Abstract
Red alder (Alnus rubra) trees form an essential component of the communities along coastal streams in the Pacific Northwest. However, canopy dieback has been observed in red alder for several years. Symptomatic trees found at two sites in the greater Vancouver area, British Columbia, Canada, were investigated for disease-causing organisms. We investigated the presence of causal agents by isolating potential pathogens using direct plating of root samples on Petri dishes and by baiting for oomycetes in soil and root samples, followed by DNA barcoding. We identified three pathogenic Phytophthora species: Phytophthora cambivora, P. syringae and P. pseudosyringae. Controlled inoculations of all three on red alder leaves indicated that only P. pseudosyringae has the potential to cause both stem canker and aerial symptoms. This is the first isolation of the tree pathogen P. pseudosyringae in Canada. Additionally, the inoculation experiments revealed that P. gonapodyides, a species that is frequently detected in riparian ecosystems with diseased alders in Oregon but not directly associated with tree mortality, was more aggressive than the three pathogenic Phytophthora species tested. Our results reveal a greater complexity than previously suspected in red alder decline.
Résumé
Les aulnes rouges (Alnus rubra) constituent une composante essentielle des peuplements qui croissent le long des cours d’eau côtiers du Nord-Ouest du Pacifique. Toutefois, depuis plusieurs années, on a observé la mort en cime chez l’aulne rouge. Des arbres symptomatiques, découverts à deux sites du grand Vancouver en Colombie-Britannique, au Canada, ont été étudiés pour connaître les organismes qui causent la maladie. Nous avons étudié les agents causaux en isolant les agents pathogènes potentiels, et ce, en plaçant des échantillons de racines directement dans des boîtes de Petri et en piégeant les oomycètes dans le sol et sur les échantillons de racines pour, par la suite, utiliser le codage à barres de l’ADN. Nous avons identifié trois espèces pathogènes de Phytophthora: Phytophthora cambivora, P. syringae et P. pseudosyringae. L’inoculation contrôlée de feuilles d’aulne rouge avec les trois espèces a indiqué que seul P. pseudosyringae pouvait causer le chancre de la tige des arbres forestiers et les symptômes aériens. Il s’agit du premier isolement de l’agent pathogène forestier P. pseudosyringae au Canada. De plus, les expériences d’inoculation ont révélé que P. gonapodyides, une espèce souvent détectée dans les écosystèmes riverains peuplés d’aulnes malades en Oregon, mais pas directement associée à la mort des arbres, était plus agressif que les trois espèces de Phytophthora pathogènes testées. Nos résultats révèlent une plus grande complexité relative au dépérissement chez l’aulne rouge que ce qui avait été pressenti jusqu’ici.
Introduction
Forest pathogens are an important contributing threat to ecosystem integrity, especially through rapid tree mortality, diebacks and declines (Lipdenmayer et al. Citation2012). The major changes in temperature and precipitation regimes predicted with climate warming could make endemic pathogens overcome their natural range and activity and increase the risk of epidemics (Altizer et al. Citation2013; Trumbore et al. Citation2015). In addition, the human-mediated introduction of forest invasive alien species (FIAS), and their potential to hybridize with endemic pests, constitutes an ongoing and significant threat to natural forest ecosystems (Loo Citation2009; Sturrock et al. Citation2011; Trumbore et al. Citation2015).
The oomycete genus Phytophthora comprises some of the most threatening pathogens of trees and can cause severe outbreaks across a wide range of environments, resulting in large economic and ecological impacts (Hansen Citation2008; Hansen et al. Citation2012; Scott et al. Citation2020). For example: the invasive Phytophthora ramorum, the cause of sudden oak death in the USA and sudden larch death in the UK, and P. lateralis, a pathogen of Port-Orford cedar in its native range on the Pacific Northwest coast (PNW) in North America, are primary mortality factors of their hosts (Hansen Citation2015). Beyond these well-documented pathogens, there is a paucity of cases where tree declines have been associated with Phytophthora species, largely because their life cycles occur in roots, soil or water, making them difficult to diagnose and control. This life-cycle makes riparian ecosystems particularly conducive to the development and spread of Phytophthora diseases. One of the most recent and significant tree health issues in riparian ecosystems is the lethal root rot and stem disease of alders in Europe, caused by two of the three species of the P. alnii s.l. species complex, P. xmultiformis and P. xalnii (Ioos et al. Citation2006; Husson et al. Citation2015). In 2013, it was estimated that this disease had killed 10% of alders in southern England and Wales, increasing at approximately 2% and spreading into surrounding European countries (CFIA, Citation2018). The severity of this European threat led to currently active Canadian directives barring the transport of Alnus spp. into Canada from Europe (Canadian Food Inspection Agency Citation2018). The last species of the complex, P. uniformis, is considered indigenous to the PNW where it does not pose a significant threat to native red alder (Alnus rubra) populations (Adams et al. Citation2008; Navarro et al. Citation2015; Aguayo et al. Citation2013), but is now frequently found in the northern parts of Europe (Aguayo et al. Citation2013; Redondo et al. Citation2015).
