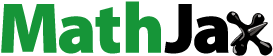
Abstract
Cercospora beticola populations resistant to methyl benzimidazole carbamate (MBC), quinone outside inhibitor (QoI), and demethylation inhibitor (DMI) fungicides have been reported in Egypt. This pathogen causes a difficult to control variant of Cercospora leaf spot (CLS) on sugar beet. We conducted field experiments over two years to investigate treatment efficacies on MBC- and QoI-resistant, but partially DMI-sensitive, C. beticola from a research farm in Sakha, Egypt. DMI fungicides showed moderate efficacy with half the maximal effective concentration (EC50). Efficacy was 67.2–69.1% and 63.4–63.6% for epoxiconazole (EPO) and propiconazole (PRO), respectively. When each fungicide was mixed with salicylic acid (SA) at EC50 (EPO + SA) and EC50 (PRO +SA), efficacy was 77.5–79.1% and 77.0–78.2%, respectively. The QoI fungicide, azoxystrobin, showed lower efficacy (46.4–49.5%) when applied alone, but enhanced efficacy when combined with SA at EC50/EC50 (77.0–78.2%). Carbendazim alone was only 47.5–45.1% effective, but when mixed with SA, had a 67.1% co-toxicity factor in vitro, indicating antagonism between components. An Artemisia cina aqueous extract (WEA) combined with SA (WEA/SA) showed 76.0–78.2% efficacy, compared with 69.0–71.0% and 49.0–52.7% for WEA and SA, respectively. Single treatments were classified into five sensitivity classes from sensitive (<1) to resistant (>100) based on their EC50 values. Significant increases in insensitivity were observed from 2015/16 to 2017/18. All treatments increased total yield, sucrose percentage, and recoverable white sugar compared with the untreated control. A combination of control strategies could allow for effective disease and fungicide resistance management.
Résumé
Des populations de Cercospora beticola résistantes au carbamate de méthyl benzimidazole (CMB), à l’inhibiteur externe de la quinone (IQe) et aux fongicides inhibiteurs de la déméthylation (IDM) ont été rapportées en Égypte. Cet agent pathogène engendre un variant de la cercosporose difficile à maîtriser chez la betterave à sucre. Nous avons mené des expériences au champ pendant plus de deux ans pour étudier l’efficacité des traitements chez C. beticola résistant au CMB et à l’IQe, mais partiellement résistant à l’IDM, dans une ferme expérimentale de Sakha, en Égypte. Les fongicides à base d’IDM ont affiché une efficacité modérée avec la moitié de la concentration maximale efficace (EC50). L’efficacité était de 67,2 à 69,1% et de 63,4 à 63,6% pour l’époxiconazole (EPO) et le propiconazole (PRO), respectivement. Lorsque chaque fongicide était mélangé à de l’acide salicylique (AS) à EC50 (EPO + AS) et EC50 (PRO + AS), l’efficacité était de 77,5 à 79,1% et de 77,0 à 78,2%, respectivement. Le fongicide à base d’IQe, l’azoxystrobine, a affiché une efficacité plus faible (46,4 à 49,5%) lorsque appliqué seul, mais une efficacité accrue lorsque combiné à l’AS à EC50/EC50 (77,0 à 78,2%). Le carbendazime appliqué seul n’était efficace que de 47,5 à 45,1%, mais, lorsque combiné à l’AS, affichait un facteur de cotoxicité de 67,1% in vitro, indiquant un antagonisme entre les composants. Un extrait aqueux d’Artémisia cina (EAA) combiné à l’AS (EAA/AS) a affiché une efficacité de 76,0 à 78,2%, comparativement à une de 69,0 à 71,0% et de 49,0 à 52,7% pour l’EAA et l’AS, respectivement. Les traitements uniques ont été classés en cinq classes de sensibilité, de sensible (< 1) à résistant (> 100), en se basant sur leurs valeurs d’EC50. Des augmentations significatives en matière d’insensibilité ont été observées de 2015-2016 à 2017-2018. Tous les traitements ont accru le rendement total, le pourcentage de sucrose et la teneur en sucre blanc extractible, comparativement au témoin non traité. Une combinaison des stratégies de lutte pourrait permettre une gestion efficace de la maladie et de la résistance aux fongicides.
Introduction
Cercospora leaf spot (CLS), caused by the fungal pathogen Cercospora beticola, first described by Saccardo (Citation1876) in Italy, is the most prevalent foliar disease of sugar beet (Beta vulgaris L. ssp. vulgaris) worldwide (Holtschulte Citation2000; Jacobsen et al. Citation2009; Khan and Carlson Citation2009). CLS causes leaf regrowth, forcing the plant host to reroute energy from root growth and sugar production towards leaf growth. As its severity increases, it lowers root yield, sugar content, and quality. This can lead to decreased total sugar production by as much as 42%; further, 40%–100% yield losses can occur in the absence of fungicide treatment (Shane and Teng Citation1992; Jacobsen et al. Citation2009). Various preventive and systemic fungicides have been used in different parts of the world to control CLS. The chemical classes of fungicides available include (a) protective dithiocarbamate (Fungicide Resistance Action Committee (FRAC) Code M 03) and phenytoin derivatives, (b) MBC -fungicides (methyl benzimidazole carbamates) (FRAC Code 1) with systemic and therapeutic activity, (c) DMI fungicides)demethylation inhibitors) (FRAC Code 3) (including epoxiconazole (EPO) and propiconazole (PRO), and amines, and (d) the relatively new and highly effective exogenous QoI-fungicides (FRAC Code 11, Quinone outside Inhibitors); DMIs and QOIs have both preventive and curative effects (Ioannidis et al. Citation2010). Foliar application of these active ingredients is meant to control CLS on sugar beet (Tedford et al. Citation2019). The resistance of C. beticola to fungicides typically develops more quickly if a fungicide is repeatedly used within one growing season. Resistance to MBCs has been reported in many countries (Georgopoulos and Dovas Citation1973; Weiland and Halloin Citation2001), additionally, resistance to DMIs also occurs (Karaoglanidis et al. Citation2000). The occurrence of such resistance, its consequences, and the associated concerns, have been comprehensively summarized (Skaracis et al. Citation2010). Currently, chemical-control strategies for CLS involve different classes of fungicides, mixture rotations, and preservation. Spraying frequency is minimized while ensuring responsiveness to disease pressure (Ioannidis et al. Citation2010).
A previous study described C. beticola strains resistant to QoIs, MBCs, and DMIs (Chahal et al. Citation2020). CLS is an annual threat in many beet-growing regions, causing low crop yield and sugar losses (Jacobsen et al. Citation2009). Notably, disease severity may intensify at a relative humidity (RH) > 65% and temperatures >15°C (Franc Citation2010). The pathogen causes sugar beet yield losses by decreasing photosynthetic capacity, which lowers harvest yield and the percentage of extractable sugars (Jacobsen et al. Citation2009; Bolton et al. Citation2012). Moreover, roots harvested from CLS-affected beet plants may have poor post-harvest storability (Jacobsen et al. Citation2009). Cercospora beticola cultures resistant to MBC and QoI fungicides have been isolated (Trueman et al. Citation2013; Tedford et al. Citation2019). DMI fungicides are the most effective for controlling CLS (Trueman and Burlakoti Citation2015).
