Abstract
Three Macrophomina species (M. phaseolina, M. pseudophaseolina, and M. euphorbiicola) associated with various crops worldwide have been distinguished to date by DNA sequence analysis; however, no studies have been conducted to identify Macrophomina species occurring on chickpea in Mexico. The aims of this study were to identify Macrophomina isolates associated with chickpea wilt through the use of species-specific primers, as well as to determine their virulence and mycelial compatibility. During the 2019 growing season, 58 Macrophomina isolates were obtained from symptomatic plants collected from 19 chickpea crops distributed in the states of Sinaloa and Sonora, Mexico. The identity of all 58 isolates was determined by PCR using three sets of primers specific to three Macrophomina species (M. phaseolina, M. pseudophaseolina, and M. euphorbiicola). Virulence was determined by inoculating chickpea seedling roots with a mycelial suspension and disease severity was assessed 30 days after inoculation. Molecular detection with species-specific primers indicated that all isolates belong to M. phaseolina, with significant differences found in their virulence. Mycelial compatibility testing showed that there are at least six mycelial compatibility groups of M. phaseolina distributed in chickpea fields in Sinaloa and Sonora. This information will serve as a basis for future studies on the epidemiology and management of the disease caused by M. phaseolina on chickpea in Mexico.
Résumé: Trois espèces de Macrophomina (M. phaseolina, M. pseudophaseolina et M. euphorbiicola), associées à diverses cultures dans le monde, ont été différenciées à ce jour par l’analyse des séquences de l’ADN. Toutefois, aucune étude n’a été menée pour identifier l’espèce de Macrophomina qui vit sur les pois chiches au Mexique. Les buts de cette étude étaient d’identifier les isolats de Macrophomina associés à la flétrissure du pois chiche grâce à l’utilisation d’amorces spécifiques des espèces, de même que de déterminer leur virulence et leur compatibilité mycélienne. Durant la saison de croissance de 2019, 58 isolats de Macrophomina ont été obtenus de plants symptomatiques collectés dans 19 cultures de pois chiches des États du Sinaloa et du Sonora, au Mexique. L’identité des 58 isolats a été déterminée par PCR en utilisant trois jeux d’amorces spécifiques de trois espèces de Macrophomina (M. phaseolina, M. pseudophaseolina et M. euphorbiicola). La virulence a été établie en inoculant des racines de plantules de pois chiches avec une suspension de mycélium, puis la gravité de la maladie a été évaluée 30 jours après l’inoculation. La détection moléculaire avec les amorces spécifiques des espèces a indiqué que tous les isolats appartenaient à M. phaseolina, mais que leur degré de virulence variait significativement. Les essais sur la compatibilité mycélienne ont démontré qu’il y a au moins six groupes de compatibilité mycélienne de M. phaseolina répartis dans les champs de pois chiches du Sinaloa et du Sonora. Cette information servira de référence aux prochaines études sur l’épidémiologie et la gestion de la maladie causée par M. phaseolina chez les pois chiches au Mexique.
Introduction
Chickpea (Cicer arietinum L.), the only cultivated species of the genus Cicer, is native to eastern Turkey. It is also the third most important legume worldwide, with 96% of production in developing countries. Mexico ranks 8th in the world with a production of202846tons; the main producing states include Sinaloa, Sonora, Michoacán, Guanajuato, and Baja California Sur, with the first two contributing the highest percentage (79%) of production (SIAP Citation2020). Chickpea is valued for the high protein content in its seed and is used increasingly as a substitute for animal protein (Shinde and Hunje Citation2019). There are two different types of chickpeas: desi and kabuli (white); the latter is considered economically more important and receives a higher price in the market compared with the desi type (Jendoubi et al. Citation2017).
Among the main limiting factors in chickpea production are root rot and wilt caused by a complex of soilborne fungi such as Fusarium spp., Macrophomina phaseolina (Tassi) Goid., Sclerotium rolfsii Sacc., and Rhizoctonia solani Kühn (Fierros-Leyva et al. Citation2019). This complex of fungi can cause yield losses of up to 80% in Mexico (Padilla-Valenzuela et al. Citation2008).