Red alder is an early successional tree species which provides a wide range of ecological benefits to riparian ecosystems (Harrington Citation2006). A symbiotic relationship between the roots of A. rubra and nitrogen fixing bacteria (Frankia sp.) plays a key role in soil quality and the stability of riparian water banks (Harrington Citation2006). In the PNW, red and white alder (A. rhombifolia) dieback has been observed for several years (Navarro et al. Citation2015; Sims et al. Citation2015). Visual signs and symptoms of Phytophthora infection observed on dying A. rubra and A. rhombifolia include black cankers, red weeping from the stem, cracking of the bark, decline and thinning of the crown, all of which tends to lead to eventual tree death (Sims et al. Citation2015). Several potential Phytophthora species have been associated with the dieback, among which three (P. gonapodyides, P. chlamydospora and P. siskiyouensis) seem to be consistently retrieved in declining stands (Sims et al. Citation2015; Navarro et al. Citation2015). However, no aggressive species of Phytophthora that could be directly responsible for the dieback has been identified yet (Navarro et al. Citation2015).
For several years, dieback symptoms have been observed on red alders in riparian ecosystems located in lower mainland in British Columbia (B.C.), Canada. The objective of this study was to conduct a preliminary investigation into possible causal agents that may be responsible for the symptoms observed on diseased A. rubra trees located in semi-natural environments, through the baiting of soil, plating of alder root samples, DNA barcoding identification of cultures and controlled inoculations on A. rubra.
Material and Methods
Sampling
Four plots (15.2 m x 15.2 m) located in the vicinity of the City of Burnaby, B.C., were selected for the collection of soil and root samples in October 2018. In each plot, two A. rubra trees with visual signs of bleeding cankers, dieback in the crowns and/or complete mortality, were selected. For each tree, two soil samples including fine alder roots were collected at 1 m from the base.
Pear baiting and culturing
Root and soil samples were baited with pears following the protocol established by Ivors (Citation2015a), substituting bleached and rinsed 0.5 L Tupperware containers for plastic bags, and using ‘Anjou’ pears. For the negative control, a pear was submerged in sterile water with no soil or roots. Upon appearance of a lesion (5–10 days incubation), eight 0.5 cm2 plugs were cut from the margin and transferred onto PARP(H)-CMA plates, (pimaricin, ampicillin, rifampicin, pentachloronitrobenzene, hymexazol – corn meal agar; Ivors Citation2015b). Cultures were incubated in the dark at 20°C for 5 days before DNA extraction.
DNA barcoding
For each culture, mycelium was frozen at −20°C in a 2.0 mL microcentrifuge tubes with two tungsten metals beads and then ground in a Retsch® MM 300 tissue lyzer for 2 min at a frequency of 30 oscillations/second. DNA was extracted using the DNeasy® Plant Mini Kit (Qiagen, Toronto, ON), eluted in 50 µL Buffer AE and stored in a − 20 C° freezer until PCR amplification. PCR was carried out in a Bio-Rad T100™ Thermal Cycler with a 25 µL mixture per sample containing 2.5 µL 10x PCR buffer (ThermoFisher Scientific, Carlsbad, CA), 1 µM of each of ITS6 and ITS4 primer, 1.5 mM MgCl2, 0.2 mM dNTP, 0.1 µL (0.5 U) Platinum™ Taq DNA polymerase (ThermoFisher Scientific), 2 µL of template DNA and 14.15 µL of molecular biology grade water. The cycling profile consisted of an initial denaturing step at 95°C for 3 min, then 35 cycles of 30s at 94°C, 30s at 55°C, 45s at 72°C, for denaturation, annealing and elongation, respectively, and a final extension step of 72°C for 10 min.
PCR-DNA fragments were visualized on Agarose gel (0.8 g-Agarose, 40 mL-1xTBE), prepared in standard casting trays before being sequenced on the Sanger-sequencing platform at the University of Laval in Quebec (Q.C.). Chromatograms were trimmed, assessed for quality and aligned for consensus using Sequencher® v. 5.4.6 DNA sequence analysis software (Gene Codes Corporation, Ann Arbor, MI, http://www.genecodes.com). Sequence identity was assigned using the BLASTn (Altschul et al. Citation1990) on the NCBI nucleotide collection (nt) with highest similarity homolog sequences, 10–20 of which were selected per isolate sequence and downloaded for tree creation. Maximum likelihood (ML) phylogenetic trees were constructed with RAxML (Stamatakis Citation2014). Modetest-NG (Darriba et al. Citation2020) was used to identify the best fitting nucleotide evolution model. Maximum parsimony (MP) tree was identified by conducting a heuristic search and 100 random additions of sequences (RAS) with PAUP v. 4.0b10 (Swofford Citation2003). Statistical support for nodes on the ML and MP phylogenetic trees were obtained with 100 bootstrap replicates, saved in Newick format and then edited using TreeGraph 2 (Stöver and Müller Citation2010), collapsing nodes for the minimum 50% consensus rule. Nucleotide sequences obtained in this study were deposited in GenBank under accession numbers OL830702 to OL830709.
