbird’ population evaluated near Toluca, Mexico and Lethbridge, Canada. Additive effects of (a) 2B and 3A.1 at Toluca 2017–2019, (b) 2B and 3A.2 at Toluca 2017–2019, and 3A.2 and 5B at Lethbridge 2016 and 2017. QTL descriptions: 2B stands for QYr.spa-2B, 3A.1 for QYr.spa-3A.1, 3A.2 for QYr.spa-3A.2, and 5B for QYr.spa-5B. Bars with the same letter within each test environment are not significantly different according to the Duncan’s Multiple Range test at P = 0.05.
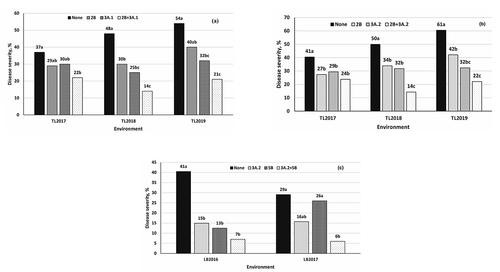
Discussion
The variation observed among the phenotypic distributions of the DH lines across environments indicates that environmental conditions produce a differential effect on the expression of resistance. Temperature is known to affect the expression of some Pst-resistance genes, such as the high-temperature adult plant gene Yr36 (Uauy et al. Citation2005), Yr8 (Chen and Zhao Citation2007), Yr17 (Milus et al. Citation2015), and Yr28 (Zheng et al. Citation2020). The shape of the distribution of DH lines to stripe rust in Toluca is rather similar each year, with most lines being resistant to moderately resistant. The more varied distributions might also be caused by differences in Pst race virulence and/or aggressiveness. This variation in phenotypic distributions of the lines across environments is in line with the low to moderate correlations observed among years at Lethbridge and Toluca and Lethbridge not being correlated in 3 of 4 years. The skewness of the frequency distribution of the DH lines for stripe rust severity towards resistance indicates the additive effect of the minor to moderate effect resistance identified in either parent of the population. The effectiveness of QYr.spa-2B and QYr.spa-3A.1 at Toluca, and their absence at Lethbridge indicates the presence of virulent Pst races unique to Canada. Furthermore, the detection of QYr.spa-5B at Lethbridge and its absence at Toluca indicates the ineffectiveness of this QTL against the MX16.04 Pst race. Considering the fact that natural infections were also present as the main sources of an inoculum in the field tests, the simultaneous detection of QYr.spa-3A.2 in Canada and Mexico suggests that this QTL is effective against Pst races prevalent in both countries.
‘Strongfield’ contributed QYr.spa-2B associated with markers located on chromosome arm 2BS, the expression of which was consistent across years at Toluca. The absence of this QTL in Canada might be due to the presence of virulent Pst races specific to Canada or an environment not favourable to expression. Three Yr genes Yr27, Yr31 and Yr41 have been reported to reside on chromosome 2BS (Luo et al. Citation2009; McIntosh et al. Citation2014; Maccaferri et al. Citation2015; Wu et al. Citation2017). QYr.spa-2B is unlikely to be controlled by Yr27 because a marker for the gene, Xgwm55 (McDonald et al. Citation2004), was located 96.6 cM from QYr.spa-2B marker RAC875_c87052_193. Moreover, Yr27 is susceptible to Pst populations common in Mexico (McDonald et al. Citation2004). The effectiveness of Yr31 has been lost due to a virulent race MX16.04 that was detected at Toluca in 2009 (Rosewarne et al. Citation2012; Yang et al. Citation2013) suggesting it is also different from QYr.spa-2BS. Yr41 is an all-stage resistance gene and associated with Xgwm410 (Jiang et al. Citation2020). Xgwm410 is located 107 cM from the QYr.spa-2B associated marker RAC875_c87052_193 on the consensus map by Wen et al. (Citation2017) suggesting the factor controlling QYr.spa-2B is distinct from Yr41. QYr.spa-2B from ‘Strongfield’ was not detected by Singh et al. (Citation2013) in the ‘Sachem’/‘Strongfield’ mapping population tested for stripe rust in Toluca, Mexico, in 2009 and 2011, and Njoro, Kenya, in 2010. In contrast, they reported a different QTL contributed by Sachem on chromosome 2B associated with DArT marker wPt-3632 at Toluca in 2011. The QYr.spa-2B would not be detected if both ‘Sachem’ and ‘Strongfield’ have the QTL.