Macrophomina phaseolina is a seed- and soilborne fungus with great morphological, physiological, pathogenic, and genetic variability, which increases its adaptability to various environmental conditions (Beas-Fernández et al. Citation2006; Reyes-Franco et al. Citation2006; Marquez et al. Citation2021). This fungus belongs to the family Botryosphaeriaceae and attacks more than 500 plant species (Kaur et al. Citation2012; Sarr et al. Citation2014), including economically important hosts such as soybean (Glycine max (L.) Merr.), common bean (Phaseolus vulgaris L.), potato (Solanum tuberosum L.), maize (Zea mays L.), sorghum (Sorghum bicolor (L.) Mönch.), peanut (Arachis hypogaea L.), chilli (Capsicum annuum L.), chickpea, cotton (Gossypium hirsutum L.), and canola (Brassica napus L.) (Leyva-Mir et al. Citation2015). Disease symptoms caused by M. phaseolina include poor growth, yellowing, and wilting (Dhingra and Sinclair Citation1978; Manjunatha and Saifulla Citation2018); these occur because the pathogen affects the roots and invades the xylem vessels, thereby blocking the water flow in the plant (Muñoz-Cabañas et al. Citation2005). In addition, phytotoxic metabolites produced by M. phaseolina have been described, including phaseolinon, botryodiplodin, and patulin, which are believed to play a role in the initial stages of infection, causing wilting of seedlings and formation of necrotic lesions on leaves and roots (Abbas et al. Citation2020; Salvatore et al. Citation2020; Marquez et al. Citation2021).
Several studies have associated M. phaseolina as the only Macrophomina species causing root rot and wilt symptoms in chickpea (Díaz-Franco and Montes-García Citation2008; Ávila-Miramontes et al. Citation2015; Khan et al. Citation2017; Lakhran et al. Citation2018; Živanov et al. Citation2019). In India, this pathogen can cause yield losses of 10% to 25% in the crop (Manjunatha et al. Citation2013). However, three cryptic species of Macrophomina (M. phaseolina, M. pseudophaseolina Crous, Sarr & Ndiaye, and M. euphorbiicola Machado, Soares & Pereira) have been recently recognized worldwide. These species can only be distinguished by using species-specific primers (Santos et al. Citation2020) or by multilocus phylogenetic analysis with combined sequence data from the internal transcribed spacer (ITS) region, as well as fragments of genes such as actin (ACT), β-tubulin (βT), calmodulin (CAL), and translation elongation factor 1-alpha (TEF-1α) (Sarr et al. Citation2014; Machado et al. Citation2019).
Mycelial compatibility has been used to assess genetic diversity among M. phaseolina isolates in various countries (Barzegar and Banihashemi Citation2019; Csöndes Citation2011; Csüllög and Tarcali Citation2020). In Mexico, there are no studies on the mycelial compatibility of Macrophomina isolates, and the identity of the different species has not been verified with species-specific primers for each of the three species described worldwide. The objectives of this research were to identify Macrophomina isolates associated with root rot and wilt symptoms on chickpea plants in fields in Sinaloa and Sonora, Mexico, by using species-specific primers of the TEF1-α gene, in addition to estimating virulence and determining the mycelial compatibility of fungal isolates.
Materials and methods
Sample collection
During the 2019 growing season, chickpea plants (cv. Blanco Sinaloa ´92) with chlorosis, root rot, and wilt symptoms () were collected from 18 commercial fields distributed in the municipalities of Guasave, Mocorito, Salvador Alvarado, Angostura, and Culiacán in the state of Sinaloa, Mexico. In addition, diseased plants were collected from a site located in the municipality of Hermosillo, Sonora, Mexico. Samples were collected from each field at three phenological stages (leaf growth, flowering, and pod fill).
Isolation, purification, and preservation of fungi
Macrophomina isolates were obtained using the procedures described by Crous et al. (Citation2009). For isolation, 5-mm long pieces of diseased root tissue were cut, surface disinfested by immersion in 2% sodium hypochlorite (NaClO) for 2 min, rinsed twice in sterile distilled water for 1 min, and dried with sterile absorbent paper. Subsequently, the disinfested pieces were placed in Petri dishes with potato dextrose agar (PDA) culture medium (Difco®, USA). The Petri dishes were incubated at 25°C in the dark for 72 h and then mycelial plugs (5 mm in diameter) from the edge of actively growing Macrophomina-like colonies were transferred to Petri dishes with fresh PDA and incubated at 25°C for seven days. To verify the identity of the isolates, morphology of mycelia and microsclerotia of all isolates was examined using light microscopy. Pure cultures were obtained using the hyphal-tip technique on water agar (WA) culture medium and transferred to plates with PDA medium. The Macrophomina isolates used in this study were deposited in the Culture Collection of Phytopathogenic Fungi at the Centro de Investigación en Alimentación y Desarollo (Culiacán, Sinaloa, Mexico) under the accession nos. CCLF20 to CCLF77. Mycelial plugs of the fungal isolates were maintained in sterile distilled water at 4°C and in 10% glycerol at −80°C.
