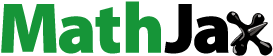
Abstract
This study aimed to evaluate the effect of plant growth-promoting rhizobacteria (PGPR) and compost, applied alone or in combination, on tomato resistance to vascular wilt caused by Verticillium dahliae. Improved management of vascular wilt is important for enhanced productivity of greenhouse-grown susceptible tomato plants. The application of compost and PGPR resulted in a reduction in infection parameters compared with untreated plants inoculated with the pathogen, as well as activation of plant defence mechanisms. In the presence of the pathogen, compost application significantly improved the total fresh and dry weights by 97% and 71%, respectively, compared with pathogen-inoculated controls. The combination of compost with PGPR significantly increased chlorophyll fluorescence and stomatal conductance compared with the infected-control. In addition, the combination of compost and PGPR reduced the accumulation of stress markers such as malondialdehyde and hydrogen peroxide by 48% and 77%, respectively, and promoted the activity of antioxidant enzymes. Fruit yield and quality were also significantly improved relative to the control. The results suggest that compost applied alone or in combination with PGPR helps to suppress vascular wilt and to improve the yield and quality of tomatoes.
Résumé
Cette étude visait à évaluer les effets des rhizobactéries favorisant la croissance des plantes (RFCP) et du compost, appliqué seul ou combiné, sur la résistance de la tomate à la flétrissure vasculaire causée par Verticillium dahliae. Afin d’accroître la productivité des tomates de serre sensibles, il importe d’améliorer la gestion de la flétrissure vasculaire. L’application de compost et de RFCP a entraîné une réduction des paramètres infectieux, comparativement aux plants non traités inoculés avec l’agent pathogène, de même qu’une activation des mécanismes de défense de la plante. En présence de l’agent pathogène, l’application de compost a significativement amélioré les poids totaux frais et secs de 97% et 71%, respectivement, comparativement aux témoins inoculés. La combinaison de compost et de RFCP a accru significativement la fluorescence chlorophyllienne et la conductance stomatique, comparativement aux témoins infectés. En outre, la combinaison de compost et de RFCP a réduit l’accumulation de marqueurs de stress comme le malonaldéhyde et le peroxyde d’hydrogène de 48% et de 77%, respectivement, et a promu l’activité des enzymes antioxydantes. Le rendement et la qualité des fruits ont été aussi notablement améliorés par rapport aux témoins. Les résultats suggèrent que le compost appliqué seul ou avec les RFCP contribue à supprimer la flétrissure vasculaire et à améliorer le rendement et la qualité des tomates.
Introduction
Vascular wilts caused by soilborne pathogens such as Verticillium dahliae have been among the most destructive and widespread diseases of vegetable, ornamental and tree crops (Klosterman et al. Citation2009; Inderbitzin and Subbarao Citation2014; Chen et al. Citation2021). The infection cycle of V. dahliae starts with microsclerotia, which are structures capable of surviving in the soil and without a host for several decades (Klimes et al. Citation2015). These structures generate infectious hyphae that directly penetrate the host plant epidermis and invade the root cortex (Vallad and Subbarao Citation2008; Boutaj et al. Citation2019). Moreover, once the pathogen spreads into the vascular tissues of a host, typical symptoms of V. dahliae infection begin to appear 21 days after infection in the lower leaves, including yellowing, chlorosis and necrotic lesions that develop into browning and leaf death (Jones et al. Citation2016). Verticillium dahliae is capable of infecting more than 200 plant species and causes severe losses to economically important crops including tomato (Lycopersicon esculentum Mill. = Solanum lycopersicum L.) (Fradin and Thomma Citation2006; Ait Rahou et al. Citation2021).