Sequencing of ASF-like alleles in P. cambivora
Validation of the identity of P. cambivora was done by testing and sequencing the six allele-specific forward primers (ASF-PAM1-F, ASF-PAM2-F, ASF-PAU-F, ASF-PCJC17a6-F, ASF- PC643e5-F and ASF-PCJC17c6-F) and the ASF-E2-2R reverse primer targeting the single-copy ASF-like gene (Ioos et al. Citation2006). PCR and sequencing protocols were the same than those described above for DNA-barcoding with ITS4 and ITS5.
Leaf inoculations
Detached terminal (3rd leaf from a branch tip) A. rubra leaves collected from 60 cm to 80 cm DBH trees were inoculated with one isolate each of P. pseudosyringae (Alder 13) and P. syringae (Alder 3). The P. cambivora isolate obtained in this study was not inoculated as this culture did not survive storage. One P. gonapodyides isolate was used as a positive control as this species is known to be pathogenic on alder tissues (Navarro et al. Citation2015). Leaves were wounded with 5 mm incisions and inoculated with pairs of 5-mm diameter plugs (four inoculated leaves per isolate) removed from the growing margin of CMA colonies and placed ~3 cm apart on the adaxial surface of the leaf, separated by the midrib. Negative controls consisted of mock-inoculated leaves with CMA plugs without Phytophthora mycelium. The leaves were placed in large Petri dishes (15 cm-diam.) containing 2 mm of sterile dH2O. Pictures were taken of the leaves every day to monitor the growth of the lesions until the end of the experiment at 11 days post-inoculation.
Stem inoculations
The isolates used for leaf inoculations were inoculated on stems of 1-year-old nursery grown red alder seedlings (usually about 5-mm stem diameter). Nine seedlings were inoculated for each isolate, at two inoculation points on the stem spaced equally apart. At each inoculation point, a 5 to 10-mm long transverse cut was made through the bark to the cambium using a sterile scalpel. Inoculum material consisted of 5 mm diameter mycelial plugs, 2–3 mm thick, taken from 7-day-old cultures grown on CMA. The plug was inserted on the wound and covered with a moist cotton ball and then wrapped into a 2-cm wide aluminium foil. Controls consisted of nine mock-inoculated seedlings using mycelium-free CMA plugs. Inoculated plants were incubated in a growth chamber set for a 16-h light cycle and temperature of 20°C day, 15°C night. After one week of incubation, the aluminium foil and cotton ball were removed. At 3 weeks after inoculation, the uppermost bark layer was removed around the wound to reveal the margins of lesions, and lesion length and width were measured. Re-isolation of inoculated material was attempted by plating small sections of necrotic lesions on CMA-PARP(H) plates.
Results
Pear-baiting and DNA-barcoding identifications
Soil and root baiting resulted in the isolation of 17 oomycete-like cultures, among which nine Phytophthora (53%) and eight Pythium (47%) were identified by DNA-barcoding. The nine Phytophthora cultures were all isolated from samples collected at the Marine Way sites; only Pythium (four cultures) was obtained from the two other sites ().
Table 1. Number of oomycetes detected and identified in four red alder sampling sites with dieback symptoms (indicated with percentage of live crown) in the Vancouver area, B.C., Canada.
Three Phytophthora species were identified, P. syringae, P. pseudosyringae, and P. cambivora. Three ITS sequences from Marine Way site 1 and one sequence from site 2 clustered with sequences of P. syringae (Bootstrap support [BS] of 100%; ) and showed 99.8 to 100.0% similarity with the sequence of the P. syringae voucher specimen P10330 (Genbank accession number HQ261716) over an alignment of 810 base pairs (bp). Four sequences (three from Marine Way site 1 and one from Marine Way site 2) matched P. pseudosyringae sequences, forming a phylogenetic clade with a BS of 100.0% (). Each of these sequences showed a 100% similarity with sequences from the ex-type isolates PSEU6 (AY230190) and CPHST BL 51 G (MG865574) over an 805 bp alignment. Finally, one sequence from a culture obtained from a Marine Way site 1 sample (Alder24) showed 99.5% similarity with the P. cambivora voucher specimen (HQ261516), and an average of 98.9% similarity with sequences from the ex-type specimen of the P. alni complex (98.70% with P. xalni [AY689133], 99.0% with P. xmultiformis [MK786372] and 99.1% with P. uniformis [MK496514]). A maximum likelihood tree reconstruction including ITS sequences from close relatives in Phytophthora clade 7a supported clustering of this sequence with P. cambivora sequences (BS = 72.0%; ). However, this node was not statistically supported in the Maximum parsimony analysis (BS < 50.0%); only a deeper node including this sequence, and sequences from P. cambivora and P. uniformis obtained a BS > 50.0% in both analyses ().
Fig. 1 Phytophthora syringae and P. pseudosyringae isolates obtained from the red alder rhizosphere. Maximum-likelihood phylogenetic trees constructed from nucleotide alignments (A, 810 bp; B, 805 bp) of the internal transcribed spacer regions (ITS) of the nuclear rDNA including P. syringae isolates (A, Alder3, 7, 12 and 25) and P. pseudosyringae isolates (B, Alder1, 5, 9 and 13) and reference sequences for closest homologs retrieved from the NCBI database (with Genbank accession number; *, voucher specimen; **, type and ex-type specimen). Numbers above nodes are statistical support values obtained from 100 bootstrap samples in Maximum parsimony/Maximum likelihood; statistical support under 50% is not represented. Scale bars indicate the number of nucleotide substitution per site.