In another study, Lan et al. (Citation2014) reported a seedling resistance gene QYr.cim-2BS (designated YrF) in wheat line ‘Francolin#1ʹ that conferred intermediate seedling reaction and resistance at the adult plant stage in Mexican and Chinese environments. However, a marker for YrF, Xwmc474, was 85.59 cM from RAC875_c87052_193 on the Bokore et al. (Citation2020) consensus map, suggesting distinct factors. In the Canadian spring wheat population ‘Carberry’/’AC Cadillac’, Singh et al. (Citation2014) reported two stripe rust-resistance QTL on chromosome 2B. The first, from ‘Carberry’, was associated with Xwmc25, which was located at 16.7 cM from a peak marker for QYr.spa-2B on the consensus map by Bokore et al. (Citation2020). The second, from ‘AC Cadillac’, was associated with Xwmc770 and was located at 22.6 cM from the closest marker for the QYr.spa-2B in ‘Strongfield’ on the map by Bokore et al. (Citation2020). Despite their proximity to the chromosome, the similarity of ‘AC Cadillac’ or ‘Carberry’ QTL with that of ‘Strongfield’ requires further investigation. Perez-Lara et al. (Citation2017) identified a QTL, QYrlo.wpg-2BS, associated with HTAP stripe rust resistance in an American spring wheat cultivar ‘Louise’ on chromosome 2BS within a region flanked by Xwmc474 and Xgwm148. QYrlo.wpg-2BS should be different from QYr.spa-2B as Xwmc474 is 85.59 cM from QYr.spa-2B (Bokore et al. Citation2020). The most closely associated QYr.spa-2B marker, wsnp_Ra_c4321_7860456, has been reported to be associated with resistance to tan spot caused by Pyrenophora tritici-repentis in Canadian spring wheat cultivars (Perez-Lara et al. Citation2017) which suggests the usefulness of this chromosomal region. Such close association of the two traits may be a benefit or a challenge depending on whether or not the leaf spot and stripe rust resistance are in coupling or repulsion. Overall, the QYr.spa-2B may represent a new QTL not reported before in durum wheat.
The Blackbird has two stripe rust resistance QTL on chromosome 3A located at a physical distance of about 191 Mbp on the IWGSC refseq. V. 2.0 based on the most closely associated markers JD_c1187_1398 for QYr.spa-3A.1 and Tdurum_contig51339_447 for QYr.spa-3A.2. No named Yr gene is found on the chromosome where the two Yr QTL, QYr.spa-3A.1 and QYr.spa-3A.2 from ‘Blackbird’ are found (Rosewarne et al. Citation2013; McIntosh et al. Citation2014; Maccaferri et al. Citation2015; McIntosh et al. Citation2018a). However, a few studies report QTL conferring resistance against Pst on the chromosome (Christopher et al. Citation2013; Singh et al. Citation2014; Ma et al. Citation2016). For example, Christopher et al. (Citation2013) report a QTL in a US American winter wheat-line Neuse. Singh et al. (Citation2014) similarly found a Yr QTL on chromosome 3A expressed in a single environment in Canada derived from the spring wheat cultivar ‘AC Cadillac’. Both Christopher et al. (Citation2013) and Singh et al. (Citation2014) used DArT markers in their studies making a comparison of their QTL with those in our study difficult. Lillemo et al. (Citation2008) report a major QTL for stripe rust resistance on chromosome 3A in the wheat line ‘Saar’, but it resides on the short arm of the chromosome as opposed to the markers for the two QTL in ‘Blackbird’ – the QYr.spa-3A.1 that is located in the centromeric region of chromosome 3A and the QYr.spa-3A.2 that is located on 3AL. To our understanding, there is no Yr gene previously reported on chromosome 3AL of durum wheat. Therefore, QYr.spa-3A.1 and QYr.spa-3A.2 are most likely novel.
The sporadic ‘Blackbird’ derived QTL, QYr.spa-5B, conditioned resistance against Pst races specific to Canada. This QTL generated a significant LOD value at Lethbridge in 2016 and a lower stripe rust severity in the ‘Blackbird’ haplotype at Lethbridge in 2017 () but not significant at the 5% level. Yr47 maps to the short arm of chromosome 5B, which disqualifies it as the source of QYr.spa-5B which associated with markers located on the long arm of 5B and there has been no Yr gene reported the 5BL thus far (Maccaferri et al. Citation2015; Qureshi et al. Citation2017; McIntosh et al. Citation2018a). Singh et al. (Citation2014) reported a stripe rust QTL on chromosome 5B associated with Xbarc59 in the spring wheat cultivar ‘AC Cadillac’. Xbarc59 is located 76.7 cM from the peak marker associated with QYr.spa-5B from ‘Blackbird’ on the hexaploid wheat SNP consensus map of Bokore et al. (Citation2020) suggesting the Singh et al. (Citation2014) QTL is different from QYr.spa-5B. Hou et al. (Citation2015) reported all an stage stripe rust HTAP-resistance QTL on 5BL associated with wsnp_JD_rep_c50403_34392266 in a soft white winter wheat cultivar ‘Druchamp’ (PI 174622) introduced to the US Pacific Northwest for production in 1949 from France. This QTL may be controlled by the same factor as QYr.spa-5B mapping within 49 cM of QYr.spa-5B on the consensus map by Bokore et al. (Citation2020). Using the same consensus map, a peak marker BS00062762_51 for the QYr.spa-5B in ‘Blackbird’ was located 43.21 cM from wsnp_Ku_c4427_8029592, a marker associated with QYr.usw-5B in durum wheat-line W9262-260D3 (Lin et al. Citation2018). Another QTL was reported by Yang et al. (Citation2013) in hexaploid wheat that was effective in China, but not effective in Mexico, where QYr.spa-5B was similarly ineffective. The relatedness of these genes with the QYr.spa-5B requires further resolution.
