Abstract
Blackleg, caused by Leptosphaeria maculans, is an important disease of canola, managed primarily through host--plant resistance. Fungal avirulence (AvrLm) genes code for effectors recognized by major host--plant resistance (Rlm) genes. AvrLm4-7 is predominant in the pathogen population in Oklahoma and Kansas, USA, and interacts with the Rlm4 and Rlm7 resistance genes. High-resolution melting analysis and sequencing were used to evaluate the genetic variability of AvrLm4-7 in avirulent (A) and virulent (a) reference isolates, and in isolates (n = 67) collected in Oklahoma from 2014 to 2017. Melting analysis of PCR products from the central primers (493 bp) and from new upstream external primers (105 bp) produced melting profiles that differed among avirulent (AvrLm4-AvrLm7 (A4A7)) and virulent (avrLm4-AvrLm7 (a4A7) and (avrLm4-avrLm7 (a4a7)) pathogen genotypes. Genotypic classification by melting analysis was supported by pathogenicity scores on seedling cotyledons of susceptible, Rlm4, and Rlm7 differentials. All of the local isolates were a4A7 genotypes. In the sequences of a4A7 reference isolates, all showed the expected mutation at bp 358 of C to G resulting in virulence on Rlm4, and silent mutations of either C to T at bp 256 or A to G at bp 134. These two haplotypes displayed unique melting profiles from the central primers in 64% and 36% of local isolates, respectively. Only the central primers produced pathotype-specific melting curves. Sustainable deployment of Rlm7 canola is needed for effective management of blackleg in the region. High-resolution melting will be useful to monitor allelic variability in AvrLm4-7 and for rapid detection of a4a7 strains.
Résumé
La nécrose du collet, causée par Leptosphaeria maculans, est une importante maladie du canola qui se gère principalement grâce à la résistance de la plante hôte. Les gènes d’avirulence fongiques (AvrLm) codent pour les effecteurs reconnus par les principaux gènes de résistance des plantes hôtes (Rlm). AvrLm4-7 prédomine dans les populations d’agents pathogènes de l’Oklahoma et du Kansas, aux États-Unis, et interagit avec les gènes de résistance Rlm4 et Rlm7. La fusion à haute résolution et le séquençage ont été utilisés pour évaluer la variabilité génétique d’AvrLm4-7 chez les isolats de référence avirulents (A) et virulents (a) ainsi que chez les isolats (n = 67) collectés en Oklahoma de 2014 à 2017. L’analyse de la fusion des produits de la PCR, situés sur les amorces centrales (493 bp) et sur de nouvelles amorces externes situées en amont (105 bp), a produit des profils de fusion qui ont varié chez les génotypes des agents pathogènes avirulents (AvrLm4-AvrLm7 [A4A7]) et virulents (avrLm4-AvrLm7 [a4A7] et avrLm4-avrLm7 [a4a7]). La classification génotypique par fusion a été appuyée par les scores de pathogénicité obtenus des cotylédons de plantules de différentiels sensibles Rlm4 et Rlm7. Tous les isolats locaux étaient des génotypes d’a4A7. Dans les séquences des isolats de référence d’a4A7, tous ont affiché la mutation attendue à la bp 358 de C à G, provoquant la virulence à l’égard de Rlm4, et des mutations silencieuses de C ou T à la bp 256 ou de A ou G à la bp 134. Ces deux haplotypes ont affiché des profils de fusion uniques issus des amorces centrales chez 64% et 36% des isolats locaux, respectivement. Le déploiement durable du canola porteur du gène Rlm7 est requis pour gérer efficacement la nécrose du collet dans la région. La fusion à haute résolution sera utile pour suivre la variabilité allélique chez AvrLm4-7 et pour détecter rapidement les souches d’a4a7.
Introduction
The fungus Leptosphaeria maculans (Desm.) Ces. & DeNot, causes blackleg, one of the most important diseases of canola (Brassica napus) worldwide (West et al. Citation2001). The basal stem cankers, which develop late in the season, can result in up to 50% yield losses (Rouxel and Balesdent Citation2005). Therefore, managing blackleg is essential to limit losses. Genetic resistance is an effective control for this disease (Kutcher et al. Citation2010). Major-gene resistance (Flor Citation1971) in B. napus is mostly based on the recognition of leucine-rich repeat domain proteins encoded by resistance genes (R) in B. napus and small proteins secreted by the pathogen, also known as effectors, resulting in effector-triggered immunity (Hammond-Kosack and Jones Citation1997). The immunity produces a hypersensitive response (HR) or cell death at the infection site preventing the systemic invasion of the plant by the pathogen (Jones and Dangl Citation2006). Recently, a wall-associated kinase-like protein encoded by Rlm9 has been described as an additional mechanism of race-specific resistance (Larkan et al. Citation2020). In L. maculans, 17 effectors encoded by avirulence genes (AvrLm) have been identified and AvrLm1, AvrLm2, AvrLm3, AvrLm4-7, AvrLm5-9, AvrLm6, AvrLm10, AvrLm11, AvrLmS/AvrLep2, and AvrLm14 have been cloned. Several of these avirulence genes reside in two separate clusters consisting of AvrLm1-2-6 and AvrLm3-4-7-9-LepR1 (Balesdent et al. Citation2002; Marcroft et al. Citation2012; Ghanbarnia et al. Citation2015, Citation2018; Fudal et al. Citation2007; Gout et al. Citation2006; Plissonneau et al. Citation2016, Citation2018; Fernando et al. Citation2018; Petit‐Houdenot et al. Citation2019; Neik et al. Citation2022; Degrave et al. Citation2021). In Brassica spp., 18 major resistance genes consisting of Rlm1 to Rlm11, RlmS, LepR1 to LepR4, BLMR1, and BLMR2 have been identified but only Rlm2, Rlm9, and LepR3 have been cloned (Marcroft et al. Citation2012; Fernando et al. Citation2018; Larkan et al. Citation2020).
The use of genetic resistance in canola cultivars can reduce the impact of blackleg (Brun et al. Citation2010). However, deletions or mutations of avirulence genes can lead to loss of corresponding R gene effectiveness (Van de Wouw et al. Citation2010; Marcroft et al. Citation2012). The rapid loss of R gene effectiveness has been reported in Australia where cultivars harbouring RlmS became susceptible (Sprague et al. Citation2006) and in France when Rlm1 was overcome three years after it was deployed (Rouxel et al. Citation2003). A notable exception has been Rlm7 which has remained effective for over 10 years in Europe when combined with multigenic (quantitative) resistance to stem canker in adult plants (Brun et al. Citation2010; Plissonneau et al. Citation2017).
The AvrLm4-7 effector was the first in this host--pathogen interaction found to confer dual recognition of resistance genes Rlm4 and Rlm7 (Parlange et al. Citation2009). A point mutation in AvrLm4-7 caused by a change from a glycine to an arginine residue in the effector is responsible for the loss of recognition by Rlm4 and virulence. Fortunately, this mutation does not affect Rlm7 recognition (Blondeau et al. Citation2015; Parlange et al. Citation2009) and Rlm7 remains effective against avrLm4/AvrLm7 isolates. Alleles of AvrLm4-7 that include avirulence on both genes (A4A7), virulence on Rlm4 (a4A7), and virulence on both genes (a4a7) have been reported on winter canola in Europe (Stachowiak et al. Citation2006; Plissonneau et al. Citation2017). Another avirulence gene with dual recognition is AvrLm1, recognized by resistance genes LepR3 and Rlm1 that reside in distinct loci (Larkan et al. Citation2014, Citation2016).