DNA extraction and PCR amplification
Genomic DNA was extracted according to the CTAB method (Doyle and Doyle Citation1990). Aerial mycelium from 7-day-old Macrophomina colonies cultured on PDA medium was scraped with a sterile spatula, macerated in a sterile mortar using liquid nitrogen, and transferred to a 1.5 mL microcentrifuge tube containing 500 µL of buffer solution (100 mM Tris, pH 8; 20 mM of EDTA, pH 8; 1.4 M NaCl; 3% CTAB; 0.2 mg mL−1 of proteinase K), vortexed for 10s and incubated for 60 min at 65°C. Then, 700 µL of chloroform-isoamyl alcohol (24:1 v/v) was added, vortexed for 10s and placed in a 5810 R centrifuge (Eppendorf®, USA) at13000g for 10 min. The supernatant was transferred to a 1.5 mL microcentrifuge tube and 700 µL of isopropanol was added. The tubes were mixed by inversion four to five times and stored at −20°C for 10 min, then centrifuged at13 000 g for 10 min and the supernatant was discarded. Subsequently, 500 µL of 70% ethanol was added to each tube, centrifuged at13 000 g for 5 min, and the supernatant was again discarded. The tubes were placed on blotting paper until the ethanol evaporated, and when the pellet was dry, 100 µL of DNasa/RNasa-free distilled water was added. DNA quality and concentration were quantified with a Q‒3000 UV spectrophotometer (Quawell®, USA). The DNA was stored at −20°C for later use.
For the PCR analysis, we used species-specific primers targeting the translation elongation factor 1-α (TEF1-α) gene of M. phaseolina (MpTefF‒AAACACACTTTTCGCACTCCTGC, MpTefR‒ TATGCTCGCAGAGAAGAACACGA), M. pseudophaseolina (MsTefF‒GCACACTTTTCGCGCTTCTGTA, MsTefR‒TGTGCTCGCTGGGAAGAACATGA), and M. euphorbiicola (MeTefF‒AAGCATACTTTTCGTGCTCCTGC, MeTefR‒ AAAGGAACATGAGTGGCCAAAAA), which were designed and reported by Santos et al. (Citation2020). The reaction mixture was prepared with a final volume of 25 µL, 1x PCR buffer, 2.5 mM MgCl2, 0.2 mM dNTP, 0.4 µM of each primer, 0.04 U DNA polymerase (Promega®, USA) and 4 ng of DNA. PCR was carried out in a C-1000 thermal cycler (Bio-Rad®, USA) under the following conditions: an initial denaturation step at 95°C for 3 min, followed by 35 cycles of denaturation at 95°C for 30s, annealing for 30s at 67, 69, or 65°C for the primers MpTefF/MpTefR, MsTefF/MsTefR, and MeTefF/MeTefR, respectively; extension at 72°C for 1 min and a final extension step at 72°C for 10 min. The amplified products were verified by 1% agarose gel electrophoresis with 1X TAE buffer and stained with ethidium bromide. To run electrophoresis at 90 volts, 7 µL of the PCR product and 3 µL of loading buffer were used. The gel was analysed on a Gel Doc XR + Gel Documentation System transilluminator (Bio-Rad®, USA). Positive controls of DNA for M. phaseolina, M. pseudophaseolina, and M. euphorbiicola were kindly provided by Dr. Sami Jorge Michereff (Universidade Federal do Cariri, Brazil).
Pathogenicity and virulence test
The pathogenicity of Macrophomina isolates was verified by inoculating chickpea seedlings of a susceptible genotype (Blanco Sinaloa ´92) under greenhouse conditions according to the procedures described by Robles-Yerena et al. (Citation2016) and Jarek et al. (Citation2018), with some modifications. For inoculum preparation, each Macrophomina isolate was cultured in PDA medium at 25°C for 10 days. The mycelial growth was scraped with a slide, placed in sterile distilled water, and liquefied for 10s using a waring blender. The inoculum suspension was adjusted to a concentration of 1 × 105 mycelial fragments mL−1 and Tween 20® was added. Chickpea seeds were sown in 128-cavity polystyrene trays (60 mL per cavity) containing an autoclaved mixture of peat moss and sand (2:1). Seedlings were regularly watered to keep the growth substrate in a wet condition. Fifteen-day-old chickpea seedlings were carefully removed from their cavities.
The roots were washed with sterile distilled water and inoculated by immersion in the mycelial suspension for 30 min. Once the time had elapsed, the seedlings were placed again in trays with sterile substrate and kept in a greenhouse at a temperature of 25 to 35°C. Each isolate was inoculated on seven plants and the experiment was repeated twice. The roots of 10 control seedlings were immersed in sterile distilled water.
The observation of symptom progress was performed daily and the evaluation of disease severity was carried out 30 days after inoculation using a 5-category visual scale, where 0 = no visible symptoms, 1 = less than 25% foliage diseased, 2 = 25 to 50% foliage diseased, 3 = 50 to 75% foliage diseased, 4 = more than 75% foliage diseased. The scale values were transformed to percent values and virulence assay data were analysed. Normality and homogeneity of variances were first checked through Shapiro–Wilk test and Levene test, respectively. Variances of the two experiments were not statistically different for each test; therefore, the raw data for the two repeats of each experiment were combined for subsequent analysis. Then, data were subjected to analyses of variance (ANOVA) and means were compared by Fisher’s least significant difference (LSD) test, at 5% probability using PROC GLM in SAS (version 9.3; SAS Institute, Cary, NC). Isolates were treated as fixed effects. Uninoculated controls were excluded from statistical analysis.
