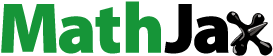
Abstract
This study aimed to examine the relationship between Macrotyloma axillare ‘Java’ and Meloidogyne javanica at different levels of nematode inoculum, and to assess the activity of plant defence enzymes in the presence of nematodes. In Experiment 1, ‘Java’ and soybean (control) were inoculated with 300 to 8000 eggs + second-stage juveniles (J2) of M. javanica in two different periods (Trials 1 and 2). To confirm the results, a third trial was conducted using 1000 to 4000 eggs + J2 per plant. In Experiment 2, β-1,3-glucanase (GLU), phenylalanine ammonia-lyase (PAL), peroxidase (POX), and polyphenol oxidase (PPO) activities were measured in non-inoculated plants and in plants inoculated with 2000 eggs + J2. ‘Java’ presented a reproduction factor (RF) < 1 at all inoculum levels in Trial 1 (RF of 0.17–0.78) and at 2000, 3000, and 6000 eggs + J2 in Trial 2 (RF of 0.47, 0.86, and 0.59, respectively). In Trial 3, ‘Java’ showed a RF < 1 at all inoculum levels except for 3000 eggs + J2 (RF = 1.1). There was no difference in ‘Java’ vegetative variables in relation to inoculum levels in any experiment. In Experiment 2, inoculated plants showed increased GLU, PAL, POX, and PPO activities, when compared with non-inoculated plants. ‘Java’ appears to be moderately resistant to M. javanica, reducing nematode reproduction at different inoculum levels. The plant exhibits increased GLU, PAL, POX, and PPO activities in the presence of M. javanica.
Résumé
Cette étude consistait à examiner la relation entre Macrotyloma axillare ‘Java’ et Meloidogyne javanica à différents taux d’inoculum du nématode, puis à évaluer l’activité des enzymes de défense de la plante en présence des nématodes. Dans l’Expérience 1, le cultivar ‘Java’ et du soya (témoin) ont été inoculés avec 300 à 8 000 œufs + des juvéniles de deuxième stade (J2) de M. javanica, et ce, en deux phases distinctes (Essais 1 et 2). Afin de confirmer les résultats, un troisième essai a été mené avec 1 000 à 4 000 œufs + J2 par plant. Au cours de l’Expérience 2, les activités de la β-1,3-glucanase (GLU), de la phénylalanine ammonia-lyase (PAL), de la peroxydase (POX) et de la polyphénol oxydase (PPO) ont été mesurées chez des plants non inoculés et chez des plants inoculés avec 2 000 œufs + J2. Le cultivar ‘Java’ a affiché un facteur de reproduction (FR) < 1 pour tous les taux d’inoculation de l’Essai 1 (FR de 0,17-0,78) ainsi que pour 2 000, 3 000 et 6 000 œufs + J2 au cours de l’Essai 2 (FR de 0,47, 0,86 et 0,59, respectivement). Au cours de l’Essai 3, ‘Java’ a affiché un FR < 1 pour tous les taux d’inoculum, sauf pour 3 000 œufs + J2 (FR 1,1). Il n’y avait aucune différence dans les variables végétatives de ‘Java’ quant aux taux d’inoculum, peu importe l’expérience. Dans l’Expérience 2, les plants inoculés ont affiché des activités accrues de la GLU, de la PAL, de la POX et de la PPO lorsqu’ils étaient comparés aux plants non inoculés. ‘Java’ semble être moyennement résistant à M. javanica, limitant la reproduction des nématodes à différents taux d’inoculum. Le cultivar affiche des activités accrues de la GLU, de la PAL, de la POX et de la PPO en présence de M. javanica.
Introduction
Plant-parasitic nematodes are one of the major limiting factors affecting crop production worldwide (Stirling Citation2014). Root-knot nematodes (Meloidogyne spp.) are particularly worrisome because of their wide geographical distribution and broad host range, including almost all vascular plant species (Jones et al. Citation2013). Nematodes of the genus Meloidogyne are obligate biotrophs that induce physiological and biochemical changes in host roots, in addition to cellular hypertrophy and hyperplasia, resulting in the formation of feeding structures, known as giant cells (Rodiuc et al. Citation2014). Disturbance of these feeding sites can compromise the development and reproduction of root-knot nematodes and may eventually lead to death (Portillo et al. Citation2013).
Studies have shown that resistant plants may be able to prevent the formation of feeding sites, thereby halting nematode development and reproduction (Silva et al. Citation2013; Marini et al. Citation2016; Miamoto et al. Citation2020). Resistant plants recognize pathogen-associated and/or damage-associated molecular patterns, which trigger the release of pathogenesis-related (PR) proteins, such as β-1,3-glucanase (GLU) and peroxidase (POX), and plant defence enzymes, such as phenylalanine ammonium-lyase (PAL) and polyphenol oxidase (PPO). These are responsible for activating plant defence mechanisms that hinder the success of parasitism: cell wall breakdown and alterations in the nematode cuticle (Mosolov and Valueva Citation2008), production of reactive oxygen species (Kim et al. Citation2019), hypersensitivity reactions (Thakker et al. Citation2013), lignin synthesis (Barros et al. Citation2016), and production of phenolic compounds and phytoalexins (Seenivasan Citation2011).
The legume Macrotyloma axillare (E. Mey.) Verdc. ‘Java’, commonly known as Java in Brazil, has been studied for its nematode resistance in crop rotation systems. It was shown to be resistant to Meloidogyne javanica (Treub) Chitwood (Miamoto et al. Citation2016), Meloidogyne incognita (Kofoid & White) Chitwood, and Rotylenchulus reniformis Linford & Oliveira and moderately resistant to Pratylenchus brachyurus (Godfrey) Filipjev & Schuurmans Stekhoven (Miamoto et al. Citation2018). The mode of action of Java against nematodes has not yet been fully elucidated, but it is known that the plant impairs nematode development after penetration and causes deformation of M. javanica females (Miamoto et al. Citation2020). A hypothesis is that Java might express increased levels of natural defence substances in response to pathogens.
