Abstract
Stem diseases of soybean with symptoms typical of infection by Diaporthe spp. are common in the Northern USA. Information is limited, however, on the characteristics of the associated pathogens. This study determined the identity and characteristics of Diaporthe spp. associated with symptomatic and asymptomatic soybean stems across diverse environments in Minnesota. Plants with symptoms of stem canker, pod and stem blight, and plant dieback, as well as asymptomatic plants, were sampled. Agar-based media and PCR assays were used to isolate and detect Diaporthe spp. from stems. The isolates were identified to species based on DNA sequencing and morphology. Diaporthe spp. were common in symptomatic and asymptomatic plants across all environments. Three species were detected: D. longicolla, D. caulivora, and D. cucurbitae. Diaporthe longicolla was the most widespread in distribution. Diaporthe cucurbitae was isolated from symptomatic soybean and confirmed to be a soybean pathogen for the first time. Only D. longicolla was detected in asymptomatic plants. While all isolates were pathogenic to soybean, virulence differed among isolates, and cultivars differed in susceptibility. Temperatures of 25°C and 32°C differentially influenced the growth of D. longicolla and D. caulivora. All isolates were sensitive to pyraclostrobin and tebuconazol. The results advance our understanding of the Diaporthe species associated with different soybean stem diseases, as well as their characteristics and management.
Résumé: Les maladies de la tige du soya affichant des symptômes typiques de l’infection causée par Diaporthe spp. sont courantes dans le Nord-Est américain. Toutefois, il y a peu d’information sur les caractéristiques des agents pathogènes qui leur sont associés. Cette étude a établi l’identité et les caractéristiques des Diaporthe spp. associés aux tiges de soya symptomatiques et asymptomatiques dans divers environnements au Minnesota. Les plants présentant des symptômes de chancre de la tige, de brûlure phomopsienne et de dépérissement ainsi que des plants asymptomatiques ont été échantillonnés. Des milieux gélosés et des épreuves par PCR ont été utilisés pour isoler et détecter Diaporthe spp. sur les tiges. Les isolats ont été identifiés à l’espèce en se basant sur le séquençage de l’ADN et la morphologie. Diaporthe spp. étaient courants chez les plants symptomatiques et asymptomatiques, et ce, dans tous les environnements. Trois espèces ont été détectées: D. longicolla, D. caulivora et D. cucurbitae. Diaporthe longicolla était le plus largement disséminé. Diaporthe cucurbitae a été isolé de plants de soya symptomatiques et confirmé en tant qu’agent pathogène du soya pour la première fois. Seul D. longicolla a été détecté chez des plants asymptomatiques. Tandis que tous les isolats étaient pathogènes pour le soya, la virulence variait chez ces derniers et les cultivars ont réagi diversement quant à leur susceptibilité à leur égard. Des températures de 25°C et de 32°C ont influencé différemment la croissance de D. longicolla et de D. caulivora. Tous les isolats étaient sensibles à la pyraclostrobine et au tébuconazole. Les résultats font progresser notre compréhension des espèces de Diaporthe associées aux différentes maladies de la tige du soya de même que de leurs caractéristiques et de leur gestion.
Introduction
Fungal stem diseases of soybean [Glycine max (L.) Merrill] are common in many regions of the world. These include stem canker, pod and stem blight, brown stem rot, and white mould (Malvick et al. Citation2003; Harrington et al. Citation2003; Malvick and Bussey Citation2008; Peltier et al. Citation2012). Stem canker and pod and stem blight diseases incited by Diaporthe Nitschke [= Phomopsis (Sacc.) Bubak] spp. are especially significant worldwide and cause large yield losses (Kulik Citation1984; Sinclair Citation1988, Citation1993; Koenning and Wrather Citation2010; Vidić et al. Citation2011; Udayanga et al. Citation2015; Vidić et al. Citation2015; Allen et al. Citation2017). Although stem diseases caused by Diaporthe spp. occur in the Northern USA. information is limited on their aetiology and the phenotypic and genotypic characteristics of the associated pathogens.
The identification of Diaporthe spp. in many studies has been based on morphology, host--pathogen interactions, and physiological characteristics, sometimes leaving uncertainty about the identity of pathogens involved (van Niekerk et al. Citation2005; Mengistu et al. Citation2007; Lu et al. Citation2010). Molecular and phylogenetic methods now facilitate the identification of Diaporthe spp.; and sequences of two or more genes have enabled resolving and re-assessment of Diaporthe spp. associated with soybean diseases (Santos et al. Citation2011; Gomes et al. Citation2013; Guillin et al. Citation2014; Udayanga et al. Citation2015; Dissanayake et al. Citation2017; Hosseini et al. Citation2020; Petrović et al. Citation2021). For example, a primary soybean pod and stem blight pathogen is D. sojae (syn D. phaseolorum var. sojae), the northern stem canker pathogen is D. caulivora (syn D. phaseolorum var. caulivora), and a common seed decay pathogen is D. longicolla (syn Phomopsis longicolla) (Santos et al. Citation2011; Gomes et al. Citation2013; Guillin et al. Citation2014; Udayanga et al. Citation2015). This, however, is likely an incomplete depiction of the Diaporthe spp. associated with different diseases on soybean. For example, D. longicolla also infects soybean stems, D. gulyae and other Diaporthe spp. can cause stem canker, and multiple species were recently identified as causal agents of seed decay (Mathew et al. Citation2017; Sinclair Citation1988; Rupe Citation1990; Li et al. Citation2010; Hartman et al. Citation2015; Petrović et al. Citation2021). Thus, the identity and characteristics of Diaporthe spp. associated with various soybean diseases in different geographic areas warrants further study.
In the North Central USA and Canada, several Diaporthe spp. have been associated with soybean stem diseases (Gravert et al. Citation2001; Li et al. Citation2004; Bradley and Li Citation2007; Xue et al. Citation2007; Lu et al. Citation2010; Gebreil et al. Citation2015; Abdelmagid et al. Citation2019). Diaporthe caulivora and D. sojae have been associated with northern stem canker and pod and stem blight, respectively, whereas D. longicolla appears more adaptable and has been associated with seed, pod, and stem diseases (Hartman et al. Citation2015). Much research on the Diaporthe spp. associated with soybean has focused on southern stem canker and seed decay in regions with hot and humid summers (Rupe Citation1990; Hartman et al. Citation2015). Less is known about stem canker, pod and stem blight, and the identity of Diaporthe spp. associated with these diseases in northern soybean production areas, which has implications for disease management and breeding for resistance.
In addition to their roles as stem pathogens, Diaporthe spp. have been detected in asymptomatic soybean stems as endophytes or latent pathogens (Miller and Roy Citation1982; Sinclair Citation1988; Impullitti and Malvick Citation2013; Leite et al. Citation2013; Kontz et al. Citation2016; Batzer and Mueller Citation2020). The relationships between endophytic or latent Diaporthe spp. that colonize soybean early in the growing season and the development of stem canker or stem blight later in the season when plants are maturing or stressed is incompletely understood. Kulik (Citation1984) reported that Diaporthe (Phomopsis) spp. inoculated on soybean did not cause symptoms or produce pycnidia until after plant senescence, and Hill (Citation1981) reported that D. sojae could be dormant in soybean near the inoculation site until plant senescence.