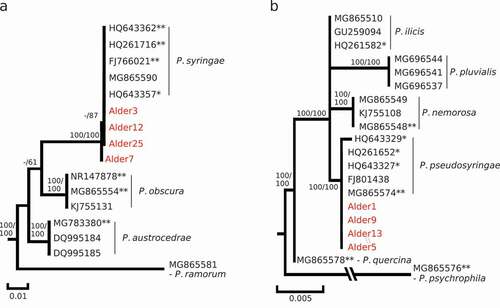
Fig. 2 Phylogenetic identification of Phytophthora cambivora (Alder24) isolated from the red alder rhizosphere. A, Maximum-likelihood phylogenetic tree constructed from a 821 bp nucleotide alignment of the internal transcribed spacer regions (ITS) of the nuclear rDNA including reference sequences for closest homologs to Alder24 retrieved from the NCBI database (with Genbank accession number; *, voucher specimen; **, type and ex-type specimen); Numbers above nodes are statistical support values obtained from 100 bootstrap samples in Maximum parsimony/Maximum likelihood. B, Nucleotide alignment of polymorphic sites in ASF-like alleles sequenced with the ASF-PAU-F/ASF-E2-2 R and ASF- PCJC17c6-F/ASF-E2-2 R primer pairs; the ASF-like alleles sequenced in the Alder24 culture are indicated in red; PAU, P. uniformis cluster; PAM1 and 2, P. xmultiformis clusters as described in Ioos et al. (Citation2006). C, Maximum-likelihood phylogenetic tree constructed from a 411bp nucleotide alignment of ASF-like alleles obtained for Alder24 and related ASF-alleles for the PAU, PAM1, PAM2, P. cambivora clusters described in Ioos et al. (Citation2006); Numbers above nodes are statistical support values obtained from 100 bootstrap samples in Maximum parsimony/Maximum likelihood.
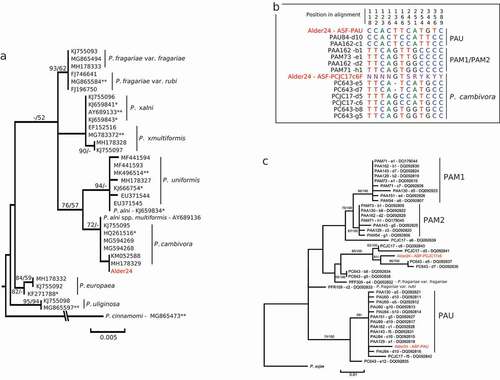
To test the hypothesis that the Alder24 culture could be a member of the P. alni complex, we tested the PCR-amplification of its DNA with the six allele-specific forward primers (ASF-PAM1-F, ASF-PAM2-F, ASF-PAU-F, ASF- PCJC17a6-F, ASF- PC643e5-F and ASF- PCJC17c6-F) and the ASF-E2-2R reverse primer targeting the single-copy ASF-like gene (Ioos et al. Citation2006). Only two primer combinations resulted in PCR products: ASF-PAU-F/ASF-E2-2R and ASF-PCJC17c6-F/ASF-E2-2R. Sequencing of the PCR product (renamed Alder24 – ASF-PAU) generated with the ASF-PAU-F/ASF-E2-2R combination revealed five private polymorphisms shared with the unique allele obtained from three P. uniformis (PAU60, PAU84 and PAU89) and five P. xalni (PAA129, PAA130, PAA142, PAA151 and PAA162) isolates sampled in Europe (alignment positions 118, 122, 123, 126 and 134; ). ML and MP phylogenetic analysis supported this similarity by including this allele in the P. uniformis (PAU) cluster described in Ioos et al. (Citation2006). However, the node for this cluster was statistically supported only in MP with a BS of 59.0% () and the hypothesis of a P. uniformis or P. xalni identification for this culture was rejected as the allele sequenced could be differentiated from the corresponding allele in P. uniformis and P. xalni by three substitutions (positions 166, 344 and 356; ). Sequencing of P. cambivora-specific allele (Alder24 – ASF-PCJC17c6 amplified with ASF- PCJC17c6-F/ASF-E2-2R) in Alder24 resulted in a 282 bp sequence that contained six intra-individual polymorphisms (revealed by overlapping signal in the sequencing chromatogram), suggesting the amplification of two additional alleles in the Alder24 culture (). Three of these overlaps corresponded to a superposition of one nucleotide specific to an allele described for P. cambivora and another specific to a second allele described for the same species in Ioos et al. (Citation2006) (positions 245, 251 and 344; ).
Inoculation tests
Only P. pseudosyringae and P. gonapodyides induced necrotic lesions on red alder leaves 10 days post-inoculation (dpi) (). The average area of the lesions induced by P. pseudosyringae was significantly lower than the area observed for the P. gonapodyides control at 4 and 6 dpi; at 10 dpi, the difference between these two species was not significant, likely due to a strong variance between P. gonapodyides replicates (). The average area observed for P. syringae was not different from the control and near to null at each time-point (). Both P. syringae and P. gonapodyides were successfully re-isolated from infected leaves on CMA-PARP(H) medium, completing Koch’s postulates.