However, the reaction of plants can vary depending on nematode species and the level of inoculum. For instance, the accession ‘Y50’ of Psidium spp. was found to be resistant to M. enterolobii Yang & Eisenback when inoculated with 1600 eggs + second-stage juveniles (J2), but susceptible when treated with an initial inoculum of 600 or 2000 eggs + J2 (Oliveira et al. Citation2019). Such a variation in plant responses is related to differences in the establishment of J2 in host roots as a function of nematode population density in soil (Burla et al. Citation2010). Therefore, it is important to examine the response of plants at different levels of inoculum to avoid false results.
The purpose of this study was to investigate the response of M. axillare ‘Java’ to different inoculum levels of M. javanica and assess the activity of PR-proteins and plant defence enzymes in the presence of the nematode.
Materials and methods
General experimental procedures
All experiments were conducted in a greenhouse. The nematode inoculum used in all experiments was obtained from a pure population of M. javanica multiplied on tomato (Solanum lycopersicum L.) ‘Santa Clara’ under greenhouse conditions. Nematodes were extracted from plant roots by the method developed by Hussey and Barker (Citation1973) and adapted by Boneti and Ferraz (Citation1981). After extraction, eggs and possible J2 were counted by using a Peter’s counting chamber under a microscope at 40× magnification.
Experiment 1: Effect of M. javanica inoculum level on M. axillare ‘Java’ and soybean
The first experiment was designed to investigate the response of ‘Java’ to increasing inoculum levels of M. javanica. For this, three trials were conducted at different times. Trial 1 lasted from March to May 2018, when the mean minimum, average, and maximum temperatures were 18.3, 23.2, and 28.4°C, respectively. Trial 2 (a repetition of Trial 1) was conducted between October and December 2018 (minimum, average, and maximum temperatures of 19.8, 25.2, and 30.4°C, respectively). Trial 3 lasted from March to May 2019, when the minimum, average, and maximum temperatures were 20.0, 24.4 and 29.1°C, respectively.
Trials 1 and 2 followed a completely randomized design with 12 treatments (inoculum level = 300, 500, 700, 900, 1000, 2000, 3000, 4000, 5000, 6000, 7000, and 8000 eggs + J2 of M. javanica), and seven replications. Given that the reproduction factor of M. javanica on ‘Java’ was greater than one at some inoculum levels in Trial 2, we decided to perform a third trial to confirm the results. Trial 3 was a completely randomized design with four inoculum levels = 1000, 2000, 3000, and 4000 eggs + J2 of M. javanica, with seven replications. Intermediate population levels were chosen in order to avoid competition for feeding sites among nematodes, which could generate false-negative results (Burla et al. Citation2010; Miranda et al. Citation2010).
Seedlings of ‘Java’ and soybean (Glycine max L. Merrill) ‘M6210 IPRO’ (used as a control) were grown in polystyrene trays containing commercial substrate (sphagnum peat, coconut fibre, rice husk, pine bark, vermiculite). At 15 days after emergence, seedlings were transplanted into 500 mL expanded polystyrene pots containing soil and sand at a 1:2 (v/v) ratio. The substrate was previously sterilized by autoclaving at 120°C for 2 h (Fabry et al. Citation2007). Each plant was inoculated with 2 mL of nematode suspension, pipetted into two 2 cm deep holes equidistant and 1 cm away from the plant.
At 60 days after nematode inoculation, plants were collected and their shoots separated from the root. The roots were washed and weighed, then eggs + J2 were extracted by the above-mentioned method (Hussey and Barker Citation1973; Boneti and Ferraz Citation1981). The total number of eggs + J2 was counted and divided by the root fresh weight to obtain the number of eggs + J2 per gram of root (population density). The reproduction factor (RF) was calculated as the ratio of the final population to the initial population (Oostenbrink Citation1966). The shoot fresh and dry weights were determined on a semi-analytical balance (four decimal places), and for dry weight determination, shoots were placed in paper bags and dried in a forced-air oven at 60°C, for 72 h (or until a constant weight was achieved).
Experiment 2: Activity of PR-proteins and plant defence enzymes in M. axillare ‘Java’ parasitized by M. javanica
Experiment 2 was conducted to examine the activity of PR-proteins and plant defence enzymes in ‘Java’ inoculated or not with M. javanica. The experiment was conducted (Trial 1) and repeated (Trial 2) in May 2019. Each trial was carried out according to a completely randomized design, with two treatments (inoculated and non-inoculated M. axillare), four replications, and two analyses per sample.
Four ‘Java’ seeds were sown in 180 mL expanded polystyrene pots containing a 1:2 (v/v) mixture of soil and sand autoclaved at 120°C for 2 h. After germination, plants were carefully thinned to one per pot. At 15 days after germination, plants in the nematode treatment group were inoculated with 2 mL of a suspension containing 2000 eggs + J2 of M. javanica. Four plants per treatment were collected at 5, 8, and 12 days after inoculation (DAI), separating a mass of 0.1 g of roots for analysis. The shoots were discarded, and the roots were collected and stored individually in aluminium foil at −80°C until analysis.
Samples were ground in liquid nitrogen by using a mortar and mixed with 4 mL of an extraction solution consisting of 1% polyvinylpyrrolidone, 0.1 mM EDTA, and 50 mM potassium phosphate buffer (pH 7.0). After this step, samples were centrifuged at14500rpm and 4°C for 30 min. The supernatant was transferred to 1.5 mL microtubes, stored at −80°C, and used as enzyme extract for the determination of total proteins and enzyme activity.