Management of Diaporthe stem diseases of soybean may include the use of resistant soybean varieties and fungicides (Hartman et al. Citation2015; Tormen and Blum Citation2020). For example, varieties have been identified with resistance to southern stem canker caused by D. aspalathi and northern stem canker caused by D. caulivora (Benavidez et al. Citation2010; Chang et al. Citation2016; Peruzzo et al. Citation2019). There is limited understanding of resistance to stem blight caused by Diaporthe spp., and more research is needed to clarify the role of pathogen variability on resistance (Kontz et al. Citation2016; Ghimire et al. Citation2019). In addition, fungicides may play a role in the management of Diaporthe soybean stem diseases (Lu et al. Citation2010; Soto-Arias and Munkvold Citation2011). Information is limited, however, on the sensitivity of Diaporthe spp. from soybean stems to fungicides. Moreover, the effects of fungicide sprays on infection of soybean stems by Diaporthe spp. have been inconsistent, where fungicides have decreased and increased infection in different studies (Soto-Arias and Munkvold Citation2011; Batzer and Mueller Citation2020).
This study was undertaken to address knowledge gaps in the identity, pathogenicity, and other characteristics of Diaporthe spp. associated with soybean stem diseases across diverse environments in Minnesota. The specific objectives were to; (i) determine the Diaporthe species associated with symptomatic and asymptomatic infection of soybean stems, (ii) determine if isolates and species vary in virulence, sensitivity to fungicides, and growth at different temperatures, and (iii) determine if selected northern soybean cultivars differ in susceptibility to prevalent Diaporthe spp. and isolates. This work aims to expand our knowledge of the identity, characteristics, and management of Diaporthe species associated with soybean stem disease.
Materials and methods
Sampling and identification of Diaporthe spp. in symptomatic plants
Soybean plants were sampled that had symptoms of stem disease and grouped into three categories: stem canker [discrete brown to reddish brown lesion at node(s)], pod and stem blight (dark pycnidia on stem arranged in rows, darkened stem without discrete lesions), and plant dieback (yellowing and/or death of upper stem with no other apparent root or stem symptoms) (Yang Citation2001; Hartman et al. Citation2015; Mueller et al. Citation2016). The plants (n = 87) were collected at R3 – R6 growth stages (beginning pod to full seed) from 50 fields in 19 counties across Minnesota (). Sections of symptomatic stems, 2 by 8 mm, were surface disinfected with 0.26% NaOCl for 2 m, rinsed in sterile distilled water for 1 m, and blotted dry on sterile paper towels. The pieces were then placed on 0.5x potato dextrose agar (PDA, BD Difco Lab., Sparks, MD) in Petri dishes and incubated at 25°C for 7 to 10 d with a 10 h photoperiod.
Fig. 1 Location (counties) in Minnesota where soybean stems were collected and tested for the presence of Diaporthe spp. (shaded). Symbols indicate where Diaporthe caulivora (●), D. longicolla (X), and D. cucurbitae (▲) were detected. Includes plants with symptoms of pod and stem blight, stem canker, and top dieback, as well as asymptomatic plants.
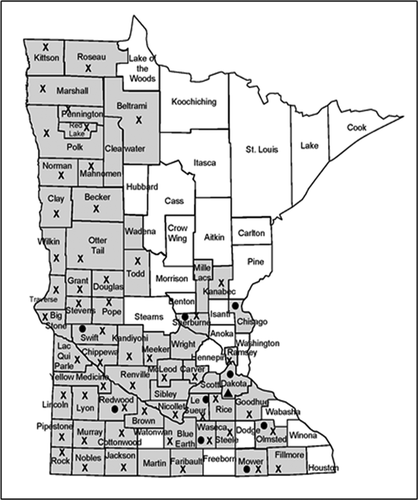
Fungal isolates with colony morphology characteristic of Diaporthe, i.e. white floccose growth on PDA, large stromata, and perithecia (Hartman et al. Citation2015; Vidić et al. Citation2015) were selected from 37 plants collected over a large geographical area. All putative Diaporthe isolates were subcultured by hyphal-tipping two to three times to purify the cultures. The genus and species of the isolates were subsequently identified based on DNA sequences and morphological characteristics. The morphology of isolates from this study, as well as isolates D. caulivora Dc IA41 and D. longicolla Dl IA17 from Iowa, was studied on 0.5x PDA following incubation at 24°C under 14 h fluorescent lights for 14 d.
For sequencing analysis, the isolates were grown in PD broth (BD Difco Laboratories, Franklin Lakes, NJ) for 7 d, and total DNA was extracted from all 37 isolates with a FastDNA® kit (MP Biomedical, Solon, OH). A standard PCR (sPCR) assay using primers Phom I and Phom II was used for presumptive confirmation that the isolates were Diaporthe spp. (Zhang et al. Citation1997). The species identity for all 37 isolates was then determined by sequencing the nuclear ribosomal internal transcribed spacer (ITS) region, amplified with ITS4 and ITS5 primers, and the beta-tubulin (TUB) gene amplified using the primers BT2A and BT2B (White et al. Citation1990; Glass and Donaldson Citation1995; Zhang et al. Citation1997; Lu et al. Citation2010; Udayanga et al. Citation2012) (). DNA sequencing was performed at the University of Minnesota BioMedical Genomics Center (St. Paul, MN) using an Applied Biosystems Prism 3730xl DNA analyzer. Amplification conditions used for all sequences were as follows: 1 cycle of 96°C for 60 seconds followed by 25 cycles of the following: 96°C for 10 seconds, 50°C for 5 seconds, and 60°C for 75 seconds. Consensus sequences were created using BioEdit 7.0.5.3 (Hall Citation1999) and compared to known sequences using BLASTN (www.ncbi.nlm.nih.gov/).
Table 1. Isolate code, species, geographical origin, associated symptoms, source, and virulence group for isolates of Diaporthe spp. used in these studies.
For further confirmation of the identity and to determine relationships among isolates, ITS region and the TUB gene sequences for 13 D. longicolla and 11 D. caulivora isolates were analyzed phylogenetically (). The ITS and TUB sequences were concatenated (~1400 bp) and aligned using Muscle in MEGA7.0.21 (Kumar et al. Citation2016). Sequences from GenBank and Diaporthe spp. isolates from Iowa were included for comparison (Santos et al. Citation2011; Gomes et al. Citation2013; Udayanga et al. Citation2015) (). Analysis was also conducted in MEGA and a tree was constructed with concatenated ITS and TUB sequences (34 nucleotide sequences) and inferred by using the Maximum Likelihood method based on the Tamura 3-parameter model (Tamura Citation1992) using MEGA7. The tree was rooted to D. sojae. Initial tree(s) for the heuristic search were obtained by applying Neighbour-Join and BioNJ algorithms to a matrix of pairwise distances estimated using the Maximum Composite Likelihood approach and selecting the topology with superior log likelihood (−2834.2204). A discrete Gamma distribution was used to model evolutionary rate differences [5 categories (+G, parameter = 1.5788)]. The tree was drawn to scale with branch lengths measured in the number of substitutions per site. Positions with < 95% site coverage were eliminated. Confidence levels were determined with bootstrap analysis (1000 reps).
Symptomatic plants (n = 56) that did not yield isolates of Diaporthe spp. were tested for the presence and identity of Diaporthe spp. using standard and quantitative PCR (sPCR and qPCR) assays. Individual stem pieces were ground and DNA was extracted using a modified FastDNA® protocol (Malvick and Grunden Citation2005). The presence of Diaporthe spp. was confirmed in 10 of the plants using the sPCR assay with Phom I and Phom II primers as noted above. The identity of Diaporthe spp. in the 10 plants was then determined with species-specific quantitative PCR (qPCR) assays using primer/probe sets PL-3 and DPC-3; which were specific for D. longicolla and D. caulivora, respectively (Zhang et al. Citation1999; Kontz et al. Citation2016). The assays were performed on an Applied Biosystems 7500 Real-Time PCR System (Foster City, CA).
Asymptomatic soybean plants (n = 154) at the R6 growth stage were also arbitrarily collected from 111 fields in 62 counties representing diverse soybean production areas across Minnesota (). The fields were selected using distance-based transects in each county and plants were sampled arbitrarily 4 m from the edge of the fields. DNA was extracted from the stems and the presence of Diaporthe spp. was determined with sPCR as described above. The 73 samples that were positive with the sPCR assay were then tested with the previously described qPCR assay to determine species.