Mycelial compatibility
Mycelial compatibility tests for the 58 Macrophomina isolates were carried out in Petri dishes (90 mm in diameter) containing PDA medium. A mycelial plug (6 mm in diameter) from five different isolates was individually placed on the periphery of a Petri dish and one different isolate was placed in the centre. The 58 Macrophomina isolates were tested in combinations with three replicates for each combination. Each isolate was paired with 57 other isolates and itself at least three times. Petri dishes were incubated at 25°C in constant darkness and the union of colonies was evaluated at 7, 10, and 14 days. The relationship was defined visually based on Csöndes (Citation2011); it was considered compatible when the mycelia grew into each other and one continuous colony was created, whereas, the relationship was considered incompatible when a clearly defined barrier (blocking zone) was formed between the colonies and some of the hyphae were destroyed. Mycelial compatibility groups (MCGs) were identified based on data from compatible reactions among tested isolates. The entire experiment was performed twice. Isolates that had conflicting results between replications were paired with each other a fourth time.
Results
Fungal isolates
A total of 58 Macrophomina isolates were obtained from chickpea plants with chlorosis and wilt symptoms across 19 fields distributed in the municipalities of Salvador Alvarado (17 isolates), Mocorito (11 isolates), Guasave (6 isolates), Angostura (3 isolates), Culiacán (1 isolate) in Sinaloa, as well as in Hermosillo (20 isolates) in Sonora.
Identification with species-specific primers
Identification of Macrophomina isolates associated with chickpea wilt in Sinaloa and Sonora, Mexico with species-specific TEF1-α gene primers indicated that all 58 isolates were M. phaseolina. This was because only the expected 217-bp PCR amplicon of the species-specific primers for M. phaseolina (MpTefF/MpTefR) was obtained (Santos et al. Citation2020), but there was no amplification when using the species-specific primers for M. pseudophaseolina (MsTefF/MsTefR) and M. euphorbiicola (MeTefF/MeTefR).
Pathogenicity and virulence
All inoculated chickpea plants showed chlorosis symptoms, wilting, and premature defoliation 30 days after inoculation (), while control plants remained disease-free (), confirming the pathogenicity of the 58 Macrophomina phaseolina isolates. Statistical analysis showed significant differences (P ≤ 0.05) between the virulence of fungal isolates (). The most virulent isolates (>70% severity) were recorded in chickpea fields in the municipalities of Salvador Alvarado, Mocorito, and Guasave, while the less virulent isolates (<60% severity) occurred at Salvador Alvarado, Mocorito, Guasave, and Angostura in the state of Sinaloa. Of the 20 isolates obtained from a chickpea field in the municipality of Hermosillo, in the state of Sonora, three showed a mean severity of greater than 70%, 14 isolates had a mean of 60 to 70%, and three isolates had a mean <60% ().
Fig. 2 Chickpea plants cv. Blanco Sinaloa 92 artificially inoculated with Macrophomina phaseolina. (a) Symptoms on chickpea plants, 30 days after inoculation. (b) Control plants.
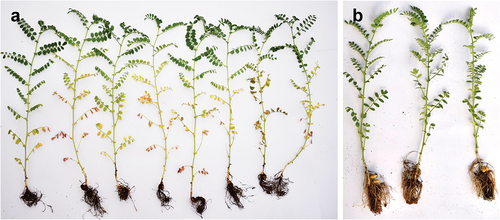
Fig. 3 Disease severity caused by 58 Macrophomina phaseolina isolates associated with chickpea wilt in the Northwest region of Mexico (States of Sinaloa and Sonora), 30 days after inoculation onto roots of chickpea cv. Blanco Sinaloa. Error bars represent standard error. Columns with the same letter do not differ significantly, according to Fisher’s LSD test (P ≤ 0.05).
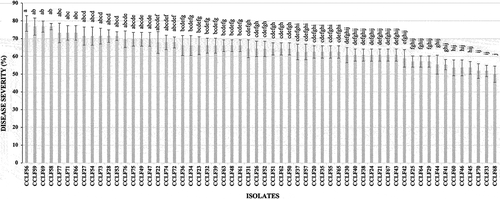
Mycelial compatibility
The compatibility tests among the 58 M. phaseolina isolates showed a total of six MCGs, which are shown in . Forty-two isolates (72.4%) were assigned to MCG-I, five isolates (8.62%) to MCG-II, four isolates (6.89%) to MCG-III, three isolates (5.17%) to MCG-IV, two isolates (3.44%) to MCG-V, and two isolates (3.44%) to MCG-VI.