Fig. 3 Inoculations of leaves (A and B) and stems (C and D) of red alder under controlled conditions with Phytophthora syringae and P. pseudosyringae and P. gonapodyoides used as a positive control. A, necrotic lesion symptoms on red alder leaves 10 days post-inoculation (dpi). B, development of necrotic lesion area over a 10 days period following inoculation of red alder leaves. The data at each time-point are the means ± SD of three replicates; for each time-point, means sharing a letter are not significantly different (P > 0.05) according to a Tukey’s HSD test. C, canker symptoms on red alder stems 10 weeks post-inoculation (wpi). C, necrotic canker area at 10 wp. Boxplot means (from alder seedling replicates for each isolate) sharing a letter are not significantly different (P > 0.05) according to a Tukey’s HSD test.
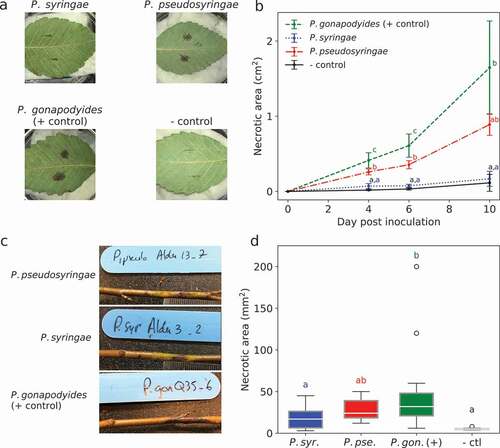
The pathogenicity trial on red alder seedlings showed that P. pseudosyringae, P. syringae and P. gonapodyides can cause a progressive necrotic lesion from the site of inoculation at 3 weeks post-inoculation (). Only the average lesion area measured for P. gonapodyides was significantly greater for the control (45.6 mm2 ± 50.6 mm2 vs. 5.4 mm2 ± 1.4 mm2; T-test value = 4.28; P < 0.001). However, values measured for P. pseudosyringae (27.9 mm2 ± 12.9 mm2) were in the same range as those of P. gonapodyides (T-test value = 1.93 P < 0.22) and higher but not significantly different from those obtained for P. syringae (17.8 mm2 ± 12.6 mm2; T-test value = 1.1 P < 0.68) ().
Discussion
Herein we provide a first overview on Phytophthora species found in the red alder rhizosphere in the lower mainland of British Columbia. Sampling of the soil rhizosphere of four trees in two adjacent sites resulted in the isolation of three Phytophthora species reported as emerging invasive tree pathogens in Europe (Jung et al. Citation2003, Citation2005; Webber and Brasier Citation2005). We obtained cultures of P. cambivora, a common pathogen associated with tree root rot and bleeding cankers that has been recognized as an occasional alder pathogen in Europe (Brasier and Kirk Citation2001). We also found P. syringae, a pathogen with a wide host range causing crown rot, collar rot, stem canker and wilting on trees and shrubs (Erwin and Ribeiro Citation1996). We also identified the tree and shrub pathogen P. pseudosyringae. This is the first time that this pathogen is reported from Canada (CABI EPPO Citation2010).
The ITS sequence of one of the P. cambivora cultures obtained in this study had a strong similarity with both P. cambivora and the three species of the P. alni complex (98.7 to 99.5%) described in Europe (Husson et al. Citation2015). Sequencing of the different alleles of the nuclear ASF-like gene was required to validate the identification of this culture. We confirmed the strong relatedness between this P. cambivora culture, P. uniformis and P. xalni, with one ASF-like allele (ASF-PAU) shared between the three species. However, the hypothesis of an identity of this culture to P. uniformis or P. xalni was rejected as two P. cambivora-specific ASF-like alleles were also amplified (with ASF-PCJC17c6). In addition, the presence of three fixed polymorphisms between the ASF-PAU allele usually sequenced in P. uniformis and the corresponding allele sequenced in the P. cambivora isolate obtained in our study support the conclusions made by Ioos et al. (Citation2006) regarding the absence of a direct parent-progeny relationship between P. cambivora and P. uniformis.
