Abstract
Fusarium graminearum is a mycotoxin-producing pathogen involved in Fusarium head blight (FHB) disease of cereals, and is responsible for compromising grain yield and quality.Over the course of infection, the fungus secretes various metabolites and proteins, of which only a handful have been fully characterized in the context of FHB. We report herein the role of a secreted cerato-platanin protein (FGSG_10212, FgCPP1) in the growth and aggressiveness of F. graminearum. Pathogen strains in which FgCPP1 was disrupted (Δfgcpp1) or overexpressed (FgCPP1-OX1) did not differ from the wild-type with respect to macroconidial germination or mycelial growth, nor in sensitivity to chemicals targeting fungal cell walls. Host—plant interaction studies were conducted including disease assays in wheat and host-cell localization of FgCPP1 in tobacco cells. Consistent with previous reports for FgCPP1 disruption mutants of F. graminearum, no difference was observed among FgCPP1-OX1, Δfgcpp1 and the wild-type in their ability to cause disease spread in point-inoculated wheat spikes. Spray inoculation of the wheat spikes with FgCPP1-OX1 resulted in an increase in diseased spikelets as early as 3 days post-inoculation in each of the three wheat genotypes assessed; however, this increase was not observed when the disease assay was repeated in the cultivar ‘Penhold’ with an additional FgCPP1-OX transformant, FgCPP1-OX2. FgCPP1-OX1 also resulted in increased levels of deoxynivalenol accumulation in axenic culture, as well as in spray-inoculated wheat spikes. We propose that FgCPP1 is a minor contributor to pathogen aggressiveness in wheat.
Résumé
Fusarium graminearum est un agent pathogène qui produit une mycotoxine intervenant dans la brûlure de l’épi (BEF) chez les céréales et qui compromet le rendement et la qualité des grains. Au cours de l’infection, le champignon sécrète différents métabolites et protéines parmi lesquels seulement quelques-uns ont été entièrement caractérisés dans le contexte de la BEF. Nous relatons ici le rôle d’une protéine sécrétée de la famille des cérato-platanines (FGSG_10212, FgCPP1) joué dans la croissance et l’agressivité de F. graminearum. Les souches d’agents pathogènes dans lesquelles FgCPP1 a été dissociée (Δfgcpp1) ou surexprimée (FgCPP1-OX1) ne différaient pas des types sauvages quant à la germination des macroconidies ou à la croissance du mycélium, ni à la sensibilité aux produits de synthèse qui ciblent les parois cellulaires des champignons. Des études sur les interactions des plantes hôtes ont été menées, y compris des biotests sur les maladies chez le blé et la localization des cellules hôtes de FgCPP1 dans des cellules de tabac. Conformément aux rapports antérieurs sur les mutants issus de la dissociation de FgCPP1 de F. graminearum, aucune différence n’a été observée parmi FgCPP1-OX1, Δfgcpp1 et les types sauvages quant à leur capacité à disséminer la maladie chez des épillets de blé inoculés ponctuellement. L’inoculation par aspersion des épillets de blé avec FgCPP1-OX1 a engendré un accroissement du nombre d’épillets infectés dès le troisième jour après l’inoculation chez chacun des trois génotypes de blé évalués. Toutefois, cet accroissement n’a pas été observé lorsque le biotest a été reproduit sur le cultivar ‘Penhold’ avec un transformant supplémentaire de FgCPP1-OX, FgCPP1-OX2. FgCPP1-OX1 a également engendré un accroissement des taux d’accumulation de désoxynivalénol dans une culture axénique de même que dans les épis de blé inoculés par aspersion. Nous proposons donc que FgCPP1 joue un rôle de contributeur marginal dans l’agressivité de l’agent pathogène chez le blé.
Introduction
Fusarium graminearum Schwabe is a fungal pathogen of cereals involved in Fusarium head blight (FHB) disease of wheat (Triticum aestivum L.) and barley (Hordeum vulgare L). The fungus secretes a suite of secondary metabolites including mycotoxins that contaminate the grain, leading to significant economic losses for farmers around the world (Goswami and Kistler Citation2004) and posing health risks for human and animal consumers (Rocha et al. Citation2005; Pestka Citation2010; Pinton et al. Citation2010; Sobrova et al. Citation2010). The trichothecene mycotoxin deoxynivalenol (DON) is the most common contaminant in wheat heads (Foroud et al. Citation2019a), and its secretion is known to contribute to the pathogen aggressiveness (Proctor et al. Citation1995; Bai et al. Citation2002).
In addition to the secretion of secondary metabolites, fungi also secrete small proteins involved in fungal—plant interactions (Kim et al. Citation2016) and some of these contribute to disease development while others can activate plant defence responses (Rep Citation2005). The F. graminearum secretome includes several hundred proteins, many of which are potential participants in pathogen aggressiveness (Paper et al. Citation2007; Rampitsch et al. Citation2013). The role of the vast majority of these secreted proteins are undefined in FHB pathogenesis, and only a couple have been clearly identified as factors of aggressiveness in this disease. In 2005, Voigt et al. described a F. graminearum secreted lipase as a virulence factor, and demonstrated that when the encoding gene is disrupted the fungus has a reduced ability to cause disease spread in wheat, despite having an increase in DON production (Voigt et al. Citation2005, Citation2007). More recently, another secreted protein, orphan protein Osp24, was shown to be important for infectious growth of F. graminearum within the wheat rachis (Jiang et al. Citation2020).
The F. graminearum secretome includes two small cysteine-rich non-enzymatic proteins called cerato-platanin proteins (CPPs), encoded by FgCPP1 (FGSG_10212) and FgCPP2 (FGSG_11205) (Paper et al. Citation2007; Rampitsch et al. Citation2013; Lu and Edwards Citation2016). In addition to FgCPP1 and FgCPP2, three putative F. graminearum CPPs (FGSG_04471, FGSG_03971 and FGSG_17103) have also been reported; however, the encoded proteins share lowsequence identity (<10%) with FgCPP1 and FgCPP2 (Quarantin et al. Citation2016). Furthermore, they deviate from other fungal CPPs in protein length and number of cysteine residues, and they lack the presence of two peptide motifs (Quarantin et al. Citation2016) responsible for necrosis-inducing activity (Frías et al. Citation2014). Fungal CPPs possess a single domain, which forms a double φβ-barrel fold reminiscent of the D1 domain of expansin proteins (de Oliveira et al. Citation2011; Barsottini et al. Citation2013) and some CPPs exhibit cellulose/cell wall weakening ability similar to expansins (Barsottini et al. Citation2013; Baccelli et al. Citation2014; Luti et al. Citation2017; Quarantin et al. Citation2019). Expansins have been implicated in various physiological and developmental processes in plants owing to their ability to mediate cell wall extension (Sampedro and Cosgrove Citation2005; Yennawar et al. Citation2006), and expansin-like proteins with cellulose loosening abilities have been reported in both fungi and bacteria (Chen et al. Citation2010; Georgelis et al. Citation2011). Some fungal CPPs can bind chitin in vitro (Barsottini et al. Citation2013; Frischmann et al. Citation2013; Baccelli et al. Citation2014) and fungal cell wall localization has been reported for two CPPs (Boddi et al. Citation2004; Frías et al. Citation2014).
Quarantin et al. (Citation2019) observed expansin like-activity for both of the F. graminearum CPPs, FgCPP1 and FgCPP2, where recombinant proteins enhanced the cellulase activity of a fungal β-1,4-glucanase on various cellulosic substrates, but unlike other fungal CPPs, were found to bind cellulose and not chitin. Both of these proteins are secreted during interaction with the wheat spike (Paper et al. Citation2007), although Rampitsch et al. (Citation2013) observed a reduced abundance of FgCPP1 in the secretome of a non-pathogenic TRI6 (transcriptional regulator of trichothecene biosynthesis; Nasmith et al. Citation2011) deletion mutant of the F. graminearum GZ3639 strain under DON-inducing conditions. Further to this, expression of the FgCPP1 gene is significantly upregulated in F. graminearum strain 8/1 during the early stages of wheat spike infection (Quarantin et al. Citation2016). This finding, together with the results of Quarantin et al. (Citation2016, Citation2019), suggest a possible role for FgCPP1 in host plant infection. Disruption mutants of genes encoding these two CPPs were characterized by Quarantin et al. (Citation2016), where both single (Δfgcpp1) and double (ΔΔfgcpp1,2) knockout mutants were investigated in wild-type (WT) strain 8/1. Neither the single nor the double mutant showed altered aggressiveness in point inoculated wheat spikes (Quarantin et al. Citation2016). For this study, both overexpression and disruption mutants of this gene were developed in WT strain GZ3639 to further investigate the role of FgCPP1 in disease aggressiveness. The mutants were screened for changes in their ability to cause initial FHB infection and disease spread by spray and point inoculation of wheat spikes, and observed changes in trichothecene accumulation in planta and in axenic cultures. Additionally, in planta localization of FgCPP1 was investigated by transient expression in tobacco cells.
Materials and methods
F. graminearum strain and growth parameters
Fusarium graminearum strain GZ3639 served as the WT strain in this study. The fungus was grown in 90 mm petri plates on potato dextrose agar (PDA, BD DifcoTM) or water agar. Macroconidia were prepared as previously described (Foroud et al. Citation2019b) in 100 mL of carboxymethylcellulose (CMC) medium (1.5% carboxymethylcellulose, 0.1% NH4NO3, 0.1% KH2PO4, 0.05% MgSO4.7H2O, 0.1% yeast extract). Macroconidia were separated from mycelia by filtration through an autoclaved paper towel (WypAll®), and then washed in sterile water by centrifugation. Macroconidial concentration was determined using a haemocytometer and adjusted to working concentrations (defined according to activities described below) in sterile distilled water.
Bioinformatic analyses of FgCPP1
The presence of a signal peptide and its cleavage site were predicted for the FgCPP1 protein sequence (FGSG_10212, XP_390388.1) using SignalP 5.0 (Almagro Armenteros et al. Citation2019). Protein domains were identified using SMART database (Letunic et al. Citation2015). The FgCPP1 amino acid sequence was aligned with CPPs from a group of ascomycete fungal species, excluding their signal peptides. The alignment was initially carried out using the Clustal Omega Multiple Sequence Alignment software from EMBL-EBI. To determine the evolutionary relationships of FgCPP1 with other characterized fungal CPPs, amino acid sequences of CPPs from ascomycete fungi were aligned by ClustalW and a phylogenetic tree was constructed by the Neighbour-Joining method using MEGA-X.