Novel disease management strategies should include integrated approaches involving the application of alternative fungicides to overcome C. beticola fungicide resistance resulting from product overuse and to mitigate environmental pollution caused by such agents. Nevertheless, chemical treatment remains the dominant method of CLS control. MBCs and DMIs have been the main systemic fungicides used to control sugar beet CLS. Over the last decade, however, QoIs have come into use (Secor et al. Citation2010b). DMI fungicides inhibit cytochrome P450-dependent sterol-14α-demethylase activity during fungal ergosterol biosynthesis. As DMI fungicides have a single-site mode of action, there is a high risk of pathogen resistance developing (Yuzo and Yuri Citation1987; Karaoglanidis et al. Citation2000; Ioannidis et al. Citation2010; Secor et al. Citation2010a, Citation2010b; Trueman et al. Citation2017). MBC fungicides also have a single-site mode of action. These agents interfere with mitosis and disrupt fungal cytoskeleton integrity by binding β-tubulin (Sisler Citation1988). MBC resistance has already been observed in C. beticola (Percich et al. Citation1987; Rosenzweig et al. Citation2015). QoI fungicides inhibit cellular respiration by binding the ubiquinol oxidation centre (Qo site) of the mitochondrial cytochrome bc1 complex (Sauter et al. Citation1999; Bartlett et al. Citation2002). QoI fungicide resistance has also been found in C. beticola in sugar beet-producing regions (Bartlett et al. Citation2002; Trueman et al. Citation2013; Bolton et al. Citation2013a, Citation2013b; Rosenzweig et al. Citation2015). Hence, CLS management in sugar beet requires an integrated approach that utilizes resistant cultivars and a combination of foliar fungicides (Secor et al. Citation2010b).
Mixtures of DMI and QoI fungicides have been applied and tested on sugar beet in accordance with disease and pathogen resistance forecasting (Khan et al. Citation2007; Kaiser et al. Citation2010). Sensitivity monitoring is also invaluable in the development of fungicide resistance management strategies (Thomas et al. Citation2012). Intensive MBC use for CLS control has culminated in the rapid emergence of MBC-resistant C. beticola populations and dramatic decreases in field efficacy (Marić et al. Citation1976). To attenuate the onset and propagation of resistant pathogen populations, systemic fungicides are combined with protectants, such as chlorothalonil, fentin acetate, and fentin hydroxide (Karaoglanidis and Ioannidis Citation2010). The methods of blending and applying QoI and DMI ultimately influence C. beticola resistance (Karaoglanidis and Thanassoulopoulos Citation2003; Secor et al. Citation2010b). MBCs have been used in mixtures rather than as standalone formulations (Trkulja et al. Citation2009). In general, DMI fungicides have been most effective at controlling CLS. Plants treated with various combinations of such agents produce more sugar and have lower disease severity than untreated controls (Trueman and Burlakoti Citation2015).
Artemisia species, widespread throughout the world, are medicinal and aromatic plants. Many members of the genus produce essential oils and are used in modern folk medicine to control fungal, bacterial, and viral infections (Abad et al. Citation2012). The antifungal activity of these natural products is attributed to the presence of biologically active compounds, which have been reported to be effective against Alternaria solani (Ahmadi et al. Citation2010; Derbalah et al. Citation2012). Furthermore, aqueous extracts of Artemisia cina are effective against squash powdery mildew caused by Pseudoperonospora cubensis (Morsy Citation2010); its fungicidal activity may be due to its santonin (2–3.5%), artemisin (8- hydroxyl santonin), cineole, terpineol, and terpinene contents (Balbaa et al. Citation1976). Although the antifungal activities of such natural products are mainly attributable to their previously mentioned major constituent compounds, the synergistic or antagonistic effects of some compounds in the mixtures should be taken into account (Ragasa et al. Citation2002). Each component influences the biological activity of the extract against the pathogens tested.
Salicylic acid (SA) induces host resistance, which provides partial protection against C. beticola infection. Systemic acquired resistance (SAR) induced by SA reduces CLS by 70–91% in susceptible taxa and presents a degree of efficacy similar to those of the fungicides commonly used in species more resistant to fungal pathogens (Jacobsen et al. Citation2004). Host plant disease resistance is associated with the production of SA-associated pathogen resistance (PR) proteins, including peroxidase, 13-glucanase, and chitinase (Jacobsen et al. Citation2004). Although breeding sugar beet varieties for genetic resistance to CLS strains has been recently carried out globally, such strategies have been unsuccessful (Holtschulte et al. Citation2010); notably, Egypt cannot develop sugar beet varieties locally as the climate is not conducive for flowering. Therefore, it relies on imports from Europe and North America. To develop efficacious and environmentally safe management strategies against CLS caused by C. beticola resistant to QoI, MBC, and DMI, we carried out in vitro and in vivo trials to (i) establish the current status of QoI, MBC, and DMI efficacy against CLS in Egypt, (ii) identify the occurrence and quantities of fungicide-resistant C. beticola isolates, (iii) determine how increases in fungicide resistance influence CLS management status and perspectives, (iv) address resistance to QoI and DMI by combining the agents with SA-triggered host PR, and (v) test safe fungicide alternatives such as aqueous extracts of A. cina, SA, and their combinations. We demonstrated that aqueous extracts of A. cina, alone or mixed with SA, were effective in overcoming C. beticola resistance to fungicides and increasing root yield and recoverable white sugar (RWS).
Materials and methods
Cercospora beticola isolate collection
Cercospora beticola isolates were collected from the leaves of sugar beet plants with CLS symptoms in the fields of the Sakha Agricultural Research Station, Kafrelsheikh, Egypt. The sugar beet (Beta vulgaris L. ‘Pleno’) tested here is susceptible to C. beticola (El-Sayed Citation2000). Up to 20 leaves per infected plant were collected in 2015/16, 2016/17, and 2017/18. Pure cultures were isolated from individual CLS lesions, and their sensitivities to the compounds listed in were assessed. Briefly, the leaves were surface-disinfected with a 10% (v/v) NaClO solution, placed into plastic bags with moist paper towels to maintain humidity near 100%, and placed under fluorescent lights with an 8-h photoperiod at 24°C for 7 d to promote sporulation. Spore-bearing lesions of similar size and sporulation stage were selected. Cercospora beticola conidia were transferred to glass Petri dishes (9 cm) containing Sugar Beet Leaf Extract, Dextrose, and Agar medium (SBLEDA) consisting of beet leaf extract (100 mL), dextrose (20 g), and agar (15 g). Streptomycin (40 ppm) was added to the media to prevent bacterial contamination. The Petri dishes were incubated at 27 ± 2°C for 3–7 d and inspected daily for signs of fungal growth. The fungi were examined microscopically and purified using the hyphal tip technique, as previously described (Dhingra and Sinclair Citation1995; Derbalah et al. Citation2013). Pure C. beticola cultures were isolated on potato dextrose agar (PDA, El Nasr Pharmaceutical Chemicals Co. (ADWIC). EGYPT.) for the subsequent fungicide sensitivity assays. Fungal isolates were immediately screened to avoid loss of sensitivity caused by subculturing and/or long-term storage.
Table 1. Fungicides and plant resistance inducers evaluated in vitro and in vivo against Cercospora beticola.
Preparation of aqueous Artemisia cina extract
Whole A. cina plants were air-dried at room temperature and ground in a blender (Chain, BEIJAMEI Commercial grain flour mill grinder stainless, 500 g) to a fine powder. A 100-g mixture of powdered A. cina leaves and flowers (1:3 L:F mass ratio) was macerated in 300 mL distilled water for 1 week at 20°C. During maceration, the samples were mixed at 150 rpm in an orbital electrical shaker (Mumbai, Maharashtra, Hally Instruments) for 5 h. The extracts were filtered through sterile cotton and Whatman 1005–042 qualitative filter paper (2.5-μm pore size), stored in 330-mL bottles (28 mm neck diameter, Amber; Pharma Bottles & Containers), and refrigerated at 5 ± 1°C (Morsy Citation2010). In vitro, C. beticola compound sensitivity was determined by concentration gradation.