Pathogenicity of Diaporthe isolates and resistance of soybean cultivars
Six soybean cultivars (cvs.) were randomly chosen and inoculated to determine their reaction to 24 isolates of Diaporthe species (). The cvs. were: ‘AG2107’ (Monsanto Co., St Louis, MO), ‘MN1606SP’ (Univ. of Minnesota), ‘BSR101’, ‘Corsoy 79’, ‘IA2008R’ (Albert Lea Seed Co, Albert Lea, MN), and ‘S19-A6’ (Syngenta AG, Basel, Switzerland). Three seeds of each cv. were sown in pots containing Sunshine LC8 growing mix (Sun Gro Horticulture, Belleview, WA).
Isolates of D. longicolla (n = 13) and D. caulivora (n = 11) were selected for virulence studies to represent different geographic areas of origin. The isolates were grown on 0.5x PDA (BD Difco Lab.) for 7 d at 25°C, and then used to inoculate the plants growing in pots using a cut-stem inoculation method. Stems were cut just below the fourth node at growth stage V3, and 200 μL sterile micropipette tips containing two PDA plugs (4-mm diameter) colonized with individual isolates were placed over the cut stems (Bradley and Li Citation2007; Li et al. Citation2010). Sterile PDA plugs were used for controls. Plants in four replicate pots per cv. were inoculated with each isolate. Pots were arranged in a randomized complete block design and maintained in a greenhouse at 24°C day and 18°C night with a 14 h photoperiod. Pipette tips were removed from plants 10–12 d post inoculation. Disease severity for each inoculated plant was determined 6 weeks post inoculation (wpi) based on percentage necrotic length of the inoculated internode and on percentage branch dieback (number of dead branches/total number of branches on each plant x 100). The average disease severity and branch dieback were calculated per pot. Symptomatic stem pieces for each variety and isolate combination were collected, surface disinfected with bleach, rinsed in sterile water, and placed on 0.5x PDA. Petri dishes were incubated at 25°C with a 10 h photoperiod for 7 to 10 d. The morphology of each isolate from inoculated plants was compared to the isolate used for inoculations. The experiment was repeated once.
Detection and characterization of Diaporthe cucurbitae
Soybean plants exhibiting stem cankers and severe dieback of leaves and upper branches were collected from a field near Rosemount, Dakota County, MN in September 2017 (). Stems of five diseased plants were split and vascular browning was also present. Isolations were made from the stems as previously described, resulting in a culture with characteristics of Diaporthe spp. (Hartman et al. Citation2015; Udayanga et al. Citation2015). The fungus was grown in potato dextrose broth, DNA was extracted, and partial ITS and TUB regions were sequenced as described above, and the translation elongation factor-1 alpha (EF1-α) was sequenced using primers EF1-728 F/EF1-986 R (Glass and Donaldson Citation1995; Carbone and Kohn Citation1999; Udayanga et al. Citation2012). The isolate was also tested using the sPCR and specific qPCR assays described above. Koch’s postulates were completed on soybean cv. ‘MN1606SP’ using the cut-stem inoculation method noted above. Diaporthe caulivora isolate (Dc M6) was used as a positive control and sterile PDA was the negative control. Four pots with three seeds each were used per treatment. Stems were rated for symptom development 6 weeks post inoculation (wpi). Fungi were isolated from symptomatic stems 6 wpi as described above, and the morphology and DNA sequences of recovered isolates were compared to the isolate used for inoculation.
Effects of temperature and fungicides on growth of Diaporthe isolates
Radial growth of D. longicolla (n = 13) and D. caulivora (n = 11) isolates () was determined at 15, 25 and 32°C. An agar plug (5-mm-diam.) from each isolate grown 2 weeks on 0.5x PDA at 25°C was transferred to the centre of three replicate Petri dishes containing 0.5x PDA. Cultures were incubated in darkness for 14 d at each temperature in a completely random design and colony diameters were measured twice across each colony at right angles. The experiment was repeated once.
Sensitivity to pyraclostrobin (Headline®, BASF Corp.) and tebuconazole (Folicur® 3.6 F, Bayer CropScience) was determined with 13 isolates of D. longicolla and 11 isolates of D. caulivora. Each fungicide was added to 0.5x PDA at 0.01, 0.05, 0.1, 0.5, 1 and 5 µg a.i. mL−1. Salicylhydroxamic acid (SHAM) was dissolved in methanol and added at 100 µg mL−1 to media with pyraclostrobin. Non-amended media was used as a control for tebuconazole, and media with 100 µg mL−1 SHAM was the control for pyraclostrobin. Agar disks (5 mm) from 2-week-old cultures were placed on media in three replicate Petri dishes per treatment and incubated in darkness for 7 d at 25°C in a completely random design. Colony diameters were measured and EC50 values were calculated by regression against the log fungicide concentration using the two concentrations bracketing 50% growth (Lonergan et al. Citation2015). This study was repeated once.
Data analysis
Data were analyzed using JMP version 14.3.0 (SAS Institute Inc., Cary, NC). For the greenhouse studies, virulence data were Arcsine transformed and analysis of variance (ANOVA) was calculated. When significant treatment effects were detected, means were separated by Tukey’s test (P < 0.05). There was an isolate by cultivar interaction and factors were analyzed separately. Average lesion severity (%) was calculated across all cvs., and virulence groups were generated using the ‘Interactive Binning’ add-in (low virulence <46%; moderate virulence 46–71%; high virulence >71%). Due to non-normality of data for percentage branch dieback, a non-parametric analysis was conducted using Fit Y by X function, and a Wilcoxon test was used for mean separation. For the teimperature study on fungal growth, a Kruskal-Wallis test was used for mean separation, and means were separated using Dunn’s test (Bonferroni adjustment). EC50 data from the two fungicide sensitivity experiments could not be combined and were analyzed separately; then the data were log transformed and ANOVA was conducted. When significant treatment effects were detected, means were separated by Tukey’s test for isolates and Student’s T-Test for fungicides and species (P < 0.05). Fungicide by isolate interactions occurred and fungicides were analyzed separately.
Results
Identification of isolates of D. caulivora and D. longicolla
For all 37 isolates sequenced, except one as described below, the ITS and TUB sequences had 99–100% sequence identity matches to either D. longicolla or D. caulivora in BLASTN. The sequences were deposited in the NCBI GenBank database (Table S1). The sequencing and specific qPCR results agreed for the isolates. Phylogenetic analysis of the Diaporthe isolates based on concatenated ITS and TUB sequences separated the isolates into two main clusters representing D. longicolla and D. caulivora (). The D. longicolla isolates from Minnesota, along with two D. longicolla isolates from Iowa and two reference isolates of D. longicolla are in one main cluster. Diaporthe sojae is related and a more distant but related branch contains D. cucurbitae and D. phaseolorum. The other main cluster contains all of the D. caulivora isolates included in this study. This analysis resulted in the same clustering of isolates regardless of whether ITS or TUB sequences were used alone or concatenated for analysis ().
Fig. 2 Phylogenetic analysis based on combined ITS and TUB DNA sequences. The tree is drawn to scale with branch lengths measured in the number of substitutions per site. Bootstrap values with 1000 replications are shown at the nodes. Isolate codes noted as DL are Diaporthe longicolla and DC are D. caulivora. The D. longicolla, D. caulivora, and D. cucurbitae sequences are from isolates collected in this study and the others are from GenBank for comparison ().