Total proteins were quantified by the Bradford method (Bradford Citation1976) using a standard curve of bovine serum albumin. Results are expressed in mg mL−1. GLU (EC 3.2.1.6) activity was determined by colourimetric quantification of reducing sugars released from laminarin (Vogelsang and Barz Citation1993). Reducing sugar concentrations were determined against a standard curve of glucose. GLU activity is expressed in mg glucose h−1 mg−1 protein. PAL (EC 4.3.1.5) activity as described by Umesha (Citation2006). Results were calculated from the difference in absorbance between a mixture containing the sample and a blank, prepared without l-phenylalanine. Values were plotted against a standard curve of trans-cinnamic acid. PAL activity is expressed in mg trans-cinnamic acid h−1 mg−1 protein. POX (EC 1.11.1.7) activity was estimated by the guaiacol method, which measures the conversion of guaiacol to tetraguaiacol in the presence of hydrogen peroxide. Results are presented as change in absorbance (Δabs) min−1 mg−1 protein (Lusso and Pascholati Citation1999). Finally, PPO (EC 1.10.3.2) activity was determined by the catechol method and the results are expressed as Δabs min−1 mg−1 protein (Duangmal and Apentem Citation1999).
Statistical analysis
All data were transformed to to meet Shapiro–Wilk normality assumptions. In Experiment 1, differences related to inoculum levels were investigated by regression analysis. In Experiment 2, mean enzyme activities were compared between inoculated and non-inoculated plants by the Bonferroni t-test (P > 0.05). All statistical analyses were performed using Sisvar software (Ferreira Citation2014).
Results
Experiment 1: Effect of M. javanica inoculum level on M. axillare ‘Java’ and soybean
In Trial 1, the relationship between inoculum level and total eggs + J2 at the end of the experiment was best described by a quadratic model () (p < 0.05, R2 = 0.80). The regression model predicted maximum reproduction (2097 eggs + J2) at an inoculum level of 6584 per plant. The best prediction of the number of eggs + J2 per g root was also by a quadratic model () (p < 0.05, R2 = 0.71), with maximum population density (5431 eggs + J2 per gram of root) at an inoculum level of 5063 (). The reproductive factor (RF) was < 1 at all inoculum levels and declined monotonically with inoculum rate ()
Fig. 1 The regression line of (a) total number of eggs + second-stage juveniles (J2) of Meloigodyne javanica, (b) number of eggs + J2 of M. javanica per gram of root and (c) reproduction factor of M. Javanica in Macrotyloma axillare ‘Java’ under different inoculum levels in Experiment 1 – Trial 1. Raw data are presented in the figure and the regression and statistical equations are based on data transformed by .
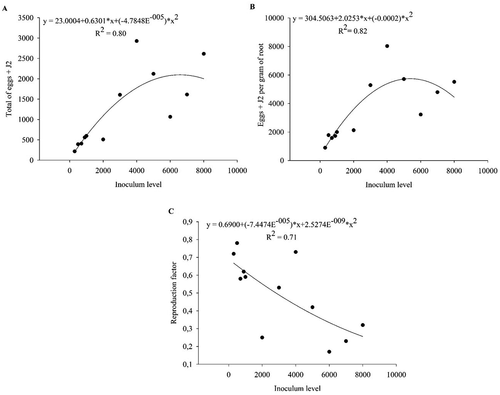
In Trial 2, the number of eggs + J2 total was described by a quadratic model, while eggs + J2 g−1 root increased linearly with inoculum level (). The RF generally declined with inoculum rate but was only less than 1 at inoculum levels of 2000, 3000 and 6000 J2 and eggs per pot ().
Fig. 2 The regression line of (a) total number of eggs + second-stage juveniles (J2) of Meloigodyne javanica, (b) number of eggs + J2 of M. javanica per gram of root and (c) reproduction factor of M. javanica in Macrotyloma axillare ‘Java’ under different inoculum levels in Experiment 1 – Trial 2. Raw data are presented in the figure and the regression and statistical equations are based on data transformed by .
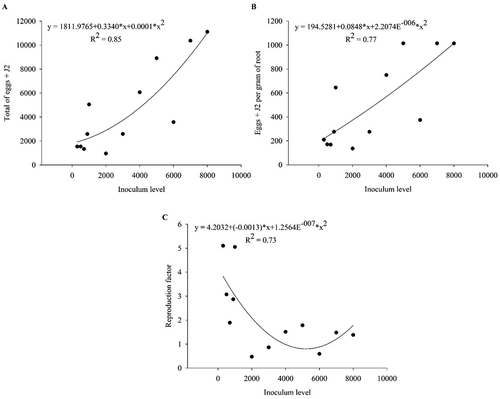
In Trial 3, quadratic regression (p < 0.05, R2 = 0.81) predicted maximum reproduction at an inoculum level of 2737 nematodes per pot, with 2812 eggs + J2 total (). The number of eggs + J2 per gram of root increased linearly with inoculum levels (). ‘Java’ presented RF < 1 for inoculum densities of 1000, 2000 and 4000 J2 and eggs per pot, with the exception of level 3000, where RF = 1.1 ().
Fig. 3 The regression line of (a) total number of eggs + second-stage juveniles (J2) of Meloigodyne javanica, (b) number of eggs + J2 of M. javanica per gram of root and (c) reproduction factor of M. javanica in Macrotyloma axillare ‘Java’ under different inoculum levels in Experiment 1 – Trial 3. Raw data are presented in the figure and the regression and statistical equations are based on data transformed by .