AvrLm4-7 also interacts with other L. maculans effectors. For example, the resistance gene Rlm3 is unable to recognize AvrLm3 in the presence of AvrLm4-7 because of their related linkage (Plissonneau et al. Citation2016). Recognition of AvrLm9 by Rlm9 is also suppressed by the AvrLm4-7 effector (Ghanbarnia et al. Citation2018). These avirulence genes form a group located in supercontig 12 of the L. maculans genome that is distinct from other Avr genes (Plissonneau et al. Citation2016; Ghanbarnia et al. Citation2018).
In Oklahoma and Kansas in the U.S. southern Great Plains where winter canola is grown, AvrLm4-7 is a prevalent avirulence gene, being found in nearly all isolates sampled from 2009 to 2013 (Diaz et al. Citation2019). Based on genotyping with PCR amplification, AvrLm4-7 is also found at high frequencies in Europe, Australia, and Canada (Patel et al. Citation2015; Rouxel and Balesdent Citation2017; Fernando et al. Citation2018). Pathogenicity tests along with PCR amplification enables the identification of virulent (a4A7 and a4A7) alleles. Based on pathogenicity to the cultivar ‘Jet Neuf’ (Rlm4) and PCR, the a4A7 allele is predominant in the region suggesting Rlm7 would be highly effective in Oklahoma and Kansas much like in Europe. While ‘Jet Neuf’ was susceptible to the 2009–2013 local isolates on seedling cotyledons, the cultivar is an important source of quantitative resistance to blackleg (Delourme et al. Citation2006) and is in the pedigree of cultivars grown in the U.S. southern Great Plains (Rife et al. Citation2001). Therefore, changes to the AvrLm4-7 gene have important ramifications for disease management and cultivar selection by farmers in the region.
A rapid method for avirulence allele profiling would facilitate the monitoring of virulence changes (Van de Wouw et al. Citation2018). Pathogenicity tests on differential B. napus genotypes that harbour either the resistance gene Rlm4 or Rlm7 and the amplification of the AvrLm4-7 gene by PCR are currently required for the identification and characterization of AvrLm4-7. However, the end point PCR is not able to discriminate isolates that have lost Rlm4 recognition (Carpezat et al. Citation2014). Furthermore, intermediate phenotypes can occur on the Rlm4 differentials ‘Jet Neuf’ and ‘Pixel’, leading to inconclusive genotyping. The atypical (non-hypersensitive) resistant response is independent of PCR amplification of the AvrLm4 gene (Dilmaghani et al. Citation2009).
High-resolution melting is a post-PCR technique that differentiates PCR amplicons based on their melting temperature measured when the DNA is denaturing from double stranded to single stranded by an increase in temperature (Wittwer Citation2009). This technique has been applied for genotyping and mutation scanning with a high degree of sensitivity (Wittwer et al. Citation2003; Erali et al. Citation2008). Carpezat et al. (Citation2014) used high-resolution melting analysis of a large fragment in the central region of the AvrLm4-7 gene. Genotyping by melting analysis of the fragment was used to classify the virulence profiles of isolates on Rlm4 and Rlm7 that was 93% in agreement with phenotyping on seedling cotyledons. The objective of this study was to assess the genetic variability of the AvrLm4-7 gene among L. maculans reference isolates using pathogenicity and molecular techniques including high-resolution melting analysis, restriction fragment length polymorphisms, and sequencing. Techniques were then validated on a set of unknown isolates collected in Oklahoma for the continued monitoring of avirulence genotypes.
Materials and methods
Fungal isolates
Fourteen L. maculans isolates with known avirulence gene profiles (races) were used as reference isolates (). Two of the isolates were previously collected in Oklahoma, phenotyped for AvrLm1 to AvrLm4 on seedling cotyledons, and genotyped for AvrLm6 and AvrLm4-7 by PCR assay (Diaz et al. Citation2019). The presence of additional avirulence genes in the reference isolates from Oklahoma were evaluated using KASP markers, PCR + RFLP, and differential cultivars (). The other isolates, some of which were part of the International Blackleg Crucifer Network (IBCN) collection, were provided by Dr. A. Van de Wouw, School of Botany, The University of Melbourne, Australia. The race of each isolate was previously identified by pathogenicity on seedling cotyledons using differential cultivars with known Rlm genes and by PCR amplification of AvrLm1, AvrLm6, and AvrLm4-7 genes (Balesdent et al. Citation2005; Marcroft et al. Citation2012). Three of the double virulent (a4a7) isolates had Random Insertion Point (RIP)-like mutations of from 39 to 43 bp mutations that generated nine stop codons within each allele (Van de Wouw and Howlet Citation2012). One of the virulent isolates (a4A7) had point mutations of G to A at bp256 (G256→A256) in addition to the G358→C358 mutation common to all a4A7 isolates (Van de Wouw and Howlet Citation2012) (). An additional 67 isolates were obtained from winter canola leaves with blackleg symptoms collected in Oklahoma from 2014 to 2017. Single pycnidia were removed from leaves with sporulating lesions with a sterile surgical blade and cultured on V8 juice agar (200 mL L−1 V8 juice clarified with 3 g L−1 CaCO3 + 16 g L−1 agar) amended with 100 mg L−1 streptomycin sulphate (SV8 agar). Isolates were hyphal-tip purified at least twice. For long-term storage, the fungus was grown on V8 juice agar overlaid with sterile filter paper. Filter paper colonized by the fungus was desiccated and small discs were stored in sterile vials at 4°C.
Table 1. Reference isolates of Leptosphaeria maculans used in this study and their race classification.
Pathogenicity tests
Isolates were grown on SV8 agar under continuous artificial light at room temperature (22 to 25°C) for 7 to 8 d. Conidia from a single pycnidium were streaked on YPS agar (for 1 L: 1 g yeast extract, 1 g peptone, 1 g dextrose, 17 g agar, 100 mg penicillin, 100 mg streptomycin) using a sterile loop. YPS plates were incubated for 8 to 10 d as described above and conidial suspensions were harvested in sterile water, filtered through sterile cheesecloth to remove excess mycelia, and adjusted to 106 spores per mL using a haemocytometer.
The 14 reference isolates and the 67 isolates from Oklahoma were evaluated for interaction phenotype on the differential cultivars ‘Westar’ (susceptible) ‘Jet Neuf’ (Rlm4) (Balesdent et al. Citation2005; Dilmaghani et al. Citation2009) and on the ‘Topas’ introgression line with Rlm4 and the susceptible cultivar ‘DH16516’ (Larkan et al. Citation2016). In addition, the reference isolates were evaluated for AvrLm7 on the Rlm7 hybrid ‘DK-Sensei’ (Dekalb.Co., UK). Pregerminated seedlings were transplanted and grown for 7 d in a growth chamber at 24°C, 80% relative humidity (RH), and continuous fluorescent light. Plants received soluble fertilizer at 0.6–0.2-0.4 g L−1 N-P-K, respectively within the first week. Eight plants were inoculated for each isolate and genotype combination. Seedlings were wounded 7 d after transplanting by puncturing each of four cotyledon lobes/plant with a 200-µL micropipette tip. A 10 µL drop of the conidial suspension was applied to each wound. Autoclaved water was used as a control on each differential. After inoculation, plants were kept at 100% RH in the dark in a dew chamber at 25°C for 48 hr. After high-humidity incubation, plants were further incubated in a growth chamber at 24°C, 80% RH, with a 12 hr light/dark cycle. Disease severity was evaluated 10 to 12 d after inoculation using the IMASCORE rating scale which contains six infection classes (IC) where IC1 to IC3 are resistant responses and IC4 to IC6 are susceptible reactions (Balesdent et al. Citation2001). IC1 is a typical hypersensitive response (HR), IC2 is a dark lesion less than 3 mm in diameter, and IC3 represents a larger lesion surrounded by a dark necrotic margin. IC4 to IC5 represent large grey-green lesions. IC4 lesions lack a dark margin and pycnidial formation, IC5 lesions have a few pycnidia while IC6 lesions produce abundant pycnidial formation and leaf necrosis. The isolates were considered virulent when the mean IC disease severity score was ≥ 4, while a disease severity score < 4 was considered avirulent.