Generation of FgCPP1 disruption and in locus overexpression transformants
The disruption (Δfgcpp1) and overexpression (FgCPP1-OX) transformants were generated using the method of Frandsen et al. (Citation2008) with pRF-HU2 (for gene disruption) and pRF-HU2E (for overexpression) vectors. A detailed method is presented in the Fig. S1. The disruption and the overexpression cassettes were integrated into F. graminearum GZ3639 by an Agrobacterium tumefaciens-mediated transformation system, as previously described (Frandsen et al. Citation2012). In the Δfgcpp1 mutant, the FgCPP1 gene was replaced by an HYGROMYCIN PHOSPHOTRANSFERASE (HPH) selection marker cassette. The FgCPP1-OX strains (FgCPP1-OX1 and FgCPP1-OX2) were designed for in locus overexpression using a strong constitutive GLYCERALDEHYDE-3-PHOSPHATE DEHYDROGENASE (GPDA) promoter from Aspergillus nidulans; an HPH selection marker cassette followed by the GPDA promoter was integrated upstream of the FgCPP1 coding sequence.
Verification of Δfgcpp1 and FgCPP1-OX transformants
DNA isolation from the transformants was performed according to Tendulkar et al. (Citation2003). The transformants were screened by PCR (Fig. S2a and S2b). Single spores were isolated from PCR-positive transformants, which where then re-verified by PCR.
Copy number of FgCPP1 disruption and overexpression cassette in Δfgcpp1 and FgCPP1-OX1 transformants, respectively, was determined by DNA blot analysis. Ten micrograms of genomic DNA digested with EcoRI and NcoI (both from New England BioLabs) was run on 0.8% agarose gel. The DNA was then transferred from the gel to a positively charged nylon membrane (Sigma-Aldrich). For hybridization, a probe synthesized by PCR (primer pair 11; ) with digoxigenin (DIG)-labelled dUTP was used (PCR DIG Probe Synthesis Kit, Sigma-Aldrich). Hybridization was performed overnight at 47°C in DIG Easy Hyb buffer (Sigma-Aldrich). Hybridized DNA fragments were detected with an Anti-DIG antibody conjugated to alkaline phosphatase (DIG Nucleic Acid Detection Kit, Sigma-Aldrich). Restriction enzyme cutting sites and probe binding sites are indicated in Fig. S2c.
Table 1. Primer names and sequences for PCR and real time PCR.
Reverse transcription-quantitative PCR (RT-qPCR) was employed to determine FgCPP1 and FgCPP2 expression levels in FgCPP1 transformants. Total RNA was isolated from mycelia grown in potato dextrose broth for 5 days using QIAGEN’s RNeasy Plant Mini Kit. RNA samples were treated with DNase I (Thermo ScientificTM) and elimination of DNA was confirmed by failed PCR amplification of the elongation factor housekeeping gene (primer pair 15; ) when using the RNA as a template. cDNA was synthesized using SuperScriptR IV Reverse Transcriptase (Thermo ScientificTM) according to manufacturer’s instructions. RT-qPCR was performed in a QuantStudioTM 6 Flex Real-Time PCR System (Applied BiosystemsTM) with PerfeCTa SYBR Green SuperMix Low ROX (Quanta BiosciencesTM) (primer pairs 12 and 13 for FgCPP1 and FgCPP2, respectively; ). Samples were held at 50°C (2 min) followed by 95°C (3 min), followed by a PCR stage consisting of 40 cycles at 95°C (15 s) and 60°C (1 min), and finally a 3-step melt curve stage at 95°C (15 s), 60°C (1 min) and 95°C (15 s). FgCPP1 expression was normalized with F. graminearum β-TUBULIN (FGSG_09530) and ELONGATION FACTOR 1A (FGSG_08811) genes (primer pairs 14 and 15, respectively; ) and FgCPP2 expression with β-TUBULIN gene.
Mycelial growth and macroconidia germination
To measure vegetative growth, a 10-mm diameter mycelial plug collected using a cork borer from PDA cultures of Δfgcpp1, FgCPP1-OX1 or the WT was placed upside down at the centre of a 90 mm Petri plate containing PDA. Five PDA plates were used for each transformant and the plates were incubated at 27°C. The diameter of the colony was measured in two perpendicular directions every 24 h for 4 days. The average of the two measurements was used to evaluate mycelial growth.
To compare macroconidial germination in Δfgcpp1 and FgCPP1-OX1 with that of the WT, a modified protocol from Spolti et al. (Citation2012) was employed. A grid was drawn on the back of 90-mm Petri plates containing 1.5% water agar and squares (1cm2 each) were spotted with 5 μL of macroconidia (5 x 103 macroconidia mL−1). The Petri plates were incubated at 27°C, and the number of germinated and non-germinated macroconidia were counted at 6 and 9 h using a compound microscope. There were five replicates (squares) per treatment and 7–14 macroconidia were evaluated per replicate.
Cell wall stress assays
To assess stress tolerance in FgCPP1 transformants, F. graminearum growth was measured on PDA plates supplemented with 0.2 mg mL−1 calcofluor white (CFW), 0.5 mg mL−1 Congo red (CR), 0.01% sodium dodecyl sulphate (SDS) and 1 M NaCl. The concentrations tested for SDS and NaCl were selected based on previous studies (Chen et al. Citation2014; Quarantin et al. Citation2016). The concentration for CFW and CR were selected by first screening the effects of 0.05, 0.1, 0.15 or 0.2 mg mL−1 CFW and 0.3, 0.4 or 0.5 mg mL−1 CR on WT F. graminearum, where 0.2 and 0.5 mg mL−1 for CFW and CR were respectively found to be the minimum inhibitory concentrations for mycelial growth (data not shown). Cell growth inhibition was assessed by spot inoculating PDA plates (supplemented with or without the cell wall stress agents) with 5 µL of 10-fold dilutions of Δfgcpp1, FgCPP1-OX1 or the WT macroconidia starting at 6 × 105 macroconidia mL−1. Pictures were taken after 40 h of growth at 25°C in the dark. The experiment was repeated two times, each with three replicates.
In order to assess sensitivity of FgCPP1 transformants to cell wall-degrading enzymes, F. graminearum macroconidia germination was monitored in yeast malt (YM) broth (0.4% sucrose, 0.4% yeast extract and 0.4% malt extract) containing 1.4 or 1.6 mg mL−1 Glucanex using a modified protocol from Rittenour et al. (Citation2013). These two concentrations were selected by first screening the effects of 0.5–1.7 mg mL−1 Glucanex on WT F. graminearum. While 1.4 mg mL−1 slightly affected macroconidia germination, 1.6 mg mL−1 almost completely inhibited it (data not shown). The effects of these two Glucanex concentrations on the macroconidia germination of FgCPP1 transformants were assessed by incubating Δfgcpp1, FgCPP1-OX1 or WT macroconidia (25 μL from 106 macroconidia mL−1) in YM broth with or without Glucanex at 28°C for 14 h. The germinating conidia were observed and photographed using EVOS FL Auto Imaging System (Thermo ScientificTM) with a 20X objective lens. The experiment was repeated two times, each with three replicates and 8 macroconidia were observed for each replicate.
Wheat spike infection by spray and point inoculations
Wheat cultivars ‘Roblin’, ‘Penhold’, ‘Awesome’ and ‘Tenacious’, possessing different levels of FHB resistance and susceptibility (), were used for infection assays. Plants were grown in 2.7-L pots with Cornell mix (Boodley and Sheldrake Citation1972) in a greenhouse at 22/18°C (day/night) with a 16 h photoperiod. Pots were watered daily and fertilized biweekly. Prophylactic sprays were given at 5–7 leaf stage with Nova (Dow AgroSciences Canada) to prevent powdery mildew.
Table 2. Wheat cultivars used for infection assays and their major resistance mechanisms.
Plants were inoculated with Δfgcpp1, FgCPP1-OX1 or WT at anthesis. Wheat cultivars ‘Roblin’, ‘Penhold’, and ‘Awesome’ were sprayed with 105 macroconidia mL−1 of the strains with 0.05% Tween 20 (each spike was sprayed 10 times, where each spray is roughly equivalent to 0.1 mL of inoculum) using an atomized hand sprayer. Wheat cultivars ‘Roblin’, ‘Penhold’, and ‘Tenacious’ were point inoculated by pipetting 10 µL of 105 macroconidia mL−1 of the strains between lemma and palea of a spikelet located approximately at one-third of the length of the spike from the top. Following inoculations, plants were kept in a mist-irrigated growth cabinet with 95% relative humidity (90% for point inoculation) and 25°C (day/night). After 3 days, plants were returned to the greenhouse. Disease severity was evaluated as the percentage of spikelets per spike exhibiting symptoms at 3, 6, 9 and 18 days post-inoculation (dpi) for spray inoculated plants; for point inoculation, the number of diseased spikelets below the inoculated spikelet, including the inoculated spikelet, were counted at 7, 12 and 18 dpi. The spikelets above the point of inoculation can develop a blighted appearance due to blockage of xylem vessels resulting from the infection at the inoculation site and were not counted as symptomatic spikelets. For each inoculation type, there were five replications per treatment consisting of five spikes, each from different plants. The experiments were each repeated three times.
Another spray inoculation experiment was carried out in ‘Penhold’, as detailed above, but using the additional overexpression strain FgCPP1-OX2, as well as the WT, Δfgcpp1 and FgCPP1-OX1. For each strain, 10 spikes, each from different plants, were inoculated and the experiment was repeated three times. Percentage of diseased spikelets was recorded at 3, 6 and 9 dpi.
Fungal biomass estimation in wheat spikes
Spikes of the cultivar ‘Penhold’ were spray inoculated with Δfgcpp1, FgCPP1-OX1 or the WT, as described above. Five spikes per treatment from each of the experimental repetitions were harvested together at 3 dpi and flash-frozen in liquid nitrogen. The tissue was homogenized to a powder in liquid nitrogen using a mortar and pestle, and DNA was isolated from 100 mg of the powder with QIAGEN’s DNeasy Plant Mini Kit. qPCR was performed in a QuantStudioTM 6 Flex Real-Time PCR System (Applied BiosystemsTM) with PerfeCTa SYBR Green SuperMix Low ROX (Quanta BiosciencesTM) (primer pair 15; ) using the following program: a hold stage of 50°C (2 min) followed by 95°C (10 min), a PCR stage (40 cycles) of 95°C (15 s) and 58°C (45 s), and a melt curve stage of 95°C (15 s) and 60°C (1 min) followed by 95°C (15 s). The amount of fungal DNA in wheat samples was calculated from a standard curve generated using 10-fold dilution series of F. graminearum genomic DNA (primer pair 15; ). The results were expressed as picogram (pg) of fungal DNA per 25 ng of total DNA (wheat and F. graminearum) (Rossi et al. Citation2007).