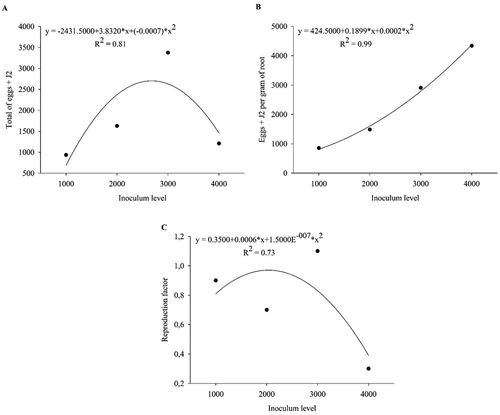
Soybean was evaluated to verify inoculum viability, and in all Trials a RF > 1 was observed for all inoculum levels studied. For Trial 1, the RF in soybean ranged from 1.9 for inoculum level 5000 and 15.2 for inoculum level 300. In Trial 2, the variation was 1.4 for inoculum level 1000 and 10.9 for inoculum level 4000. Finally, in Trial 3, there was a RF variation of 5.4 for the 4000 inoculum level to 8.8 for the 2000 inoculum level (Table 1S – Supplemental material).
In all trials, were no statistically significant relationships between inoculum level and plant growth parameters (Table 2S – Supplemental material).
Experiment 2: Activity of PR-proteins and plant defence enzymes in M. axillare ‘Java’ parasitized by M. javanica
At 5 DAI, there was an increase in PPO activity in Trial 1 (). At 8 DAI, GLU and POX activity was higher in Java plants inoculated with M. javanica in Trial 2 (). By 12 DAI, there was an increase in the activity of all PR-proteins and plant defence enzymes in Java plants inoculated with M. javanica compared with non-inoculated plants, except for POX activity in Trial 1 and PAL activity in Trial 2 (). Although there was no statistical difference between plants inoculated or non-inoculated for POX in Trial 1 and PAL in Trial 2, the activity was 31% and 88% higher in plants parasitized by M. javanica, respectively, at 12 DAI.
Fig. 4 Specific activity of β-1,3-glucanase (a), phenylalanine ammonia-lyase (b), peroxidase (c) and polyphenol oxidase (d) in Macrotyloma axillare ‘Java’ inoculated or not with Meloidogyne javanica – Trial 1. Bars with the same lowercase letter do not differ at the same time of evaluation by the Bonferroni T test (p > 0.05). DAI = days after inoculation. I = inoculated. NI = non-inoculated.
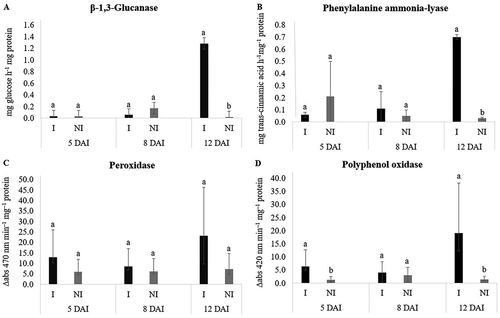
Fig. 5 Specific activity of β-1,3-glucanase (a), phenylalanine ammonia-lyase (b), peroxidase (c) and polyphenol oxidase (d) in Macrotyloma axillare ‘Java’ inoculated or not with Meloidogyne javanica – Trial 2. Bars with the same lowercase letter do not differ at the same time of evaluation by the Bonferroni T test (p > 0.05). DAI = days after inoculation. I = inoculated. NI = non-inoculated.
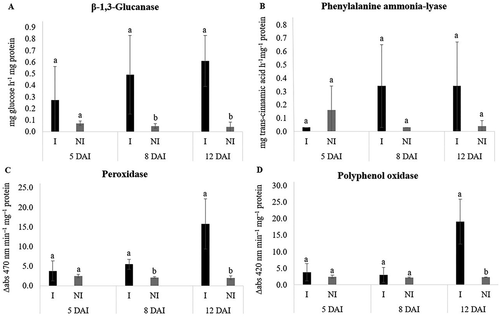
Discussion
Macrotyloma axillare ‘Java’ had a RF < 1 to all M. javanica inoculum levels in Trial 1, to inoculum levels of 2000, 3000, and 6000 eggs + J2 in Trial 2, and to inoculum levels of 1000, 2000, and 4000 eggs + J2 in Trial 3 (Experiment 1). Previous studies showed that ‘Java’ had a RF < 1 to M. javanica when inoculated with 700, 1000, or 2000 eggs + J2 (Miamoto et al. Citation2016, Citation2020), as well as to M. incognita and R. reniformis when inoculated with 700 or 1000 nematodes (Miamoto et al. Citation2018).
In Trial 2 of Experiment 1, Java exhibited RF > 1 at most M. javanica inoculum levels (300, 500, 700, 900, 1000, 4000, 5000, 7000, and 8000 eggs + J2). In all trials, plants were irrigated daily, and moisture was present throughout the development period. Thus, we hypothesize that the high moisture and temperature conditions occurring during Trial 2 favoured nematode development and parasitism. Even in resistant plants, high temperature and humidity conditions can contribute to nematode multiplication (Campos et al. Citation2011; Costa et al. Citation2014). Furthermore, genes that confer resistance to phytopathogens might have reduced expression under high temperature conditions (Van Der Plank Citation1975). An example of this phenomenon is the resistance gene Mi, isolated from different varieties of Solanum peruvianum L. The gene’s expression is inhibited when plants are subjected to temperatures above 28°C for 10–12 h per day; Mi stability depends on heat intensity and exposure duration (Cortada et al. Citation2008; Devran et al. Citation2010; Verdejo-Lucas et al. Citation2013).
The variation in the response of Java to M. javanica observed in this study is similar to that reported for other plant species, including Crotalaria juncea L. and C. ochroleuca G. Don. These species were found to be resistant to Meloidogyne spp. in one study (Miamoto et al. Citation2016) and susceptible in other studies (Charchar et al. Citation2007; Rosa et al. Citation2013).