In addition to the present study, the ‘Strongfield’/‘Blackbird’ population has previously been used in different trait mapping studies including leaf rust resistance and Fusarium head blight resistance (Sari et al. Citation2018). ‘Blackbird’ is susceptible to leaf rust, while ‘Strongfield’ is resistant (Pei Citation2019). None of the leaf rust QTL identified by Pei (Citation2019) using this population corresponded with or located near the stripe resistance QTL we discovered in the present study. Sari et al. (Citation2018) determined several FHB resistance QTL segregating in the ‘Strongfield’/‘Blackbird’ population of which a QTL on chromosome 3A of ‘Blackbird’ was associated with the same SNP marker, BS00021981_51, as the ‘Blackbird’ derived QYr.spa-3A.1. Knowledge of such gene relationships is helpful in stacking of genes governing different traits in new varieties.
The identification of the QYr.spa-2B in ‘Strongfield’ is an addition to the stripe rust QTL Singh et al. (Citation2013) detected on chromosome 7B of this cultivar in the ’Sachem’/’Strongfield’ population in Toluca, Mexico, in 2011. The absence of segregation among DH lines of the ‘Blackbird’/‘Strongfield’ population could be due to the QTL being common in both parents but could also be the result of a race change in Mexico.
It is challenging finding consistent and durable resistance genes with the constant evolution of new rust strains and their adaptation to higher temperatures (Bryant et al. Citation2014). For example, despite their effectiveness in Toluca, where one of the aggressive Pst races MEX16.04 was present, the two ‘Blackbird’ derived QTL, QYr.spa-2B and QYr.spa-3A.1, were not effective at Lethbridge, Canada.
The major route for the migration of Pst into southern Alberta (Lethbridge) is the Pacific Northwest of United States (Oregon, Washington, and northern Idaho) (Line Citation2002; Xi et al. Citation2015; Aboukhaddour et al. Citation2020). Alternatively, movement to Canada occurs through the Central Great Plains ‘Puccinia pathway’ from inoculum that primarily overwinters in southern Texas, northern Mexico and along the Gulf Coast in Louisiana and Mississippi. Deployments of QTL, such as QYr.spa-3A.2, that are effective against different Pst populations in multiple regions, and countries should be helpful in controlling the disease, regardless of the source of the pathogen.
In summary, we mapped a stripe rust resistance QTL contributed by the durum wheat cultivar ‘Strongfield’ on chromosome arm 2B (QYr.spa-2B) and three QTL contributed by the T. carthlicum accession ‘Blackbird’ on chromosomes 3A (2 loci, QYr.spa-3A.1, QYr.spa-3A.2) and 5B (QYr.spa-5B). As this study was based on adult plant response, seedling test studies are needed to investigate the nature of these QTL. The QYr.spa-3A.2 QTL was effective in Canada and Mexico, QYr.spa-2B and QYr.spa-3A.1 in Mexico, and QYr.spa-5B was effective in Canada indicating the difference in the Pst populations between these two countries, or the effect of environment on expression. To our knowledge, none of these QTL corresponds with the previously reported Yr genes or QTL in durum wheat suggesting they are new genetic factors. The identification of the QYr.spa-3A.2 in ‘Blackbird’ is of particular interest because it represents a stable QTL that is effective in two countries over multiple years. The SNP markers associated with the QTL identified will be useful in marker assisted breeding of resistance to Pst in durum wheat.
Acknowledgements
We thank Lethbridge Research and Development Centre, AAFC and the International Maize and Wheat Improvement Center (CIMMYT), Mexico for providing field rust nurseries and technical support.