Simultaneous infestations by multiple oomycete species have been associated with tree diebacks (Jung and Blaschke Citation2004; Corcobado et al. Citation2017; Ruffner et al. Citation2019). For instance, multiple Phytophthora species have been detected in soil rhizosphere samples of keystone tree species such as in declining forest ecosystems (Scanu et al. Citation2015; Jung et al. Citation2017; Ruffner et al. Citation2019). Among the three Phytophthora species isolated from the same rhizosphere sample in this study, P. cambivora and P. pseudosyringae were already known to co-infest riparian alder stands in western Oregon and Alaska (Navaro et al. Citation2015; Sims et al. Citation2015; Reeser et al. Citation2011). Although the direct association of these species with alder dieback symptoms has not been clearly established, their involvement in weakening alder root systems and predisposing the tree to other pathogens has been hypothesized (Sims et al. Citation2015; Navarro et al. Citation2015). Specifically, P. pseudosyringae had been reported to cause root rot and stem canker on the riparian alder species A. glutinosa in Europe (Jung et al. Citation2003). Our inoculation results confirmed that this species is pathogenic on red alder by inducing visible canker-like lesions on stems and necrosis on leaves. However, no significant differences in aggressiveness were observed between the P. pseudosyringae isolate that was tested and the P. gonapodyides used, which is part of the group of the three main species (with P. chlamydospora and P. siskiyouensis) that are consistently found in declining alder stands in Oregon (Sims et al. Citation2015; Navarro et al. Citation2015). The fact that P. pseudosyringae induces necrosis symptoms on red alder leaves is striking, particularly when compared with one of the other species identified in this study, P. syringae, which was in our experiment completely innocuous on red alder leaves. The Phytophthora genus is subdivided in 10 clades based on phylogenetic analysis and P. pseudosyringae is part of the phylogenetic clade 3 (Martin et al. Citation2014). This clade is often referred to include ‘aerial’ pathogens (Scanu and Webber Citation2016) that can infect some trees through the stems, branches and leaves by aerial spores (Scanu and Webber Citation2016). For example, two closely related species included in clade 3, P. ilicis and P. nemerosa, have for substrate leaves, twigs and the bark of holly and oak, respectively (Hansen et al. Citation2003; Scanu et al. Citation2014). Another member of clade 3, P. pluvialis, infects Pinaceae needles, causing a needle cast disease on radiata pine and Douglas-fir in the Pacific Northwest and New Zealand (Brar, Citation2018). In contrast, P. syringae is typically a ‘soil pathogen’, which has been widely reported in plant nurseries on the US west coast causing root rot and decay (Parke et al. Citation2014). Its presence in forest streams and soils in natural habitats on the Pacific Northwest coast is rather anecdotal and this pathogen has always been considered non-threatening in natural forests (Hansen Citation2015). However, given the wide range of symptoms caused by P. syringae and its potential to survive severe droughts in dormant states (Pérez-Sierra et al. Citation2013), the possibility that P. syringae plays a secondary role in red alder decline depending on different environmental conditions should still be investigated.
In conclusion, recent surveys in undisturbed natural environments have uncovered the presence of many Phytophthora species, sometimes in high abundance, generally in the absence of apparent disease on associated vegetation (Hansen et al. Citation2012; Burgess et al. Citation2009; Jung et al. Citation2017). With continuous human expansion and increased environmental pressures linked to global climate change, more research is needed to determine which Phytophthora species are native or introduced, and what effects these pathogens could have on sensitive ecosystems and hosts, like A. rubra (Harvell et al., Citation2002; Desprez-Loustau et al., Citation2007). Red alder trees are a pioneer species in the early successional stages of coastal riparian ecosystems in the PNW. They fix nitrogen for soil quality, increase bank stability, and provide shade to streams and habitat for wildlife. Disturbance in red alder ecosystems could lead to an increase of water runoff, erosion of streambanks, a decrease in soil quality, and overall decline of the thermal insulative properties which these trees provide. Monitoring the health of a short-lived pioneer species like A. rubra may serve as a tool to predict the decline of other tree species, via the identification of pathogens known to have a wide host range. Greater efforts should be put forth to improve understanding of what is causing dieback in A. rubra and how.
Disclosure statement
No potential conflict of interest was reported by the author(s).
Additional information
Funding
References
- Adams GC, Catal M, Trummer L, Hansen EM, Reeser P, Worrall JJ. 2008. Phytophthora alni subsp. uniformis found in Alaska beneath thin leaf alders. Plant Hea. Prog. 9:1.
- Aguayo J, Adams GC, Halkett F, Catal M, Husson C, Zá N, Hansen EM, Marçais B, Frey P. 2013. Strong genetic differentiation between North American and European populations of Phytophthora alni subsp. uniformis. Phytopathology. 103(2):190–199. doi:https://doi.org/10.1094/PHYTO-05-12-0116-R.
- Altizer S, Ostfeld RS, Johnson PTJ, Kutz S, Harvell CD. 2013. Climate change and infectious diseases: from evidence to a predictive framework. Science. 341(6145):514–519. doi:https://doi.org/10.1126/science.1239401.
- Altschul SF, Gish W, Miller W, Myers EW, Lipman DJ. 1990. Basic local alignment search tool. J. Mol. Biol. 215(3):403–410. doi:https://doi.org/10.1016/S0022-2836(05)80360-2.
- Brar S, Tabima JF, McDougal RL, Dupont P-Y, Feau N, Hamelin RC, Panda P, LeBoldus JM, Grünwald NJ, Hansen EM, et al. 2018. Genetic diversity of Phytophthora pluvialis, a pathogen of conifers, in New Zealand and the west coast of the United States of America. Plant Pathol. 67(5):1131–1139. doi:https://doi.org/10.1111/ppa.12812.
- Brasier CM, Kirk SA. 2001. Comparative aggressiveness of standard and variant hybrid alder phytophthoras, Phytophthora cambivora and other Phytophthora species on bark of Alnus, Quercus and other woody hosts. Plant Pathol. 50(2):218–229. doi:https://doi.org/10.1046/j.1365-3059.2001.00553.x.
- Burgess TI, Webster JL, Ciampini JA, White D, GEStJ H, Stukely MJC. 2009. Re-evaluation of Phytophthora species isolated during 30 years of vegetation health surveys in Western Australia using molecular techniques. Plant Dis. 93(3):215–223. doi:https://doi.org/10.1094/PDIS-93-3-0215.