Table 1. Origin, mycelial compatibility groups (MCGs) of Macrophomina phaseolina isolates collected from chickpea plants in Sinaloa and Sonora, Mexico.
MCG-I had a wide geographical distribution and occurred in all municipalities except at Culiacán, Sinaloa. In contrast, the remaining five MCGs had a limited geographical distribution. MCG-II was found at Mocorito and Angostura municipalities from Sinaloa state, as well as at Hermosillo from Sonora state. MCG-III was recorded at Culiacán, Salvador Alvarado, and Hermosillo (Sonora). MCG-IV occurred only at Guasave, Sinaloa and Hermosillo, Sonora. MCG-V was found only at Mocorito and Salvador Alvarado in Sinaloa. MCG-VI was recorded at Salvador Alvarado, Sinaloa and Hermosillo, Sonora.
Regarding sampled states, all MCGs were identified in Sinaloa, whereas MCG-V was not present in Sonora; however, it was not possible to relate the mycelial compatibility grouping and geographic distribution of the isolates because of the small number of isolates analysed.
Discussion
This study determined, by using species-specific primers amplifying the TEF-1α gene, that M. phaseolina is the predominant Macrophomina species in chickpea fields in the states of Sinaloa and Sonora, Mexico. Previous studies identified three Macrophomina species (M. phaseolina, M. pseudophaseolina, and M. euphorbiicola) by multilocus phylogenetic analyses using combined ITS, ACT, βT, CAL, and TEF1-α sequence data (Sarr et al. Citation2014; Machado et al. Citation2019) as well as phylogenetic analyses using only sequences from the ITS region (Wagan et al. Citation2019; Pandy et al. Citation2020). However, Machado et al. (Citation2019) and Santos et al. (Citation2020) indicated that ITS sequences are not sufficient to distinguish between the three Macrophomina species. Species-specific primers that amplify a fragment of the TEF1-α gene are just as effective, but much cheaper, faster, and easier to use compared to multilocus phylogenetic analyses, which are laborious and expensive; they also require time and knowledge of phylogenetic identification for species distinction (Santos et al. Citation2020). Similarly, Negreiros et al. (Citation2019) reported that the use of species-specific primers showed potential to be used as a tool to identify known species of Macrophomina and other Botryosphaeriaceae species.
All M. phaseolina isolates evaluated in this study were pathogenic to inoculated chickpea plants and the symptoms observed were root rot, chlorosis, and premature defoliation. Regarding virulence, M. phaseolina isolates showed significant differences and were grouped into three categories. These differences may be due to the fact that M. phaseolina is highly variable because of the large number of polykaryotic characteristics of the mycelium, mutation, hyphal fusion, and mitotic recombination; this pathogenic variability has been described in different hosts, including soybean and sunflower (Helianthus annuus L.) (Dhingra and Sinclair Citation1978; Jiménez et al. Citation1983). There are several studies similar to ours in which the pathogenicity M. phaseolina has been reported in other plant hosts with differences in virulence, which may vary depending on the region of origin of the isolates (Echávez and Perdomo Citation1991; Mayek-Pérez et al. Citation2001; Muñoz-Cabañas et al. Citation2005; Aghakhani et al. Citation2009; Manjunatha and Saifulla Citation2018; Negreiros et al. Citation2019; García et al. Citation2019).
In the present study, six MCGs were identified, indicating a high level of genetic variability amoung Mexican Macrophomina phaseolina isolates. Mayék-Pérez et al. (Citation2001) and Muñoz-Cabañas et al. (Citation2005) obtained high percentages of polymorphism by means of AFLP analysis in M. phaseolina isolates from Mexico, which revealed the existence of great genetic diversity, showing the polykaryotic nature or the possibility of genetic recombination in this plant pathogenic fungus. Similarly, Barzegar and Banihashemi (Citation2019) reported a total of 33 different MCGs of M. phaseolina in Iran, showing the existence of high genetic diversity among the fungal isolates. In contrast, Csöndes (Citation2011) registered high compatibility among 53 M. phaseolina isolates obtained from sunflower, maize, and soybean in Serbia, Hungary, and Spain. This result is significant since mycelial compatibility is a precondition for DNA exchange through anastomosis between two compatible isolates, which increases the possibility of parasexual recombination and therefore the maintenance of genetic diversity in M. phaseolina (Almeida et al. Citation2003; Krnjaja et al. Citation2013).