Additional information
Funding
References
- Aboukhaddour R, Fetch T, McCallum BD, Harding MW, Beres BL, Graf RJ. 2020. Wheat diseases on the prairies: a Canadian story. Plant Pathol. 69(3):418–255. doi:https://doi.org/10.1111/ppa.13147.
- Ali S, Gladieux P, Leconte M, Gautier A, Justesen AF, Hovmøller MS, Enjalbert J, De Vallavieille-pope C, McDonald BA. 2014. Origin, migration routes and worldwide population genetic structure of the wheat yellow rust pathogen Puccinia striiformis f.sp. tritici. PLoS Pathog. 10(1):e1003903. doi:https://doi.org/10.1371/journal.ppat.1003903.
- Ali S, Rodriguez-Algaba J, Thach T, Sørensen CK, Hansen JG, Lassen P, Nazari K, Hodson DP, Justesen AF, Hovmøller MS. 2017. Yellow rust epidemics worldwide were caused by pathogen races from divergent genetic lineages. Front Plant Sci. 8:1057. doi:https://doi.org/10.3389/fpls.2017.01057.
- Beddow JM, Pardey PG, Chai Y, Hurley TM, Kriticos DJ, Braun HJ, Park RF, Cuddy WS, Yonow T. 2015. Research investment implications of shifts in the global geography of wheat stripe rust. Nat Plants. 1(10):15132. doi:https://doi.org/10.1038/nplants.2015.132.
- Bokore FE, Knox RE, Cuthbert RD, Pozniak CJ, McCallum BD, N’Diaye A, DePauw RM, Campbell HL, Munro C, Singh A, et al. 2020. Mapping quantitative trait loci associated with leaf rust resistance in five spring wheat populations using single nucleotide polymorphism markers. PLoS ONE. 15(4):e0230855. doi:https://doi.org/10.1371/journal.pone.0230855
- Brar GS, Ali S, Qutob D, Ambrose S, Lou K, Maclachlan R, Pozniak CJ, Fu YB, Sharpe AG, Kutcher HR. 2018. Genome re-sequencing and simple sequence repeat markers reveal the existence of divergent lineages in the Canadian Puccinia striiformis f. sp. tritici population with extensive DNA methylation. Environ Microbiol. 20(4):1498–1515. doi:https://doi.org/10.1111/1462-2920.14067.
- Brar GS, Fetch T, McCallum BD, Hucl PJ, Kutcher HR. 2019. Virulence dynamics and breeding for resistance to stripe, stem, and leaf rust in Canada since 2000. Plant Dis. 103(12):2981–2995. doi:https://doi.org/10.1094/PDIS-04-19-0866-FE.
- Bryant RRM, GRD M, Mitchell AR, H-j S, La B, Uauy C, Dorling S, CJ R. 2014. A change in temperature modulates defence to yellow (stripe) rust in wheat line UC1041 independently of resistance gene Yr36. BMC Plant Biol. 14(1):10. doi:https://doi.org/10.1186/1471-2229-14-10.
- Carleton MA. 1915. A serious new wheat rust in this country. Science. 42(1071):58–59. doi:https://doi.org/10.1126/science.42.1071.58-a.
- Chen W, Wellings C, Chen X, Kang Z, Liu T. 2014. Wheat stripe (yellow) rust caused by Puccinia striiformis f. sp. tritici. Mol Plant Path. 15(5):433–446. doi:https://doi.org/10.1111/mpp.12116.
- Chen X, Moore M, Milus EA, Long DL, Line RF, Marshall D, Jackson L. 2002. Wheat stripe rust epidemics and races of Puccinia striiformis f. sp. tritici in the United States in 2000. Plant Dis. 86(1):39–46. doi:https://doi.org/10.1094/PDIS.2002.86.1.39.
- Chen X, Zhao J. 2007. Identification of molecular markers for Yr8 and a gene for high-temperature, adult-plant resistance against stripe rust in the AVS/6*Yr8 wheat line. American phytopathological society abstracts. San Diego (CA): S21. 7/28-8/2/07, 97.
- Chen XM. 2005. Epidemiology and control of stripe rust (Puccinia striiformis f. sp. tritici) on wheat. Can J Plant Path. 27(3):314–337. doi:https://doi.org/10.1080/07060660509507230.
- Chen XM. 2007. Challenges and solutions for stripe rust control in the United States. Aus J Agri Res. 58(6):648–655. doi:https://doi.org/10.1071/AR07045.
- Christopher MD, Liu S, Hall MD, Marshall DS, Fountain MO, Johnson JW, Milus EA, Garland-Campbell KA, Chen X, Griffey CA. 2013. Identification and mapping of adult-plant stripe rust resistance in soft red winter wheat cultivar ‘USG 3555ʹ. Plant Breed. 132(1):53–60. doi:https://doi.org/10.1111/pbr.12015.