PCR detection of avirulence genes
DNA was isolated from cultures grown in V8 broth for 3 wk at room temperature. Mycelial samples (0.7 g) were collected into 2 mL screw cap tubes containing 5-mm diameter stainless steel beads. The capped tubes were first flash frozen with liquid nitrogen and then homogenized in a Cole-Parmer Mini bead beater instrument for 20 seconds. Bead beating was repeated twice. Genomic DNA was extracted using the CTAB protocol described by (Weising et al. Citation1995) as modified by (Orquera-Tornakian et al. Citation2017). A volume of 1 mL of extraction buffer (0.8% CTAB, 140 mM sorbitol, 220 mM Tris-HCl, 22 mM EDTA, 800 mM NaCl, and 1% C12H25NaSO4) was added on each tube. After homogenization, 400 µL of chloroform: isoamyl alcohol (24:1) was incorporated and incubated at 58°C for 30 min with agitation at 500 rpm. Each tube was centrifuged at 12 000 x g at 4°C for 15 min. The supernatant was transferred to new 2 mL centrifuge tubes, and the DNA was precipitated using 750 µL of isopropanol with an incubation of 30 min at 20°C. After incubation, the tubes were centrifuged at 14 000 x g for 8 min, and the upper aqueous phase was discarded. The pellet was air dried and resuspended in 50 µL of TE buffer (1 mM EDTA, 10 mM Tris-HCl).
The avirulence gene AvrLm4-7 was amplified by end point PCR using primers that produced a 783 bp fragment (Parlange et al. Citation2009) (). The total volume of each PCR reaction was 20 µL and consisted of 2 µL of DNA (10 ng µL−1), 6 µL of autoclaved water, 1 µL of each set of primers (5 µM), and 10 µL of GoTaq® Green Master Mix (Promega Co. Road Madison, WI). PCR amplifications were performed using a MastercyclerTM PCR System (Thermo Fisher Scientific, Grand Island, NY) using the following cycling conditions: 3 min at 95°C, followed by 30 cycles of 45s at 95°C, 30s at 60°C and 60s at 72°C, with a final extension of 5 min at 72°C. PCR products were visualized by electrophoresis on a 1.5% agarose gel (60 min at 100 V). The presence or absence of each gene was determined by amplification or not of the expected region. The reference isolates 124 (AvrLm5-6-7-11 (8–10-S), D5 (AvrLm1-2-4-7-S), and D9 (AvrLm5-6-7-8) were used as controls for each reaction.
Table 2. Oligonucleotide primers used in PCR detection of AvrLm4-7 in L. maculans.
The avirulence gene AvrLm4 was evaluated by restriction fragment length polymorphism (RFLP) digestion of unpurified PCR product. The product from primers AVR47intUp/extLo (AvrLm4-7) () was digested with the RFLP enzymeHaelll (New England BioLabs, Inc., Ipswich, MA) as described by Van de Wouw et al. (Citation2018). Each PCR reaction consisted of 1.5 µL of DNA (10 ng µL−1), 8.5 µL of autoclaved water, 1.25 µL of each set of primers (6 µM), and 12.5 µL of GoTaq® Green Master Mix. PCR amplifications with the MastercyclerTM PCR System used the following cycling conditions: 3 min at 95°C, followed by 35 cycles of 30s at 95°C, 30s at 59°C and 60s at 72°C, with a final extension of 6 min at 72°C. After amplification, 24 µL of PCR products were digested by adding 3 µL of NEBuffer (1X), 2.5 µL of autoclaved water, and 0.5 µL (10 U) of the restriction enzyme. The PCR product was digested at 37°C for 15 min. Restriction fragments were visualized by agarose gel electrophoresis as described above. Restriction fragments of the avirulent allele AvrLm4 were 145 and 179 bp, and for the virulent allele avrLm4 was a 324 pb fragment. The reference isolates 124 (AvrLm5-6-7-11 (8–10-S)), D5 (AvrLm1-2-4-7-S), and D9 (AvrLm5-6-7-8), were used as controls for each reaction.
Analysis of the AvrLm4-7 gene
The AvrLm4-7 gene was amplified in isolates () using the specific primers Avr47extUp3 and Avr47extLo () described by (Parlange et al. Citation2009). The polymerase chain reaction (PCR) mix consisted of 2 µL of DNA (10 ng µL−1), 6 µL of autoclaved water, 1 µL of primers forward and reverse (5 µM), and 10 µL of GoTaq® Green Master Mix. PCR amplifications were performed using the MastercyclerTM PCR System under the following conditions: 3 min at 95°C, followed by 30 cycles of 45s at 95°C, 30s at 60°C and 60s at 72°C, with a final extension of 5 min at 72°C. PCR products were visualized by agarose gel electrophoresis as described above. The QIAquick Gel Extraction Kit (Qiagen S.A., Germantown, MD) was used to clean the PCR products prior to submitting them to the Oklahoma State University Recombinant DNA/Protein Resource Facility for sequencing. The sequences were aligned using MEGA 7 software (Kumar et al. Citation2016) and compared to identify polymorphic sites. DNA sequences were translated to their corresponding amino acid sequences using EMBOSS Transeq. A reference amino acid sequence previously described (GenBank: AM998638.1, Parlange et al. Citation2009) was used as a query sequence for the protein sequence alignment.
For the primer design, the sequence of the AvrLm4-7 gene (GenBank: AM998638.1) was retrieved from the National Center for Biotechnology Information (NCBI). The sequences of the internal primers AvrLm7UP1and AvrLm7LOW2 () described by Carpezat et al. (Citation2014) were used to identify the amplicon region in the AvrLm4-7 gene using the software Primer3. Two sequences from the surrounding external regions upstream (5ʹuntranscribed region (UTR)) and downstream (3ʹUTR) from the internal primers were selected and two sets of external primers named AvrLm47-A and AvrLm47-B () were designed using Primer 3 (). The web interface mFold was used to predict in silico the thermodynamics, self-dimer formation, and internal structures of each primer (Zuker Citation2003). The designed set of primers was tested and verified in silico using the Basic Alignment Search Tool (BLAST), and the melting curves were predicted using the software uMELTSM v2.0 (Dwight et al. Citation2011). Finally, two sets of primers (AvrLm47A-F/R and AvrLm7UP1/LOW2) were selected for the high-resolution melting analysis due to the polymorphic sites that were found in these regions.
Fig. 1 Graphical representation of the AvrLm4-7 sequence (GenBank: AM998638.1), showing the location of the primer sets used for high-resolution melting analysis. Internal primers AvrLm7UP1/AvrLm7LOW2 were developed previously by Carpezat et al. (Citation2014), and the external primer sets AvrLm47A-F/AvrLm47A-R and AvrLm47B-F/AvrLm47B-R were design for this study. 5’ and 3’ UTRs are indicated in grey shading. The intron is shown in between black lines (521 to 566 bp). Stars above the nucleotides represent the location of the point mutations found in this study. The translated amino acid sequence is shown below the nucleotide sequence beginning at base pair 118.