- CABI/EPPO. 2010. Phytophthora pseudosyringae. [Distribution map]. Distribution Maps of Plant Diseases, No. October, Wallingford (UK): CABI. https://www.cabi.org/isc/datasheet/109197
- Canadian Food Inspection Agency. 2018. D-00-08: requirements to Prevent the Introduction of undescribed species of Phytophthora pathogenic to Alder (Alnus spp.), Effective Date: 2013 Apr 10 Effective Date. https://inspection.canada.ca/plant-health/invasive-species/directives/date/d-00-08/eng/1312332936247/1312333485361
- Corcobado T, Miranda-Torres JJ, Martín-García J, Jung T, Solla A. 2017. Early survival of Quercus ilex subspecies from different populations after infections and co-infections by multiple Phytophthora species. Plant Pathol. 66(5):792–804. doi:https://doi.org/10.1111/ppa.12627.
- Darriba D, Posada D, Kozlov AM, Stamatakis A, Morel B, Flouri T. 2020. ModelTest-NG: a new and scalable tool for the selection of DNA and protein evolutionary models. Mol. Biol. Evol. 37(1):291–294. doi:https://doi.org/10.1093/molbev/msz189.
- Desprez-Loustau ML, Robin C, Reynaud G, Déqué M, Badeau V, Piou D, Husson C, and Marçais B. 2007. Simulating the effects of a climate-change scenario on the geographical range and activity of forest-pathogenic fungi. Can. J. Plant Pathol. 29(2):101–120.
- Erwin DC, Ribeiro OK. 1996. Phytophthora Diseases Worldwide. APS Press, St. Paul, Minnesota; p. 562.
- Hansen EM. 2008. Alien forest pathogens: phytophthora species are changing world forests. Boreal Env. Res. 13:33–41.
- Hansen EM. 2015. Phytophthora species emerging as pathogens of forest trees. Curr. Forestry Rep. 1(1):16–24. doi:https://doi.org/10.1007/s40725-015-0007-7.
- Hansen EM, Reeser PW, Davidson JM, Garbelotto M, Ivors K, Douhan L, Rizzo DM. 2003. Phytophthora nemorosa, a new species causing cankers and leaf blight of forest trees in California and Oregon, U.S.A. Mycotaxon. 88:129–138.
- Hansen EM, Reeser PW, Sutton W. 2012. Phytophthora beyond agriculture. Ann. Review of Phytopathol. 50(1):359–378. doi:https://doi.org/10.1146/annurev-phyto-081211-172946.
- Harrington CA 2006. Biology and ecology of red alder. In Red alder — a state of knowledge. Edited by Deal RL, Harrington CA. General Technical Report. PNW-GTR-669. U.S. Department of Agriculture, Forest Service, Pacific Northwest Research Station. Portland, Ore. pp. 21–43.
- Harvell CD, Mitchell CE, Ward JR, Altizer S, Dobson AP, Ostfeld RS, and Samuel MD. 2002. Climate warming and disease risks for terrestrial and marine biota. Science. 296:2158–2162.
- Husson C, Aguayo J, Revellin C, Frey P, Ioos R, Marçais B. 2015. Evidence for homoploid speciation in Phytophthora alni supports taxonomic reclassification in this species complex. Fungal Genet. Biol. 77:12–21. doi:https://doi.org/10.1016/j.fgb.2015.02.013.
- Ioos R, Andrieuz A, Marçais B, Frey P. 2006. Genetic characterization of the natural hybrid species Phytophthora alni as inferred from nuclear and mitochondrial DNA analyses. Fungal Genet. Biol. 43(7):511–529. doi:https://doi.org/10.1016/j.fgb.2006.02.006.
- Ivors K. 2015a. PROTOCOL 01-11.1: isolation and detection of Phytophthora spp. using Rhododendron leaf baits. In: Ivors KL, editor. Laboratory Protocols for Phytophthora Species. St., pp. 1–2. Paul (MN): APS Press.
- Ivors K. 2015b. PROTOCOL 07-03.1: PARP(H)-CMA. In: Ivors KL, editor. Laboratory Protocols for Phytophthora Species. St. Paul (MN): APS Press.
- Jung T, Blaschke M. 2004. Phytophthora root and collar rot of alders in Bavaria: distribution, modes of spread and possible management strategies. Plant Pathol. 53(2):197–208. doi:https://doi.org/10.1111/j.0032-0862.2004.00957.x.
- Jung T, Hudler GW, Jensen-Tracy SL, Griffiths HM, Fleischmann F, Osswald W. 2005. Involvement of Phytophthora species in the decline of European beech in Europe and the USA. Mycologist. 19:159–166.
- Jung T, Jung MH, Cacciola SO, Cech T, Bakonyi J, Seress D, Mosca S, Schena L, Seddaiu S, Pane A, et al. 2017. Multiple new cryptic pathogenic Phytophthora species from Fagaceae forests in Austria, Italy and Portugal. IMA Fung. 8(2):219–244. doi:https://doi.org/10.5598/imafungus.2017.08.02.02.