- Clarke JM, McCaig TN, DePauw RM, Knox RE, Clarke FR, Fernandez MR, Ames NP. 2005. Strongfield durum wheat. Can J Plant Sci. 85(3):651–654. doi:https://doi.org/10.4141/P04-119.
- De Vallavieille-pope C, Bahri B, Leconte M, Zurfluh O, Belaid Y, Maghrebi E, Huard F, Huber L, Launay M, Bancal MO. 2018. Thermal generalist behaviour of invasive Puccinia striiformis f. sp. tritici strains under current and future climate conditions. Plant Pathol. 67(6):1307–1320. doi:https://doi.org/10.1111/ppa.12840.
- Fetch T, McCallum B, Menzies J, Rashid K, Tenuta A. 2011. Rust diseases in Canada. Prairie Soils and Crops Journal. 4:86–96.
- Fowler DB. 1997. CDC Kestrel winter wheat. Can J Plant Sci. 77(4):673–675. doi:https://doi.org/10.4141/P96-193.
- Grant MN. 1980. Registration of norstar wheat 1 (Reg. no. 626). Crop Sci. 20(4):552. doi:https://doi.org/10.2135/cropsci1980.0011183X002000040042x.
- Hou L, Chen X, Wang M, See DR, Chao S, Bulli P, Jing J. 2015. Mapping a large number of QTL for durable resistance to stripe rust in winter wheat Druchamp using SSR and SNP markers. PLoS ONE. 10(5):e0126794. doi:https://doi.org/10.1371/journal.pone.0126794.
- Hovmøller MS, Justesen AF. 2007. Rates of evolution of avirulence phenotypes and DNA markers in a northwest European population of Puccinia striiformis f. sp. tritici. Mol Ecol. 16(21):4637–4647. doi:https://doi.org/10.1111/j.1365-294X.2007.03513.x.
- Hovmøller MS, Sørensen CK, Walter S, Justesen AF. 2011. Diversity of Puccinia striiformis on cereals and grasses. Annu Rev Phytopathol. 49:197–217. doi:https://doi.org/10.1146/annurev-phyto-072910-095230.
- Hovmøller MS, Yahyaoui AH, Milus EA, Justesen AF. 2008. Rapid global spread of two aggressive strains of a wheat rust fungus. Mol Ecol. 17(17):3818–3826. doi:https://doi.org/10.1111/j.1365-294X.2008.03886.x.
- Huerta-Espino J, Singh RP. 2017. First vetection of virulence in Puccinia striiformis f. sp. tritici to wheat resistance genes Yr10 and Yr24 (=Yr26) in Mexico. Plant Dis. 101(9):1676. doi:https://doi.org/10.1094/PDIS-04-17-0532-PDN.
- Humphreys DG, Knox RE. 2015. Doubled haploid breeding in cereals. In: Al-Khayri J, Jain S, Johnson D, editors. Advances in plant breeding strategies: breeding, biotechnology and molecular tools. Cham (Switzerland): Springer; p. 241–290.
- International Grains Council [IGC]. (2020). World grain statistics 2016. [accessed 2021 March 16]. https://www.igc.int/en/subscriptions/subscription.aspx.
- Jamil S, Shahzad R, Ahmad S, Fatima R, Zahid R, Anwar M, Iqbal MZ, Wang X. 2020. Role of genetics, genomics, and breeding approaches to combat stripe rust of wheat. Front Nutr. 7:580715. doi:https://doi.org/10.3389/fnut.2020.580715.
- Jiang C, Wang X, Chen W, Liu T, Zhong S, Huang Q, Ren T, Li Z, Tan F, Luo P. 2020. Resistance performance of wheat stripe rust resistance gene Yr41 and its effect on yield parameters in F2 populations under field conditions. Crop Prot. 134:105168. doi:https://doi.org/10.1016/j.cropro.2020.105168.
- Jin Y, Szabo LJ, Carson M. 2010. Century-old mystery of Puccinia striiformis life history solved with the identification of berberis as an alternate host. Phytopathology. 100(5):432–435. doi:https://doi.org/10.1094/PHYTO-100-5-0432.
- Klymiuk V, Yaniv E, Huang L, Raats D, Fatiukha A, Chen S, Feng L, Frenkel Z, Krugman T, Lidzbarsky G, et al. 2018. Cloning of the wheat Yr15 resistance gene sheds light on the plant tandem kinase-pseudokinase family. Nature Comm. 9(1):3735. doi:https://doi.org/10.1038/s41467-018-06138-9
- Lan C, Rosewarne GM, Singh RP, Herrera-Foessel SA, Huerta-Espino J, Basnet BR, Zhang Y, Yang E. 2014. QTL characterization of resistance to leaf rust and stripe rust in the spring wheat line francolin#1. Mol Breed. 34(3):789–803. doi:https://doi.org/10.1007/s11032-014-0075-6.