Measurement of 15-acetyldeoxynivalenol (15-ADON) and deoxynivalenol (DON)
The trichothecenes 15-ADON and DON were measured in axenic culture and in planta, respectively. To induce trichothecene biosynthesis in axenic culture, a modified protocol from Miller and Blackwell (Citation1986) was used. A 6-well Clear Multiwell Plate (Falcon, catalogue # 353 046) with a sterile 100 µm nylon net filter (NY1H type, Millipore Ref# NY1H02500) and 4 mL of first stage medium (0.3% NH4Cl, 0.2% MgSO4.7H2O, 0.02% FeSO4.7H2O, 0.2% KH2PO4, 0.2% peptone, 0.2% yeast extract, 0.2% malt extract and 2% glucose, pH 7.0) per well was inoculated with 10 μL of 2 × 104 macroconidia mL−1 of Δfgcpp1, FgCPP1-OX1 or WT. The culture plates were sealed with parafilm and incubated in the dark at 27°C, 170 rpm for 24 h, after which, the medium was removed using a sterile transfer pipette without disturbing the mycelia, which were washed two times with sterile distilled water. The nylon filter with mycelia was re-suspended in 4 mL of second stage medium (0.1% putricine di-HCl, 0.3% KH2PO4, 0.02% MgSO4.7H2O, 0.5% NaCl, 4% sucrose and 1% glycerol, pH 4.0). After 48 h of incubation in the dark at 27°C, 170 rpm, 450 µL of the supernatant filtered through a 0.2-micron membrane was mixed with 150 µL of MeOH for HPLC analysis using a Shimadzu prominence LC-20AD (Mandel) equipped with a Shimadzu SIL-20A HT prominence auto sampler. One hundred microlitres of sample were loaded onto a Restek Pinnacle DB C18 Column (5 µm, 150 × 4.6 mm Cat#9 414 565) with a 22.5% isocratic MeOH: H2O flow over 20 minutes at a rate of 1 mL min−1. 15-ADON was monitored by UV at 220 nm, quantified using a standard curve with pure 15-ADON (provided by Dr. Barbara Blackwell, Ottawa Research and Development Centre, Agriculture and Agri-Food Canada) and normalized against the mycelial dry weight.
Spikes of the cultivar ‘Penhold’ were point or spray inoculated with Δfgcpp1, FgCPP1-OX1 or WT, as described in the section on wheat spike infection assays. Spray inoculation was carried out as described for the infection assay. For each point inoculated spike, 10 µL of spore suspension was pipetted into one floret from each of two adjacent spikelets per spike. For each of the three experimental repetitions, 5 spikes and 10 spikelets from spray and point inoculated treatments, respectively, were harvested together at 1, 2 and 3 dpi and flash-frozen in liquid nitrogen. The amount of DON was estimated in inoculated wheat tissues using ELISA, as previously described (Sinha et al. Citation1995).
Subcellular localization of FgCPP1
The FgCPP1 coding sequence with or without its signal peptide (SP) was amplified by Phusion Hotstart II DNA polymerase (Thermo Scientific) (primer pair 16 for FgCPP1+ SP and 17 for FgCPP1-SP; ) and the PCR product was cloned into pDONRTM221 by Gateway BP cloning technology (Thermo Fisher Scientific). The resulting entry clones were cloned into pGWB451 (for C-terminal fusion with G3GFP) or pGWB454 (for C-terminal fusion with mRFP) (Nakagawa et al. Citation2007) by LR reaction (Thermo Fisher Scientific) generating 35S:FgCPP1-SP:GFP, 35S:FgCPP1+ SP:GFP, 35S:FgCPP1-SP:mRFP and 35S:FgCPP1+ SP:mRFP, respectively. These constructs were verified by sequencing and transformed into A. tumefaciens strain GV3101. The positive fluorescent controls, 35S:GFP in pBIN+ (van Engelen et al. Citation1995) and 35S:mCherry in pCAMBIA (Beauchemin and Laliberté Citation2007), as well as the C-terminally GFP tagged plasma membrane marker CD3-1003 (Nelson et al. Citation2007) were transformed into A. tumefaciens strain GV3101.
Agroinfiltration assays in Nicotiana benthamiana were performed as described in Montenegro Alonso et al. (Citation2020) using a final infiltration OD600 of 0.3. For co-agroinfiltration assays with the plasma membrane marker, Agrobacterium strains carrying corresponding plasmids were mixed in equal concentrations for a final infiltration OD600 of 0.5. Agroinfiltration of individual constructs or a combination of constructs was carried out using a minimum of three-week-old N. benthamiana plants. One day after agroinfiltration, confocal microscopy was performed, and leaf samples were collected for protein blots.
Proteins were extracted from agroinfiltrated leaves of N. benthamiana for protein blots as described in Montenegro Alonso et al. (Citation2020). An anti-GFP (Clontech Living Colours JL-8) or anti-mCherry (Abcam 1C51) antibody was used to probe the membranes. Coomassie staining of the membranes was used as the loading control for protein blots.
A Leica TCS SP8 confocal laser scanning microscope in a sequential scanning mode was used to visualize fluorescence. Cells from at least two different locations of an infiltrated leaf were visualized from a minimum of two independently agroinfiltrated plants and the experiment was repeated twice. Plasmolysis was induced by exposing agroinfiltrated leaves of N. benthamiana at 24 hours post-agroinfiltration (hpi) to 0.75 M sucrose for 4 hours before visualization. Excitation at 480 nm and emission at 493–550 nm was used to detect the GFP and excitation at 587 nm and emission at 598–644 nm was used for mCherry. Chloroplasts were excited at 480 nm and visualized by collecting at 680–700 nm.
Statistical analyses
RT-qPCR data were analysed using REST© software (Pfaffl et al. Citation2002) to obtain relative expression of genes in FgCPP1 transformants compared to WT. Data from the remaining experiments were analysed using one-way analysis of variance (ANOVA) at p < 0.05. The means between treatments were separated by Fisher’s least significant difference (LSD). Data from experimental repetitions were pooled together for analysis when there was homogeneity of variances according to Levene’s test (p < 0.05).
Results
Bioinformatic analyses of FgCPP1
FgCPP1 is a secreted protein (Paper et al. Citation2007), and the analysis of the sequence indicates that the first 18 amino acids constitute the predicted signal peptide and the remaining residues (19–137) encode the CP domain of this protein. Multiple sequence alignment of CPPs from different ascomycete fungal species revealed four highly conserved cysteine residues (). A D77 residue essential for the expansin-like activity in Ceratocystis platani CP (Luti et al. Citation2017) is conserved in other fungal CPPs (). A phylogenetic tree was constructed with amino acid sequences of FgCPP1 and other CPPs from ascomycete fungi (). FgCPP1 was found to be most closely related to CPPs from Fusarium pseudograminearum and Fusarium poae, and similar to BcSpl1 and SsCP1, which have been identified as factors contributing to aggressiveness in Botrytis cinerea and Sclerotinia sclerotiorum, respectively (Frías et al. Citation2011; Yang et al. Citation2018). FgCPP2 was distant from FgCPP1 in the phylogenetic tree and appeared to be more closely related to FocCP1, which was found to be essential for Fusarium oxysporum f.sp. cubense Tropical Race 4 aggressiveness in banana plants (Liu et al. Citation2019; Feng et al. Citation2021).
Fig. 1 (a) Protein sequence alignment and (b) phylogenetic analysis of CPPs from ascomycete fungal species, excluding their signal peptide domain. In the alignment, four highly conserved cysteine residues are highlighted in grey, and a conserved aspartate residue thought to be essential for the expansin-like activity of fungal CPPs is underlined in blue font. The alignment was created using the Clustal Omega Multiple Sequence Alignment software from EMBL-EBI. The Neighbour-Joining phylogenetic tree was constructed ClustalW aligned sequences using the bootstrap method (500 replicates) using MEGA-X software. The GenBank accession numbers of CPPs used for the alignment are ABM63505.1, XP_024547455, XP_001588549.1, EXM33099.1, XP_018240137.1, OBS29207.1, XP_390388.1, XP_009255055.1, XP_003710181.1, EGY15345.1, XP 391381.1, XP_018252361, AAZ80388.1 and ABE73692.1, respectively in the order shown in the alignment.
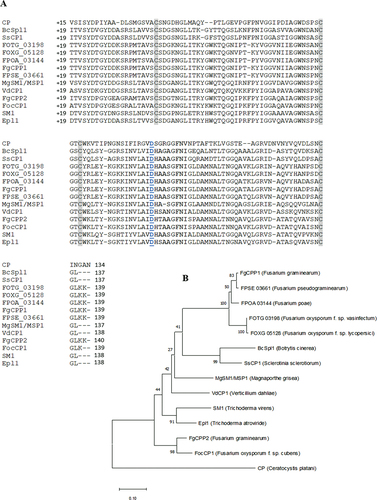
Analysis of Δfgcpp1 and FgCPP1-OX transformants
DNA blot analysis confirmed that a single copy of the FgCPP1 overexpression construct was integrated into the FgCPP1-OX1 transformant and the FgCPP1 gene was properly disrupted in Δfgcpp1 mutant (Fig. S2). FgCPP1 and FgCPP2 gene expressions in FgCPP1 disruption and overexpression strains were analysed from mycelia grown in potato dextrose broth. Expression analysis by RT-qPCR revealed a 3.3- and 2.7-fold increase in FgCPP1 expression in FgCPP1-OX1 and FgCPP1-OX2, respectively compared to WT. As expected, no FgCPP1 transcript was detected in the Δfgcpp1 disruption mutant (). Disruption or overexpression of FgCPP1 did not effect any significant change in FgCPP2 expression ().
Fig. 2 (a) FgCPP1 and (b) FgCPP2 expression in FgCPP1 disruption and overexpression transformants relative to WT as determined by RT-qPCR. Fusarium graminearum β-TUBULIN and ELONGATION FACTOR 1A genes were used for FgCPP1 normalization and β-TUBULIN gene for FgCPP2 normalization. Bars represent standard error from three replicates, each performed in triplicate. Asterisks indicate statistically significant differences compared with the WT (p < 0.05, pairwise fixed reallocation randomization test).
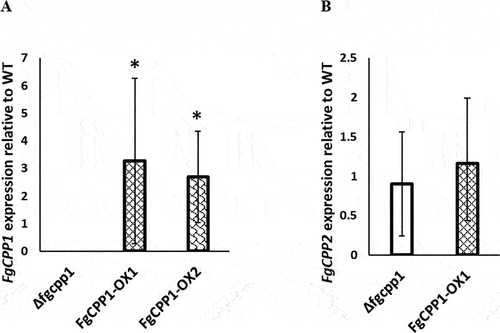
Altered expression of FgCPP1 does not affect fungal growth or germination
The effect of FgCPP1 disruption or overexpression on F. graminearum macroconidial germination and mycelial growth was monitored. Macroconidia of Δfgcpp1 or FgCPP1-OX1 incubated on water agar did not show a significant difference in germination compared to WT. Approximately, 75% and 100% germination was observed, respectively at 6 h and 9 h after incubation in both the WT and altered strains (). Similarly, no change in mycelial growth was observed on PDA over a 4-day period ().