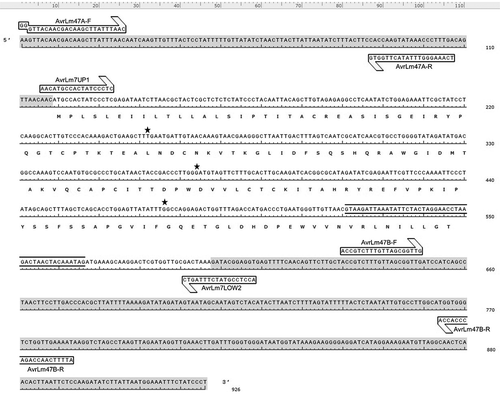
High-resolution melting
PCR amplification and high-resolution melting were performed using a Rotor-GeneTM 6000 real-time rotary analyzer in a 72-tube Rotor-Disc (Qiagen S.A., Germantown, MD). A final volume of 10 µL of the high-resolution melting mix included 1 µL of genomic DNA (10 ng µL−1), 1 µL of autoclaved water, 1 µL of primers forward and reverse (each at 5 µM), 1 µL of LCGreen (BioFire Defence, Salt Lake City, UT), and 5 µL of Hot-Start DNA Polymerase (Thermo Fisher Scientific, Grand Island, NY). The reaction was done with the following cycling conditions: an initial denaturation step at 95°C for 3 min, followed by 30 cycles of 95°C for 45s, 55°C for 30s, 72°C for 1 min, and a final extension at 72°C for 5 min. The melting temperature range after the PCR cycle was from 50°C to 99°C, with an increment of 0.25°C per second. Each reaction included the unknown samples, the positive controls, and one non-template (water) control. Separate PCR-high-resolution melting reactions were performed for the AvrLm47A-F/R primers developed in this study and the previously described primers AvrLm7UP1/LOW2.
High-resolution melting fluorescence and melting temperatures were collected and analyzed using the Rotor-Gene Q series software (Qiagen S.A., Germantown, MD). The melting profiles were exported from the software and analyzed separately. Melting profiles included the derivative curve (-df/dt vs T° where f = fluorescence and t = time) which indicates the melting temperature of each isolate, the normalized fluorescence during the melting transition which allows differentiation of the melting profiles, and the fluorescence difference graph. For fluorescence difference, the data for one melting curve is selected and subtracted for normalization (becoming a horizontal baseline of zero) in the difference plot. Since the fluorescence of the reference genotype is subtracted from each of the samples, the newly generated melting profiles evidence the differences in fluorescence and facilitates the differentiation among isolates (Wittwer et al. Citation2003).
Based on the melting curve shape of each sample, differences among isolates were visually compared with the reference a4a7, a4A7, and A4A7 isolates. High-resolution melting curve profiles of the 67 local isolates collected from 2014 to 2017 were produced for each set of primers and the identification of the various AvrLm4-7 alleles were inferred. The letters B and C were assigned to identify the high-resolution melting curve profiles obtained with the AvrLm47A and AvrLm7UP1/LOW2 primer sets, respectively.
Results
AvrLm4-7 phenotyping and genotyping
Except for isolate D14, which had low conidial production, the previously reported avirulence/virulence phenotypes on differential cultivars and hybrids were confirmed for the reference isolates (). The reference isolates were all virulent on differentials with no Rlm genes (). Isolates that harboured a functional avirulence allele and had the A4A7 phenotype were avirulent on the differential Rlm4 cultivars ‘Jet Neuf’ and ‘Topas-Rlm4’, and the Rlm7 hybrid ‘DK-Sensei’. Isolates that had the virulent a4A7 phenotype, including the two reference isolates from Oklahoma, were virulent on the Rlm4 differentials but avirulent on the Rlm7 hybrid. The isolates that had the double virulent a4a7 phenotype caused a susceptible reaction on the Rlm4 and Rlm7 differentials.
Fig. 2 Cotyledons of ‘Westar’ (No Rlm gene), ‘Jet Neuf’ (Rlm4), and ‘DK-Sensei’ (Rlm7) 11 days after inoculation with conidial suspension of Leptosphaeria maculans isolates with different AvrLm4-7 genotypes, D6 (a4a7), D9 (a4A7), and D5 (A4A7).
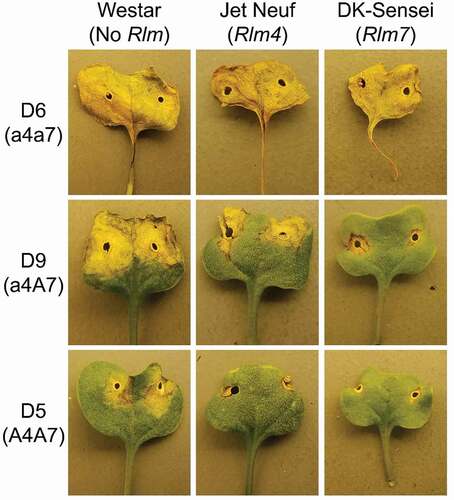
Table 3. Characterization of AvrLm4-7 in reference isolates of Leptosphaeria maculans based on pathogenicity tests on differential canola cultivars and end-point PCR.
The presence of the avirulence gene AvrLm4-7 was also determined by the positive amplification of the end point PCR using the Avr47extUp3/Lo set of primers. The expected 788 bp amplicon of the AvrLm4-7 gene was present for the isolates that either have the avirulent A4A7 phenotype or the Rlm4-virulent a4A7 phenotype (). There was no amplification of the AvrLm4-7 gene for the double virulent a4a7 isolates.
The AvrLm4-7 phenotypes and genotypes of the 67 local isolates were assessed by cotyledon assays on ‘Westar’ and ‘Jet Neuf’ (Rlm4), PCR amplification of AvrLm4-7, and PCR+RFLP (). All isolates were virulent on ‘Westar’. Most isolates (87%) were virulent on ‘Jet Neuf’, had AvrLm4-7 amplification, and produced RFLP bands expected for Rlm4-virulent phenotypes. These isolates were clearly a4A7 phenotypes. There were 9% of isolates that had mean IC ratings of below 4.0 considered virulent but had PCR amplification of AvrLm4-7. Based on their virulent-type Rlm4 RFLP banding patterns, and their lack of strong (HR) resistance (Dilmaghani et al. Citation2009) the intermediate types were considered a4A7 isolates. The remaining isolates (4%) were virulent on ‘Jet Neuf’ but lacked PCR amplification of AvrLm4-7 suggesting they could be double virulent (a4a7) isolates. However, these were avirulent on seedling cotyledons of the Rlm7 hybrid ‘DK Sensei’ (data not shown) and were also considered a4A7 isolates.
Table 4. Classification of AvrLm4-7 alleles in Leptosphaeria maculans isolates collected from winter canola grown in Oklahoma from 2014 to 2017.
A 660 bp segment corresponding to the reference AvrLm4-7 gene was sequenced following amplification with the Avr47extUp3/Lo set of primers. There was no amplification for the double virulent a4a7 reference isolates using PCR and these isolates could not be sequenced (). Following PCR amplification, sequencing, and alignment of the other 10 reference isolates, polymorphic sequences were found in the a4A7 isolates compared to the reference sequence (GenBank: AM998638.1) and the A4A7 reference isolates. Point mutations in the coding sequence were found after the alignment of the reference isolates (, ). A non-synonymous change of guanine to cytosine at bp 358 (G358→C358) from the start of the coding sequence (bp 119 in ) was identified in all a4A7 isolates. This variation corresponds to a change of glycine to arginine (G120→R120) in the amino acid sequence () and confers virulence to Rlm4. The alignment showed a second nucleic acid change in most of the a4A7 reference isolates. This was a non-synonymous substitution of G256→A256. The two reference isolates from Oklahoma showed an additional non-synonymous nucleic acid substitution of T134→C134. All of these point mutations were previously reported by Parlange et al. (Citation2009) and the G256→A256 mutation was prevalent in Australia (Van de Wouw and Howlet Citation2012).