- Li H, Bariana H, Singh D, Zhang L, Dillon S, Whan A, Bansal U, Ayliffe M. 2020. Cloning of the wheat Yr15 resistance gene sheds light on the plant tandem kinase-pseudokinase family. Theor Appl Genet. 133(11):3049–3066. doi:https://doi.org/10.1007/s00122-020-03654-5.
- Lillemo M, Asalf B, Singh RP, Huerta-Espino J, Chen XM, He ZH, Bjørnstad A. 2008. The adult plant rust resistance loci Lr34/Yr18 and Lr46/Yr29 are important determinants of partial resistance to powdery mildew in bread wheat line saar. Theor Appl Genet. 116(8):1155–1166. doi:https://doi.org/10.1007/s00122-008-0743-1.
- Lin X, N’Diaye A, Walkowiak S, Nilsen KT, Cory AT, Haile J, Kutcher HR, Ammar K, Loladze A, Huerta-Espino J, et al. 2018. Genetic analysis of resistance to stripe rust in durum wheat (Triticum turgidum L. Var. durum). PLoS ONE. 13(9):e0203283. doi:https://doi.org/10.1371/journal.pone.0203283
- Line RF. 2002. Stripe rust of wheat and barley in North America: a retrospective historical review. Annu Rev Phytopathol. 40(1):75–118. doi:https://doi.org/10.1146/annurev.phyto.40.020102.111645.
- Luo P, Hu X, Zhang H, Ren Z. 2009. Genes for resistance to stripe rust on chromosome 2B and their application in wheat breeding. Progr Nat Sci. 19(1):9–15. doi:https://doi.org/10.1016/j.pnsc.2008.02.017.
- Lyon B, Broders K. 2017. Impact of climate change and race evolution on the epidemiology and ecology of stripe rust in central and eastern USA and Canada. Can J Plant Path. 39(4):385–392. doi:https://doi.org/10.1080/07060661.2017.1368713.
- Ma DF, Fang ZW, Yin JL, Chao KX, Jing JX, Li Q, Wang BT. 2016. Molecular mapping of stripe rust resistance gene YrHu derived from psathyrostachys huashanica. Mol Breed. 36(6):64. doi:https://doi.org/10.1007/s11032-016-0487-6.
- Maccaferri M, Zhang J, Bulli P, Abate Z, Chao S, Cantu D, Bossolini E, Chen X, Pumphrey M, Dubcovsky J. 2015. A genome-wide association study of resistance to stripe rust (Puccinia striiformis f. sp. tritici) in a worldwide collection of hexaploid spring wheat (Triticum aestivum L.). G3. 5(3):449–465. doi:https://doi.org/10.1534/g3.114.014563.
- Markell SG, Milus EA. 2008. Emergence of a novel population of Puccinia striiformis f. sp. tritici in Eastern United States. Phytopathology. 98(6):632–639. doi:https://doi.org/10.1094/PHYTO-98-6-0632.
- McDonald DB, McIntosh RA, Wellings CR, Singh RP, Nelson JC. 2004. Cytogenetical studies in wheat XIX. Location and linkage studies on gene Yr27 for resistance to stripe (yellow) rust. Euphytica. 136(3):239–248. doi:https://doi.org/10.1023/B:EUPH.0000032709.59324.45.
- McIntosh RA, Dubcovsky J, Rogers WJ, Morris CF, Appels R, Xia CX. 2014. Catalogue of gene symbols for wheat: 2013–14 supplement. [accessed 2021 March 16]. http://wwwshigennigacjp/wheat/komugi/genes/symbolClassListjsp.
- McIntosh RA, Dubcovsky J, Rogers WJ, Xia CX, Raupp WJ. 2020. Catalogue of gene symbols for wheat: 2020 supplement. [accessed 2021 March 16]. https://wheatpwusdagov/GG3/wgc.
- McIntosh RA, Dubcovsky J, Rogers WJ, Xia XC, Raupp WJ. 2018a. Catalogue of gene symbols for wheat: 2018 supplement. Annu Wheat News Letter. 64:73–93.
- McIntosh RA, Mu J, Han D, Kang Z. 2018b. Wheat stripe rust resistance gene Yr24/Yr26: a retrospective review. The Crop J. 6(4):321–329. doi:https://doi.org/10.1016/j.cj.2018.02.001.