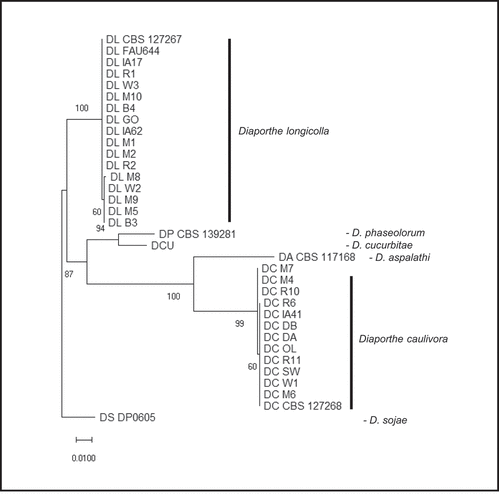
The morphology of the isolates identified as either D. caulivora or D. longicolla was the same as described previously (Hobbs et al. Citation1985; Santos et al. Citation2011; Hartman et al. Citation2015; Udayanga et al. Citation2015; Hosseini et al. Citation2020; Mena et al. Citation2020; Petrović et al. Citation2021). Growth of the isolates of D. caulivora was white with tan to brown areas and the reverse side of the dish developed tan pigmentation. Growth of the isolates of D. longicolla was dense, white, with occasional greenish-coloured patches, and the underside of colonies developed large, black stromata (Fig S1). Growth on 0.5x PDA was fast for all isolates of D. caulivora and D. longicolla (average 8.3 mm d−1).
Distribution of Diaporthe spp. associated with symptomatic and asymptomatic infection of soybean
Diaporthe spp. were common inhabitants of symptomatic and asymptomatic soybean stems across the diverse environments and soybean maturity group zones that were sampled. Diaporthe longicolla was widespread in symptomatic (29%) and asymptomatic (47%) soybean plants across Minnesota from the Iowa border to near the Manitoba border (, ). Diaporthe caulivora was detected only in plants in the southern half of Minnesota and was not detected in asymptomatic plants (, ). Diaporthe cucurbitae was isolated and confirmed from soybean in one field in southern Minnesota. Other Diaporthe spp. were not detected in symptomatic or asymptomatic plants via isolations or PCR assays.
Table 2. Diaporthe spp. detected in soybean stems with different types of symptoms.
Diaporthe spp. were detected differentially via isolation on media or with qPCR from soybean stems with different types of symptoms. Diaporthe longicolla was detected in 32%, 37%, and 9% of plants with symptoms of pod and stem blight, stem canker, and top dieback, respectively. Whereas D. caulivora was isolated or detected in 40%, 20%, and 5% of plants with these symptoms, respectively (). Isolation of Diaporthe was most successful from plants with stem canker and pod and stem blight symptoms (47% and 54%, respectively) and less successful from plants with top dieback symptoms (4.5%). Of the symptomatic plants that did not yield Diaporthe in isolations, 20% were positive for Diaporthe with the sPCR assay and all of those were determined to be either D. longicolla or D. caulivora based on the species-specific qPCR positive assays ().
Detection and characterization of Diaporthe cucurbitae
One isolate from soybean was confirmed to be D. cucurbitae based on ITS, TUB, and EF1 sequences and morphology, and its pathogenicity to soybean was confirmed with Koch’s postulates. The sequences were deposited in the NCBI GenBank database as accession numbers OL843922, OL860962, and OL999089 for ITS, EF1-α, and TUB, respectively. The ITS, TUB, and EF1-α sequences were 98–100% identical to D. cucurbitae sequences in GenBank (accessions KP118848, NR_147563, KM453211, DAOM 42078). The D. cucurbitae culture produced white, fluffy mycelia with dark green to brown colouration in the centre. Growth rate of the isolate was moderate (4.5 mm d−1) on 0.5x PDA. Alpha conidia were 8.3–10 µm by 4.3 µm and aseptate, hyaline, and biguttulate. Beta conidia were abundant and curved, hooked, aseptate, and 25 µm by 1.25 µm (Fig S2). In the greenhouse pathogenicity assays, 100% of the plants inoculated with D. cucurbitae developed dark brown stem cankers and 17% developed top dieback (Figs S3 and S4). For comparison, 67% of the check plants inoculated with D. caulivora developed stem cankers and >50% developed dieback. Diaporthe cucurbitae and D. caulivora (used as a control) were recovered from symptomatic tissue in inoculated plants and their identities confirmed with sequencing and qPCR assays. The negative control plants did not develop symptoms of infection and Diaporthe was not isolated from them.
Virulence of Diaporthe isolates on different soybean cultivars
Inoculation of soybean with D. longicolla and D. caulivora resulted in sunken brown cankers, wilted leaves, and branch dieback starting at inoculated nodes. Virulence differed between D. caulivora and D. longicolla and among isolates within each species (). Diaporthe longicolla produced significantly greater lesion severity than D. caulivora on all six soybean cvs., and D. caulivora produced significantly greater branch dieback on four of the cvs. (, ). All isolates of D. longicolla were moderately to highly virulent (64–91% severity), whereas the D. caulivora isolates ranged from low to high (15–84% severity).
Table 3. Virulence of 11 isolates of Diaporthe caulivora (Dc) and 13 isolates of D. longicolla (Dl) on six soybean cultivars. Virulence was measured as percentage of internode that was symptomatic 6 weeks post-inoculation in a greenhouse at 24°C. Treatments in the same column not designated by the same letter are significantly different (α = 0.05).
Fig. 3 Average virulence of 11 isolates of D. caulivora (cross hatch bars) and 13 isolates of Diaporthe longicolla (black bars) on six soybean cultivars. Virulence was measured as percentage of internode length with brown lesions 6 weeks after inoculation in a greenhouse at 24°C. Each cv. was analysed separately. Treatments designated by different letters are significantly different (α = 0.05).
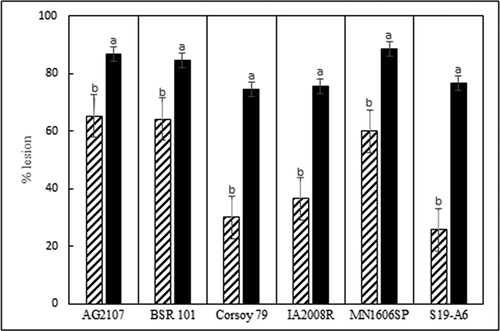
Fig. 4 Branch dieback (average) resulting from inoculation of six soybean cultivars with 11 isolates of Diaporthe caulivora (cross hatch bars) and 13 isolates of D. longicolla (black bars), 6 weeks post inoculation in a greenhouse at 24°C. Percentage branch dieback was determined as the number of dead branches/total number of branches on each plant x 100. Each cv. was analysed separately. Treatments noted with different letters are significantly different (α = 0.05).
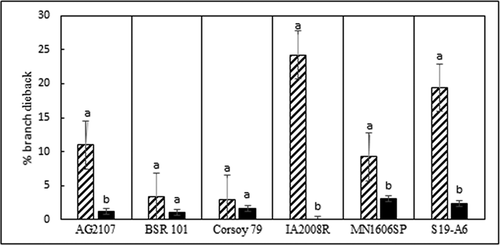
The isolates within each species of Diaporthe also varied in virulence on each soybean cv. (). For each of the six cvs., at least one isolate was significantly different from others based on lesion severity (). The two isolates that caused the most severe branch dieback (Dc DB and Dc M6) were also highly virulent based on stem lesion severity.
The six soybean cvs. differed significantly in reaction to D. caulivora and D. longicolla (). Cultivars ‘S19-A6’, ‘Corsoy 79’, and ‘IA2008R’ were least susceptible to D. caulivora based on lesion severity, but all cvs. were similarly susceptible to D. longicolla (, ). Branch dieback was greater following inoculation with D. caulivora than D. longicolla for all cvs. except for ‘Corsoy 79’ and ‘BSR 101’ (). The cvs. ‘IA 2008 R’ and ‘S19-A6’ had the highest levels of branch dieback following inoculation with D. caulivora, and ‘Corsoy 79’ and ‘BSR 101’ had the lowest (). In all virulence studies, Diaporthe isolates were recovered from the inoculated stem tissue and Diaporthe was not isolated from control plants.