Fig. 3 Role of FgCPP1 in general fitness of Fusarium graminearum. (a) Percentage germination of macroconidia of Δfgcpp1, FgCPP1-OX1 and WT on water agar after 6 and 9 h incubation at 27°C. Bars represent standard error from five replicates. (b) Mycelial growth of Δfgcpp1, FgCPP1-OX1 and WT over 4 days of growth on PDA at 27°C. Bars represent standard error from five replicates.
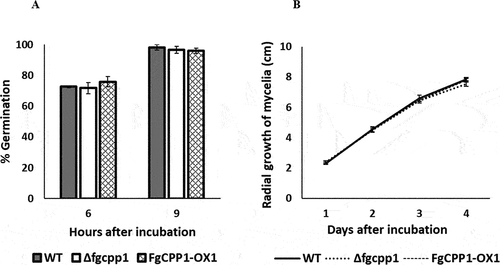
Altered expression of FgCPP1 does not influence cell wall integrity
Since CPPs from some fungal species have been suggested to have a protective role against cell wall stress agents (Pan et al. Citation2018) or cell wall degrading enzymes (Quarantin et al. Citation2016; Zhang et al. Citation2017a), experiments were carried out to assess the effect of cell wall stress in Δfgcpp1 and FgCPP1-OX1.
PDA plates were supplemented with CFW or CR to assess cell wall integrity, SDS to test cell membrane integrity, and NaCl to assess sensitivity to osmotic stress. The plates were then inoculated with Δfgcpp1 and FgCPP1-OX1 or WT macroconidia. All transformants exhibited a similar growth pattern as the WT on PDA plates with the different stress agents ().
Fig. 4 Sensitivity of FgCPP1 transformants to chemicals targeting fungal cell wall/membranes. (a) Mycelial growth from different macroconidia dilutions of Δfgcpp1, FgCPP1-OX1 and WT on PDA containing cell wall/membrane stress agents. SDS = sodium dodecyl sulphate, CFW = calcofluor white and CR = Congo red. Images were taken after 40 h of growth at 25°C. (b) Macroconidia of Δfgcpp1, FgCPP1-OX1 and WT germinating in YM broth containing cell wall degrading enzyme, Glucanex. Images were taken after 14 h of incubation at 28°C. Scale bar = 200 μm. Image background was removed, and individual images were combined into a single file in power point. Images for A and B are representative of two independent experiments, each with three replicates.
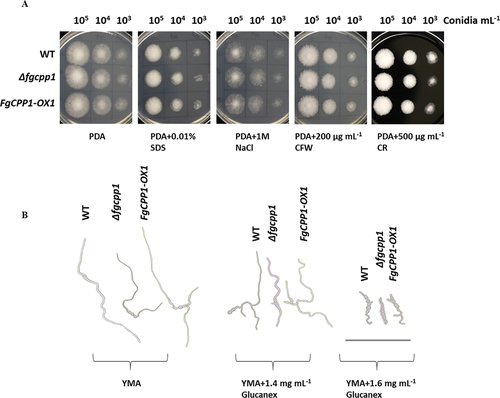
To determine whether altered expression of FgCPP1 leads to a change in sensitivity of F. graminearum to cell wall degrading enzymes, the macroconidia of the transformants were incubated with Glucanex (a commercial mixture of β-glucanase, cellulase and chitinase) and macroconidia germination was monitored. The two concentrations used in this experiment affected macroconidia germination; however, the transformants and the WT showed similar sensitivity to Glucanex ().
FgCPP1 overexpression has a stimulatory effect on disease incidence
To determine the involvement of FgCPP1 in F. graminearum infection, wheat plants were inoculated with Δfgcpp1, FgCPP1-OX1 and WT. Wheat cultivars with a range of FHB resistance and susceptibility () were selected for spray inoculation (‘Roblin’, ‘Penhold’ and ‘Awesome’) and point inoculation (‘Roblin’, ‘Penhold’ and ‘Tenacious’).
Following spray inoculation with FgCPP1-OX1, a significant increase (p < 0.05) in disease was observed at 3 dpi in ‘Roblin’, ‘Penhold’ and ‘Awesome’, where the percentage of diseased spikelets was 1.4, 1.7 and 1.6 times higher than in plants infected with WT, respectively. By contrast, disease caused by spray inoculation of Δfgcpp1 resulted in comparable levels of infection as the WT in each of the cultivars assessed (). Early disease symptoms from spray inoculated plants may be thought of as initial infection, and despite an increase in the percentage of diseased spikelets as early as 3-days post spray inoculation with FgCPP1-OX1, the fungal biomass estimated at 3 dpi from spray inoculated ‘Penhold’ spikes did not differ from the WT (). Meanwhile, cultivars ‘Roblin’, ‘Penhold’ and ‘Tenacious’ point inoculated with FgCPP1-OX1 or Δfgcpp1 exhibited similar disease spread as the WT ().
Fig. 5 Role of FgCPP1 in Fusarium graminearum infection. (a) Percentage of diseased spikelets of wheat cultivars ‘Roblin’, ‘Penhold’ and ‘Awesome’ 3, 6, 9 and 18 days after spray inoculation with Δfgcpp1, FgCPP1-OX1 or WT. The experiment was performed three times, each time with five replicates per treatment. For ‘Roblin’, ‘Penhold’ and ‘Awesome’, data pooled from repetitions had equal variances and the pooled data were used for analysis. Asterisks indicate statistically significant differences compared with the WT (p < 0.05). (b) Quantity of Δfgcpp1, FgCPP1-OX1 or WT in spikes of ‘Penhold’ 3 days after spray inoculation as determined by qPCR. The inoculations were performed three times, each time with five replicates per treatment. The five replicates (spikes) per treatment for each repetition were pooled for DNA extraction. From each DNA sample, three technical replicates were made for qPCR. Data from three repetitions were used for analysis. (c) Number of diseased spikelets of ‘Roblin’, ‘Penhold’ and ‘Tenacious’ 7, 12 and 18 days after point inoculation with Δfgcpp1, FgCPP1-OX1 or WT. The experiment was performed three times, each time with five replicates per treatment. For ‘Roblin’ and ‘Tenacious’, data pooled from repetitions had equal variances and the pooled data were used for analysis. For ‘Penhold’, data from one repetition was used for analysis and the results agreed with other two repetitions. (d) Percentage of diseased spikelets of wheat cultivar ‘Penhold’ 3, 6 and 9 days after spray inoculation with Δfgcpp1, FgCPP1-OX1, FgCPP1-OX2 or WT. The experiment was performed three times, each time with 10 replicates per treatment. Data pooled from repetitions had equal variances and the pooled data were used for analysis.
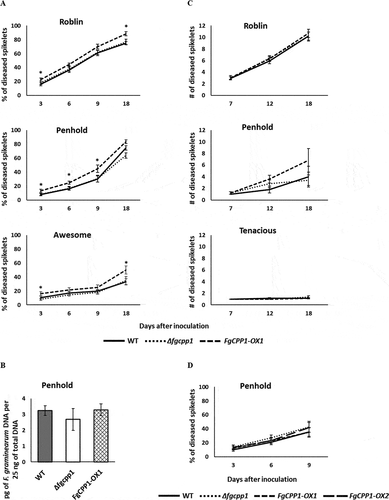
Since there was no apparent increase in fungal biomass where an increase in disease was consistently observed for FgCPP1-OX1, and since the increase in disease was relatively small (less than two times different), a second FgCPP1-OX transformant, namely FgCPP1-OX2, was assessed for its ability to cause disease for comparison. In this experiment, Δfgcpp1, FgCPP1-OX1 and FgCPP1-OX2 were spray inoculated on ‘Penhold’ spikes. However, in this experiment none of the strains showed any statistical difference in the percentage of diseased spikelets compared with the WT ().
FgCPP1 overexpression results in increased 15-ADON and DON production in F. graminearum
To determine whether FgCPP1 disruption or overexpression influences trichothecene accumulation, the amount of 15-ADON and DON produced by FgCPP1 transformants in axenic culture and in planta, respectively, was determined. 15-ADON production by Δfgcpp1 did not differ significantly from the WT, whereas FgCPP1-OX1 accumulated significantly higher amounts of 15-ADON in axenic culture compared to WT ().
Fig. 6 Effect of FgCPP1 on DON production. (a) 15-ADON accumulation by Δfgcpp1, FgCPP1-OX1 or WT in axenic culture under DON-inducing conditions. Bars represent standard error from six replicates. (b) DON accumulation in spray inoculated ‘Penhold’ spikes at 1, 2 and 3 dpi with Δfgcpp1, FgCPP1-OX1 or WT. The inoculations were performed three times, each with five replicates for each time point. For each repetition, five replicates (spikes) were harvested together for each time point for DON estimation. Data from three repetitions were used for analysis. (c) DON production in point inoculated ‘Penhold’ spikelets at 1, 2 and 3 dpi with Δfgcpp1, FgCPP1-OX1 or WT. The inoculations were performed three times, each with five replicates for each time point. For each repetition, five replicates were harvested together fore each time point for DON estimation. Data from three repetitions were used for analysis. Asterisks indicate statistically significant differences compared with the WT (p < 0.05).
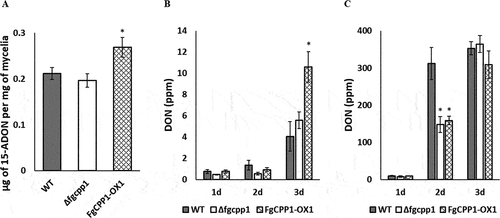
In spray inoculated ‘Penhold’ spikes, DON accumulation by FgCPP1-OX1 was comparable to the WT at 1 and 2 dpi, but by 3 dpi an increase in DON accumulation (2.6 times) was observed in FgCPP1-OX1 infected spikes. Meanwhile, DON accumulation in Δfgcpp1 infected spikes did not significantly differ from WT at any sampling dates (). DON levels from point inoculated spikelets showed a different pattern than spray inoculated spikes. While DON accumulation at 1 and 3 dpi did not differ in Δfgcpp1 or FgCPP1-OX1 infected spikes compared to WT, DON levels were 2.1 times and 1.97 times lower for Δfgcpp1 and FgCPP1-OX1, respectively compared to WT at 2 dpi ().