The mechanism of resistance of ‘Java’ to M. javanica has not been well understood, although some similarities with Crotalaria species can be observed. Crotalaria is another legume that is resistant to many species of Meloidogyne and is useful as a rotation cover crop (Curto et al. Citation2015). As observed in Crotalaria spp., ‘Java’ attracts and allows J2 penetration (Miamoto et al. Citation2016), after which the plant hinders nematode development, impeding the formation of adult M. javanica females (Miamoto et al. Citation2020) and reducing M. incognita female numbers (Miamoto et al. Citation2018).
Inhibition of adult female formation by resistant plants may occur due to damage to the nematode feeding site; in such a case, giant cells do not form properly; the site is established and then cells become disorganized (Motta et al. Citation2012; Marini et al. Citation2016; Miamoto et al. Citation2020). These processes were observed in Crotalaria sp. (Silva et al. Citation1990) and classified as hypersensitivity reactions (Watanabe and Lam Citation2004). It is known that when phytopathogens overcome physical barriers and manage to infect the host, plant receptors initiate the activation of signalling pathways that modulate the expression of defence response genes (Andersen et al. Citation2018).
Resistant plants can act on nematodes in different ways, such as by expressing PR-proteins and defence enzymes involved in the synthesis of phytoalexins, phenols, and lignins. These compounds can affect nematode feeding and movement in plant tissues (Seenivasan Citation2011; Vaganan et al. Citation2014; Sankar et al. Citation2017). The resistance of ‘Java’ may be attributed to these compounds, as the activities of the evaluated PR-proteins and defence enzymes were higher in roots of plants inoculated with M. javanica than in non-inoculated roots.
β-1,3-glucanase (GLU) can be activated by elicitors or pathogen exposure (Yinxiu et al. Citation2012), as occurred in this experiment. GLU acts on glycoprotein and carbohydrate complexes present on the outer surface of the cuticle of plant-parasitic nematodes, serving as a substrate for glucanase and glycosidase activities and promoting the release of exogenous elicitors as a natural resistance mechanism (Sundararaj and Kathiresan Citation2012). Moreover, GLU expression induces phytoalexin accumulation (Edreva and Kostoff Citation2004). Phytoalexins are secondary metabolites produced by plants in response to biotic and abiotic stresses and show antimicrobial action against fungi, bacteria, and nematodes (Ahuja et al. Citation2012). Phytoalexins are synthesized by the shikimic acid and polyacetate pathways, accumulating at the site of infection or adjacent cells, which act on membrane integrity, affecting cellular processes (Vaganan et al. Citation2014).
Phenylalanine ammonium-lyase (PAL), found to have higher activity in ‘Java’ plants infected with M. javanica, is responsible for catalysing the first reaction in the phenylpropanoid route, converting l-phenylalanine to cinnamic acid and affording phytoalexins and lignin precursors (monolignols), which confer great resistance to the plant cell wall (Nakazana et al. Citation2001; Veronico et al. Citation2018). As a result, nematode parasitism becomes less efficient. Lignification strengthens physical barriers, causing the stiffening of cell walls and, thereby, hindering or preventing nematode penetration and movement within the cortex (Devi et al. Citation2009). Thus, nematodes are limited to a small area (Wang et al. Citation2018), reducing the possibility of reinfection (Holbein et al. Citation2019). Lignification chemically modifies the enzymes secreted by pathogens that degrade plant cell walls (Ride Citation1975). Like other members of the legume family, ‘Java’ mostly produces isoflavonoid phytoalexins (Singh and Chandrawat Citation2017). Such compounds can affect the nematode cycle, inhibiting hatching and mobility, repelling nematodes from plant roots, altering fertility, and signalling other plant defence pathways (Chin et al. Citation2018).
Peroxidase (POX), in turn, can act directly in the defence of plants against pathogens and indirectly in signalling pathways related to other physiological processes, such as ethylene biosynthesis, indoleacetic acid decarboxylation, lignification, and cell wall suberization (Passardi et al. Citation2004, Citation2005; Pinto et al. Citation2011). Accumulation of lignin and suberin in cell membranes makes cells more resistant to compressive forces or degradation by hydrolytic enzymes secreted by pathogens such as nematodes (Wuyts et al. Citation2006). Furthermore, POX makes the cellular content toxic and unfavourable to pathogens by stimulating the production of reactive oxygen species (Schaffer and Bronnikova Citation2012).
Finally, PPO is associated with the catalysis of o-hydroxylation of monophenols to o-diphenols and the oxidation of o-diphenols to o-quinones, compounds that are highly toxic to phytopathogens (Das et al. Citation2010). These compounds lead to tissue darkening and lesion formation, reducing secondary infections and pathogen spread (Vaughn and Duke Citation1984). Previous studies reported an increase in PPO concentration in nematode-resistant plants, such as resistant carrot inoculated with Meloidogyne hapla Chitwood (Sivakumar and Vadivelu Citation1995) and resistant potato inoculated with Globodera pallida (Stone) Behrens (Amalraj Citation1995).
Overall, our findings suggest that ‘Java’ may be used in crop rotation for the control of M. javanica as part of an integrated management system. However, our data indicate that M. javanica can reproduce to a limited extent on ‘Java’, and additional field and greenhouse experiments are needed to determine the conditions under which such multiplication occurs. Even so, our results provide important information, as the use of ‘Java’ is another option for insertion within the integrated management of nematodes as there are still difficulties in finding plants that can be used in this system, due to the wide host range of M javanica.
In conclusion, Macrotyloma axillare ‘Java’ appears to be moderately resistant to M. Javanica, reducing nematode reproduction at different inoculum levels. The plant exhibits increased GLU, PAL, POX, and PPO activities in the presence of M. javanica.