For each of the compounds listed in , five concentrations were prepared (Morsy Citation2005; Tomlin Citation2009). The required concentrations were obtained by adding the appropriate amounts of stock solutions to 100-mL of autoclaved PDA cooled to 45°C. Five 9 cm-diameter Petri dishes were prepared as replicates for each treatment. After the medium solidified, each dish was centrally inoculated with a 0.5-cm mycelial disc punched from a 25-d old C. beticola culture. The dishes were incubated at 27 ± 2°C (Model BD 56, Germany), and colony diameters were measured (Morsy Citation2005; Trkulja et al. Citation2015). Linear growth (cm) was measured by taking the average of two perpendicular diameters. These measurements were used to determine EC50 and EC90. Percentage inhibition (I%) was calculated according to a previously described method (Topps and Wain Citation1957), as follows:
Where I% is the percentage inhibition, A the mean diameter of the control, and B the mean diameter of the treatment.
In vitro component mixture feasibility determination
The activities of azoxystrobin (AZO)/SA, carbendazim (CAR)/SA, EPO/SA, PRO/SA, and the aqueous extract of A. cina (WEA)/SA at IC50 + IC50 against C. beticola were assayed. The joint toxicity of each combination was evaluated according to previously reported methods (Mansour et al. Citation1966; Morsy Citation2005), and calculated as follows:
Where Obs. I% represents the observed inhibition percentage and Exp. I% is the expected inhibition percent. Results were classified as follows: ≥ +20: synergism. ≥ −20: antagonism. An intermediate value (−20 < x < +20) was considered to be additive.
In vivo efficacy of compounds against Cercospora beticola
In vivo experiments were conducted at the Sakha Agricultural Research Station during 2016/17 and 2017/18. Plots (16.5 m × 6 m) in the area were arranged based on a randomized complete block design with four replicates. Each plot consisted of 12 rows with 100 plants per row to minimize possible border effects or cross-contamination among treatments. The experimental plots were separated by 1 m. Initial antifungal compound applications were administered when the foliar CLS incidence reached 5%. Three applications were performed per treatment at 400 kPa and 10-d intervals with a Model T4 backpack sprayer (Bellspray Inc., Opelousas, LA, USA). For each experimental area, 3-L antifungal compound solutions and their mixtures were prepared at their IC50 concentrations () (Trkulja et al. Citation2015). Infection rates were assessed using a previously published rating scale, where disease severity (DS) was expressed as an average percentage of affected leaf area, which was calculated for each plot by averaging the severity estimates of each leaf (Verreet et al. Citation1996). DS was evaluated at 10-d and 15-d intervals by examining 100 leaves sampled from four central rows in the experimental plots. DS was expressed as the mean % of infected leaf area calculated for each plot by averaging severity estimates for each leaf. The disease incidence parameter AUDPC (area under the disease progress curve) was calculated for the assessment period according to a previously reported method (Wolf and Verreet Citation2002) and the following equation:
Observed fungicide efficacies were expressed in % and calculated as follows:
To estimate interactions between fungicides applied as a mixture, the observed and expected efficacies were compared as follows:
Where A and B are the control levels of the individual fungicides. If the ratio between the observed and expected mixture efficacy was < 0.5, the fungicides were considered mutually antagonistic. If the ratio was 0.5 ≤ x ≤ 1.5, the fungicide interaction was considered additive. If the ratio was > 1.5, fungicidal synergy was considered to be present (Gisi Citation1996).
Cercospora beticola sensitivity to AZO, CAR, EPO, WEA, SA, AZO/SA, EPO/SA, PRO/SA, and WEA/SA
The sensitivity of C. beticola to the compounds and mixtures listed in was evaluated by calculating the effective concentration of fungicide that inhibited C. beticola by 50% relative to the no fungicide control (EC50) (Weiland and Halloin Citation2001; Karaoglanidis and Bardas Citation2006). To determine the sensitivity distributions, isolates were categorized five EC50-based sensitivity categories as follows: S, sensitive (< 1 ppm); RS, reduced sensitivity (1–10 ppm); MI, moderately insensitive (10–50 ppm); I, insensitive (50–100 ppm); and R, resistant (> 100 ppm) (Karaoglanidis and Ioannidis Citation2010; Secor et al. Citation2010a). The resistance factor (RF) was calculated as the ratio of the mean population EC50 values for each of the seasons 2016–17 and 2017–18 to that of 2015 (Rosenzweig et al. Citation2020).
Yield and sugar measurements
Yield (t ha−1) and white sugar content were determined for a random subsample of 10 roots representing ~27–72% of the total harvest, depending on the root size. Average root weights were evaluated to calculate the average number of plants per unit area (70 000 ha−1) (Mahmoud et al. Citation2012). Roots were sent to the Delta Sugar Company laboratories (Kafrelsheikh Governorate, Egypt) for percent sugar content measurements. The refined white sugar yield per sample was determined using the automatic sugar polarity scale method (Le Docte Citation1927) previously described elsewhere (McGinnis Citation1971). The refined (white) sugar content was calculated by linking non-sugar K, Na, and α-amino-N (mEq 100 g−1 beetroot) as described previously (Harvey and Dutton Citation1993):
Where ZB is the corrected sugar content (% beet) and NBI the α-amino-N, determined using the polarimeter method (Halvorson et al. Citation1978). White sucrose mass per ton of fresh beet RWST (kg t−1) was calculated using a previously reported equation (Van Eerd et al. Citation2012). White sucrose per hectare (RWSH) was calculated as follows (Tedford et al. Citation2019):
Antioxidant enzyme activity
Catalase and peroxidase activities were determined by homogenizing 0.5 g fresh sugar beet leaves at 0–4°C in 3 mL of 50 mM TRIS buffer (pH 7.8) containing 1 mM EDTA-Na2 and 7.5% (w/v) polyvinylpyrrolidone (PVP). The homogenates were centrifuged (SIGMA 3–18 K Universal refrigerated table top centrifuge, Germany) at 17.709 g and 4°C for 20 min. Total soluble enzyme activity was measured in the supernatant spectrophotometrically, at 480 nm for peroxidase enzyme activity, and the 440-nm peak for catalase activity (T 80+ Model UV-VIS spectrophotometer, PG Instruments Ltd., Leicester, UK) (Hafez Citation2010). In vitro enzyme activity was measured in the sugar beet leaves within 72 h of the final fungicide applications. Catalase (CAT) activity was determined according to a previously reported method (Aebi Citation1984). Peroxidase (POX) activity was determined according to a procedure published earlier (Hammerschmidt et al. Citation1982).
Statistical analysis
Data are presented as means ± SD. The data listed in were subjected to analysis of variance (ANOVA) and compared by the Least Significant Difference (LSD) test. Differences were considered significant at P = 0.05 and highly significant at P = 0.01 (Snedecor and Cochran Citation1989). The data in Table and Fig. a–d were subjected to ANOVA, followed by Duncan’s multiple range test at P = 0.01. Data were processed in Computer Statistical Package (CoStat) v6.45. (Cohort Software, Birmingham, UK). IC50 and EC90 for the data in were calculated and analysed using LdP Line (http://embakr.tripod.com/ldpline/ldpline.htm), which was also used to execute Finney’s probit analyses (Abbott Citation1925; Finney Citation1971).