Subcellular localization of FgCPP1
Fluorescently tagged FgCPP1 with or without its signal peptide (FgCPP1+ SP and FgCPP1-SP, respectively) was transiently expressed by agroinfiltration in N. benthamiana leaves followed by confocal microscopy. At 24 hpi, FgCPP1-SP tagged with either GFP or mRFP fluorescent moieties at its C-terminal end localized to the cytosol and nucleoplasm of N. benthamiana cells a-ii,b-ii), similar to the localization observed for the positive fluorescent controls GFP or mCherry (a-I,b-i). On the other hand, FgCPP1+SP with either tag was found localized to the cytosol, nucleoplasm and chloroplast of N. benthamiana cells (a-iii,b-iii). Plasmolysis of N. benthamiana cells was performed to reveal the apoplast and fluorescence therein. No fluorescence was detected in the apoplastic space of plasmolysed N. benthamiana cells expressing FgCPP1+ SP:GFP or FgCPP1+ SP:mRFP (a-iv,b-iv). Protein blots confirmed the presence of the full-length FgCPP1-SP:GFP (41 kDa), FgCPP1+ SP:GFP (43 kDa), FgCPP1-SP:mRFP (40 kDa) and FgCPP1+ SP:mRFP (42 kDa) as well as some break-down products in N. benthamiana cells at 24 hpi as detected by respective antibodies (). While the localization of FgCPP1 (-SP and +SP) tagged with either GFP or mRFP was similar, overall fluorescence and protein accumulation of FgCPP1:mRFP (-SP and +SP) chimeras was lower compared with the same GFP-tagged constructs and also involved a higher amount of break-down products (). No colocalization with a plasma membrane marker was observed upon co-expression with FgCPP1:mRFP supporting an intracellular localization for this protein (Fig. S3).
Fig. 7 Intracellular localization of FgCPP1 in Nicotiana benthamiana cells. Leaves of N. benthamiana were agroinfiltrated with FgCPP1 constructs tagged with florescent proteins and used for confocal microscopy at 24 hpi. (a) The fluorescent control GFP (i) and FgCPP1-SP:GFP (ii) showed a nucleoplasm and cytosolic (white arrow) localization while FgCPP1+ SP:GFP (iii) showed a nucleoplasm, cytosolic and chloroplastic (yellow arrow) localization. Localization of FgCPP1+ SP:GFP was not detected in the apoplast as evident by the lack of fluorescence on the apoplastic space (asterisk) visualized after plasmolysis (iv). (b) The fluorescent control mCherry (i) and FgCPP1-SP:mRFP (ii) showed nucleoplasm and cytosolic (white arrow) localization. FgCPP1+ SP:mRFP (iii) fluorescence localized to the nucleoplasm, cytosol and chloroplast (yellow arrow). Induction of plasmolysis in cells expressing FgCPP1+ SP:mRFP (iv) showed no apoplastic (asterisk) fluorescence. (c) Protein blots of agroinfiltrated plants used in section (a) showed the presence of the full-length GFP (27 kDa), FgCPP1-SP:GFP (41 kDa) and FgCPP1+ SP:GFP (43 kDa) along with some break-down products (two products of ~30 and ~35 kDa) at 24 hpi. (d) Protein blots of agroinfiltrated plants of section (b) detected the full-length mCherry (27 kDa), FgCPP1-SP:mRFP (40 kDa) and FgCPP1+ SP:mRFP (42 kDa) accompanied by some break-down products (~35 kDa products for FgCPP1-SP:mRFP and FgCPP1+ SP:mRFP) at 24 hpi. The loading control corresponds to the large unit of Rubisco visualized upon exposing membranes to Coomassie stain. Representative results of two experimental repeats of confocal microscopy and protein blots are shown. The images in section a-b constitute snapshots of a single optical section and the scale bar on them represents 10 µm.
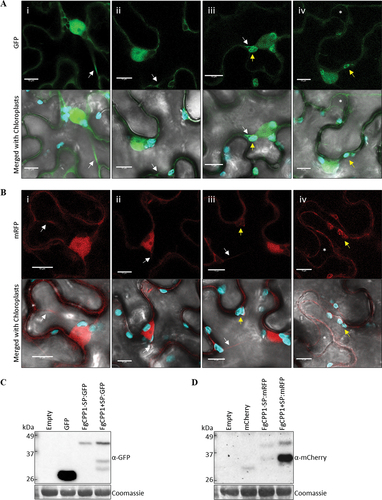
Discussion
CPPs are cysteine-rich proteins with signal peptides for secretion, and CPPs have been detected in fungal culture filtrates (Pazzagli et al. Citation1999; Wilson et al. Citation2002; Jeong et al. Citation2007; Frías et al. Citation2011; Lu and Edwards Citation2016; Yang et al. Citation2018). Genes encoding CPPs are upregulated in some fungal pathogens during infection of their host plants (Wilson et al. Citation2002; Frías et al. Citation2011; Quarantin et al. Citation2016; Zhang et al. Citation2017a; Yang et al. Citation2018; Liu et al. Citation2019), and genetic studies have shown that some of these genes contribute to fungal virulence (Frías et al. Citation2011; Zhang et al. Citation2017a; Yang et al. Citation2018; Liu et al. Citation2019). The role of FgCPP1, whose transcripts were found to be upregulated in F. graminearum strain WT:8/1 during wheat spike infection (Quarantin et al. Citation2016) while protein abundance was decreased in the secretome of a non-aggressive Δtri6 mutant (Rampitsch et al. Citation2013), was selected for this study. Fusarium graminearum strains with targeted FgCPP1 gene disruption or in locus constitutive overexpression of the gene were generated in the GZ3639 WT background by Agrobacterium-mediated transformation in order to study the role of FgCPP1 in fungal growth and plant infection. This is the first study to employ in locus overexpression to study the function of a Fusarium gene and the first report investigating overexpression of a FgCPP gene. The FgCPP1 disruption mutants from previous reports (Quarantin et al. Citation2016, Citation2019) were developed in the WT:8/1 strain. It is unknown whether FgCPP1 from different F. graminearum strains contribute differently to aggressiveness. However, the cerato-platanin MSP1 from Magnaporthe grisea had different roles in infection in two different M. grisea strains. While deletion of this gene in M. grisea strain Guy 11 did not affect its aggressiveness in rice (Wang et al. Citation2016), deletion of the same gene in strain 70–15 led to reduced aggressiveness (Jeong et al. Citation2007).
A single copy of the overexpression cassette integrated at the FgCPP1 locus in a FgCPP1-OX1 transformant resulted in upregulated expression of the FgCPP1 gene (, Fig. S2b and S2d). No FgCPP1 transcripts were detected in the Δfgcpp1 strain and no compensatory effect on the expression of the other cerato-platanin gene, FgCPP2, was observed (). The three putative F. graminearum CPPs (FGSG_04471, FGSG_03971 and FGSG_17103) previously reported to share only 8% sequence identity with FgCPP1 and FgCPP2, are not likely to compensate for the absence of FgCPP1. In their analysis of the ΔΔfgcpp1,2 double mutant, Quarantin et al. (Citation2016) found that the relative expression of FGSG_03971 was comparable to that of the WT during host infection, and FGSG_04471 was downregulated, behaviour that is not consistent with compensatory activities for either FgCPP1 or FgCPP2.
No change in macroconidial germination or mycelial growth was observed in the disruption or overexpression transformants of FgCPP1 in F. graminearum compared with the WT (). This is consistent with a previous study in which mycelial growth of disruption mutants Δfgcpp1 or ΔΔfgcpp1,2 which did not differ from WT (Quarantin et al. Citation2016). Furthermore, deletion of several genes encoding CPPs from other fungal species, despite their expression during in vitro growth, did not alter their phenotype related to growth and development (Jeong et al. Citation2007; Frías et al. Citation2011; Frischmann et al. Citation2013; Zhang et al. Citation2017b; Yang et al. Citation2018).
CPPs were previously shown to be localized to the fungal cell wall (Boddi et al. Citation2004; Frías et al. Citation2014) and some fungal CPPs have also been found to bind chitin (Barsottini et al. Citation2013; Frischmann et al. Citation2013; Baccelli et al. Citation2014), suggesting a potential role for CPPs in protecting the fungal cell wall from host defence responses. Indeed, CPP deletion mutants of Verticillium dahliae and F. graminearum (Δvdcp1 and ΔΔfgcpp1,2, respectively) showed increased sensitivity to the cell wall degrading enzyme, chitinase (Quarantin et al. Citation2016; Zhang et al. Citation2017a), and RNAi-directed silencing of a CPP-encoding gene, SM1 in S. sclerotiorum, resulted in its hypersensitivity to cell wall stress agents (Pan et al. Citation2018). Therefore, it was surprising that Δfgcpp1 or FgCPP1-OX1 strains used in this study did not show, in comparison to WT, an altered sensitivity to cell wall stress agents or cell wall degrading enzymes (). This discrepancy could be explained by differences in experimental approaches. In this study, macroconidia of Δfgcpp1 mutant were incubated with Glucanex (β-glucanase, cellulase and chitinase activities) and the hyphal growth was examined using a microscope. By contrast, Quarantin et al. (Citation2016) incubated macroconidia of the double mutant (ΔΔfgcpp1,2) with chitinase alone, and used absorbance of resazurin dye to determine the effect of the enzyme on fungal growth. While the expression of FgCPP2 was unaffected in the Δfgcpp1 mycelia grown in rich medium, the possibility that FgCPP2 activity during cell wall stress may compensate for this in the single mutant (Δfgcpp1, this study) cannot be discounted. Interestingly, the protective role of FgCPPs against chitinase was not observed when purified FgCPPs were incubated with a chitin solution in the presence of chitinase (Quarantin et al. Citation2019). Importantly, the authors (Quarantin et al. Citation2019) determined that, unlike many other fungal CPPs, which have been shown to bind chitin in vitro (Barsottini et al. Citation2013; Frischmann et al. Citation2013; Baccelli et al. Citation2014), FgCPP1 and FgCPP2 were not able to do so. Thus, the lack of difference in sensitivity in Δfgcpp1 or FgCPP1-OX1 transformants to cell wall stress agents or cell wall degrading enzymes compared to WT may be explained by the inability of FgCPP1 to bind chitin, and hence to the fungal cell wall. It should also be noted that the localization of FgCPP1 to the cell wall has not been reported in F. graminearum, and in fact, in tobacco cells, FgCPP1 was localized to the cytosol and nucleus (a-ii,b-ii).