Supplemental_File_2.docx
Download MS Word (13.9 KB)Supplemental_File_1.docx
Download MS Word (14.1 KB)Acknowledgements
Thanks to the State University of Maringá for the infrastructure for conducting the research, and thanks to ‘Matsuda Sementes’ for donating Macrotyloma axillare cv. Java seeds. Thanks to CAPES for the doctorate's degree scholarship for A. Miamoto (grant number 88882.344530/2019-01), L. K. Rinaldi (grant number 88882.344503/2019-01) andT. M. Mioranza (grant number 88881.189366/2018-1), for the master's degree scholarship for A. P. Mattos (grant number 88882.344559/2019-01), A. Calandrelli (grant number 88882.344568/2019-01), to CNPq for the master's degree scholarship for M. T. R. Silva (grant number 132466/2019-8), and to CNPq for the research productivity scholarship for C. R. Dias-Arieira (grant number 303269/2020-0).
Disclosure statement
No potential conflict of interest was reported by the author(s).
Supplementary material
Supplemental data for this article can be accessed online here: https://doi.org/10.1080/07060661.2022.2088621
References
- Ahuja I, Kissen R, Bones AM. 2012. Phytoalexin in defense against pathogens. Trends Plant Sci. 17(2):73–90. doi:10.1016/j.tplants.2011.11.002.
- Amalraj SFA. 1995. Enzyme activity associated with resistance in potato to the early stage of Globodera pallida infection. Nematol Mediterr. 23(2):199–202.
- Andersen EJ, Shaukat A, Byamukama E, Yen Y, Nepal MP. 2018. Disease resistance mechanisms in plants. Genes. 9(7):1–30. doi:10.3390/genes9070339.
- Barros J, Serrani-Yarce JC, Chen F, Bexter D, Venables BJ, Dixon RA. 2016. Role of bifunctional ammonia-lyase in grass cell wall biosynthesis. Nat Plants. 2(16050):1–9. doi:10.1038/nplants.2016.50.
- Boneti JIS, Ferraz S. 1981. Modificação do método de Hussey e Barker para extração de ovos de Meloidogyne exigua de raízes de cafeeiro. Fitopatol Bras. 6(1):553.
- Bradford MM. 1976. A rapid and sensitive method for the quantification of microgram quantities of protein utilizing the principle of protein-dye binding. Anal Biochem. 72(1):248–254. doi:10.1016/0003-2697(76)90527-3.
- Burla RS, Souza RM, Gomes VM, Corrêa FM. 2010. Comparação entre níveis de inóculo, época de avaliação e variáveis para seleção de Psidium spp. visando à resistência a Meloidogyne mayaguensis. Nematol Bras. 34(2):82–90.
- Campos HD, Silva JRC, Campos VP, Silva LHCP, Costa LSAS, Silva WJR. 2011. Effect of soil temperature on infectivity and reproduction of Meloidogyne javanica and Heterodera glycines in soybean cultivars. Cienc Agrotec. 35(5):900–907. doi:10.1590/S1413-70542011000500006.
- Charchar JM, Gonzaga V, Vieira JV, Oliveira VR, Moita AW, Aragão FAS. 2007. Efeito de rotação de culturas no controle de Meloidogyne spp. em cenoura na região norte do Estado de Minas Gerais. Nematol Bras. 31(1):173–179.
- Chin S, Behm CA, Mathesius U. 2018. Functions of flavonoids in plant–nematode interactions. Plants. 7(4):1–17. doi:10.3390/plants7040085.
- Cortada L, Sorribas FJ, Ornat C, Kaloshian I, Verdejo-Lucas S. 2008. Variability in infection and reproduction of Meloidogyne javanica on tomato rootstocks with the Mi resistance gene. Plant Pathol. 57(6):1125–1135.
- Costa MJN, Pasqualli RM, Prevedello R. 2014. Effect of soil organic matter content, cover crop and planting system on the control of Pratylenchus brachyurus in soybean. Summa Phytopathol. 40(1):63–70. doi:10.1590/S0100-54052014000100009.
- Curto G, Dallavalle E, Santi R, Casadei N, D’Avino L, Lazzeri L. 2015. The potential of Crotalaria juncea L. as a summer green manure crop in comparison to Brassicaceae catch crops for management of Meloidogyne incognita in the Mediterranean area. Eur J Plant Pathol. 142(4):829–841. doi:10.1007/s10658-015-0655-2.
- Das SC, Balamohan TN, Poornima K, Seenivasan N, Bergh VD, De Waele D. 2010. Reaction of Musa hybrids to the burrowing nematode, Radopholus similis. Indian J Nematol. 40(2):189–197.
- Der Plank JE V. 1975. Principles of plant infection. New York: Academic Press; p. 216.
- Devi AN, Ponnuswami V, Sundararaju P, Van den Bergh I, Kavino M. 2009. Histopathological changes in banana roots caused by Pratylenchus coffeae, Meloidogyne incognita and Radopholus similis, and identification of RAPD markers associated with P. coffeae resistance. Acta Hort. 828(1):283–290. doi:10.17660/ActaHortic.2009.828.28.
- Devran Z, Söğüt MA, Mutlu N. 2010. Response of tomato rootstocks with the Mi resistance gene to Meloidogyne incognita race 2 at different soil temperatures. Phytopathol Mediterr. 49(1):11–17.
- Duangmal K, Apenten RKO. 1999. A comparative study of poliphenoloxidases from taro (Colocasia esculenta) e potato (Solanum tuberosum var Romano). Food Chem. 64(3):351–359. doi:10.1016/S0308-8146(98)00127-7.
- Edreva A, Kostoff D. 2004. A novel strategy for plant protection: induced resistance. J Mol Cell Biol. 3(1):61–69.