- Milus EA, Kristensen K, Hovmøller MS. 2009. Evidence for increased aggressiveness in a recent widespread strain of Puccinia striiformis f. sp. tritici causing stripe rust of wheat. Phytopathology. 99(1):89–94. doi:https://doi.org/10.1094/PHYTO-99-1-0089.
- Milus EA, Lee KD, Brown-Guedira G. 2015. Characterization of stripe rust resistance in wheat lines with resistance gene Yr17 and implications for evaluating resistance and virulence. Phytopathology. 105(8):1123–1130. doi:https://doi.org/10.1094/PHYTO-11-14-0304-R.
- Milus EA, Seyran E, McNew R. 2006. Aggressiveness of Puccinia striiformis f. sp. tritici isolates in the south-central United States. Plant Dis. 90(7):847–852. doi:https://doi.org/10.1094/PD-90-0847.
- Pei X. 2019. Genetic mapping of leaf rust (Puccinia triticina Eriks.) resistance in durum wheat (Triticum turgidum var. durum L.) [MSc thesis]. Manitoba (MN): University of Manitoba.
- Perez-Lara E, Semagn K, Tran VA, Ciechanowska I, Chen H, Iqbal M, N’Diaye A, Pozniak C, Strelkov SE, Hucl PJ, et al. 2017. Population structure and genomewide association analysis of resistance to disease and insensitivity to Ptr toxins in Canadian spring wheat using 90K SNP array. Crop Sci. 57(3):1522–1539. doi:https://doi.org/10.2135/cropsci2016.10.0859
- Peterson RF, Campbell AB, Hannah AE. 1948. A diagrammatic scale for estimating rust intensity on leaves and stems of cereals. Can J Res. 26(5):496–500. doi:https://doi.org/10.1139/cjr48c-033.
- Qureshi N, Bariana H, Forrest K, Hayden M, Keller B, Wicker T, Faris J, Salina E, Bansal U. 2017. Fine mapping of the chromosome 5B region carrying closely linked rust resistance genes Yr47 and Lr52 in wheat. Theor Appl Genet. 130(3):495–504. doi:https://doi.org/10.1007/s00122-016-2829-5.
- Randhawa MS, Lan CX, Basnet BR, Bhavani S, Huerta-Espino J, Forrest K, Hayden M, Singh RP. 2018. Interactions among genes Sr2/Yr30, Lr34/Yr18/Sr57 and Lr68 confer enhanced adult plant resistance to rust diseases in common wheat (Triticum aestivum L.) line Arula. Aust J Crop Sci. 12(6):1023–1033. doi:https://doi.org/10.21475/ajcs.18.12.06.PNE1305.
- Rinaldo A, Gilbert B, Boni R, Krattinger SG, Singh D, Park RF, Lagudah E, Ayliffe M. 2017. The Lr34 adult plant rust resistance gene provides seedling resistance in durum wheat without senescence. Plant Biotechnol J. 15(7):894–905. doi:https://doi.org/10.1111/pbi.12684.
- Rosewarne GM, Herrera-Foessel SA, Singh RP, Huerta-Espino J, Lan CX, He ZH. 2013. Quantitative trait loci of stripe rust resistance in wheat. Theor Appl Genet. 126(10):2427–2449. doi:https://doi.org/10.1007/s00122-013-2159-9.
- Rosewarne GM, Singh RP, Huerta-Espino J, Herrera-Foessel SA, Forrest KL, Hayden MJ, Rebetzke GJ. 2012. Analysis of leaf and stripe rust severities reveals pathotype changes and multiple minor QTLs associated with resistance in an Avocet × Pastor wheat population. Theor Appl Genet. 124(7):1283–1294. doi:https://doi.org/10.1007/s00122-012-1786-x.
- Sari E, Berraies S, Knox RE, Singh AK, Ruan Y, Cuthbert RD, Pozniak CJ, Henriquez MA, Kumar S, Burt AJ, et al. 2018. High density genetic mapping of fusarium head blight resistance QTL in tetraploid wheat. PLoS ONE. 13(10):e0204362. doi:https://doi.org/10.1371/journal.pone.0204362
- Schwessinger B. 2017. Fundamental wheat stripe rust research in the 21(st) century. New Phytol. 213(4):1625–1631. doi:https://doi.org/10.1111/nph.14159.
- Singh A, Knox RE, DePauw RM, Singh AK, Cuthbert RD, Campbell HL, Shorter S, Bhavani S. 2014. Stripe rust and leaf rust resistance QTL mapping, epistatic interactions, and co-localization with stem rust resistance loci in spring wheat evaluated over three continents. Theor Appl Genet. 127(11):2465–2477. doi:https://doi.org/10.1007/s00122-014-2390-z.