Previous reports show that fungal CPPs possess cellulose weakening abilities (Barsottini et al. Citation2013; Baccelli et al. Citation2014), a property shared by expansins (Chen et al. Citation2010; Georgelis et al. Citation2011). In their study, Quarantin et al. (Citation2019) observed a synergistic effect for FgCPP1 and FgCPP2 on the activity of a fungal cellulase, β-1,4-glucanase, on various cellulosic substrates such as carboxymethylcellulose (CMC), filter paper and wheat cell walls. Incubation of CMC or wheat cell walls with β-1,4-glucanase released more reducing sugars in the presence of FgCPPs than in their absence. The expansin-like cellulose loosening activity of FgCPPs is likely responsible for the improved β-1,4-glucanase activity on these cellulosic materials (Quarantin et al. Citation2019). This synergistic effect of FgCPPs on fungal cellulase may facilitate advancement of fungal hyphae into the plant tissue. Similar synergies for cellulose hydrolysis activity has also been reported for bacterial expansin-like protein, EXLX1 (Yan et al. Citation2012). Deletion of EXLX1 in Bacillus subtilis affected the ability of this bacterium to colonize maize roots (Kerff et al. Citation2008). Furthermore, overexpression of an expansin-like protein, swollenin, improved the ability of Trichoderma asperellum to colonize cucumber roots (Brotman et al. Citation2008). In this study, overexpression of a cerato-platanin-encoding gene (FgCPP1) resulted in a significant increase in diseased spikelets of different wheat cultivars at 3 days post-spray inoculation (). Despite the observed increase in disease symptoms by FgCPP1-OX1 in wheat spikes, fungal biomass estimated from FgCPP1-OX1-inoculated wheat spikes was not significantly higher than that from WT-inoculated spikes (). In an attempt to validate the results where an increase in disease was observed, with no difference in fungal biomass, the disease assay was repeated with the additional FgCPP1-OX2 overexpression strain. In this experiment, contrary to the earlier results for FgCPP1-OX1 (), neither of FgCPP1 overexpression strains showed a statistically significant increase in percentage of diseased spikelets in the wheat cultivar ‘Penhold’ (). FHB disease data is variable in nature and this along with a minor role for FgCPP1, if any, in FHB may explain why the small increase in disease for FgCPP1-OX1 in the first experiment was not observed in the second experiment. Upregulation of FgCPP1 gene expression in F. graminearum WT during wheat spike infection has been previously reported (Quarantin et al. Citation2016). It may be that the expression levels of FgCPP1 in the WT strain during infection does not differ much from the level of the constitutively overexpressing FgCPP1 in the FgCPP1-OX strains. That said, an increase in DON accumulation was observed in planta in a separate experiment when comparing the FgCPP1-OX1 to the WT strain during its interaction with the host (), which may explain the increase in disease symptoms reported in the first infection assay experiment ().
Deletion of FgCPP1 (Δfgcpp1) had no effect on initial infection of wheat spikes by F. graminearum (). Quarantin et al. (Citation2019) reported a significantly higher cellulase activity for their ΔΔfgcpp1,2 mutant compared to WT. Thus, it seems likely that the increased cellulase activity by Δfgcpp1 could compensate for the loss of expansin-like activity of FgCPP1 in this mutant, and may explain the fact that no change was observed in initial infection by the disruption mutant. Δfgcpp1 and FgCPP1-OX1 transformants were also screened by point inoculation for their change in ability to cause disease spread in wheat spikes. Neither FgCPP1 disruption nor overexpression was found to have an effect on disease spread by F. graminearum in the rachis of wheat spikes (). Quarantin et al. (Citation2016) observed similar results when comparing Δfgcpp1 or ΔΔfgcpp1,2 with WT in point inoculated wheat spikes. Any potential effect of this protein and its expansin-like activity on disease spread could be dampened by more potent effectors secreted by F. graminearum. For example, DON is secreted by F. graminearum during infection and is required for its spread, as evidenced by the inability of DON non-producing mutants to spread through wheat rachis (Proctor et al. Citation1995; Bai et al. Citation2002; Jansen et al. Citation2005; Maier et al. Citation2006).
While FgCPP1-OX1 had increased disease phenotype in the spray inoculated spikes, the same was not observed in point inoculated wheat. In the former, the inoculum is sprayed on the whole spike and the disease evaluation involves a combined assessment of both initial infection and disease spread, where early assessments of infection can offer a better assessment of initial infection. On the contrary, for point inoculation, a specific amount of inoculum is placed in a spikelet and the disease spread alone is assessed. Thus, these two methods of inoculation are designed to assess different aspects of pathogenicity, and the role of a given factor of aggressiveness is not necessarily expected to be the same when comparing the two methods. This dichotomy in the effect of a gene on disease response has been reported in other instances; for example, the disruption of FgTRI5 (trichothecene non-producing mutant) resulted in a strain unable to spread from point inoculated wheat spikelets, but when wheat spikes were sprayed with the same mutant the percentage diseased spikelets within the spike by 18 dpi, was the same when compared with the WT strain (Bai et al. Citation2002). In the case of this study, FgCPP1-OX1 appeared to increase symptoms in spray inoculated spikes, but did not affect disease spread. It could be considered that any possible masking effect by other effectors on the potential role of FgCPP1-OX1 in disease spread could lead to a non-detectable phenotype for this strain in point inoculation.
The FgCPP1-OX1 transformant accumulated significantly higher levels of 15-ADON in axenic culture compared to WT (). This increased trichothecene accumulation by FgCPP1-OX1 was also observed in spray inoculated ‘Penhold’ spikes at 3 dpi, where DON levels were 2.6 times higher compared to WT (). Meanwhile, DON analysis from point inoculated ‘Penhold’ spikelets showed no significant differences in the amount of DON produced by Δfgcpp1 or FgCPP1-OX1 compared to WT at 1 and 3 dpi, whereas both Δfgcpp1 and FgCPP1-OX1 accumulated lower levels of DON at 2 dpi (). These observed changes in DON accumulation by Δfgcpp1 or FgCPP1-OX1 do not correlate with their aggressiveness. While DON is required for disease spread in wheat (Proctor et al. Citation1995; Bai et al. Citation2002), the correlation between DON accumulation levels and disease spread is not clearly understood. Some studies have shown an association between DON accumulation and F. graminearum aggressiveness in terms of disease spread in some wheat genotypes (for example, Foroud et al. Citation2012); however, these were comparisons of different strains which naturally produced different levels of DON.
FgCPP1 is secreted during infection of wheat heads (Paper et al. Citation2007), but its localization and function in planta is unknown. FgCPP1 localization was observed here in agroinfiltrated leaves of N. benthamiana with fluorescent labelled FgCPP1 fusion constructs. FgCPP1-SP protein was distributed in the cytosol and nucleoplasm of N. benthamiana cells (a-ii,b-ii), whereas FgCPP1+ SP was localized to the cytosol, nucleoplasm as well as the chloroplast of tobacco cells (a-iii,b-iii). The absence of FgCPP1+ SP localization to the apoplast was confirmed by plasmolysis of N. benthamiana cells (a-iv,b-iv). This observation was surprising since SsCP1 of S. sclerotiorum and FocCP1 of F. oxysporum f.sp. cubense Tropical Race 4 with their signal peptides were previously shown to be localized to the N. benthamiana apoplast (Yang et al. Citation2018; Liu et al. Citation2019). In our system, the localization of FgCPP1+ SP in the apoplast could depend on the rate of protein exported out from the cytoplasm of the agroinfiltrated cells to the apoplast vs the rate of protein degradation in the apoplast. Such apoplastic degradation of the full-length protein cannot be ruled out as higher levels of breakdown products were observed in protein blots upon expression of FgCPP1+ SP in N. benthamiana ().
Subcellular localization predictions showed that FgCPP1 lacks a predicted chloroplast transit peptide. It should be noted that chloroplast localization was only observed when expressing FgCPP1+ SP, and not FgCPP1-SP. Comparison of protein blots for FgCPP1+ SP and FgCPP1-SP showed more breakdown products for the former () and the possibility of these breakdown products entering and fluorescing in the chloroplast cannot be ruled out. Nevertheless, many bacterial and a few fungal effectors are localized to the chloroplast and some of these effectors are known to target chloroplast proteins (reviewed in Kretschmer et al. Citation2019). While the subcellular localization of FgCPP1 protein in cereals during infection may be different from what was observed here in N. benthamiana, results from the transient expression in N. benthamiana cells may provide valuable insights into the subcellular destination of FgCPP1 protein and could lead to the future discovery of interacting partner(s) in planta and its function during infection.
In summary, a secreted FgCPP1 protein with a reduced abundance in the secretome of a non-pathogenic Δtri6 mutant of GZ3639 strain of F. graminearum was characterized herein. In planta localization of this protein did not reveal its presence in the apoplast as might be expected, and while protein degradation in the apoplast cannot be ruled out, both the FgCPP1+ SP and FgCPP1-SP signals were detected in the cytosol and nucleoplasm of tobacco cells. FgCPP1 disruption did not affect macroconidial germination or mycelial growth of F. graminearum, nor did it influence DON accumulation and aggressiveness. This body of work represents the first study of FgCPP1 overexpression. Previous reports on FgCPP1 knockout mutants did not reveal any change in host-infection, as was also observed here, but overexpression of this gene did result in a significant increase in DON accumulation and disease in each of the three wheat cultivars assessed (‘Roblin’, ‘Penhold’ and ‘Awesome’), in an experiment that was replicated three times. That said, when the experiment was repeated in ‘Penhold’, neither FgCPP1-OX1 nor -OX2 showed any change in disease. This discrepancy in results is likely related to the fact that FHB disease assays can be variable in nature, and the biological significance of small but statistically significant differences in disease data should be interpreted with caution. Finally, while the results presented herein do not demonstrate an obvious role of FgCPP1-OX1 in FHB disease, based on the cumulative data available on this gene, it is possible that the FgCPP1 has a minor and potentially redundant role in contributing to FHB disease.
AE_Revised_Supplementary_Figures.docx
Download MS Word (918.1 KB)Acknowledgements
Dr. Daria Ryabova provided technical assistance for some aspects of this work. The wheat cultivars ‘Penhold’, ‘Awesome’ and ‘Tenacious’ were provided by Dr. Harpinder Randhawa at AAFC, Lethbridge.
Disclosure statement
No potential conflict of interest was reported by the author(s).
Supplementary material
Supplemental data for this article can be accessed online here: https://doi.org/10.1080/07060661.2022.2044910
Additional information
Funding
References
- Almagro Armenteros JJ, Tsirigos KD, Sønderby CK, Petersen TN, Winther O, Brunak S, von Heijne G, Nielsen H. 2019. SignalP 5.0 improves signal peptide predictions using deep neural networks. Nat Biotechnol. 37(4):420–423. doi:https://doi.org/10.1038/s41587-019-0036-z.