Fig. 3 Protein sequences alignment of reference isolates and the reference a4A7 sequence (AM998634.1) showing the variants of the AvrLm4-7 protein. Isolates virulent and avirulent towards Rlm4 and Rlm7 respectively display the amino acid change of Glycine to Arginine (G120→R120) which is responsible for the loss of avirulence towards Rlm4. Local isolates (102 and 124) show an amino acid change of Leucine to Serine (L45→S45), and reference isolates D8, D9, D16, and D17 display a different amino acid change of Aspartic acid to Asparagine (D86→N86).
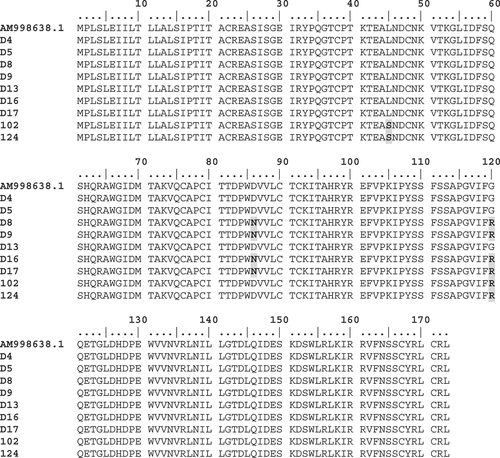
Table 5. PCR amplification and high-resolution melting profiles of the AvrLm4-7 gene in Leptosphaeria maculans isolates.
High-resolution melting analysis
The high-resolution melting curves of the avirulence gene AvrLm4-7 () were characterized in reference isolates. The upstream amplicon of 105 bp comprising the 5ʹUTR was amplified using the primer set AvrLm47A-F/R (). Five different melting curve profiles were observed from B0 to B4 (, ). The AvrLm4-7 gene was not amplified for isolates D6, D7, and D10 using end point PCR. However, high-resolution melting curves were obtained for these isolates, possibly due to the elevated sensitivity of high-resolution melting analysis. The double virulent a4a7 isolates (D6, D7, and D10) had similar melting curves designated B4. The avirulent A4A7 isolates D4 and D5 shared the B0 melting profile. The other avirulent isolate D13 and the a4A7 isolates D14, D16, and D17 had the B2 melting profile. The B1 melting profile was assigned to the a4A7 isolates D8 and D9. The reference isolates 102 and 124 from Oklahoma shared a distinct B3 melting profile. Melting temperatures (Tm) were similar within the B0 to B3 profiles (). The central fragment of AvrLm4-7 () is an amplicon of 493 bp that was produced with the primers AvrLm7UP1/LOW2. The four melting profiles obtained for this AvrLm4-7 gene fragment were designated C0 to C3. Similar to the upstream fragment of the gene, the expected amplicon for double virulent a4a7 isolates was not produced using end point PCR. However, melting curves for avirulent isolates were similar and assigned a melting profile of C3 (; ). The avirulent A4A7 isolate D13 and the a4A7 isolates D8, D9, D14, D16, and D17 had the C1 melting profile. However, the melting temperature displayed by D13 differed slightly (0.3°C) from the virulent a4A7 variants with the same C1 melting profile (). The other avirulent A4A7 isolates D5 and D4 had the C0 melting profile which differed slightly from the C1 profile. The a4A7 isolates 102 and 124 from Oklahoma had the C2 melting profile which clearly differed compared to the other isolates. Most of the isolates displayed double melting domains (peaks) in the derivative melting curve graph as a consequence of the large, 493 bp amplicon (). The downstream fragment of the AvrLm4-7 sequence was analyzed by the AvrLm47B primers (; ) that produced a band size of 153 bp from the 3ʹUTR. The expected band was produced for avirulent (A4A7) and Rlm4-virulent (a4A7) isolates, but not for the double virulent (a4a7) isolates. However, no variation was found in this fragment when comparing the alignments between A4A7 and a4A7 genotypes ().
Fig. 4 High-resolution melting curves obtained from PCR-amplified segments of the AvrLm4-7 gene with the primers AvrLm47A and AvrLm7UP1/LOW2. A and D represent the derivative melting curves. B and E represent the loss of fluorescence normalized curves plotted against increasing temperature. C and F are difference graphs derived from the normalized data in which groups B0 and C0 were subtracted. C, melting profiles obtained using the set of primers AvrLm47A. B0 (A4A7), B1, B2, and B3 (a4A7), B4 (a4a7). F, melting profiles obtained using the primers AvrLm7UP1/LOW2, C0 (A4A7), C1 and C2 (a4A7), and C3 (a4a7).
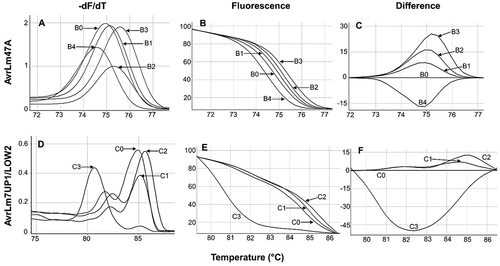
The 67 isolates collected in Oklahoma from 2014 to 2017 were also characterized for AvrLm4-7 genotype using high-resolution melting in comparison to the reference isolates. In the analysis of the upstream sequence with the AvrLm47A primers, 23 isolates displayed the B2 melting profile corresponding to the a4A7 genotype (). The other 44 isolates displayed the B3 melting profile that also corresponded to the a4A7 genotype. For the central region amplified with the AvrLm7 Up1/Low2 primers, 43 isolates displayed the C1 melting profile while the other 24 isolated had the C2 profile, both representing the a4A7 genotype.
Discussion
The a4A7 allele of the AvrLm4-7 gene remained a predominant avirulence allele found in local isolates from Oklahoma collected from 2014 to 2017 as well as in isolates collected in previous years (Diaz et al. Citation2019). We used high-resolution melting analysis of PCR products from the central primers (493 bp) reported previously (Carpezat et al. Citation2014) and from new upstream (5ʹUTT) external primers (105 bp) to distinguish among alleles of AvrLm4-7. In reference isolates, melting profiles generally differed among avirulent (A4A7) and virulent (a4A7 and a4a7) phenotypes using both primer sets. Single unique melting profiles were observed for most A4A7 and a4a7 phenotypes, but multiple profiles were observed for a4A7 phenotypes. However, only the central primers gave melting profiles directly related to the point mutation found in the coding region. The shorter upstream PCR product had better melting curve resolution which was easier to interpret than the longer central fragment with two melting peaks, but the upstream primers did not significantly improve the identification of AvrLm4-7 phenotypes. There was no genetic variation found in an external downstream fragment (3ʹUTT) also evaluated in this study. All of the local isolates were classified as a4A7 phenotypes and genotypic classification by melting analysis was generally supported by pathogenicity scores on seedling cotyledons of susceptible, Rlm4, and Rlm7 differentials; by endpoint PCR and RFLP analysis (Van de Wouw et al. Citation2018).