Our results showed that five of six MCGs occurred in Sonora, and MCG-V was only present in Sinaloa, indicating that the MCGs could be distributed at different geographical sites in the states of Sinaloa and Sonora; however, further studies examining a large number of isolates from several MCGs are required in order to resolve this uncertainty. The variability of a pathogen in a geographic area can be estimated through this type of study, in addition to other factors that can provide information on this variation, such as rapid growth, mycelial dispersion, and the abundant production of microsclerotia (Purkayastha et al. Citation2006). Other authors such as Mihail and Taylor (Citation1995), Vandemark et al. (Citation2000), Csöndes (Citation2011) Csüllög and Tarcali (Citation2020), stated that identical or similar fungal genotypes can spread over long distances via transport of infected seed and material with resistance structures (microsclerotia) of the fungus, which play a very important role in the dissemination and propagation.
In conclusion, molecular detection by amplification of a fragment of the TEF1-α gene using species-specific primers revealed that all 58 Macrophomina isolates from chickpea were M. phaseolina. Inoculation of the fungus onto chickpea roots indicated that all isolates were pathogenic and there is a difference in their virulence. In addition, the mycelial compatibility test showed that some isolates had the ability to fuse hyphae, giving a total of six MCGs among the 58 isolates of M. phaseolina obtained from chickpea fields in the states of Sinaloa and Sonora, Mexico. These findings have important implications and further studies on the impact, epidemiology, fungicide sensitivity, and disease resistance are needed for the development of suitable management strategies for the disease in Mexican chickpea fields.
Acknowledgements
We thank Rosalía López-Corrales, Edén Salenko Guerrero-Soto, and Alsino Villarreal-Wong for providing assistance during the pathogenicity tests. We also thank the anonymous reviewers for their critical comments and suggestions for improving the manuscript.
Disclosure statement
No potential conflict of interest was reported by the author(s).
References
- Abbas HK, Bellaloui N, Butler AM, Nelson JL, Abou-Karam M, Shier WT. 2020. Phytotoxic responses of soybean (Glycine max L.) to botryodiplodin, a toxin produced by the Charcoal Rot Disease Fungus, Macrophomina phaseolina. Toxins. 12:25. doi:10.3390/toxins12010025.
- Aghakhani M, Dubey SC. 2009. Determination of genetic diversity among Indian isolates of Rhizoctonia bataticola causing dry root rot of chickpea. Antonie van Leeuwenhoek. 96:607‒619. doi:10.1007/s10482-009-9375-y.
- Almeida AMR, Abdelnoor RV, Arrabal-Arias CA, Calvalho VP, Jacoud-Filho SS, Marín SRR, Benato LC, Pinto MC, Carvalho CGP. 2003. Genotypic diversity among Brazilian isolates of Macrophomina phaseolina revealed by RAPD. Fitopatol Bras. 28:279–285. doi:10.1590/S0100-41582003000300009.
- Ávila-Miramontes JA, Padilla-Zaldo G, Martínez-Heredia D, Rivas-Santoyo FJ, Coronado-Espericueta, Ortega-Murrieta P. 2015. Respuesta de algunos componentes del rendimiento del cultivo de garbanzo (Cicer arietinum L.) a la inoculación de Mesorhizobium ciceri, Trichoderma harzianum y Bacillus subtilis en la región agrícola de la Costa de Hermosillo. Rev Cienc Biol Salud. 17(3):3‒8. doi:10.18633/bt.v17i3.212.
- Barzegar FM, Banihashemi Z. 2019. Mycelial compatibility groups of Macrophomina phaseolina in Iran. Iran J Plant Pathol. 55(2):115–128. doi:10.22034/ijpp.2019.37319.
- Beas-Fernández R, De Santiago-De Santiago A, Hernández-Delgado S, Mayék-Pérez N. 2006. Characterization of Mexican and non-Mexican isolates of Macrophomina phaseolina based on morphological characteristics, pathogenicity on bean seeds and endoglucanase genes. J Plant Pathol. 88(1):53‒60. [accessed 2021 Nov 30]. https://www.jstor.org/stable/41998280
- Crous PW, Verkley GJM, Groenewald JZ, Samson RA. 2009. Fungal biodiversity. CBS laboratory manual series. Utrecht: Westerdijk Fungal Biodiversity Institute, p. 269.
- Csöndes I. 2011. Mycelial compatibility of Hungarian Macrophomina phaseolina isolates. Acta Agronomica Hungarica. 59(4):371–377. doi:10.1556/AAgr.59.2011.4.8.
- Csüllög K, Tarcali G. 2020. Investigation of the mycelial compatibility of Macrophomina phaseolina. Folia Oecologica. 47(2):153–158. doi:10.2478/foecol-2020-0018.
- Dhingra OD, Sinclair JB. 1978. Biology and pathology of Macrophomina phaseolina. Viçosa (Brazil): Universidade Federal de Viçosa. p 116.
- Díaz-Franco A, Montes-García N. 2008. La fitopatología en la región semiárida de Tamaulipas, México: reseña histórica. Rev Mex Fitopatol. 26(1):62‒70.