- Jung T, Nechwatal J, Cooke DE, Hartmann G, Blaschke M, Osswald WF, Duncan JM, Delatour C. 2003. Phytophthora pseudosyringae sp. nov., a new species causing root and collar rot of deciduous tree species in Europe. Mycol Res. 107(7):772–789. doi:https://doi.org/10.1017/S0953756203008074.
- Lindenmayer DB, Laurance WF, Franklin JF. 2012. Global decline in large old trees. Science. 338(6112):1305–1306. doi:https://doi.org/10.1126/science.1231070.
- Loo JA. 2009. Ecological impacts of non-indigenous invasive fungi as forest pathogens. Biol. Invasions. 11(1):81–96. doi:https://doi.org/10.1007/s10530-008-9321-3.
- Martin FN, Blair JE, Coffey MD. 2014. A combined mitochondrial and nuclear multilocus phylogeny of the genus Phytophthora. Fungal Genet. Biol. 66:19–32. doi:https://doi.org/10.1016/j.fgb.2014.02.006.
- Navarro S, Sims L, Hansen E. 2015. Pathogenicity to alder of Phytophthora species from riparian ecosystems in western Oregon. For. Path. 45:358–366. doi:https://doi.org/10.1111/efp.12175.
- Parke JL, Knaus BJ, Fieland VJ, Lewis C, Grünwald NJ. 2014. Phytophthora community structure analyses in Oregon nurseries inform systems approaches to disease management. Phytopathology. 104:1052–1062.
- Pérez-Sierra A, López-García C, León M, García-Jiménez J, Abad-Campos P, Jung T. 2013. Previously unrecorded low temperature Phytophthora species associated with Quercus decline in a Mediterranean forest in Eastern Spain. Forest Pathol. 43:331–339. doi:https://doi.org/10.1111/efp.12037.
- Redondo MA, Boberg J, Olsson CH, Oliva J. 2015. Winter conditions correlate with Phytophthora alni subspecies distribution in Southern Sweden. Phytopathology. 105(9):1191–1197. doi:https://doi.org/10.1094/PHYTO-01-15-0020-R.
- Reeser PW, Sutton W, Hansen EM, Remigi P, Adams GC. 2011. Phytophthora species in forest streams in Oregon and Alaska. Mycologia. 103(1):22–35. doi:https://doi.org/10.3852/10-013.
- Ruffner B, Rigling D, Schoebel CN. 2019. Multispecies Phytophthora disease patterns in declining beech stands. For Path. 49:e12514.
- Scanu B, Linaldeddu BT, Deidda A, Jung T. 2015. Diversity of Phytophthora species from declining Mediterranean maquis vegetation, including two new species, Phytophthora crassamura and P. ornamentata sp. nov. PLoS ONE. 10:e0143234.
- Scanu B, Linaldeddu BT, Peréz-Sierra A, Deidda A, Franceschini A. 2014. Phytophthora ilicis as a leaf and stem pathogen of Ilex aquifolium in Mediterranean Islands. Phytopathol. Mediterr. 53:480–490.
- Scanu B, Webber JF. 2016. Dieback and mortality of Nothofagus in Britain: ecology, pathogenicity and sporulation potential of the causal agent Phytophthora pseudosyringae. Plant Pathol. 65(1):26–36. doi:https://doi.org/10.1111/ppa.12399.
- Scott P, Martin K-F, Baderc MK-F, Burgess T, Hardy G, Williams N. 2019. Global biogeography and invasion risk of the plant pathogen genus Phytophthora. Environ. Sci. Policy. 101:175–182. doi:https://doi.org/10.1016/j.envsci.2019.08.020.
- Sims LL, Sutton W, Paul Reeser P, Hansen EM. 2015. The Phytophthora species assemblage and diversity in riparian alder ecosystems of western Oregon, USA. Mycologia. 107:889–902.
- Stamatakis A. 2014. RAxML Version 8: a tool for phylogenetic analysis and post-analysis of large phylogenies. Bioinformatics. 30(9):1312–1313. doi:https://doi.org/10.1093/bioinformatics/btu033.
- Stöver BC, Müller KF. 2010. TreeGraph 2: combining and visualizing evidence from different phylogenetic analyses. BMC Bioinformatics. 11(1):7. doi:https://doi.org/10.1186/1471-2105-11-7.
- Sturrock RN, Frankel SJ, Brown AV, Hennon PE, Kliejunas JT, Lewis KJ, Worrall JJ, Woods AJ. 2011. Climate change and forest diseases. Plant Pathol. 60(1):133–149. doi:https://doi.org/10.1111/j.1365-3059.2010.02406.x.
- Swofford DL. 2003. PAUP*: phylogenetic analysis using parsimony (*and other methods). Version 4.0b10. Sunderland (MA): Sinauer Associates Inc.
- Trumbore S, Brando P, Hartmann H. 2015. Forest health and global change. Science. 349(6250):814–818. doi:https://doi.org/10.1126/science.aac6759.
- Webber JF, Brasier CM Global pathways for tree pathogens: the challenge of Phytophthora species as invasive threats, Proceedings of the BCPC International Congress – Crop Science and Technology 2005, Vol. 2 (pp. 741–748). Glasgow, Scotland.