Most of the avirulence genes in L. maculans including AvrLm4-7 are found in AT-rich repetitive regions of the genome which cause high levels of mutations when those genes are under resistance pressure (Blondeau et al. Citation2015; Plissonneau et al. Citation2016). In the sequences of a4A7 reference isolates, all showed the expected mutation of C358→G358 that produces virulence on Rlm4. This mutation causes a change in the amino acid composition of the AvrLm4-7 protein, which generates a loss of Rlm4 recognition, likely by altering the effector protein structure (Parlange et al. Citation2009). In addition to this mutation, two silent non-synonymous mutations of either C256→A256 or A134→G134 occurred that did not alter protein function. These two haplotypes produced two melting profiles using the central primers and have been previously reported in a4A7 isolates. Other previously reported silent mutations of G123→C123 and T239→C239 were not found in the reference isolates (Parlange et al. Citation2009; Daverdin et al. Citation2012; Carpezat et al. Citation2014). Using melting analysis with the central primers to infer genotypes of the 67 local isolates, the G256→A256 haplotype was dominant at 64% and the T134→C134 haplotype was present in 36% of local isolates. However, the variation in melting curves produced with the upstream primers developed in this study could not be assigned to specific point mutations because only a region that covers most of the central portion of the AvrLm4-7 gene (660 bp) was covered by Sanger sequencing. Also, short portions (~50 bp) of the extremes of the sequences were removed after the analysis of the sequencing chromatogram due to the poor resolution and overlapped nucleotide peaks. Possible point mutations in external regions of the AvrLm4-7 gene including UTRs, promoter regions, enhancers or splice junction signals could cause a complete loss of transcription or incorrect RNA folding causing alternative splicing, and thus impact gene expression (Skeeles et al. Citation2013).
The results obtained in this study and from isolates collected in previous years (Diaz et al. Citation2019) show that the gene AvrLm4-7 has lost the avirulent allele AvrLm4. The absence of AvrLm4 could be determined by phenotypic interaction on the differentials ‘Jet Neuf’ (Rlm4) and PCR product digestion for detection of polymorphic sites (RFLP), but polymorphisms resulting in the loss of the A7 function were not found in local isolates. Additionally, restriction enzyme analysis for AvrLm4 and high-resolution melting analysis profiles did not agree with pathogenicity scores on ‘Jet Neuf’ for 9% of the 67 local isolates. These isolates were avirulent on ‘Jet Neuf’ but lacked a functional AvrLm4 by molecular analysis. Typically, IC scores on these plants ranged from 3.0 to 3.5 and were borderline between avirulent and virulent. Others have reported ambiguous pathogenicity on ‘Jet Neuf’ (Dilmaghani et al. Citation2009). Use of the Rlm4 introgression line Topas-Rlm4 (Larkan et al. Citation2016) may improve cotyledon assay for alleles of AvrLm4-7. Previous research showed that in addition to the C358→G358 mutation in the AvrLm4-7 sequence that produces virulence on Rlm4, a total of five polymorphic sites have been identified (Parlange et al. Citation2009).
The avirulence gene AvrLm4-7 was found in 100% of the isolates collected in this study, similar to that reported previously (Diaz et al. Citation2019). There were four isolates negative for AvrLm4-7 by endpoint PCR but these were classified as a4A7 by melting analysis and avirulence on the Rlm7 hybrid ‘DK Sensei’. Rlm7 hybrids have performed well in research trials in Oklahoma and Kansas, but they have never been marketed commercially in the region. This is likely because they lack glyphosate tolerance which is important for weed control systems in the winter wheat/canola cropping system in the U.S. Southern Great Plains (Bushong et al. Citation2018). The lack of selection pressure on the population from Rlm7 and the likely fitness advantage of maintaining AvrLm4-7 in the absence of selection pressure (Parlange et al. Citation2009) are likely reasons why double virulent (a4a7) isolates have not yet been found in Oklahoma or Kansas. Conversely, the widespread use of canola cultivars and hybrids with the resistance gene Rlm4 in their genetic background in Oklahoma and Kansas likely has caused genetic pressure on local isolates producing the loss of the AvrLm4 allele (Rife et al. Citation2001; Stamm et al. Citation2012; Diaz et al. Citation2019). Additionally, detection of the AvrLm4 allele was analyzed by digestion of PCR products with a restriction enzyme. The avirulent A4 phenotype of the AvrLm4 avirulence gene was not present in any isolates collected in Oklahoma.
Studies indicated that Avrlm4-7 is closely linked with other avirulence genes such as AvrLm3, AvrLm9, and AvrLep1. The presence of AvrLm4-7 blocks the recognition of AvrLm3 by Rlm3 due to an epistatic relationship (Plissonneau et al. Citation2016). In the same way, the recognition of AvrLm9 by Rlm9 is blocked by the presence of AvrLm4-7 (Ghanbarnia et al. Citation2018). These gene interactions were apparent in this study. Because 100% of the local isolates had the A7 allele, the avirulence genes AvrLm3 and AvrLm9 did not recognize respective resistance genes. The susceptible cotyledon reactions of all local isolates on the differentials ‘Glacier’ (Rlm2,3) and ‘Bristol’ (Rlm2,9) (data not shown) supports these avirulence gene interactions.
Studying the frequency of the virulent/avirulent genotype of the L. maculans population present in Oklahoma is essential to developing an effective management strategy deploying effective and durable resistance genes needed in the field. The data provided in this study suggest that the resistance gene Rlm7 would be an effective source of resistance against blackleg in Oklahoma. Molecular analysis using high-resolution melting or restriction digestion of end-point PCR products were effective in the rapid distinction of virulent or avirulent L. maculans isolates towards resistance genes Rlm4 and Rlm7, permitting better informed decisions regarding which resistance gene is best deployed. The increased efficiency of molecular testing of avirulence gene frequency using PCR, PCR+RFLP, and high-resolution melting over cotyledon assays has been well documented (Carpezat et al. Citation2014). However, should Rlm7 cultivars be grown locally and double virulent (a4a7) genotypes appear, their molecular identification will likely prove to be more complex due to the many genetic events that can lead to virulence on Rlm7. In France, double virulent isolates resulted from gene deletions that gave no PCR amplification of AvrLm4-7 (39%) and diverse sequence polymorphisms (61%) of amplified isolates (Carpezat et al. Citation2014). These altered sequences were repeat-induced point (RIP) mutations (65%), nucleotide deletions (23%), and point mutations (12%) causing mostly unique melting profiles. In the four a4a7 reference isolates evaluated in this study, three contained multiple RIP mutations (Van de Wouw and Howlet Citation2012). These were either not amplified or produced melting profiles very different from a4A7 isolates. Due to the multiple polymorphisms, a combination of melting analysis and PCR amplification using external PCR amplification provided the best (94 to 98%) molecular identification of double virulent isolates (Carpezat et al. Citation2014).
Acknowledgements
Special thanks to Angela Van de Wouw, University of Melbourne, Australia, for providing the reference isolates of Leptosphaeria maculans used in this study; Nick Larkan and Hossein Borhan, Agriculture and Agri-Food Canada, Saskatoon, for characterizing reference isolates from Oklahoma and providing the Topas Rml1 to Rlm4 and Lep1 to Lep3 isoline differentials; Regine Delourme, INRAE, France, for providing the differential cultivar ‘Bristol’, and USDA/GRIN for providing the ‘Westar’, ‘Quinta’, ‘Glacier’, and ‘Jet Neuf’ differential cultivars.
Disclosure statement
No potential conflict of interest was reported by the author(s).
Additional information
Funding
References
- Balesdent M-H, Attard A, Ansan-Melayah D, Delourme R, Renard M, Rouxel T. 2001. Genetic control and host range of avirulence toward Brassica napus cultivars Quinta and Jet Neuf in Leptosphaeria maculans. Phytopathology. 91(1):70–76. doi:10.1094/PHYTO.2001.91.1.70.
- Balesdent M-H, Attard A, Kühn ML, Rouxel T. 2002. New avirulence genes in the phytopathogenic fungus Leptosphaeria maculans. Phytopathology. 92(10):1122–1133. doi:10.1094/PHYTO.2002.92.10.1122.