Table 2. Cercospora leaf spot disease severity in treatment and control plots of sugar beet and efficacies of the tested compounds in 2016/17 and 2017/18.
Fig. 1 Frequency distribution of azoxystrobin (AZO) sensitivity in Cercospora beticola isolates from field-grown sugar beet in Egypt; EC50 and EC90, 50% and 90% maximal effective concentration, respectively.
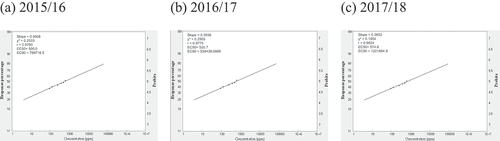
Fig. 2 Frequency distribution of carbendazim (CAR) sensitivity in Cercospora beticola isolates from field-grown sugar beet in Egypt; EC50 and EC90, 50% and 90% maximal effective concentration, respectively.
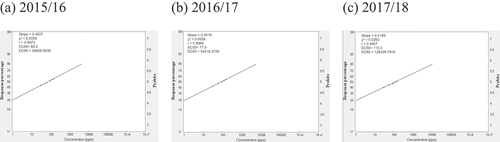
Fig. 3 Frequency distribution of epoxiconazole (EPO) sensitivity in Cercospora beticola isolates from field-grown sugar beet in Egypt; EC50 and EC90, 50% and 90% maximal effective concentration, respectively.
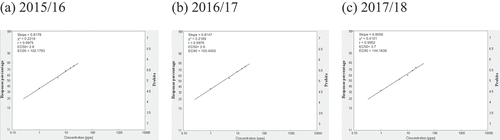
Fig. 4 Frequency distribution of propiconazole (PRO) sensitivity in Cercospora beticola isolates from field-grown sugar beet in Egypt; EC50 and EC90, 50% and 90% maximal effective concentration, respectively.
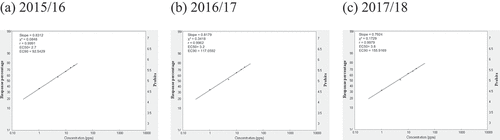
Results
Fungicide efficacy against CLS
The weather conditions were conducive for CLS development during both study seasons. Thresholds were reached by mid-November of each year. ANOVA revealed significant differences among treatment efficacies between seasons (F = 24.97; P < 0.01). There were no significant variations in disease severity for the MBC and QoI treatments in either season. The coefficients of variation were in the 0.8174–1.1402% range. The AUDPC disclosed that the disease incidences after AZO, SA, and CAR treatment were comparable to those in the untreated control (). The efficacies of AZO, SA, and CAR were in the ranges of 49.50–46.4%, 49.0–52.7%, and 47.50–45.1%, respectively. A post-hoc LSD test demonstrated pathogen reduction after treatment with DMI and aqueous extract of A. cina, applied separately or in combination (). AZO/SA, EPO/SA, PRO/SA, and WEA/SA displayed significantly high fungicidal efficacy in 2016/17, and at levels comparable to those of individual antifungal agents (E = 77.00–49.5%, 77.50–67.20%, 77.00–63.40%, and 76.00–69.00%, respectively). In 2016/17, AZO/SA exhibited synergistic efficacy relative to the standalone compounds (). In season 2, AZO/SA, EPO/SA, PRO/SA, and WEA/SA had anti-CLS efficacies higher than those of AZO, EPO, PRO, or WEA alone. EPO/SA, PRO/SA, AZO/SA, and WEA/SA exhibited the most optimal CLS control among all the treatments (79.10%, 78.20%, 78.20%, and 78.20%, respectively; ).
Temporal compound sensitivity and concentration distribution in C. beticola
Relative isolate concentration varied with compound and year (). The concentrations of the isolates with EC50 > 100 for AZO ranged from 500.0 ppm, 520.7 ppm, to 574.8 ppm in 2015/16, 2016/17, and 2017/18, respectively. The slopes were in the 0.4008–0.3852 range, and the EC90 values were in the 788 716.5–1 221 684.8 ppm range across all three seasons (). The concentrations of isolates with EC50 = 50–100 for CAR ranged from 66.2 ppm in 2015/16 to 77.9 ppm in 2016/17. However, the EC50 was 110.2 ppm in 2017/18. The slopes were in the 0.4627–0.4189 range, and the EC90 values were in the 38.929–126.33 ppm range across all three seasons (). For EPO, the concentrations of isolates with EC50 in the 1–5 ppm range varied from 2.8 ppm in 2015/16 and 2016/17 to 3.7 ppm in 2017/18. The slopes were in the 0.8178–0.8059 range, and the EC90 values were in the 102.2–144.2 ppm range (). The concentrations of isolates with EC50 values in the 1–5 range for PRO ranged from 2.7 ppm in 2015/16 to 3.2 ppm in 2016/17 to 3.8 ppm in 2017/18. The slopes were in the 0.8312–0.7924 range, and the EC90 values were in the 92.5–155.9 ppm range (). The concentrations of isolates with EC50 values in the 10–50 ppm range for WEA ranged from 23.0 ppm in 2015/16 to 22.0 ppm in 2016/17 to 22.5 ppm in 2017/18. The slopes were in the 0.7413–0.7418 range, and the EC90 values were in the 1232–1202 ppm range (). The concentrations of isolates with EC50 values in the 10–50 ppm range for SA ranged from 28.0 ppm in 2015/16 to 35.0 ppm in 2016/17 to 39.8 ppm in 2017/18. The slopes were in the 0.7449–0.7279 range, and the EC90 values were in the 1472–2295 ppm range ().
Temporal comparison of C. beticola RFs
RFs were calculated for C. beticola populations sensitive to AZO, CAR, EPO, PRO, WEA, and SA ()
Table 3. Comparison of resistance factors (RF) in Cercospora beticola populations isolated from beet leaves presenting Cercospora leaf spot symptoms in 2015/16 and 2017/18.
Table 4. In vitro fungicide mixture co-toxicity factors.
Table 5. Expected and observed fungicide mixture efficacies.
Catalase (CAT) and peroxidase (POX) activity
a and b show that compared with the untreated control, all fungicides and their combinations upregulated CAT at 72 h after application in 2016/17 and 2017/18. Compared with the control, WEA/SA, AZO/SA, EPO/SA, and PRO/SA induced higher CAT activity ([0.9880, 0.9870], [0.941, 0.940], [0.933, 0.9310], and [0.734, 0.733]) μmol min−1 mg−1 protein in 2016/17 and 2017/18, respectively). Relative to the control, WEA, SA, EPO, AZO, CAR, and PRO induced higher CAT activity ([0.741, 0.7400], [0.626, 0.6240], [0.536, 0.5270], [0.448, 0.445], [0.435, 0.422], and [0.375,0.3550] μmol min−1 mg−1 protein, respectively). The control CAT activity levels were 0.275 and 0.262 μmol min−1 mg−1 protein. a and b show that relative to the untreated control, all antifungal compounds and mixtures upregulated POX at 72 h after application in 2016/17 and 2017/18, respectively. Compared with the control, WEA/SA, AZO/SA, PRO/SA, and EPO/SA induced higher POX activity ([0.091, 0.090], [0.090, 0.089], [0.063, 0.061], and [0.058, 0.056] μmol min−1 mg−1 protein, respectively). Furthermore, relative to the control, SA, WEA, EPO, AZO, CAR, and PRO induced higher POX activity [0.084, 0.082], [0.054, 0.052], [0.044, 0.042], [0.041, 0.040], [0.041, 0.038], and [0.038, 0.036] μmol min−1 mg−1 protein, respectively). The untreated control POX activity levels were 0.036 and 0.031 μmol min−1 mg−1 protein in 2016/17 and 2017/18, respectively.