Influence of temperature and fungicides on growth of Diaporthe isolates
Average colony diameters at 14 d for all D. caulivora isolates combined at 15°C, 25°C, and 32°C were 8.5, 8.3, and 0.8 cm, respectively (). Average diameters for all D. longicolla isolates combined at 15°C, 25°C, and 32°C were 8.4, 7.5, and 5.4 cm, respectively. Growth of the two species was significantly different at 25 and 32°C (p < 0.05). Diaporthe caulivora isolates grew significantly more than D. longicolla isolates at 25°C and significantly less than D. longicolla at 32°C (). Both species of Diaporthe grew significantly slower at 32°C than at 15 and 25°C.
Fig. 5 Average colony diameter (cm) of Diaporthe caulivora (cross hatch bars) and D. longicolla (black bars) isolates after 14 d of growth on 0.5x PDA at three temperatures. Each temperature was analysed separately. Treatments designated by different letters are significantly different (α = 0.05).
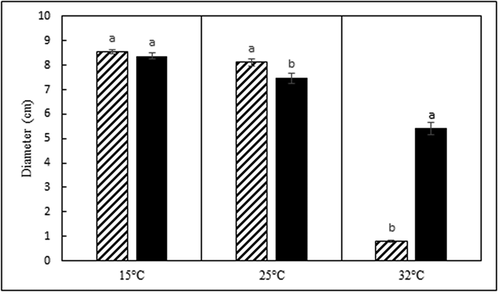
Sensitivity to the fungicides pyraclostrobin and tebuconazole varied among isolates of D. longicolla and D. caulivora and between the two fungicides tested, although all isolates of both species were sensitive to the fungicides. Isolates were generally less sensitive to tebuconazole. The mean EC50 for all isolates in the experiments were 0.05 µg mL−1 a.i. for pyraclostrobin and 0.38 µg mL−1 a.i. for tebuconazole (). The mean EC50 for both species were similar for pyraclostrobin, but for tebuconazole the mean EC50 for D. caulivora was 0.34 µg mL−1 a.i. and for D. longicolla it was 0.42 µg mL−1 a.i. The Diaporthe isolates responded similarly to both fungicides in both experiments.
Table 4. Sensitivity of 11 isolates of Diaporthe caulivora (Dc) and 13 isolates of D. longicolla (Dl) to the fungicides pyraclostrobin and tebuconazole. The EC50 (µg mL−1 a.i.) was determined based on radial growth on agar plates containing these fungicides. Fungicides were analysed separately. Treatments in the same column not designated by the same letter are significantly different (α = 0.05).
Discussion
Soybean stem diseases caused by Diaporthe spp. are significant problems worldwide. The associated pathogen species and their characteristics are complex, creating challenges for understanding and managing them. In this study, the Diaporthe spp. associated with symptomatic and asymptomatic soybean stems were characterized across an ecologically and climatically diverse geographic region in Minnesota. The Diaporthe diversity and species composition was narrow. Diaporthe caulivora and D. longicolla were the only species detected in 98% of plants sampled. Diaporthe longicolla was the predominant species associated with stem blight and stem canker symptoms and asymptomatic stems. Isolates in both species varied in virulence to soybean, response to temperature, and fungicide sensitivity. Diaporthe cucurbitae, the only other Diaporthe species detected, was confirmed to be a pathogen of soybean stems for the first time. The results expand understanding of the identity and characteristics of Diaporthe spp. associated with stem infection of soybean.
Diaporthe caulivora is reported as the primary cause of northern stem canker in soybean, although D. longicolla has also occasionally been reported as a causal agent (Cui et al. Citation2009; Lu et al. Citation2010; Mathew et al. Citation2015, Citation2017Citation2017; Mena et al. Citation2020). In this study of plants with stem canker symptoms, D. caulivora was detected in only 20% of plants and D. longicolla was detected at nearly twice that frequency. Diaporthe caulivora was also detected in 40% of plants with pod and stem blight symptoms (). There were no clear differences in appearance of symptoms produced by D. caulivora or D. longicolla on inoculated plants, but virulence was greater for D. longicolla in greenhouse trials. The results from this study support previous studies that suggest that D. caulivora and D. longicolla are both associated with stem canker.
Diaporthe sojae (syn D. phaseolorum var. sojae) has been considered the primary cause of pod and stem blight disease of soybean (Udayanga et al. Citation2012: Hartman et al. Citation2015; Mueller et al. Citation2016). This species, however, was not detected in any of the plants in this study with stem blight symptoms. Rather, D. longicolla and D. caulivora were detected at similar levels from stems with stem blight symptoms. Diaporthe longicolla has often been described in association with seed decay, although it has also been associated with stem infection (Harrington et al. Citation2000; Xue et al. Citation2007; Lu et al. Citation2010; Ghimire et al. Citation2019; Mena et al. Citation2020). The results from this and previous studies suggest that D. sojae is not the only and primary cause of pod and stem blight disease; D. longicolla is and D. caulivora may be more important causal agents in at least some areas, perhaps depending on environmental differences.
Two species of Diaporthe that have been widely reported to be associated with soybean exhibiting stem blight or stem canker symptoms are Diaporthe sojae and D. aspalathi. These species were not detected from any plants in this study. The absence of D. sojae may be due to the relatively greater importance of D. longicolla as a stem pathogen in the geographic region sampled. In addition, the historical challenges with identification of Diaporthe spp. and the recent taxonomic reassessment of species in this genus may also be relevant. The absence of D. aspalathi (syn D. phaseolorum var. meridionalis), causal agent of southern stem canker, was not surprising given that it appears to be uncommon in the northern US (Smith and Backman Citation1988; Hartman et al. Citation2015).
Several Diaporthe species may cause similar symptoms on soybean, and the challenges and changes with taxonomy and identification may have led to inconsistencies in ID of species associated with different symptoms in different studies. In this study, identification was based primarily on ITS region and TUB gene sequences as well as morphological features (Mathew et al. Citation2015; Udayanga et al. Citation2015; Dissanayake et al. Citation2017; Petrović et al. Citation2021). Gomes et al. (Citation2013), Dissanayake et al. (Citation2017), and Hosseini et al. (Citation2020) suggested that molecular data, at least sequences of ITS and TUB or HIS, are necessary for reliable identification of Diaporthe species. Additionally, if isolates are not available, PCR approaches are also useful. In this study, Diaporthe spp. in asymptomatic plants and in symptomatic plants that did not yield Diaporthe isolates were identified using qPCR assays (Zhang et al. Citation1999: Lu et al. Citation2010; Kontz et al. Citation2016). Kontz et al. (Citation2016) also applied the qPCR assay to symptomatic and asymptomatic soybean samples and detected Diaporthe spp. in plants that failed to produce cultures with isolation techniques, similar to what was found in this study and reported by Impullitti and Malvick (Citation2013). In this study, the identity of a set of isolates was cross-validated using ITS and TUB sequences and qPCR assays, and the sequencing and the specific qPCR assay results agreed.