- Fabry CFS, Freitas LG, Neves WS, Coutinho MM, Tótola MR, Oliveira JR, Dallemole-Giaretta R, Ferraz S. 2007. Obtenção de bactérias para a o biocontrole de Meloidogyne javanica por meio de aquecimento de solo e tratamento com filtrado de raízes de plantas antagonistas a fitonematóides. Fitopatol Bras. 32(1):79–82. doi:10.1590/S1413-70542014000200001.
- Ferreira DF. 2014. Sisvar: a guide for its bootstrap procedures in multiple comparisons. Cienc Agrotec. 38(2):109–112.
- Holbein J, Franke RB, Marhavý P, Fujita S, Górecka M, Sobzak M, Geldner N, Schreiber L, Grundler FMW, Siddique S. 2019. Root endodermal barrier system contributes to defence against plant‐parasitic cyst and root‐knot nematodes. Plant J. 100(2):221–236. doi:10.1111/tpj.14459.
- Hussey RS, Barker KR. 1973. A comparison of methods colleting inocula of Meloidogyne spp. including a new technique. Plant Dis. 57(1):1025–1028.
- Jones JT, Haegeman A, Danchin EGJ, Gaur HS, Helder J, Jones MGK, Kikuchi T, Manzanilla-Lopez R, Palomares-Rius JE, Wesemael WML, et al. 2013. Top 10 plant parasitic nematodes in molecular plant pathology. Mol Plant Pathol. 14(9):946–961. doi:10.1111/mpp.12057.
- Kim YH, Byung-Wook Y, Sang-Soo K. 2019. Expression of the sweet potato peroxidase gene swpa4 in Arabidopsis activates defense genes mediated by reactive oxygen species and nitric oxide. Plant Biotechnol Rep. 13(1):329–336. doi:10.1007/s11816-019-00546-z.
- Lusso MFG, Pascholati SF. 1999. Activity and isoenzymatic pattern of soluble peroxidases in maize tissues after mechanical injury or fungal inoculation. Summa Phytopatol. 25(1):244–249.
- Marini PM, Garbuglio DD, Dorigo OF, Machado ACZ. 2016. Histological characterization of resistance to Meloidogyne incognita in Avena sativa. Trop Plant Pathol. 41(1):1–7. doi:10.1007/s40858-016-0088-2.
- Miamoto A, Dias-Arieira CR, Cardoso MR, Puerari HH. 2016. Penetration and reproduction of Meloidogyne javanica on leguminous crops. J Phytopathol. 164(11–12):890–895. doi:10.1111/jph.12508.
- Miamoto A, Dias-Arieira CR, Puerari HH, Mioranza TM, Pereira CB. 2018. Antagonistic effects of Java against plant parasitic nematodes. J Agr Sci. 10(3):289–300.
- Miamoto A, Machado ACZ, Dorigo OF, Mioranza TM, Puerari HH, Silva BA, Schwan VV, Dias-Arieira CR. 2020. Antagonistic potential and histopathology of Meloidogyne javanica on Macrotyloma axillare cv. Java. Aust J Crop Sci. 14(6):940–946. doi:10.21475/ajcs.20.14.06.p2153.
- Miranda GB, Souza RM, Viana AP, Ribeiro D, Moreira R. 2010. Assessment of methods and criteria for screening Psidium spp. for resistance to Meloidogyne enterolobii. Nematol Bras. 34(4):211–219.
- Mosolov VV, Valeuva TA. 2008. Proteinase inhibitors in plant biotechnology: a review. Appl Biochem Microbiol. 44(3):233–240. doi:10.1134/S0003683808030010.
- Motta FC, Alves GCS, Giband M, Gomes ACMM, Souza FR, Mattos VS, Barbosa VHS, Barroso PAV, Nicole M, Peixoto JR, et al. 2012. New sources of resistance to Meloidogyne incognita race 3 in wild cotton accessions and histological characterization of the defense mechanisms. Plant Pathol. 62(1):1173–1183.
- Nakazana A, Nozue M, Yasuda H. 2001. Expression pattern and gene structure of phenylalanine ammonia-lyase in Phatibis mil. J Plant Res. 1114(1):323–328. doi:10.1007/PL00013994.
- Oliveira PG, Queiróz MA, Castro JMC, Ribeiro JM, Oliveira RS, Silva MJL. 2019. Reaction of Psidium spp. accessions to different levels of inoculation with Meloidogyne enterolobii. Rev Caatinga. 32(2):419–428. doi:10.1590/1983-21252019v32n215rc.
- Oostenbrink R. 1966. Major characteristics of the relation between nematodes and plants. Meded Landbouwhogeschool. 66(4):46.
- Passardi F, Penel C, Dunand C. 2004. Performing the paradoxical: how plant peroxidases modify the cell wall. Trends Plant Sci. 9(11):534–540. doi:10.1016/j.tplants.2004.09.002.
- Passardi F, Cosio C, Penel C, Dunand C. 2005. Peroxidases have more functions than a Swiss army knife. Plant Cell Rep. 24(5):255–265. doi:10.1007/s00299-005-0972-6.
- Pinto MST, Ribeiro JM, Oliveira EAG. 2011. O estudo de genes e proteínas de defesa em plantas. Rev Brasil Biocien. 9(2):241–248.
- Portillo M, Cabrera J, Lindsey K, Topping J, Andres MF, Emiliozzi M, Oliveros JC, Garcia-Cardoso G, Solano R, Koltai H, et al. 2013. Distinct and conserved transcriptomic changes during nematode-induced giant cell development in tomato compared with Arabidopsis: a functional role for gene repression. New Phytol. 197(4):1276–1290. doi:10.1111/nph.12121.
- Ride JP. 1975. Lignification in wounded wheat leaves in response to fungi and its possible role in resistance. Physiol Plant Pathol. 5(2):125–134. doi:10.1016/0048-4059(75)90016-8.