Fig. 7 Effects of different fungicide treatments on catalase (CAT) and peroxidase (POX) activity (μmol min−1 mg−1 protein) in 2016/17 and 2017/18. AZO, azoxystrobin; CAR, carbendazim; EPO, epoxiconazole; PRO, propiconazole; WEA, aqueous extract of Artemisia cina; SA, salicylic acid; AZO/SA, azoxystrobin/salicylic acid; EPO/SA, epoxiconazole/salicylic acid; PRO/SA, propiconazole/salicylic acid; WEA/SA, aqueous extract of Artemisia cina/salicylic acid. Bars represent the means of four replicates. Whiskers indicate standard deviation (SD). Different letters indicate significant difference among treatments according to Duncan’s multiple range test.
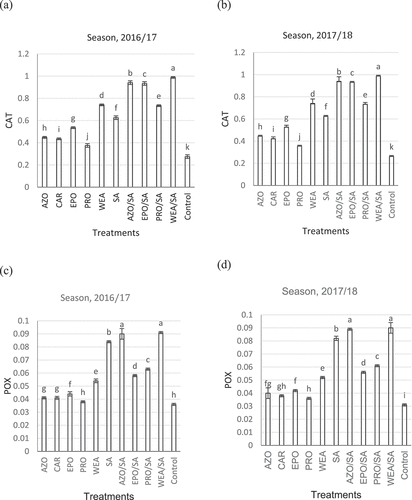
Influences of compounds applied in 2016/17 and 2017/18 on beet yield and sucrose content
Total root yield and sucrose % were influenced by all the treatments in 2016/17 and 2017/18 ()
Table 6. Yield and sucrose content of beet root harvested from plots treated with various compounds and mixtures to reduce Cercospora leaf spot in 2016/17 and 2017/18.
Discussion
Cercospora beticola isolates highly resistant to three classes of fungicides were isolated from CLS-affected sugar beet leaves collected at the commercial and research production areas of Sakha, Kafrelsheikh, Egypt, over two growing seasons. During the study period, fungicide sensitivity decreased progressively in the local C. beticola populations. Efficacious sugar beet CLS management is an integrated approach combining cultural practices, resistant cultivars, and fungicide application programmes comprising protectants and combinations of systemic fungicides (Karaoglanidis et al. Citation2001a). However, current control practices for sugar beet diseases do not effectively manage fungicide resistance. Antifungal compounds were categorized into five classes according to their relative impacts on C. beticola isolates. RF values were calculated for strains exhibiting a certain degree of tolerance to the tested fungicides (). In this study, shifts in C. beticola fungicide sensitivity were observed across the Sakha sugar beet production area between 2015 and 2017/18. From the time of the first sampling, EC50 increased for all evaluated compounds. Mean EC50 for AZO and CAR increased from 2015 to 2018. In the cases of DMI, EPO, and PRO, mean EC50 increased from 2015 to 2017 but decreased from 2017 to 2018. The resistance of C. beticola to DMI fungicides has previously been reported. In addition, MBC and QoI resistance has been reported in C. beticola in Europe (Karaoglanidis et al. Citation2000) and the U.S. (Secor et al. Citation2010a, Citation2010b). The sensitivity of various C. beticola isolates to the DMI fungicides EPO and PRO, WEA, and SA decreased from 2015 to 2018. Cercospora beticola resistance to QoI (AZO) and MBC (CAR) increased from 2015 to 2017 and further still in 2017/18. Overall, the progressive increases in fungicide sensitivity variability were reflected by increases in C. beticola resistant phenotype concentrations with respect to all the compounds analysed (Rosenzweig et al. Citation2020).
The onset of fungicide resistance in the field necessitates the introduction and adoption of novel management strategies. The application of a systemic fungicide combined with a plant immunostimulant, such as SA or other agents with a different mode of action, has been proposed as a strategy for delaying the onset of resistant isolates (Edgington et al. Citation1980). To achieve this, DMIs are often combined with protective fungicides such as maneb. Nevertheless, such strategies eventually fail to prevent an increase in the concentration of resistant populations within fungal species such as C. beticola (Karaoglanidis et al. Citation2001b). A mixture of DMI and SA for the control of C. beticola could be an efficacious alternative protocol for managing resistant pathogen populations (Mikaberidze et al. Citation2014). In the absence of fitness costs, the application of high-risk fungicide mixtures drives the emergence of resistance, causing the agents to become ineffective over time. However, in the presence of a resistance cost, a mixture with an optimized fungicide ratio can be applied without causing PR. That way, disease control can be maximized. Here, protective fungicides had limited efficacy in suppressing CLS in regions conducive for pathogen proliferation. Nevertheless, the results of the field trials conducted at Sakha suggest that DMI/SA and WEA/SA offered the highest levels of sugar beet crop protection.
A previous study (Luo and Schnabel Citation2008) suggested that selection for DMI resistance could simultaneously increase QoI resistance. Monitoring fungicide sensitivity in Monilinia fructicola showed that rotations of DMI, QoI, and MBC did not lower the concentrations of populations resistant to either fungicide type (Schnabel et al. Citation2012). The occurrence of C. beticola populations resistant to MBC and the presence of substantial variation in DMI resistance show that continuous and extensive fungicide resistance monitoring programmes are required in regions with variable selection pressures. Accurate data on the presence and prevalence of resistant populations would facilitate the design and implementation of optimal antifungal treatment protocols. In addition, such protocols may be adjusted to improve fungicidal efficacy by combining products with host plant immunostimulants, such as SA or plant extracts. The efficacies of such novel disease control could be influenced by the antifungal modes of action of the new fungicide classes, plant extracts, immunostimulants, and antifungal combinations adopted (Skaracis et al. Citation2010; Bolton et al. Citation2012). WEA exhibited good anti-CLS efficacy both as a single treatment and in combination with other agents. Earlier reports have indicated that aqueous extracts of A. cina have promising applications in plant disease management (Derbalah et al. Citation2012; Omara et al. Citation2015). The use of broad-spectrum aqueous plant extracts can inhibit the entire pathogen complex (Wilson Citation2000). Such extracts are also recognized sources of drugs (Chopra Citation1933). The antifungal action of aqueous A. cina extract may be explained by its capacity to inhibit spore germination (Morsy Citation2010). WEA can be used alone and in mixtures to control CLS.
In this study, all mixtures containing SA upregulated CAT and POX activity. Such responses could be attributed to SA enhancing the fungicidal efficacy of certain single compounds. SA induces host resistance and provides partial protection against C. beticola. SAR induced by SA can control CLS in susceptible varieties by 70–91% and has a degree of efficacy comparable to those of fungicides commonly used on more resistant fungal pathogens (Jacobsen et al. Citation2004). Host plant disease resistance is associated with the production of SA-associated pathogen resistance (PR) proteins, including peroxidase, 13-glucanase, and chitinase. Therefore, we hypothesize that the underlying signalling pathway is SA-dependent. The optimal CLS control strategy overall might entail a combination of natural host resistance and reduced chemical fungicide application rates (Jacobsen et al. Citation2004).