- Baccelli I, Luti S, Bernardi R, Scala A, Pazzagli L. 2014. Cerato-platanin shows expansin-like activity on cellulosic materials. Appl Microbiol Biotechnol. 98(1):175–184. doi:https://doi.org/10.1007/s00253-013-4822-0.
- Bai GH, Desjardins AE, Plattner RD. 2002. Deoxynivalenol-nonproducing Fusarium graminearum causes initial infection, but does not cause disease spread in wheat spikes. Mycopathologia. 153(2):91–98. doi:https://doi.org/10.1023/A:1014419323550.
- Barsottini MRO, de Oliveira JF, Adamoski D, Teixeira PJPL, Do Prado PFV, Tiezzi HO, Sforça ML, Cassago A, Portugal RV, de Oliveira PSL, et al. 2013. Functional diversification of cerato-platanins in Moniliophthora perniciosa as seen by differential expression and protein function specialization. Mol Plant Microbe Interact. 26(11):1281–1293. doi:https://doi.org/10.1094/MPMI-05-13-0148-R.
- Beauchemin C, Laliberté JF. 2007. The poly(A) binding protein is internalized in virus-induced vesicles or redistributed to the nucleolus during turnip mosaic virus infection. J Virol. 81(20):10905–10913. doi:https://doi.org/10.1128/JVI.01243-07.
- Boddi S, Comparini C, Calamassi R, Pazzagli L, Cappugi G, Scala A. 2004. Cerato-platanin protein is located in the cell walls of ascospores, conidia and hyphae of Ceratocystis fimbriata f. sp. platani. FEMS Microbiol Lett. 233(2):341–346. doi:https://doi.org/10.1111/j.1574-6968.2004.tb09501.x.
- Boodley JW, Sheldrake R. 1972. Cornell peat-lite mixes for commercial plant growing. Cornell Univ Coop Ext Div Info Bull. 43:1-8.
- Brotman Y, Briff E, Viterbo A, Chet I. 2008. Role of swollenin, an expansin-like protein from Trichoderma, in plant root colonization. Plant Physiol. 147(2):779–789. doi:https://doi.org/10.1104/pp.108.116293.
- Brown PD, Randhawa HS, Fetch JM, Meiklejohn M, Fox SL, Humphreys DG, Green D, Wise I, Fetch T, Gilbert J, et al. 2015. AAC Tenacious red spring wheat. Can J Plant Sci. 95(4):805–810. doi:https://doi.org/10.4141/cjps-2015-011.
- Campbell AB, Czarnecki E. 1987. Roblin hard red spring wheat. Can J Plant Sci. 67(3):803–804. doi:https://doi.org/10.4141/cjps87-108.
- Chen X, Ishida N, Todaka N, Nakamura R, Maruyama J, Takahashi H, Kitamoto K. 2010. Promotion of efficient saccharification of crystalline cellulose by Aspergillus fumigatus Swo1. Appl Environ Microbiol. 76(8):2556–2561. doi:https://doi.org/10.1128/AEM.02499-09.
- Chen D, Wang Y, Zhou X, Wang Y, Xu JR, Wilson RA. 2014. The Sch9 kinase regulates conidium size, stress responses, and pathogenesis in Fusarium graminearum. PLoS One. 9(8):e105811. doi:https://doi.org/10.1371/journal.pone.0105811.
- Cuthbert RD, DePauw RM, Knox RE, Singh AK, McCaig TN, McCallum B, Fetch T, Beres BL. 2017. AAC penhold Canada prairie spring red wheat. Can J Plant Sci. 98:207–214.
- de Oliveira AL, Gallo M, Pazzagli L, Benedetti CE, Cappugi G, Scala A, Pantera B, Spisni A, Pertinhez TA, Cicero DO. 2011. The structure of the elicitor Cerato-platanin (CP), the first member of the CP fungal protein family, reveals a double ψβ-barrel fold and carbohydrate binding. J Biol Chem. 286(20):17560–17568. doi:https://doi.org/10.1074/jbc.M111.223644.
- Feng Q, Gao X, An B, He C, Wang Q. 2021. Two cerato-platanin proteins FocCP1 interact with MaPR1 and contribute to virulence of Fusarium oxysporum f. sp. cubense to banana. J Plant Interact. 16(1):238–245. doi:https://doi.org/10.1080/17429145.2021.1928305.
- Foroud NA, Baines D, Gagkaeva TY, Thakor N, Badea A, Steiner B, Bürstmayr M, Bürstmayr H. 2019a. Trichothecenes in Cereal Grains – an Update. Toxins. 11(11):634. doi:https://doi.org/10.3390/toxins11110634.
- Foroud NA, McCormick SP, MacMillan T, Badea A, Kendra DF, Ellis BE, Eudes F. 2012. Greenhouse studies reveal increased aggressiveness of emergent Canadian Fusarium graminearum chemotypes in wheat. Plant Dis. 96(9):1271–1279. doi:https://doi.org/10.1094/PDIS-10-11-0863-RE.
- Foroud NA, Pordel R, Goyal RK, Ryabova D, Eranthodi A, Chatterton S, Kovalchuk I. 2019b. Chemical activation of the ethylene signaling pathway promotes Fusarium graminearum resistance in detached wheat heads. Phytopathology®. 109(5):796–803. doi:https://doi.org/10.1094/PHYTO-08-18-0286-R.
- Frandsen RJ, Andersson JA, Kristensen MB, Giese H. 2008. Efficient four fragment cloning for the construction of vectors for targeted gene replacement in filamentous fungi. BMC Mol Biol. 9(1):70. doi:https://doi.org/10.1186/1471-2199-9-70.
- Frandsen RJ, Frandsen M, Giese H. 2012. Targeted gene replacement in fungal pathogens via Agrobacterium tumefaciens- mediated transformation. Methods Mol Biol. 835:17–45.
- Frías M, Brito N, González M, González C. 2014. The phytotoxic activity of the cerato-platanin BcSpl1 resides in a two-peptide motif on the protein surface. Mol Plant Pathol. 15(4):342–351. doi:https://doi.org/10.1111/mpp.12097.
- Frías M, González C, Brito N. 2011. BcSpl1, a cerato-platanin family protein, contributes to Botrytis cinerea virulence and elicits the hypersensitive response in the host. New Phytol. 192(2):483–495. doi:https://doi.org/10.1111/j.1469-8137.2011.03802.x.
- Frischmann A, Neudl S, Gaderer R, Bonazza K, Zach S, Gruber S, Spadiut O, Friedbacher G, Grothe H, Seidl-Seiboth V. 2013. Self-assembly at air/water interfaces and carbohydrate binding properties of the small secreted protein EPL1 from the fungus Trichoderma atroviride. J Biol Chem. 288(6):4278–4287. doi:https://doi.org/10.1074/jbc.M112.427633.
- Georgelis N, Tabuchi A, Nikolaidis N, Cosgrove DJ. 2011. Structure-function analysis of the bacterial expansin EXLX1. J Biol Chem. 286(19):16814–16823. doi:https://doi.org/10.1074/jbc.M111.225037.
- Goswami RS, Kistler HC. 2004. Heading for disaster: fusarium graminearum on cereal crops. Mol Plant Pathol. 5(6):515–525. doi:https://doi.org/10.1111/j.1364-3703.2004.00252.x.
- Jansen C, von Wettstein D, Schäfer W, Kogel K-H, Felk A, Maier FJ. 2005. Infection patterns in barley and wheat spikes inoculated with wild-type and trichodiene synthase gene disrupted Fusarium graminearum. Proc Natl Acad Sci U S A. 102(46):16892–16897. doi:https://doi.org/10.1073/pnas.0508467102.
- Jeong JS, Mitchell TK, Dean RA. 2007. The Magnaporthe grisea snodprot1 homolog, MSP1, is required for virulence. FEMS Microbiol Lett. 273(2):157–165. doi:https://doi.org/10.1111/j.1574-6968.2007.00796.x.
- Jiang C, Hei R, Yang Y, Zhang S, Wang Q, Wang W, Zhang Q, Yan M, Zhu G, Huang P, et al. 2020. An orphan protein of Fusarium graminearum modulates host immunity by mediating proteasomal degradation of TaSnRK1α. Nat Commun. 11(1):4382. doi:https://doi.org/10.1038/s41467-020-18240-y.
- Kerff F, Amoroso A, Herman R, Sauvage E, Petrella S, Filée P, Charlier P, Joris B, Tabuchi A, Nikolaidis N, et al. 2008. Crystal structure and activity of Bacillus subtilis YoaJ (EXLX1), a bacterial expansin that promotes root colonization. Proc Natl Acad Sci U S A. 105(44):16876–16881. doi:https://doi.org/10.1073/pnas.0809382105.
- Kim K-T, Jeon J, Choi J, Cheong K, Song H, Choi G, Kang S, Lee Y-H. 2016. Kingdom-wide analysis of fungal small secreted proteins (SSPs) reveals their potential role in host association. Front Plant Sci. 7:186. doi:https://doi.org/10.3389/fpls.2016.00186.
- Kretschmer M, Damoo D, Djamei A, Kronstad J. 2019. Chloroplasts and plant immunity: where are the fungal effectors? Pathogens. 9(1):19. doi:https://doi.org/10.3390/pathogens9010019.
- Letunic I, Doerks T, Bork P. 2015. SMART: recent updates, new developments and status in 2015. Nucleic Acids Res. 43(D1):257–260. doi:https://doi.org/10.1093/nar/gku949.
- Liu S, Wu B, Yang J, Bi F, Dong T, Yang Q, Hu C, Xiang D, Chen H, Huang H, et al. 2019. A cerato-platanin family protein FocCP1 is essential for the penetration and virulence of Fusarium oxysporum f. sp. cubense tropical race 4. Int J Mol Sci. 20(15):3785. doi:https://doi.org/10.3390/ijms20153785.
- Lu S, Edwards MC. 2016. Genome-wide analysis of small secreted cysteine-rich proteins identifies candidate effector proteins potentially involved in Fusarium graminearum −wheat interactions. Phytopathology. 106(2):166–176. doi:https://doi.org/10.1094/PHYTO-09-15-0215-R.
- Luti S, Martellini F, Bemporad F, Mazzoli L, Paoli P, Pazzagli L, Riggs PD. 2017. A single amino acid mutation affects elicitor and expansins-like activities of cerato-platanin, a non-catalytic fungal protein. PLoS One. 12(5):e0178337. doi:https://doi.org/10.1371/journal.pone.0178337.