The unexpected species of Diaporthe detected in this study was D. cucurbitae. To our knowledge, D. cucurbitae has not been previously reported to be a pathogen of soybean. Diaporthe cucurbitae was isolated from a soybean plant expressing stem canker symptoms and confirmed to be pathogenic and cause stem cankers on soybean using Koch’s postulates. Diaporthe cucurbitae has been reported on Cucumis and Arctium spp. causing fruit rot (Udayanga et al. Citation2015). A synonym for D. cucurbitae based on the host was previously considered to be D. melonis, which has been reported on soybean, but they are clearly different species (Udayanga et al. Citation2015; Dissanayake et al. Citation2017; Zaw et al. Citation2020). While this study suggests the distribution of this pathogen may be limited on soybean, the results also suggest it has the potential to be an important soybean pathogen. The detection and confirmation of D. cucurbitae as a soybean pathogen for the first time also supports two broader issues with this genus. First, the species associated with and potentially important as pathogens of soybean are not well understood. Second, more species are found associated with soybean as more studies are conducted. For example, recent studies of soybean seed decay revealed several Diaporthe spp. not previously associated with soybean seed infection (Petrović et al. Citation2021).
The phylogenetic comparison shows that D. caulivora and D. longicolla from this study are clearly delineated based on ITS and TUB sequences, and D. caulivora and D. aspalathi are separated from D. sojae, as reported in other studies (Udayanga et al. Citation2015; Hosseini et al. Citation2020). Diaporthe cucurbitae was clearly delineated as a separate species and related to D. phaseolorum, as seen in other studies (Zaw et al. Citation2020). The sequencing and phylogenetic studies detected minimal sequence differences among isolates within each species, indicating there is little genetic diversity among isolates in this diverse geographic region that could be measured with those two sequences. The low levels of diversity detected within each species were not related to virulence (data not shown). Lu et al. (Citation2010) also reported minimal nucleotide differences among D. longicolla isolates or D. caulivora isolates from soybean stems.
Diaporthe longicolla was the only Diaporthe species detected in asymptomatic plants, where it was detected at high frequency in plants collected over a large area with different soil types, climate patterns, and growing zones. We do not know the aetiology or potential outcomes of these endophytic or latent infections. However, the D. longicolla isolates from symptomatic stems were aggressive stem pathogens in this study and this species also might be a significant latent pathogen in the asymptomatic plants that cause disease damage when plants mature or are stressed (Hartman et al. Citation2015). Numerous other studies have also found D. longicolla and undescribed Diaporthe spp. in association with asymptomatic soybean stems (Harrington et al. Citation2000; Lu et al. Citation2010; Impullitti and Malvick Citation2013; Batzer and Mueller Citation2020) and have speculated on their potential importance as pathogens.
Differential growth at various temperatures in-vitro clearly separated D. caulivora and D. longicolla. For example, all isolates of D. longicolla isolates tested grew significantly more than isolates of D. caulivora at 32°C. The differential effects of temperature suggests that these two pathogens may have different temperature optima for infection and disease development. Further studies are needed to determine the influence of temperature on infection and disease development by these Diaporthe spp.
Fungicides are often applied to soybeans in mid-growing season to manage foliar diseases and to enhance yields; however, their potential to manage stem diseases caused by Diaporthe spp. is unclear. In this study, a common QoI fungicide (pyraclostrobin) and triazole fungicide (tebuconazole) were used to determine their potential to suppress growth of the Diaporthe spp. These fungicides inhibited mycelial growth of D. longicolla and D. caulivora isolates at low levels, suggesting that the isolates were sensitive to these fungicides and that these fungicides have potential for managing stem diseases caused by these Diaporthe spp. Soto-Arias and Munkvold (Citation2011) reported that incidence of stem infection by Phomopsis spp. (syn Diaporthe spp.) in Iowa was reduced by pyraclostrobin but not tebuconazole applications at R3 and R5 growth stages. However, in a more recent field study in Iowa, the fungicides fluxapyroxad and pyraclostrobin appeared to increase the frequency of Diaporthe spp. detected in soybean stem (Batzer and Mueller Citation2020). Thus, there is a need for more studies to determine if fungicides can influence infection, colonization, and stem disease development in soybean caused by Diaporthe spp.
The pathogenicity/virulence studies illustrate two key findings. One is that virulence differed between the species D. caulivora and D. longicolla; and another is that isolates within each species vary in virulence on and among individual soybean cultivars. The isolates of D. longicolla were more virulent than the isolates of D. caulivora. Greater virulence may partially explain why D. longicolla was more common than D. caulivora in the stems in this study. Other studies have also found differences in virulence among isolates of D. longicolla and D. caulivora (Keeling Citation1985; Pioli et al. Citation2003; Lu et al. Citation2010; Mena et al. Citation2020). In this study, the original symptoms of the plant from which isolates were obtained had no association with how aggressive the isolate was as a stem pathogen.
The virulence studies also suggest that soybean cultivars differ in susceptibility to D. caulivora and D. longicolla, as determined with a set of arbitrarily selected cultivars. The different levels of susceptibility among cultivars, which presumably were not deliberately selected for resistance to these Diaporthe spp., is encouraging and suggests that genetic traits for partial resistance may be common. The fact that isolates of Diaporthe varied in virulence across soybean cultivars and individual cultivars differed in susceptibility to different isolates, however, also suggests a need for developing resistance against a set of different isolates. These results were obtained using one inoculation method and one set of environmental conditions, which may influence the interactions between isolate and host (Ghimire et al. Citation2019). Resistance to D. caulivora has been reported (Peruzzo et al. Citation2019), but there appears to be limited knowledge on resistance to D. longicolla stem infection. The widespread occurrence of soybean stem diseases attributed to Diaporthe spp. suggests a need for more effort to select for and incorporate resistance into soybean cultivars.
In conclusion, results from this study indicate that stem diseases caused by D. longicolla and D. caulivora are a widespread and common risk to soybean production. In addition, D. cucurbitae may also be an important pathogen. Additional research is needed to identify Diaporthe stem pathogens in different regions, as well as their optimal conditions for infection and disease development. More work is also warranted on breeding and other disease management efforts using diverse virulence phenotypes of these Diaporthe spp.
TCJP-2021-0260supple.pdf
Download PDF (203.8 KB)TCJP-2021-0260suppld.pdf
Download PDF (267.6 KB)TCJP-2021-0260supplc.pdf
Download PDF (124.9 KB)TCJP-2021-0260supplb.pdf
Download PDF (153 KB)TCJP-2021-0260suppla.pdf
Download PDF (101.4 KB)Disclosure statement
No potential conflict of interest was reported by the author(s).
Supplementary material
Supplemental data for this article can be accessed online here: https://doi.org/10.1080/07060661.2022.2077450
Additional information
Funding
References
- Abdelmagid A, Hafez M, Lawley Y, Adam LR, Daayf F. 2019. First report of northern stem canker caused by Diaporthe caulivora on soybean in Western Canada. Plant Dis. 103(2):372. doi:10.1094/PDIS-07-18-1185-PDN.
- Allen TW, Bradley CA, Sisson AJ, Byamukama E, Chilvers MI, Coker CM, Collins A, Damicone JP, Dorrance AE, Dufault NS, et al. 2017. Soybean yield loss estimates due to diseases in the United States and Ontario, Canada, from 2010 to 2014. Plant Health Progress. 18(1):19–27. doi:10.1094/PHP-RS-16-0066.
- Batzer JC, Mueller DS. 2020. Soybean fungal endophytes Alternaria and Diaporthe spp. are differentially impacted by fungicide application. Plant Dis. 104(1):52–59. doi:10.1094/PDIS-05-19-1001-RE.
- Benavidez R, Pioli RN, Morandi EN. 2010. Response of the edamame edible soybean germplasm to Diaporthe phaseolorum, causal agent of soybean stem canker, in Argentina. Trop Plant Pathol. 35(1):48–51. doi:10.1590/S1982-56762010000100008.
- Bradley CA, Li S. 2007. First report of northern stem canker of soybean caused by Diaporthe phaseolorum var. caulivora in North Dakota. Plant Disease. 90(5):687. doi:10.1094/PD-90-0687A.