Relative differences in disease severity could have also contributed to the observed differences in yield among treatments. The effects of CLS on root yield, sugar yield and quality, and profit margin may depend on reaching a time-sensitive disease threshold prior to harvest. However, early discontinuation of fungicides could increase the amount of overwintering inoculum and, by extension, the risk of disease in the following year. However, such a management practice does not consider the adverse effects of CLS on post-harvest beet storability (Jacobsen et al. Citation2009). Although QoI and MBC applications tended to reduce disease severity, they had no impact on beet yield or sugar percentage, as C. beticola resistance was very high. Only the CAR/SA combination was antagonistic according to the CTF% (−67.1) (). All other fungicide mixtures improved relative root yield and RWS over two seasons (Tedford et al. Citation2019). Here, fungicide mixtures with SA resulted in a CTF% in the −7.0 to −17.3 range. Therefore, SA stably and consistently enhanced C. beticola sensitivity to the single fungicides, and the interactions between agents were additive. Such mixtures attenuated C. beticola fungicide resistance as they simultaneously affected multiple target sites in the pathogen, while stimulating host plant immunity against the fungus. The recent discovery of DMI-resistant C. beticola isolates (Trueman et al. Citation2017) highlights that the development of CLS management protocols and strategies should be sustained. In a sustainable CLS management programme, growers must practice crop rotation and tillage to control overwintering inoculum, disease-resistant cultivars must be developed, and the mechanisms of fungicide resistance must be elucidated. This study developed and tested a combined strategy for the safe and efficacious control of C. beticola isolates resistant to QoI, MBC, and DMI.
Based on the results of this study, the use of QoI, MBC, and DMI to control CLS in Egypt has become ineffective in part because of the emergence of resistant fungal pathogen isolates. This study monitored the occurrence and amounts of C. beticola isolates resistant to QoI, MBC, and DMI and demonstrated that the application of mixtures of fungicides with different modes of action could address fungal PR. Moreover, fungal QoI and DMI resistance could be mitigated by combining such agents with the host plant immunostimulant, SA. Such combinations could boost antifungal treatment efficacy, help reduce the quantities of fungicides required for effective disease management, mitigate soil, water and air pollution, decrease operating costs, improve profitability, and facilitate food security.
In conclusion, this study monitored the rates of C. beticola resistance to fungicides, which changed slowly for DMI fungicides and relatively rapidly for MBC and QoI. The results of this study could be applied to enhance the efficiency of fungicides against C. beticola and crop productivity. They also offer a potential solution for addressing resistance, in the form of fungicide mixtures at their EC50 concentrations with compounds inducing host resistance. Such a strategy that uses chemical strategies to control host resistance could reduce fungicide use, and in turn environmental contamination, in addition to the costs of production. Employing alternatives that are safer for the environment and agricultural workers has been shown to provide these benefits while maintaining good yield and crop quality. Therefore, we recommend the use of such straightforward and inexpensive alternatives within CLS control programmes.
The results of this study also present a strategy for monitoring and predicting the resistance of various pathogens of other crops. We hope that our results will encourage the adoption of novel pesticide development and manufacturing strategies, involving the use of natural productions to produce combination treatments for economic crops, which incorporate both chemical control and compounds inducing host resistance, and which would reduce the amounts of polluting chemical pesticides used by farmers. We suggest further studies investigating the feasibility and toxicology of combining chemical control with host resistance inducers, and the determination of the environmental effects of their residues on natural crop pests and diseases. Finally, the food quality of treated crops should be evaluated, considering they may have therapeutic effects. For example, WEA and WEA/SA have potential applications in the management of some human diseases.
Acknowledgements
The authors thank the Environmental Remediation of Agricultural Pollutants Laboratory (ERAP) for technical and financial support and Dr. Khaled A. Aboshady, Director of Agricultural Experiments Regional Research for North Delta, Sakha, for allocating the research farm in which this work was conducted.
Disclosure statement
No potential conflict of interest was reported by the author(s).
References
- Abad MJ, Bedoya LM, Apaza L, Bermejo P. 2012. The Artemisia L. genus: a review of bioactive essential oils. Molecules. 17(3):2542–2566. doi:https://doi.org/10.3390/molecules17032542.
- Abbott WS. 1925. A method of computing the effectiveness of an insecticide. J Econ Entomol. 18(2):265–267. doi:https://doi.org/10.1093/jee/18.2.265a.
- Aebi H. 1984. [13] Catalase in vitro. Methods in enzymology. . ; p. 121–126, https://doi.org/https://doi.org/10.1016/S0076-6879(84)05016-3
- Ahmadi F, Sadeghi S, Modarresi M, Abiri R, Mikaeli A. 2010. Chemical composition, in vitro anti-microbial, antifungal and antioxidant activities of the essential oil and methanolic extract of Hymenocrater longiflorus Benth of Iran. Food Chemical Toxicol. 48(5):1137–1144. doi:https://doi.org/10.1016/j.fct.2010.01.028.
- Balbaa S, Hilal S, Zaki A. 1976. Medicinal plant constituents. Vol. 2, General Organization for University and School Books; p. 108–109.
- Bartlett DW, Clough JM, Godwin JR, Hall AA, Hamer M, Parr‐Dobrzanski B. 2002. The strobilurin fungicides. Pest Manag Sci. 58(7):649–662. doi:https://doi.org/10.1002/ps.520.
- Bolton MD, Rivera V, Secor G. 2013b. Identification of the G143A mutation associated with QoI resistance in Cercospora beticola field isolates from Michigan, United States. Pest Manag Sci. 69(1):35–39. doi:https://doi.org/10.1002/ps.3358.
- Bolton MD, Rivera-Varas V, Del Rio Mendoza LE, Khan MFR, Secor GA. 2012. Efficacy of variable tetraconazole rates against Cercospora beticola isolates with differing in vitro sensitivities to DMI fungicides. Plant Dis. 96(12):1749–1756. doi:https://doi.org/10.1094/PDIS-03-12-0255-RE.
- Bolton MD, Rivera-Varas V, Secor GA, Cattanach AW, Metzger MS. 2013a. Identification of the G143A mutation in cytochrome b associated with QoI resistance in Cercospora beticola isolates from the Red River Valley. Plant Health Prog. 14(1):14. doi:https://doi.org/10.1094/PHP-2013-0812-02-RS.
- Chahal I, Vyn RJ, Mayers D, Van Eerd LL. 2020. Cumulative impact of cover crops on soil carbon sequestration and profitability in a temperate humid climate. Sci Rep. 10(1):1–11. doi:https://doi.org/10.1038/s41598-020-70224-6.
- Chopra RN. 1933. Indigenous drugs of India. Their medical and economic aspects. Indigenous Drugs of India. Their Medical and Economic Aspects.
- Derbalah A, Kamel S, Morsy S, El-Sawy M. 2012. Alternatives to control powdery mildew and early blight diseases of tomato under greenhouse conditions. Egypt J Biol Pest Control. 22(2):185.
- Derbalah AS, Keratrum AY, El-Dewy ME, El-Shamy EH. 2013. Efficacy of some insecticides and plant extracts against Tetranychus urticae under laboratory conditions. Egypt J Plant Prot Res. 1(3):47–69.
- Dhingra OD, and Sinclair JB. 1995. Basic plant pathology methods ,CRC press, p. 287–305 doi:https://doi.org/10.1201/9781315138138.
- Edgington LV, Martin RA, Bruin GC, Parsons IM. 1980. Systemic fungicides: a perspective after 10 years. Plant Dis. 64(1):19–23. doi:https://doi.org/10.1094/PD-64-19.
- El-Sayed A. 2000. Integrated control of fungal leaf spots on sugar beet [M. Sc. Thesis], Egypt: Plant Pathology Department Faculty of Agriculture Menoufia University.
- Finney DJ. 1971. Probit analysis. Cambridge (UK): Cambridge University Press.
- Franc GD. 2010. Ecology and epidemiology of Cercospora beticola. In: Cercospora leaf spot of sugar beet and related species; p. 7–19.