- Balesdent M-H, Barbetti M, Li H, Sivasithamparam K, Gout L, Rouxel T. 2005. Analysis of Leptosphaeria maculans race structure in a worldwide collection of isolates. Phytopathology. 95(9):1061–1071. doi:10.1094/PHYTO-95-1061.
- Blondeau K, Blaise F, Graille M, Kale SD, Linglin J, Ollivier B, Labarde A, Lazar N, Daverdin G, Balesdent MH. 2015. Crystal structure of the effector Avrlm4–7 of Leptosphaeria maculans reveals insights into its translocation into plant cells and recognition by resistance proteins. Plant J. 83:610–624. doi:10.1111/tpj.12913.
- Brun H, Chèvre AM, Fit DDL, Powers S, Besnard AL, Ermel M, Huteau V, Marquer B, Eber F, Renard F, et al. 2010. Quantitative resistance increases the durability of qualitative resistance to Leptosphaeria maculans in Brassica napus. New Phytol. 185(1):285–299. doi:10.1111/j.1469-8137.2009.03049.x
- Bushong J, Lofton J, Sanders H, Stamm M. 2018. Great plains canola production handbook. Kansas State Univ Agric Exp Sta and Coop Ext Serv. MF2734 42.
- Carpezat J, Bothorel S, Daverdin G, Balesdent MH, Leflon M. 2014. Use of high resolution melting analysis to genotype the avirulence AvrLm4-7 gene of Leptosphaeria maculans, a fungal pathogen of Brassica napus. Ann Appl Biol. 164(3):430–440. doi:10.1111/aab.12112.
- Daverdin G, Rouxel T, Gout L, Aubertot JN, Fudal I, Meyer M, Parlange F, Carpezat J, Balesdent M-H. 2012. Genome structure and reproductive behavior influence the evolutionary potential of a fungal phytopathogen. PLOS Pathog. 8(11):e1003020. doi:10.1371/journal.ppat.1003020.
- Degrave A, Wagner M, George P, Coudard L, Pinochet X, Ermil M, Gay E, Fudal I, Moreno-Rico O, Rouxel T, et al. 2021. A new avirulence gene of Leptosphaeria maculans, AvrLm14, identifies a resistance source in American broccoli (Brassica oleracea) genotypes. Mol Plant Pathol. 22(12):1599–1612. doi:10.1111/mpp.13131
- Delourme R, Chevre AM, Brun H, Rouxel T, Balesdent M-H, Dias JS, Salisbury P, Renard M, Rimmer SR. 2006. Major gene and polygenic resistance to Leptosphaeria maculans in oilseed rape (Brassica napus). Eur J Plant Pathol. 114(1):41–52. doi:10.1007/s10658-005-2108-9.
- Diaz C, Cevallos F, Damicone J. 2019. Characterization of the race structure of Leptosphaeria maculans causing blackleg of winter canola in Oklahoma and Kansas. Plant Dis. 103(9):2353–2358. doi:10.1094/PDIS-01-19-0181-RE.
- Dilmaghani A, Balesdent M-H, Didier J, Wu C, Davey J, Barbetti M, Li H, Moreno‐Rico O, Phillips D, Despeghel J. 2009. The Leptosphaeria maculans–Leptosphaeria biglobosa species complex in the American continent. Plant Pathol. 58(6):1044–1058. doi:10.1111/j.1365-3059.2009.02149.x.
- Dwight Z, Palais R, Wittwer CT. 2011. uMelt: prediction of high-resolution melting curves and dynamic melting profiles of PCR products in a rich web application. Bioinformatics. 27(7):1019–1020. doi:10.1093/bioinformatics/btr065.
- Erali M, Voelkerding KV, Wittwer CT. 2008. High resolution melting applications for clinical laboratory medicine. Exp Mol Pathol. 85(1):50–58. doi:10.1016/j.yexmp.2008.03.012.
- Fernando D, Zhang X, Selin C, Zou Z, Liban SH, McLaren DL, Kubinec A, Parks PS, Rashid MH, Padmathilake KR. 2018. A six-year investigation of the dynamics of avirulence allele profiles, blackleg incidence, and mating type alleles of Leptosphaeria maculans populations associated with canola crops in Manitoba, Canada. Plant Dis. 102(4):790–798. doi:10.1094/PDIS-05-17-0630-RE.
- Flor HH. 1971. Current status of the gene-for-gene concept. Annu Rev Phytopathol. 9(1):275–296. doi:10.1146/annurev.py.09.090171.001423.
- Fudal I, Ross S, Gout L, Blaise F, Kuhn ML, Eckert MR, Cattolico L, Bernard-Samain S, Balesdent M-H, Rouxel T. 2007. Heterochromatin-like regions as ecological niches for avirulence genes in the Leptosphaeria maculans genome: map-based cloning of AvrLm6. Mol Plant Microbe Interact. 20(4):459–470. doi:10.1094/MPMI-20-4-0459.
- Ghanbarnia K, Fudal I, Larkan NJ, Links MG, Balesdent M-H, Profotova B, Borhan MH. 2015. Rapid identification of the Leptosphaeria maculans avirulence gene AvrLm2 using an intraspecific comparative genomics approach. Mol Plant Pathol. 16(7):699–709. doi:10.1111/mpp.12228.
- Ghanbarnia K, Ma L, Larkan NJ, Haddadi P, Fernando D, Borhan MH. 2018. Leptosphaeria maculans AvrLm9: a new player in the game of hide and seek with AvrLm4‐7. Mol Plant Pathol. 19(7):1754–1764. doi:10.1111/mpp.12658.
- Gout L, Fudal I, Kuhn ML, Blaise F, Eckert M, Cattolico L, Balesdent M-H, Rouxel T. 2006. Lost in the middle of nowhere: the AvrLm1 avirulence gene of the Dothideomycete Leptosphaeria maculans. Mol Microbiol. 60(1):67–80. doi:10.1111/j.1365-2958.2006.05076.x.
- Hammond-Kosack KE, Jones JD. 1997. Plant disease resistance genes. Annu Rev Plant Phys. 48(1):575–607. doi:10.1146/annurev.arplant.48.1.575.
- Jones JD, Dangl JL. 2006. The plant immune system. Nature. 444(7117):323–329. doi:10.1038/nature05286.
- Kumar S, Stecher G, Tamura K. 2016. MEGA7: molecular evolutionary genetics analysis version 7.0 for bigger datasets. Mol Biol Evol. 33:1870–1874. doi:10.1093/molbev/msw054.
- Kutcher HR, Yu F, Brun H. 2010. Improving blackleg disease management of Brassica napus from knowledge of genetic interactions with Leptosphaeria maculans †. Can J Plant Pathol. 32(1):29–34. doi:10.1080/07060661003620961.
- Larkan NJ, Lydiate DJ, Yu F, Rimmer SR, Borhan MH. 2014. Colocalisation of the blackleg resistance genes Rlm2 and LepR3 on Brassica napus chromosome A10. BMC Plant Biol. 14(1):387–396. doi:10.1186/s12870-014-0387-z.
- Larkan NJ, Yu F, Lydiate DJ, Rimmer SR, Borhan MH. 2016. Single R gene introgression lines for accurate dissection of the Brassica–Leptosphaeria pathosystem. Front Plant Sci. 7:1771. doi:10.3389/fpls.2016.01771.
- Larkan NJ, Ma L, Haddadi P, Buchwaldt M, Parkin I, Djavaheri M, Borhan MH. 2020. The Brassica napus wall-associated kinase-like (WAKL) gene Rlm9 provides race-specific blackleg resistance. Plant J. 104(4):892–900. doi:10.1111/tpj.14966.