- Carbone I, Kohn LM. 1999. A method for designing primer sets for speciation studies in filamentous ascomycetes. Mycologia. 91(3):553–556. doi:10.1080/00275514.1999.12061051.
- Chang H-X, Lipka AE, Domier LL, Hartman GL. 2016. Characterization of disease resistance loci in the USDA soybean germplasm collection using genome-wide association studies. Phytopathology®. 106(10):1139–1151. doi:10.1094/PHYTO-01-16-0042-FI.
- Cui Y-L, Duan C-X, Wang X-M, Li H-J, Zhu Z-D. 2009. First report of Phomopsis longicolla causing soybean stem blight in China. Plant Pathol. 58(4):799. doi:10.1111/j.1365-3059.2009.02057.x.
- Dissanayake AJ, Phillips AJL, Hyde KD, Yan JY, Li XH. 2017. The current status of species in Diaporthe. Mycosphere. 8(5):1106–1156. doi:10.5943/mycosphere/8/5/5.
- Gebreil A, Micijevic A, Weber A, Hyronimus L, Mathew F. 2015. Characterization of Diaporthe species infecting soybeans (Glycine max L.) in South Dakota. Proc 100th Anniv South Dakota Acad Sci. 94:362.
- Ghimire K, Petrović K, Kontz BJ, Bradley CA, Chilvers MI, Mueller DS, Smith DL, Wise KA, Mathew FM. 2019. Inoculation method impacts symptom development associated with Diaporthe aspalathi, D. caulivora, and D. longicolla on soybean (glycine max). Plant Dis. 103(4):677–684. doi:10.1094/PDIS-06-18-1078-RE.
- Glass NL, Donaldson G. 1995. Development of primer sets designed for use with the PCR to amplify conserved genes from filamentous ascomycetes. Appl Environ Microbiol. 61(4):1323–1330. doi:10.1128/aem.61.4.1323-1330.1995.
- Gomes RR, Glienke C, Videira SIR, Lombard L, Groenewald JZ, Crous PW. 2013. Diaporthe: a genus of endophytic, saprobic and plant pathogenic fungi. Persoonia - Molecular Phylogeny and Evolution of Fungi. 31(1):1–41. doi:10.3767/003158513X666844.
- Gravert CE, Li S, Hartman GL. 2001. Occurrence of Diaporthe phaseolorum var. meridionalis on Soybean in Illinois Plant Dis. 85:1211.
- Guillin EA, Grijalba PE, Oliveira LO, Gottlieb AM. 2014. Specific boundaries between the causal agents of the soybean stem canker. Trop Plant Pathol. 39(4):316–325. doi:10.1590/S1982-56762014000400006.
- Hall TA. 1999. BioEdit: a user-friendly biological sequence alignment editor and analysis program for Windows 95/98/NT. Nucleic Acids Symp. 41:95–98.
- Harrington TC, Steimel J, Workneh F, Yang XB. 2000. Molecular identification of fungi associated with vascular discoloration of soybean in the North Central United States. Plant Dis. 84(1):83–89. doi:10.1094/PDIS.2000.84.1.83.
- Harrington TC, Steimel J, Workneh F, Yang XB. 2003. Characterization and distribution of two races of Phialophora gregata in the North-Central United States. Phytopathology®. 93(7):901–912. doi:10.1094/PHYTO.2003.93.7.901.
- Hartman GL, Rupe JC, Sikora EJ, Domier LL, Davis JA, Steffey KL, editors. 2015. Compendium of Soybean Diseases and Pests. 5th ed. ed. St Paul (MN): APS Press.
- Hill HC, Horn NL, Steffens WL. 1981. Mycelial development and control of Phomopsis sojae in artificially inoculated soybean stems. Plant Dis. 65:132–134. doi:10.1094/PD-65-132.
- Hobbs TW, Schmitthenner AF, Kuter GA. 1985. A New Phomopsis species from soybean. Mycologia. 77(4):535. doi:10.1080/00275514.1985.12025139.
- Hosseini B, El-Hasan A, Link T, Voegele RT. 2020. Analysis of the species spectrum of the Diaporthe/Phomopsis complex in European soybean seeds. Mycological Progress. 19(5):455–469. doi:10.1007/s11557-020-01570-y.
- Impullitti AE, Malvick DK. 2013. Fungal endophyte diversity in soybean. J Appl Microbiol. 114(5):1500–1506. doi:10.1111/jam.12164.
- Keeling BL. 1985. Soybean cultivar reactions to soybean stem canker caused by Diaporthe phaseolorum var. caulivora and pathogenic variation among isolates. Plant Dis. 69(2):132–133. doi:10.1094/PD-69-132.
- Koenning SR, Wrather JA. 2010. Suppression of soybean yield potential in the continental United States by plant diseases from 2006 to 2009. Plant Health Progress. 11(1):1–5. doi:10.1094/PHP-2010-1122-01-RS.
- Kontz B, Adhikari S, Subramanian S, Mathew FM. 2016. Optimization and application of a quantitative polymerase chain reaction assay to detect Diaporthe species in soybean plant tissue. Plant Dis. 100(8):1669–1676. doi:10.1094/PDIS-10-15-1204-RE.
- Kulik MM. 1984. Symptomless infection, persistence, and production of pycnidia in host and non-host plants by Phomopsis batatae, Phomopsis phaseoli, and Phomopsis sojae, and the taxonomic implications. Mycologia. 76(2):274–291. doi:10.1080/00275514.1984.12023837.
- Kumar S, Stecher G, Tamura K. 2016. MEGA7: molecular evolutionary genetics analysis version 7.0 for bigger datasets. Mol Biol Evol. 33(7):1870–1874. doi:10.1093/molbev/msw054.
- Leite TS, Cnossen-Fassoni A, Pereira OL, Mizubuti ESG, Araújo EF, Queiroz MV. 2013. Novel and highly diverse fungal endophytes in soybean revealed by the consortium of two different techniques. J Microbiol. 51(1):56–69. doi:10.1007/s12275-013-2356-x.
- Li S, Hartman GL, Boykin DL. 2010. Aggressiveness of Phomopsis longicolla and other Phomopsis spp. on soybean. Plant Dis. 94(8):1035–1040. doi:10.1094/PDIS-94-8-1035.
- Li S, Kurtzweil NC, Grau CR, Hartman GL. 2004. Occurrence of soybean stem canker (Diaporthe phaseolorum var. meridionalis) in wisconsin. Plant Disease. 88(5):576. doi:10.1094/PDIS.2004.88.5.576B.
- Lonergan E, Pasche J, Skoglund L, Burrows M. 2015. Sensitivity of ascochyta species infecting pea, lentil, and chickpea to boscalid, fluxapyroxad, and prothioconazole. Plant Dis. 99(9):1254–1260. doi:10.1094/PDIS-06-14-0620-RE.
- Lu X, Robertson AE, Byamukama EZ, Nutter FW Jr. 2010. Evaluating the importance of stem canker of soybean in Iowa. Plant Dis. 94(2):167–173. doi:10.1094/PDIS-94-2-0167.
- Malvick DK, Chen W, Kurle JE, Grau CR. 2003. Cultivar preference and genotype distribution of the brown stem rot pathogen Phialophora gregata in the midwestern United States. Plant Dis. 87(10):1250–1254. doi:10.1094/PDIS.2003.87.10.1250.
- Malvick DK, Grunden E. 2005. Isolation of fungal DNA from plant tissues and removal of DNA amplification inhibitors. Mol Ecol Notes. 5(4):958–960. doi:10.1111/j.1471-8286.2005.01044.x.
- Malvick D, Bussey K. 2008. Comparative analysis and characterization of the soybean sudden death syndrome pathogen Fusarium virguliforme in the northern U.S. Can J of Plant Pathol. 30:467–476. doi:10.1080/07060660809507544.