- Rodiuc N, Vieira P, Banora MY, de Almeida Engler J. 2014. On the track of transfer cell formation by specialized plant-parasitic nematodes. Front Plant Sci. 5(1):1–14. doi:10.3389/fpls.2014.00160.
- Rosa JMO, Westerich JN, Wilcken SRS. 2013. Meloidogyne javanica reproduction on vegetable crops and plants used as green manure. Trop Plant Patol. 38(2):133–141. doi:10.1590/S1982-56762013000200007.
- Sankar C, Soorianathasundaram K, Kumar N, Karunakaran G, Sivakumar M. 2017. Induction of resistant to Radopholus similis and defence related mechanism in susceptible and resistance banana hybrids infected with Radopholus similis. Int J Curr Microbiol Appl Sci. 6(4):1668–1684. doi:10.20546/ijcmas.2017.604.202.
- Schaffer WM, Bronnikova TV. 2012. Peroxidase- ROS interactions. Nonlinear Dynam. 68(1):413–430. doi:10.1007/s11071-011-0314-x.
- Seenivasan N. 2011. Efficacy of Pseudomonas flourescens and Paecilomyces lilacinus against Meloidogyne graminicola infesting rice under system of rice intensification. Arch Phytopathol Plant Protec. 44(15):1467–1482. doi:10.1080/03235408.2010.505788.
- Silva GS, Ferraz S, Santos JM. 1990. Histopatologia de raízes de Crotalaria parasitadas por Meloidogyne javanica. Fitopatol Bras. 15(1):46–48.
- Silva RV, Oliveira RDL, Ferreira PS, Ferreira AO, Rodrigues FA. 2013. Defense responses to Meloidogyne exigua in resistant coffee cultivar and non-host plant. Trop Plant Pathol. 38(2):114–121. doi:10.1590/S1982-56762013000200004.
- Singh R, Chandrawat KS. 2017. Role of phytoalexins in plant disease resistance. Int J Curr Microbiol Appl Sci. 6(1):125–129. doi:10.20546/ijcmas.2017.601.016.
- Sivakumar M, Vadivelu S. 1995. Biochemical changes induced by the northern root-knot nematode Meloidogyne hapla Chitwood, 1949 on the roots of carrot (Daucus carota L.) II. phenol oxidase enzymes. Curr Nematol. 6(1):67–69.
- Stirling GR. 2014. Biological control as a component of integrated nematode management: the way forward. In: Stirling GR, editor. Biological control of PPN: soil ecosystem management in sustainable agriculture. Second revised ed. Wallingford (UK): CABI; p. 393–407.
- Sundararaj P, Kathiresan T. 2012. Induction of β-1,3-glucanase and chitinase activities in resistant and susceptible sugarcane clones inoculated with Pratylenchus zeae. Int J Nematol. 22(1):1–10.
- Thakker JN, Patel S, Dhandhukia PC. 2013. Induction of defense-related enzymes in banana plants: effect of live and dead pathogenic strain of Fusarium oxysporum f. sp. cubense. ISRN Biotechnol. 2013(1):1–6.
- Umesha S. 2006. Phenylalanine ammonia lyase activity in tomato seedlings and its relationship to bacterial canker disease resistance. Phytoparasitica. 34(1):68–71. doi:10.1007/BF02981341.
- Vaganan MM, Ravi I, Nandakumar A, Sarumathi S, Sudararaju S, Mustaffa MM. 2014. Phenylpropanoid enzymes, phenolic polymers and metabolites as chemical defenses to infection of Pratylenchus coffeae in roots of resistant and susceptible bananas (Musa spp.). Indian J Exp Biol. 52(3):252–260.
- Vaughn KC, Duke SO. 1984. Function of polyphenol oxidase in higher plants. Physiol Plantarum. 60(1):106–112. doi:10.1111/j.1399-3054.1984.tb04258.x.
- Verdejo-Lucas S, Blanco M, Cortada L, Sorribas FJ. 2013. Resistance of tomato rootstocks to Meloidogyne arenaria and Meloidogyne javanica under intermittent elevated soil temperatures above 28°C. Crop Protec. 46(1):57–62. doi:10.1016/j.cropro.2012.12.013.
- Veronico P, Paciolla C, Pomar F, Leonardis S, García-Ulloa A, Melillo MT. 2018. Changes in lignin biosynthesis and monomer composition in response to benzothiadiazole and root-knot nematode Meloidogyne incognita infection in tomato. J Plant Physiol. 230(1):40–50. doi:10.1016/j.jplph.2018.07.013.
- Vogelsang R, Barz W. 1993. Purification, characterization and differential hormonal regulation of a ?-1,3-glucanase and two chitinases from chickpea (Cicer arietinum L.). Planta. 189(1):60–69. doi:10.1007/BF00201344.
- Wang Q, Cao K, Li H, Zhu G, Fang W, Chen C, Wang X, Wang L. 2018. Lignification plays an important role on resistance to root-knot nematode (Meloidogyne incognita) based on contrastive analysis in peach. Sci Hortic. 238(1):1–62. doi:10.1016/j.scienta.2018.03.036.
- Watanabe N, Lam E. 2004. Recent advance in the study of caspase-like proteases and Bax inhibitor-1 in plants: their possible roles as regulator of programmed cell death. Mol Plant Pathol. 5(1):65–70. doi:10.1111/j.1364-3703.2004.00206.x.
- Wuyts N, De Waele D, Swennen R. 2006. Effects of plant phenylpropanoid pathway products and selected terpenoids and alkaloids on the behavior of the plant-parasitic nematodes Radopholus similis, Pratylenchus penetrans and Meloidogyne incognita. Nematology. 8(1):89–101.
- Yinxiu H, Weimin Z, Shirong G. 2012. Effects of riboflavin and TYLCV inoculation on the activities of chitinase and β-1.3-glucanase in tomato. J Nanjing Univ. 35(4):135–139.