- Marcroft SJ, Elliot VL, Cozijnsen AJ, Salisbury PA, Howlett BJ, Van de Wouw AP. 2012. Identifying resistance genes to Leptosphaeria maculans in Australian Brassica napus cultivars based on reactions to isolates with known avirulence genotypes. Crop Pasture Sci. 63(4):338–350. doi:10.1071/CP11341.
- Neik TX, Ghanbarnia K, Ollivier B, Scheben A, Severn-Ellis A, Larkan NJ, Haddadi P, Fernando WGD, Rouxel T, Batley J, et al. 2022. Two independent approaches converge to the cloning of a new Leptosphaeria maculans avirulence effector gene, AvrLmS-Lep2. Mol. Plant. Pathol. 23(5):733–748. doi:10.1111/mpp.13194.
- Orquera-Tornakian GK, Garrido P, Kronmiller B, Hunger R, Tyler BM, Garzon CD, Marek SM. 2017. Identification and characterization of simple sequence repeats (SSRs) for population studies of Puccinia novopanici. J Microbiol Meth. 139:113–122. doi:10.1016/j.mimet.2017.04.011.
- Parlange F, Daverdin G, Fudal I, Kuhn ML, Balesdent M-H, Blaise F, Grezes-Besset B, Rouxel T. 2009. Leptosphaeria maculans avirulence gene AvrLm4-7 confers a dual recognition specificity by the Rlm4 and Rlm7 resistance genes of oilseed rape, and circumvents Rlm4 -mediated recognition through a single amino acid change. Mol Microbiol. 71(4):851–863. doi:10.1111/j.1365-2958.2008.06547.x.
- Patel DA, Zander M, Van de Wouw AP, Mason AS, Edwards D, Batley J. 2015. Population diversity of Leptosphaeria maculans in Australia. Int J Biol. 7(3):18–36. doi:10.5539/ijb.v7n3p18.
- Petit‐Houdenot Y, Degrave A, Meyer M, Blaise F, Ollivier B, Marais C-L, Jauneau A, Audran C, Rivas S, Veneault-Fourrey C, et al. 2019. A two genes–for–one gene interaction between Leptosphaeria maculans and Brassica napus. New Phytol. 223:397–411. doi:10.1111/nph.15762.
- Plissonneau C, Daverdin G, Ollivier B, Blaise F, Degrave A, Fudal I, Rouxel T, Balesdent M-H. 2016. A game of hide and seek between avirulence genes AvrLm4‐7 and AvrLm3 in Leptosphaeria maculans. New Phytol. 209(4):1613–1624. doi:10.1111/nph.13736.
- Plissonneau C, Blaise F, Ollivier B, Lefton M, Carpezat J, Rouxel T, Balesdent M-H. 2017. Unusual evolutionary mechanisms to escape effector-triggered immunity in the fungal phytopathogen Leptosphaeria maculans. Mol Ecol. 26(7):2183–2198. doi:10.1111/mec.14046.
- Plissonneau C, Rouxel T, Chèvre AM, Van De Wouw AP, Balesdent M-H. 2018. One gene-one name: the AvrLmJ1 avirulence gene of Leptosphaeria maculans is AvrLm5. Mol Plant Pathol. 19(4):1012–1016. doi:10.1111/mpp.12574.
- Rife C, Auld D, Sunderman H, Heer W, Baltensperger D, Nelson L, Johnson D, Bordovsky D, Minor H. 2001. Registration of “Wichita” rapeseed. Crop Sci. 41(1):263–264. doi:10.2135/cropsci2001.411263-ax.
- Rouxel T, Willner E, Coudard L, Balesdent M-H. 2003. Screening and identification of resistance to Leptosphaeria maculans (stem canker) in Brassica napus accessions. Euphytica. 133(2):219–231. doi:10.1023/A:1025597622490.
- Rouxel T, Balesdent M-H. 2005. The stem canker (blackleg) fungus, Leptosphaeria maculans, enters the genomic era. Mol Plant Pathol. 6(3):225–241. doi:10.1111/j.1364-3703.2005.00282.x.
- Rouxel T, Balesdent M-H. 2017. Life, death and rebirth of avirulence effectors in a fungal pathogen of Brassica crops, Leptosphaeria maculans. New Phytol. 214(2):526–532. doi:10.1111/nph.14411.
- Skeeles LE, Fleming JL, Mahaler KL, Toland AE. 2013. The impact of 3’ UTR variants on differential expression of candidate cancer susceptibility genes. PloS one. 8(3):e58609. doi:10.1371/journal.pone.0058609.
- Sprague SJ, Balesdent M-H, Brun H, Hayden HL, Marcroft SJ, Pinochet X, Rouxel T, Howlett BJ. 2006. Major gene resistance in Brassica napus (oilseed rape) is overcome by changes in virulence of populations of Leptosphaeria maculans in France and Australia. Eur J Plant Pathol. 114(1):33–40. doi:10.1007/s10658-005-3683-5.
- Stachowiak A, Olechnowicz J, Jedryczka M, Rouxel T, Balesdent MH, Happstadius I, Gladders P, Latunde-Dada A, Evans N. 2006. Frequency of avirulence alleles in field populations of Leptosphaeria maculans in Europe. Eur J Plant Pathol. 114(1):67–75. doi:10.1007/s10658-005-2931-z.
- Stamm M, Berrada A, Buck J, Cabot P, Claassen M, Cramer G, Dooley S, Godsey C, Heer W, Holman J. 2012. Registration of “Riley” winter canola. J Plant Regist. 6(3):243–245. doi:10.3198/jpr2011.10.0555crc.
- Van de Wouw AP, Cozijnsen AJ, Hayne JK, Brunner PC, McDonald BA, Oliver RP, Howlett BJ. 2010. Evolution of linked avirulence effectors in Leptosphaeria maculans is affected by genomic environment and exposure to resistance genes in host plants. PLoS Pathog. 6(11):e1001180. doi:10.1371/journal.ppat.1001180.
- Van de Wouw AP, Howlet BJ. 2012. Estimating frequencies of virulent isolates in field populations of a plant pathogenic fungus, Leptosphaeria maculans, using high-throughput pyrosequencing. J Appl Microbiol. 113(5):1145–1153. doi:10.1111/j.1365-2672.2012.05413.x.
- Van de Wouw AP, Howlett BJ, Idnurm A. 2018. Changes in allele frequencies of avirulence genes in the blackleg fungus, Leptosphaeria maculans, over two decades in Australia. Crop Pasture Sci. 69(1):20–29. doi:10.1071/CP16411.
- Weising K, Atkinson RG, Gardner RC. 1995. Genomic fingerprinting by microsatellite-primed PCR: a critical evaluation. Genome Res. 4(5):249–255. doi:10.1101/gr.4.5.249.
- West JS, Kharbanda PD, Barbetti MJ, Fitt BD. 2001. Epidemiology and management of Leptosphaeria maculans (phoma stem canker) on oilseed rape in Australia, Canada and Europe. Plant Pathol. 50(1):10–27. doi:10.1046/j.1365-3059.2001.00546.x.
- Wittwer CT, Reed GH, Gundry CN, Vandersteen JG, Pryor RJ. 2003. High-resolution genotyping by amplicon melting analysis using Lcgreen. Clinl Chem. 49:853–860.
- Wittwer CT. 2009. High‐resolution DNA melting analysis: advancements and limitations. Hum Mutat. 30(6):857–859. doi:10.1002/humu.20951.
- Zuker M. 2003. Mfold web server for nucleic acid folding and hybridization prediction. Nucleic Acids Res. 31(13):3406–3415. doi:10.1093/nar/gkg595.