- Georgopoulos SG, Dovas C. 1973. Occurrence of Cercospora beticola strains resistant to benzimidazole fungicides in N. Greece. Plant Disease Rep. 57:321–324.
- Gisi U. 1996. Synergistic interaction of fungicides in mixtures. Phytopathology. 86(11):1273–1279.
- Hafez Y. 2010. Control of Botrytis cinerea by the resistance inducers benzothiadiazole (BTH) and hydrogen peroxide on white pepper fruits under postharvest storage. Acta Phytopathologica et Entomologica Hungarica. 45(1):13–29. doi:https://doi.org/10.1556/APhyt.45.2010.1.2.
- Halvorson AD, Hartman GP, Cole DF, Haby VA, Baldridge DE. 1978. Effect of N fertilization on sugarbeet crown tissue production and processing quality 1. Agron J. 70(5):876–880. doi:https://doi.org/10.2134/agronj1978.00021962007000050040x.
- Hammerschmidt R, Nuckles E, Kuć J. 1982. Association of enhanced peroxidase activity with induced systemic resistance of cucumber to Colletotrichum lagenarium. Physiol Plant Pathol. 20(1):73–82. doi:https://doi.org/10.1016/0048-4059(82)90025-X.
- Harvey CW, and Dutton JV. 1993. Root quality and processing. In: The sugar beet crop. Springer; p. 571–617, https://link.springer.com/chapter/10.1007/978-94-009-0373-9_15.
- Holtschulte B. 2000. Cercospora beticola–worldwide distribution and incidence. Cercospora beticola; p. 5–16.
- Holtschulte B, Mechelke W, Stahl DJ. 2010. Conventional and novel approaches in breeding for resistance to Cercospora beticola in sugar beet. In: Lartey RT, Weiland JJ, Panella L, Crous PW, Windels CE, editors. Cercospora leaf spot of sugar beet and related species; p. 129–139.
- Ioannidis PM, Karaoglanidis GS, Lartey RT. 2010. Control of Cercospora leaf spot and powdery mildew of sugar beet with fungicides and tolerant cultivars. In: Cercospora leaf spot of sugar beet and related species. St. Paul (MN): APS Press; p. 259–274.
- Jacobsen BJ, Franc GD, Harveson RM, Hanson LE, Hein GL. 2009. Foliar disease casused by fungi and Oomycetes. In: Harveson RM, Hanson LE, Hein GL, editors. Compendium of beet diseases and pests, 2nd ed. The American Phytopathological Society. Cercospora leaf spot; p. 7–10.
- Jacobsen BJ, Zidack NK, Larson BJ. 2004. The role of Bacillus-based biological control agents in integrated pest management systems: plant diseases. Phytopathology. 94(11):1272–1275. doi:https://doi.org/10.1094/PHYTO.2004.94.11.1272.
- Kaiser U, Kluth C, Märländer B. 2010. Variety‐specific epidemiology of Cercospora beticola Sacc. and consequences for threshold‐based timing of fungicide application in sugar beet. J Phytopathol. 158(4):296–306. doi:https://doi.org/10.1111/j.1439-0434.2009.01618.x.
- Karaoglanidis GS, Bardas G. 2006. Control of benzimidazole- and DMI-resistant strains of Cercospora beticola with strobilurin fungicides. Plant Dis. 90(4):419–424. doi:https://doi.org/10.1094/PD-90-0419.
- Karaoglanidis GS, Ioannidis PM. 2010. Fungicide resistance of Cercospora beticola in Europe. Cercospora leaf spot of sugar Beet and related species. St. Paul: American Phytopathological Society.
- Karaoglanidis GS, Ioannidis PM, Thanassoulopoulos CC. 2000. Reduced sensitivity of Cercospora beticola isolates to sterol‐demethylation‐inhibiting fungicides. Plant Pathol. 49(5):567–572. doi:https://doi.org/10.1046/j.1365-3059.2000.00488.x.
- Karaoglanidis GS, Ioannidis PM, Thanassoulopoulos CC. 2001a. Influence of fungicide spray schedules on the sensitivity of Cercospora beticola to the sterol demethylation-inhibiting fungicide flutriafol. Crop Prot. 20(10):941–947. doi:https://doi.org/10.1016/S0261-2194(01)00049-7.
- Karaoglanidis GS, Thanassoulopoulos CC. 2003. Cross-resistance patterns among sterol biosynthesis inhibiting fungicides (SBIs) in Cercospora beticola. Eur J Plant Pathol. 109(9):929–934. doi:https://doi.org/10.1023/B:EJPP.0000003672.36076.8a.
- Karaoglanidis GS, Thanassoulopoulos CC, Ioannidis PM. 2001b. Fitness of Cercospora beticola field isolates - resistant and - sensitive to demethylation inhibitor fungicides. Eur J Plant Pathol. 107:337–347. doi:https://doi.org/10.1023/A:1011219514343.
- Khan J, Del Río LE, Nelson R, Khan MFR. 2007. Improving the Cercospora leaf spot management model for sugar beet in Minnesota and North Dakota. Plant Dis. 91(9):1105–1108. doi:https://doi.org/10.1094/PDIS-91-9-1105.
- Khan MFR, Carlson AL. 2009. Effect of fungicides on sugar beet yield, quality, and postharvest respiration rates in the absence of disease. Plant Health Prog. 10(1):5. doi:https://doi.org/10.1094/php-2009-1019-01-rs.
- Le Docte A. 1927. Commercial determination of sugar in the beet root using the Sacks Le-Docte process. Int Sug J. 29:488–492.
- Luo C-X, Schnabel G. 2008. Adaptation to fungicides in Monilinia fructicola isolates with different fungicide resistance phenotypes. Phytopathology. 98(2):230–238. doi:https://doi.org/10.1094/PHYTO-98-2-0230.
- Mahmoud EA, Hassanin MA, Emara EIR. 2012. Effect of organic and mineral nitrogenous fertilizers and plant density on yield and quality of sugar beet (Beta vulgaris L.). Egypt J Agron. 34(1):89–103.
- Mansour N, Eldefrawi M, Toppozada Azeid M. 1966. Toxicological studies on the Egyptian cotton leaf worm, Prodenia litura. VI. Potentiation and antagonism of organophosphorus and carbamate insecticides. J Econ Entomol. 59(2):307–311. doi:https://doi.org/10.1093/jee/59.2.307.
- Marić A, Petrov M, Maširević S. 1976. Pojava tolerantnosti kod Cercospora beticola Sacc. prema benomilu u Jugoslaviji i mogućnosti suzbijanja ovog parazita. Institut za zaštitu bilja.
- McGinnis RA. 1971. Juice purification. In: McGinnis RA, editor. Beet sugar technology. Fort Collins (CO): Beet Sugar Development Foundation; p. 87–103.
- Mikaberidze A, McDonald BA, Bonhoeffer S. 2014. Can high-risk fungicides be used in mixtures without selecting for fungicide resistance? Phytopathology. 104(4):324–331. doi:https://doi.org/10.1094/PHYTO-07-13-0204-R.
- Morsy SZA. 2010. Studies on fungicidal activity of some fungicides, plant extracts and bioagents against both powdery and downy mildew diseases on cucumber and squash (Doctoral dissertation, Ph. D., Thesis Fac. of Agric. Kafrelsheikh Univ., Egypt).
- Morsy SZ-AE-A. 2005. Studies on fungicidal activity of some fungicides and plant extracts against some plant pathogens; p. 100.