- Doyle JJ, Doyle JL. 1990. Isolation of plant DNA from fresh tissue. Focus. 12:13–15.
- Echávez BR, Perdomo F. 1991. Characterization and comparative pathogenicity of two Macrophomina phaseolina isolates from Puerto Rico. J Agric Univ P R. 75(4):419‒421.
- Fierros-Leyva GA, Acosta-Gallegos JA, Ortega-Murrieta PF, Padilla-Valenzuela I, Álvarez-Bravo A, Ramírez-Soto M, Velarde-Félix S. 2019. Distribución de hongos asociados a pudriciones de raíz del garbanzo. Rev Mex Cienc Agríc. 10(1):131‒142. doi:10.29312/remexca.v10i1.1730.
- García MFM, Souza ES, Silva JDD, Melo MP, Mota JM, Almeida NAD, Gomes RLF, Beserra JJEA. 2019. Reaction of Lima bean genotypes to Macrophomina phaseolina. Summa Phytopathol. 45(1):11‒17. doi:10.1590/0100-5405/185340.
- Jarek TM, Dos Santos AF, Tessmann DJ, Vieira ESN. 2018. Inoculation methods and aggressiveness of five Fusarium species against peach palm. Cienc Rural. 48(4):e20170462. doi:10.1590/0103-8478cr201704612.
- Jendoubi W, Bouhadida M, Boukteb A, Beji M, Kharrat M. 2017. Fusarium wilt affecting chickpea crop. Agriculture. 23(7):1–16. doi:10.3390/agriculture7030023.
- Jiménez-Diaz RM, Blanco-Lopez MA, Sackston WE. 1983. Incidence and distribution of charcoal rot of sunflower caused by Macrophomina phaseolina in Spain. Plant Dis. 67:1033‒1036. doi:10.1094/PD-67-1033.
- Kaur S, Singh DG, Kaur BS, Edward VG, Chand R, Bahadur CV. 2012. Emerging phytopathogen Macrophomina phaseolina: biology, economic importance and current diagnostic trends. Crit Rev Microbiol. 38(2):136‒151. doi:10.3109/1040841X.2011.640977.
- Khan AN, Shair F, Malik K, Hayat Z, Khan MA, Hafeez FY, Hassan MN. 2017. Molecular identification and genetic characterization of Macrophomina phaseolina strains causing pathogenicity on sunflower and chickpea. Front Microbiol. 8:1309. doi:10.2298/plf1303157k.
- Krnjaja V, Lević J, Stanković S, Vasić T. 2013. The use of vegetative compatibility test for identification of biodiversity of phytopathogenic fungi. Pestic Phytomed. 28(3):157‒165. doi:10.2298/PIF1303157K.
- Lakhran L, Ahir RR, Choudhary M, Choudhary S. 2018. Isolation, purification, identification and pathogenicity of Macrophomina phaseolina (Tassi) goid caused dry root rot of chickpea. J Pharmacogn Phytochem. 7:3314–3317.
- Leyva-Mir SG, Velázquez-Martínez GC, Tlapal-Bolaños B, Tovar-Pedraza JM, Rosas-Saito GH, Alvarado-Gómez OG. 2015. Caracterización morfológica y molecular de aislados de Macrophomina phaseolina asociados a caña de azúcar en México. Rev Argent Microbiol. 47(2):143‒147. doi:10.1016/j.ram.2015.03.003.
- Machado AR, Pinho DB, Soares DJ, Gomes AAM, Pereira OL. 2019. Bayesian analyses of five gene regions reveal a new phylogenetic species of Macrophomina associated with charcoal rot on oilseed crops in Brazil. Eur J Plant Pathol. 153(1):89–100. doi:10.1007/s10658-018-1545-1.
- Manjunatha SV, Naik MK, Khan MFR, Goswami RS. 2013. Evaluation of bio-control agents for management of dry root rot of chickpea caused by Macrophomina phaseolina. Crop Prot. 45:147‒150. doi:10.1016/j.cropro.2012.09.003.
- Manjunatha H, Saifulla M. 2018. Variation in virulence of Macrophomina phaseolina isolates causing dry root rot of chickpea and performance of chickpea genotypes against this disease. Legume Res. 41(3):468‒473. doi:10.18805/LR-3745.
- Marquez N, Giachero ML, Declerck S, Ducasse DA. 2021. Macrophomina phaseolina: general characteristics of pathogenicity and methods of control. Front Plant Sci. 12:634397. doi:10.3389/fpls.2021.634397.
- Mihail JD, Taylor SJ. 1995. Interpreting of variability among isolates of Macrophomina phaseolina in pathogenicity, pycnidium production, and chlorate utilization. Can J Bot. 73:1596‒1603. doi:10.1139/b95-172.