- Singh A, Pandey MP, Singh AK, Knox RE, Ammar K, Clarke JM, Clarke FR, Singh RP, Pozniak CJ, DePauw RM, et al. 2013. Identification and mapping of leaf, stem and stripe rust resistance quantitative trait loci and their interactions in durum wheat. Mol Breed. 31(2):405–418. doi:https://doi.org/10.1007/s11032-012-9798-4
- Somers DJ, Fedak G, Clarke J, Cao W. 2006. Mapping of FHB resistance QTLs in tetraploid wheat. Genome. 49(12):1586–1593. doi:https://doi.org/10.1139/g06-127.
- Sopiwnyk E. 2018. Durum production and consumption, a global perspective: in sustainable production of durum wheat in Canada. In: DePauw RM, editor. Durum wheat production manual. Northbrook (IL): Barilla America Ltd; 5–9. Available from: https://saskwheatca/durum-production-manual
- Statistics Canada. 2021. Table 32- 10-0359-01. Estimated areas, yield, production, average farm price and total farm value of principal field crops, in metric and imperial units. [accessed 2021 March 16]. https://doi.org/https://doi.org/10.25318/3210035901-eng.
- Uauy C, Brevis JC, Chen X, Khan I, Jackson L, Chicaiza O, Distelfeld A, Fahima T, Dubcovsky J. 2005. High-temperature adult-plant (HTAP) stripe rust resistance gene Yr36 from triticum turgidum ssp. dicoccoides is closely linked to the grain protein content locus Gpc-B1. Theor Appl Genet. 112(1):97–105. doi:https://doi.org/10.1007/s00122-005-0109-x.
- Van Ooijen JW. 2009. MapQTL® 6: software for the mapping of quantitative trait loci in experimental populations of diploid species kyazma BV. The Netherlands: Wageningen; p. 59.
- Voorrips RE. 2002. MapChart: software for the graphical presentation of linkage maps and QTLs. J Hered. 93(1):77–78. doi:https://doi.org/10.1093/jhered/93.1.77.
- Wang S, Wong D, Forrest K, Allen A, Chao S, Huang BE, Maccaferri M, Salvi S, Milner SG, Cattivelli L, et al. 2014. Characterization of polyploid wheat genomic diversity using a high-density 90 000 single nucleotide polymorphism array. Plant Biotechnol J. 12(6):787–796. doi:https://doi.org/10.1111/pbi.12183
- Wellings CR. 2007. Puccinia striiformis in Australia: a review of the incursion, evolution, and adaptation of stripe rust in the period 1979-2006. Aus J Agri Res. 58(6):567–575. doi:https://doi.org/10.1071/AR07130.
- Wen W, He Z, Gao F, Liu J, Jin H, Zhai S, Qu Y, Xia X. 2017. A high-density consensus map of common wheat integrating four mapping populations scanned by the 90K SNP array. Front Plant Sci. 8:1389. doi:https://doi.org/10.3389/fpls.2017.01389.
- Wu J, Wang Q, Liu S, Huang S, Mu J, Zeng Q, Huang L, Han D, Kang Z. 2017. Saturation mapping of a major effect QTL for stripe rust resistance on wheat chromosome 2B in cultivar napo 63 using SNP genotyping arrays. Front Plant Sci. 8:653. doi:https://doi.org/10.3389/fpls.2017.00653.
- Xi K, Kumar K, Holtz MD, Turkington TK, Chapman B. 2015. Understanding the development and management of stripe rust in central Alberta. Can J Plant Pathol. 37(1):21–39. doi:https://doi.org/10.1080/07060661.2014.981215.
- Yang EN, Rosewarne GM, Herrera-Foessel SA, Huerta-Espino J, Tang ZX, Sun CF, Ren ZL, Singh RP. 2013. QTL analysis of the spring wheat ‘Chapio’ identifies stable stripe rust resistance despite inter-continental genotype × environment interactions. Theor Appl Genet. 126(7):1721–1732. doi:https://doi.org/10.1007/s00122-013-2087-8.
- Zhang P, McIntosh RA, Hoxha S, Dong C. 2009. Wheat stripe rust resistance genes Yr5 and Yr7 are allelic. Theor Appl Genet. 120(1):25–29. doi:https://doi.org/10.1007/s00122-009-1156-5.
- Zheng S, Wu Y, Zhou M, Zeng L, Liu R, Li Y, Liu Z, Zhang C, Lu L, Zhang L. 2020. Characterization and diagnostic marker development for Yr28-rga1 conferring stripe rust resistance in wheat. Eur J Plant Pathol. 156(2):623–634. doi:https://doi.org/10.1007/s10658-019-01912-x.