- Omara R, Kamel S, Hafez Y, Morsy S. 2015. Role of non-traditional control treatments in inducing rtesistance against wheat leaf rust caused by Puccinia triticina. Egypt J Biol Pest Control. 25(3):609–618.
- Percich JA, Nickelson LJ, Huot CM. 1987. Field evaluation of various fungicides to control cercospora leaf spot of sugarbeet, caused by benomyl-resistant strains of Cercospora beticola. J Am Soc Sugar Beet Technol. 24(1):32–40. doi:https://doi.org/10.5274/jsbr.24.1.32.
- Ragasa CY, Hofilena JG, Rideout JA. 2002. New furanoid diterpenes from Caesalpinia pulcherrima. J Nat Prod. 65(8):1107–1110. doi:https://doi.org/10.1021/np0201523.
- Rosenzweig N, Hanson LE, Clark G, Franc GD, Stump WL, Jiang QW, Stewart J, Kirk WW. 2015. Use of PCR-RFLP analysis to monitor fungicide resistance in Cercospora beticola populations from sugarbeet (Beta vulgaris) in Michigan, United States. Plant Dis. 99(3):355–362. doi:https://doi.org/10.1094/PDIS-03-14-0241-RE.
- Rosenzweig N, Hanson LE, Mambetova S, Jiang Q, Guza C, Stewart J, Trueman CL, Somohano P. 2020. Temporal population monitoring of fungicide sensitivity in Cercospora beticola from sugarbeet (Beta vulgaris) in the Upper Great Lakes. Can J Plant Pathol. 42(4):469–479. doi:https://doi.org/10.1080/07060661.2019.1705914.
- Saccardo PA. 1876. Conspectus generum pyrexomycetum italicorum 11. Nuovo giornale botanico italiano memorie della Società botanica italiana, p. 11.
- Sauter H, Steglich W, Anke T. 1999. Strobilurins: evolution of a new class of active substances. Angewandte Chemie Int Ed. 38(10):1328–1349. doi:https://doi.org/10.1002/(SICI)1521-3773(19990517)38:10<1328::AID-ANIE1328>3.0.CO;2-1.
- Schnabel G, Amiri A, and Brannen PM. 2012. 10 Field kit-and internet-supported fungicide resistance monitoring. Fungicide Resistance in Crop Protection: Risk and Management. 116.
- Secor GA, Rivera VV, Khan M, Gudmestad NC. 2010b. Monitoring fungicide sensitivity of Cercospora beticola of sugar beet for disease management decisions. Plant Dis. 94(11):1272–1282. doi:https://doi.org/10.1094/PDIS-07-09-0471.
- Secor GA, Rivera-Varas VV, Gudmestad NC, Weiland JJ. 2010a. Sensitivity of Cercospora beticola to foliar fungicides in the Red River Valley of North Dakota and Minnesota. Cercospora leaf spot of sugar beet and related species/Robert T. Lartey … [et al.].
- Shane W, Teng P. 1992. Impact of Cercospora leaf spot on root weight, sugar yield, and purity of Beta vulgaris. Plant Dis. 76(8):812–820. doi:https://doi.org/10.1094/PD-76-0812.
- Sisler HD. 1988. Fungicidal action and fungal resistance mechanisms.
- Skaracis GN, Pavli OI, Biancardi E. 2010. Cercospora leaf spot disease of sugar beet. Sugar Tech. 12(3–4):220–228. doi:https://doi.org/10.1007/s12355-010-0055-z.
- Snedecor GW, Cochran WG. 1989. Statistical methods. Vol. 54, 8th ed. Ames: Iowa State University Press Iowa; p. 71–82
- Tedford SL, Burlakoti RR, Schaafsma AW, Trueman CL. 2019. Optimizing management of cercospora leaf spot (Cercospora beticola) of sugarbeet in the wake of fungicide resistance. Can J Plant Pathol. 41(1):35–46. doi:https://doi.org/10.1080/07060661.2018.1561518.
- Thomas A, Langston DB Jr, Stevenson KL. 2012. Baseline sensitivity and cross-resistance to succinate-dehydrogenase-inhibiting and demethylation-inhibiting fungicides in Didymella bryoniae. Plant Dis. 96(7):979–984. doi:https://doi.org/10.1094/PDIS-09-11-0744-RE.
- Tomlin CD. 2009. The pesticide manual: a world compendium. British Crop Production Council.
- Topps JH, Wain RL. 1957. Investigations on fungicides. III. The fungitoxicity of 3‐and 5‐alkyl‐salicylanilides and para‐chloroanilides. Ann Appl Biol. 45(3):506–511. doi:https://doi.org/10.1111/j.1744-7348.1957.tb05888.x.
- Trkulja N, Aleksić G, Starović M, Dolovac N, Ivanović Žživković S. 2009. Osetljivost izolata Cercospora beticola prema karbendazimu i flutriafolu u Srbiji. Zaštita bilja. 270:237–245.
- Trkulja N, Milosavljević A, Stanisavljević R, Mitrović M, Jović J, Toševski I, Bošković J. 2015. Occurrence of Cercospora beticola populations resistant to benzimidazoles and demethylation-inhibiting fungicides in Serbia and their impact on disease management. Crop Prot. 75:80–87. doi:https://doi.org/10.1016/j.cropro.2015.05.017.
- Trueman C, and Burlakoti RR. 2015. Evaluation of products for management of Cercospora leaf spot in sugar beet, 2014. Plant Dis Manage Rep.
- Trueman CL, Hanson LE, Rosenzweig N, Jiang QW, Kirk WW. 2013. First report of QoI insensitive Cercospora beticola on sugar beet in Ontario, Canada. Plant Dis. 97(9):1255. doi:https://doi.org/10.1094/PDIS-03-13-0285-PDN.
- Trueman CL, Hanson LE, Somohano P, Rosenzweig N. 2017. First report of DMI-insensitive Cercospora beticola on sugar beet in Ontario, Canada. New Disease Reports. 36(20):2044–2588. doi:https://doi.org/10.5197/j.2044-0588.2017.036.020.
- Van Eerd LL, Congreves KA, Zandstra JW. 2012. Sugar beet (Beta vulgaris L.) storage quality in large outdoor piles is impacted by pile management but not by nitrogen fertilizer or cultivar. Can J Plant Sci. 92(1):129–139. doi:https://doi.org/10.4141/cjps2011-054.
- Verreet JA, Wolf P, Weis FJ. 1996. Bekämpfungsschwellen als Grundlage für eine integrierte Bekämpfung von Cercospora beticola–Das IPS-Modell Zuckerrübe. Proc IIRB. 59:55–69.
- Weiland JJ, Halloin JM. 2001. Benzimidazole resistance in Cercospora beticola sampled from sugarbeet fields in Michigan, USA. Can J Plant Pathol. 23(1):78–82. doi:https://doi.org/10.1080/07060660109506912.
- Wilson JP. 2000. Pearl millet diseases: a compilation of information on the known pathogens of Pearl Millet: Pennisetum Glaucum (L.) R. Br: US Department of Agriculture, Agricultural Research Service.
- Wolf PFJ, Verreet JA. 2002. An integrated pest management system in Germany for the control of fungal leaf diseases in sugar beet: the IPM sugar beet model. Plant Dis. 86(4):336–344. doi:https://doi.org/10.1094/PDIS.2002.86.4.336.
- Yuzo Y, Yuri A. 1987. Interaction of azole antifungal agents with cytochrome P-45014DM purified from Saccharomyces cerevisiae microsomes. Biochem Pharmacol. 36(2):229–235. doi:https://doi.org/10.1016/0006-2952(87)90694-0.