- Maier FJ, Miedaner T, Hadeler B, Felk A, Salomon S, Lemmens M, Kassner H, Schäfer W. 2006. Involvement of trichothecenes in fusarioses of wheat, barley and maize evaluated by gene disruption of the trichodiene synthase (Tri5) gene in three field isolates of different chemotype and virulence. Mol Plant Pathol. 7(6):449–461. doi:https://doi.org/10.1111/j.1364-3703.2006.00351.x.
- Miller JD, Blackwell BA. 1986. Biosynthesis of 3-acetyldeoxynivalenol and other metabolites by Fusarium culmorum HLX 1503 in a stirred jar fermentor. Can J Bot. 64(1):1–5. doi:https://doi.org/10.1139/b86-001.
- Montenegro Alonso AP, Ali S, Song X, Linning R, Bakkeren G. 2020. UhAVR1, an HR-triggering avirulence effector of Ustilago hordei, is secreted via the ER–Golgi pathway, localizes to the cytosol of Barley cells during in planta-expression, and contributes to virulence early in infection. J Fungi (Basel). 6(3):178. doi:https://doi.org/10.3390/jof6030178.
- Nakagawa T, Suzuki T, Murata S, Nakamura S, Hino T, Maeo K, Tabata R, Kawai T, Tanaka K, Niwa Y, et al. 2007. Improved gateway binary vectors: high-performance vectors for creation of fusion constructs in transgenic analysis of plants. Biosci Biotechnol Biochem. 71(8):2095–2100. doi:https://doi.org/10.1271/bbb.70216.
- Nasmith CG, Walkowiak S, Wang L, Leung WW, Gong Y, Johnston A, Harris LJ, Guttman DS, Subramaniam R, Tyler B. 2011. Tri6 is a global transcription regulator in the phytopathogen Fusarium graminearum. PLoS Pathog. 7(9):e1002266. doi:https://doi.org/10.1371/journal.ppat.1002266.
- Nelson BK, Cai X, Nebenführ A. 2007. A multicolored set of in vivo organelle markers for co-localization studies in Arabidopsis and other plants. Plant J. 51(6):1126–1136. doi:https://doi.org/10.1111/j.1365-313X.2007.03212.x.
- Pan Y, Wei J, Yao C, Reng H, Gao Z. 2018. SsSm1, a Cerato-platanin family protein, is involved in the hyphal development and pathogenic process of Sclerotinia sclerotiorum. Plant Sci. 270:37–46. doi:https://doi.org/10.1016/j.plantsci.2018.02.001.
- Paper JM, Scott-Craig JS, Adhikari ND, Cuomo CA, Walton JD. 2007. Comparative proteomics of extracellular proteins in vitro and in planta from the pathogenic fungus Fusarium graminearum. Proteomics. 7(17):3171–3183. doi:https://doi.org/10.1002/pmic.200700184.
- Pazzagli L, Cappugi G, Manao G, Camici G, Santini A, Scala A. 1999. Purification, characterization, and amino acid sequence of cerato-platanin, a new phytotoxic protein from Ceratocystis fimbriata f. sp. platani. J Biol Chem. 274(35):24959–24964. doi:https://doi.org/10.1074/jbc.274.35.24959.
- Pestka J. 2010. Toxicological mechanisms and potential health effects of deoxynivalenol and nivalenol. World Mycotoxin J. 3(4):323–347. doi:https://doi.org/10.3920/WMJ2010.1247.
- Pfaffl MW, Horgan GW, Dempfle L. 2002. Relative expression software tool (REST©) for group-wise comparison and statistical analysis of relative expression results in real-time PCR. Nucleic Acids Res. 30(9):e36. doi:https://doi.org/10.1093/nar/30.9.e36.
- Pinton P, Braicu C, Nougayrede J-P, Laffitte J, Taranu I, Oswald IP. 2010. Deoxynivalenol impairs porcine intestinal barrier function and decreases the protein expression of claudin-4 through a mitogen-activated protein kinase-dependent mechanism. J Nutr. 140(11):1956–1962. doi:https://doi.org/10.3945/jn.110.123919.
- Proctor RH, Hohn TM, McCormick SP. 1995. Reduced Virulence of Gibberella zeae caused by disruption of a trichothecene toxin biosynthetic gene. Mol Plant Microbe Interact. 8(4):593–601. doi:https://doi.org/10.1094/MPMI-8-0593.
- Quarantin A, Castiglioni C, Schäfer W, Favaron F, Sella L. 2019. The Fusarium graminearum cerato-platanins loosen cellulose substrates enhancing fungal cellulase activity as expansin-like proteins. Plant Physiol Biochem. 139:229–238. doi:https://doi.org/10.1016/j.plaphy.2019.03.025.
- Quarantin A, Glasenapp A, Schäfer W, Favaron F, Sella L. 2016. Involvement of the Fusarium graminearum cerato-platanin proteins in fungal growth and plant infection. Plant Physiol Biochem. 109:220–229. doi:https://doi.org/10.1016/j.plaphy.2016.10.001.
- Rampitsch C, Day J, Subramaniam R, Walkowiak S. 2013. Comparative secretome analysis of Fusarium graminearum and two of its non-pathogenic mutants upon deoxynivalenol induction in vitro. Proteomics. 13(12–13):1913–1921. doi:https://doi.org/10.1002/pmic.201200446.
- Rep M. 2005. Small proteins of plant-pathogenic fungi secreted during host colonization. FEMS Microbiol Lett. 253(1):19–27. doi:https://doi.org/10.1016/j.femsle.2005.09.014.
- Rittenour WR, Harris SD, Yu J-H. 2013. Glycosylphosphatidylinositol-anchored proteins in Fusarium graminearum: inventory, variability, and virulence. PLoS One. 8(11):e81603. doi:https://doi.org/10.1371/journal.pone.0081603.
- Rocha O, Ansari K, Doohan FM. 2005. Effects of trichothecene mycotoxins on eukaryotic cells: a review. Food Addit Contam. 22(4):369–378. doi:https://doi.org/10.1080/02652030500058403.
- Rossi V, Terzi V, Moggi F, Morcia C, Faccioli P, Haidukowski M, Pascale M. 2007. Assessment ofFusariuminfection in wheat heads using a quantitative polymerase chain reaction (qPCR) assay. Food Addit Contam. 24(10):1121–1130. doi:https://doi.org/10.1080/02652030701551818.
- Sampedro J, Cosgrove DJ. 2005. The expansin superfamily. Genome Biol. 6(12):242. doi:https://doi.org/10.1186/gb-2005-6-12-242.
- Sinha RC, Savard ME, Lau R. 1995. Production of monoclonal antibodies for the specific detection of deoxynivalenol and 15-acetyldeoxynivalenol by ELISA. J Agric Food Chem. 43(6):1740–1744. doi:https://doi.org/10.1021/jf00054a061.
- Sobrova P, Adam V, Vasatkova A, Beklova M, Zeman L, Kizek R. 2010. Deoxynivalenol and its toxicity. Interdiscip Toxicol. 3(3):94–99. doi:https://doi.org/10.2478/v10102-010-0019-x.
- Spolti P, Barros NC, Gomes LB, dos Santos J, Del Ponte EM. 2012. Phenotypic and pathogenic traits of two species of the Fusarium graminearum complex possessing either 15-ADON or NIV genotype. Eur J Plant Pathol. 133(3):621–629. doi:https://doi.org/10.1007/s10658-012-9940-5.
- Tendulkar SR, Gupta A, Chattoo BB. 2003. A simple protocol for isolation of fungal DNA. Biotechnol Lett. 25(22):1941–1944. doi:https://doi.org/10.1023/B:BILE.0000003990.27624.04.
- van Engelen FA, Molthoff JW, Conner AJ, Nap J-P, Pereira A, Stiekema WJ. 1995. pBINPLUS: an improved plant transformation vector based on pBIN19. Transgenic Res. 4(4):288–290. doi:https://doi.org/10.1007/BF01969123.
- Voigt CA, Schäfer W, Salomon S. 2005. A secreted lipase of Fusarium graminearum is a virulence factor required for infection of cereals. Plant J. 42(3):364–375. doi:https://doi.org/10.1111/j.1365-313X.2005.02377.x.
- Voigt CA, von Scheidt B, Gácser A, Kassner H, Lieberei R, Schäfer W, Salomon S. 2007. Enhanced mycotoxin production of a lipase-deficient Fusarium graminearum mutant correlates to toxin-related gene expression. Eur J Plant Pathol. 117(1):1–12. doi:https://doi.org/10.1007/s10658-006-9063-y.
- Wang Y, Wu J, Kim SG, Tsuda K, Gupta R, Park SY, Kim ST, Kang KY. 2016. Magnaporthe oryzae -secreted protein MSP1 induces cell death and elicits defense responses in rice. Mol Plant Microbe Interact. 29(4):299–312. doi:https://doi.org/10.1094/MPMI-12-15-0266-R.
- Wilson LM, Idnurm A, Howlett BJ. 2002. Characterization of a gene (sp1) encoding a secreted protein from Leptosphaeria maculans, the blackleg pathogen of Brassica napus. Mol Plant Pathol. 3(6):487–493. doi:https://doi.org/10.1046/j.1364-3703.2002.00144.x.
- Yan Z, Ming-Xiong H, Bo W, Qi-Chun H, Qing L, Jian Z. 2012. Recombinant EXLX1 from Bacillus subtilis for enhancing enzymatic hydrolysis of corn stover with low cellulase loadings. Afr J Biotechnol. 11:11126–11131.
- Yang G, Tang L, Gong Y, Xie J, Fu Y, Jiang D, Li G, Collinge DB, Chen W, Cheng J. 2018. A cerato-platanin protein SsCP1 targets plant PR1 and contributes to virulence of Sclerotinia sclerotiorum. New Phytol. 217(2):739–755. doi:https://doi.org/10.1111/nph.14842.
- Yennawar NH, Li L-C, Dudzinski DM, Tabuchi A, Cosgrove DJ. 2006. Crystal structure and activities of EXPB1 (Zea m 1), a beta-expansin and group-1 pollen allergen from maize. Proc Natl Acad Sci U S A. 103(40):14664–14671. doi:https://doi.org/10.1073/pnas.0605979103.
- Zhang Y, Gao Y, Liang Y, Dong Y, Yang X, Yuan J, Qiu D. 2017a. The Verticillium dahliae SnodProt1-like protein VdCP1 contributes to virulence and triggers the plant immune system. Front Plant Sci. 8:1880. doi:https://doi.org/10.3389/fpls.2017.01880.
- Zhang Y, Liang Y, Qiu D, Yuan J, Yang X. 2017b. Comparison of cerato-platanin family protein BcSpl1 produced in Pichia pastoris and Escherichia coli. Protein Expr Purif. 136:20–26. doi:https://doi.org/10.1016/j.pep.2017.06.004.