- Muñoz-Cabañas RM, Hernández-Delgado S, Mayék-Pérez N. 2005. Análisis patogénico y genético de Macrophomina phaseolina (Tassi) Goid. en diferentes hospedantes. Rev Mex Fitopatol. 23(1):11‒18.
- Myék-Pérez N, López-Castañeda C, González-Chavira M, García-Espinosa R, Acosta-Gallegos JA, Martínez-de la Vega O, Simpson J. 2001. Variability of Mexican isolates of Macrophomina phaseolina on bases of pathogenesis and AFLP genotype. Physiol Mol Plant Path. 59:257‒264. doi:10.1006/pmpp.2001.0361.
- Negreiros AMP, Sales JR, León M, Melo NJA, Michereff SJ, Ambrosio MMQ, Medeiros HLS, Armengol J. 2019. Identification and pathogenicity of Macrophomina species collected from weeds in melon fields in Northeastern Brazil. J Phytopathol. 167(6):326‒337. doi:10.1111/jph.12801.
- Padilla-Valenzuela I, Valenzuela-Valenzuela RI, Armenta-Castro CM, Salinas-Pérez RA, Sánchez-Sánchez E. 2008. Comportamiento agronómico de genotipos de garbanzo en siembra tardía en el Valle del Mayo, Sonora, México. Rev Fitotec Mex. 31(1):43‒49.
- Pandy AK, Burlakoti RR, Rathore A, Nair R. 2020. Morphological and molecular characterization of Macrophomina phaseolina isolated from three legume crops and evaluation of mungbean genotypes for resistance to dry root rot. Crop Prot. 127:1‒8. doi:10.1016/j.cropro.2019.104962.
- Purkayastha S, Kaur B, Dilbaghi N, Chaudhury A. 2006. Characterization of Macrophomina phaseolina, the charcoal rot pathogen of cluster bean, using conventional techniques and PCR-based molecular markers. Plant Pathol. 55:106‒116. doi:10.1111/j.1365-3059.2005.01317.x.
- Reyes-Franco MC, Hernández-Delgado S, Beas-Fernández R, Medina-Fernández M, Simpson J, Mayék-Pérez N. 2006. Pathogenic and genetic variability within Macrophomina phaseolina from Mexico and other countries. J Phytopathol. 154:447‒453. doi:10.1111/j.1439-0434.2006.01127.x.
- Robles-Yerena L, Leyva Mir SG, Cruz-Gómez A, Camacho-Tapia M, Nieto-Ángel D, Tovar Pedraza JM. 2016. Fusarium oxysporum Schltdl. and Fusarium solani (Mart.) Sacc. cause wilting of Pinus spp. seedlings in the nursery. Rev Mex Cien For. 36(7):25–36.
- Salvatore MM, Félix C, Lima F, Ferreira V, Naviglio D, Salvatore F, Duarte AS, Alves A, Andolfi A, Esteves AC. 2020. Secondary metabolites produced by Macrophomina phaseolina isolated from Eucalyptus globulus. Agriculture. 10:72. doi:10.3390/agriculture10030072.
- Santos KM, Lima GS, Barros APO, Machado AR, Souza MCM, Correia KC, Michereff SJ. 2020. Novel specific primers for rapid identification of Macrophomina species. Eur J Plant Pathol. 156:1213‒1218. doi:10.1007/s10658-020-01952-8.
- Sarr M, Ndiaye M, Groenewald J, Crous P. 2014. Genetic diversity in Macrophomina phaseolina, the causal agent of charcoal rot. Phytopathol Mediterr. 53(2):250–268. doi:10.14601/Phytopathol_Mediterr-13736.
- Shinde P, Hunje R. 2019. Effect of seed priming on field emergence, plant growth, seed yield and disease incidence in Kabuli chickpea (Cicer arietinum L.) varieties. Int J Chem Stud. 7(1):1512‒1517.
- SIAP (Servicio de Información Agroalimentaria y Pesquera). 2020. Panorama Agroalimentario 2020. [accessed 2021 Oct 15]. https://www.gob.mx/siap
- Vandemark G, Martínez O, Pecina V, Alvarado MJ. 2000. Assessment of genetic relationships among isolates of Macrophomina phaseolina using a simplified AFLP technique and two different methods of analysis. Mycologia. 92(4):656–664. doi:10.1080/00275514.2000.12061206.
- Wagan KH, Khaskheli MI, Hajano JD, Lanjar AG. 2019. Population density and aggressiveness of Macrophomina phaseolina isolates from Sindh, Pakistan. Sarhad J Agric. 35(2):400–407. doi:10.17582/journal.sja/2019/35.2.400.407.
- Živanov D, Živanov TS, Nagl N, Savić A, Katanski S, Milić D. 2019. First report of Macrophomina phaseolina causing dry root rot of chickpea (Cicer arietinum L.) in Serbia. Plant Dis. 103(10):2685. doi:10.1094/PDIS-03-19-0652-PDN.