- Mathew FM, Castlebury LA, Alananbeh K, Jordahl JG, Taylor CA, Meyer SM, Lamppa RS, Pasche JA, Markell SG. 2015. Identification of Diaporthe longicolla on dry edible pea, dry edible bean, and soybean in North Dakota. Plant Heal Prog. 16(2):71–72. doi:10.1094/PHP-RV-14-0045.
- Mathew FM, Gulya TJ, Jordahl JG, Markell SG. 2017. First report of stem disease of soybean (Glycine max) caused by Diaporthe gulyae in North Dakota. Plant Dis. 102(1):240. doi:10.1094/PDIS-04-17-0506-PDN.
- Mena E, Stewart S, Montesano M, Ponce de León I. 2020. Soybean stem canker caused by Diaporthe caulivora; pathogen diversity, colonization process, and plant defense activation. Front Plant Sci. 10:1–21. doi:10.3389/fpls.2019.01733.
- Mengistu A, Castlebury LA, Rossman AY, Smith JR, Reddy KN. 2007. Isolates of Diaporthe-Phomopsis from weeds and their effect on soybean. Can J Plant Pathol. 29(3):283–289. doi:10.1080/07060660709507471.
- Miller WA, Roy KW. 1982. Mycoflora of soybean leaves, pods, and seeds in Mississippi. Can J Bot. 60(12):2716–2723. doi:10.1139/b82-331.
- Mueller DS, Wise KA, Sisson AJ, Smith DL, Sikora EJ, Robertson AE. editors. 2016. A farmer’s guide to soybean diseases. St Paul (MN): APS Press.
- Peltier AJ, Bradley CA, Chilvers MI, Malvick DK, Mueller DS, Wise KA, Esker PD. 2012. Biology, yield loss and control of Sclerotinia stem rot of soybean. Journal of Integrated Pest Management. 3(2):1–7. doi:10.1603/IPM11033.
- Peruzzo AM, Hernández FE, Pratta GR, Ploper LD, Pioli RN. 2019. Identification and inheritance of an Rdc gene resistance to soybean stem canker (Diaporthe phaseolorum var. caulivora). European Journal of Plant Pathology. 154(4):1179–1184. doi:10.1007/s10658-019-01716-z.
- Petrović K, Skaltsas D, Castlebury LA, Kontz B, Allen TW, Chilvers MI, Gregory N, Kelly HM, Koehler AM, Kleczewski NM, et al. 2021. Diaporthe seed decay of soybean [Glycine max (L.) Merr.] is endemic in the United States, but new fungi are involved. Plant Dis. 105(6):1621–1629. doi:10.1094/PDIS-03-20-0604-RE.
- Pioli RN, Morandi EN, Martínez MC, Lucca F, Tozzini A, Bisaro V, Hopp HE. 2003. Morphologic, molecular, and pathogenic characterization of Diaporthe phaseolorum variability in the core soybean-producing area of Argentina. Phytopathology®. 93(2):136–146. doi:10.1094/PHYTO.2003.93.2.136.
- Rupe JC. 1990. Effect of temperature on the rate of infection of soybean seedlings by Phomopsis longicolla. Can J Plant Pathol. 12(1):43–47. doi:10.1080/07060669009501041.
- Santos JM, Vrandečić K, Ćosić J, Duvnjak T, Phillips AJL. 2011. Resolving the Diaporthe species occurring on soybean in Croatia. Persoonia - Molecular Phylogeny and Evolution of Fungi. 27(1):9–19. doi:10.3767/003158511X603719.
- Sinclair JB. 1988. Diaporthe/phomopsis complex of soybeans. In: Wyllie TD, Scott DH, editors. Soybean diseases of the north central region. St Paul (MN): APS Press; p. 96–101.
- Sinclair JB. 1993. Phomopsis seed decay of soybeans- A prototype for studying seed disease. Plant Dis. 77(4):329. doi:10.1094/PD-77-0329.
- Smith EF, Backman PA. 1988. Soybean stem canker: an overview. In: Wyllie TD, Scott DH, editors. Soybean diseases of the north central region. St. Paul (MN): APS Press; p. 47–55.
- Soto-Arias JP, Munkvold GP. 2011. Effects of virus infection on susceptibility of soybean plants to Phomopsis longicolla. Plant Dis. 95(5):530–536. doi:10.1094/PDIS-10-10-0767.
- Tamura K. 1992. Estimation of the number of nucleotide substitutions when there are strong transition-transversion and G+C-content biases. Mol Biol Evol. 9(4):678–687. doi:10.1093/oxfordjournals.molbev.a040752.
- Tormen NR, Blum LEB. 2020. Row spacing, cultivar and fungicide application affect the incidence of soybean stem blight. Bioscience Journal. 36(5):1557–1563. doi:10.14393/BJ-v36n5a2020-42123.
- Udayanga D, Liu X, Crous PW, McKenzie EHC, Chukeatirote E, Hyde KD. 2012. A multi-locus phylogenetic evaluation of Diaporthe (Phomopsis). Fungal Diversity. 56(1):157–171. doi:10.1007/s13225-012-0190-9.
- Udayanga D, Castlebury LA, Rossman AY, Chukeatirote E, Hyde KD. 2015. The Diaporthe sojae species complex: phylogenetic re-assessment of pathogens associated with soybean, cucurbits and other field crops. Fungal Biol. 119(5):383–407. doi:10.1016/j.funbio.2014.10.009.
- van Niekerk JM, Groenewald JZ, Farr DF, Fourie PH, Halleen F, Crous PW, and 2005. Reassessment of Phomopsis species on grapevines. Australasian Plant Pathology. 34:27–39. doi:10.1071/AP04072.
- Vidić M, Jasnic S, Petrović K. 2011. Diaporthe/Phomopsis species on soybean in Serbia. Pesticidi i fitomedicina. 26(4):301–315. doi:10.2298/PIF1104301V.
- White TJ, Bruns TD, Lee SB, Taylor JW. 1990. Amplification and direct sequencing of fungal ribosomal RNA genes for phylogenetics. In: Innis MA, Gelfand DH, Sninsky JJ, White TJ, editors. PCR Protocols, A Guide to Methods and Applications. New York (NY): Academic Press; p. 315–322.
- Xue AG, Morrison MJ, Cober E, Anderson TR, Rioux S, Ablett GR, Rajcan I, Hall R, Zhang JX. 2007. Frequency of isolation of species of Diaporthe and Phomopsis from soybean plants in Ontario and benefits of seed treatments. Can J Plant Pathol. 29(4):354–364. doi:10.1080/07060660709507481.
- Yang XB. 2001. Biology and management of premature yellowing and death of soybean caused by Phomopsis idiaporthe fungi. Proc Int Crop Mgmt Conf. 23:181–185.
- Zaw M, Aye SS, Matsumoto M. 2020. Colletotrichum and Diaporthe species associated with soybean stem diseases in Myanmar. Journal of General Plant Pathology. 86(2):114–123. doi:10.1007/s10327-019-00902-5.
- Zhang AW, Hartman GL, Riccioni L, Chen WD, Ma RZ, Pedersen WL. 1997. Using PCR to Distinguish Diaporthe phaseolorum and Phomopsis longicolla from Other Soybean Fungal Pathogens and to Detect Them in Soybean Tissues. Plant Dis. 81(10):1143–1149. doi:10.1094/PDIS.1997.81.10.1143.
- Zhang AW, Hartman GL, Curio-Penny B, Pedersen WL, Becker KB. 1999. Molecular Detection of Diaporthe phaseolorum and Phomopsis longicolla from Soybean Seeds. Phytopathology®. 89(9):796–804. doi:10.1094/PHYTO.1999.89.9.796.