Tomato is one of the most important major vegetable crops worldwide due to its high production, with up to 4.8 million ha grown and an average yield of 37 tons per ha (FAOSTAT Citation2021). It is one of the most consumed vegetables in the world because of its high nutritional value, providing vitamin C, minerals and antioxidants (i.e. lycopene) and other health benefits, such as reduction of blood pressure and stimulation of circulation (Campestrini et al. Citation2019; Salehi et al. Citation2019; Çolak et al. Citation2020; Okatan Citation2020). Different methods and techniques are used for the management of tomato diseases, such as chemical control by fungicides including propineb and copper oxychloride (Sreenivasulu et al. Citation2019). However, the excessive use of chemical inputs has been considered an agricultural practice that decreases soil fertility and causes serious damage to human health and the environment (Singh and Strong Citation2016; Chavan et al. Citation2017). Hence, there is a need to apply biofertilizers instead of chemicals, to provide the necessary nutrients to plants without degrading the environment and to improve crop production and food security.
This research indicates that plants use cell surface pattern recognition receptors (PRRs) such as the Arabidopsis chitin receptor CERK1 (Miya et al. Citation2007) to perceive pathogen-associated molecular patterns (PAMPs) or damage-associated molecular patterns (DAMPs), and rapidly activate PAMP-triggered immunity to protect plants against pathogens (Van Ooijen et al. Citation2007; Kim and Castroverde Citation2020). A recognition cascade is initiated through signalling, beginning with a Ca2+ influx, the production of reactive oxygen species, and expression of defence-related genes. In the case of tomato, the Ve1 locus confers specific resistance and has been shown to protect against V. dahliae (Kawchuk et al. Citation2001; Fradin et al. Citation2009; De Jonge et al. Citation2012; Macho and Zipfel Citation2014; Couto and Zipfel Citation2016).
The application of plant growth promoting rhizobacteria (PGPR), microorganisms that grow at the rhizosphere zone, can provide long-term protection against pathogen attacks and can serve as practical as biocontrol agents (Haldar and Sanghamitra Citation2015). They promote plant growth and development through the secretion of substances such as auxins and cytokinins, and have the ability to fix atmospheric nitrogen, solubilize nutrients, produce phytohormones and are involved in Fe sequestration (Prasad et al. Citation2019; Oleńska et al. Citation2020; Pathania et al. Citation2020). Moreover, via the application of PGPR, plant pathogens can be reduced by several mechanisms of action, such as antagonism, competition for nutrients and induction of systemic resistance; the latter is mediated by the induction of signalling pathways that regulate induced systemic resistance (ISR) against plant pathogens (Attia et al. Citation2020; Mekonnen and Kibret Citation2021). Plant growth promoting rhizobacteria produce volatile compounds that induce defence genes like PR1 and PR2 related to the activation of phytohormone signalling pathways (Pieterse et al. Citation2014; Mhlongo et al. Citation2018).
Similarly, the application of organic amendments represents an efficient way to recycle waste-biomass and reduce soilborne diseases, allowing the beneficial reuse of organic waste from agricultural and agro-industrial activities to produce high-quality food (Mishra et al. Citation2015; Lian et al. Citation2017). The application of compost alone and/or combined with PGPR can help to control soilborne pathogens (Botrytis cinerea, Fusarium oxysporum, V. dahliae, Rhizoctonia solani) through a variety of mechanisms, including the release of fungitoxic compounds, competition for nutrients, direct parasitism or through the development of a suppressive microbiome to improve the uniformity of disease control (Bonilla et al. Citation2012; Raaijmakers and Mazzola Citation2012; Tubeileh and Stephenson Citation2020). This study investigated the application of PGPR and/or compost for their ability to reduce vascular wilt severity in greenhouse tomato susceptible to V. dahliae, in addition to evaluating the capacity of growing media enriched with these biocontrols to promote growth, yield and fruit quality.
Materials and methods
Compost material
The composting process was carried out over 3 months at the Faculty of Science Semlalia, Cadi Ayyad University, Marrakesh, Morocco. Prior to starting the composting process, wet green waste underwent sorting. Only dead leaves and grass were retained for a homogeneous compostable sample. The green waste was mixed carefully by turning to ensure proper homogenization before watering. The windrow was covered with an impermeable plastic sheet (polypropylene) to avoid runoff and leaching when the mixture was moistened and to protect from rain. The windrow was turned twice a week to ensure good aerobic degradation. To evaluate the composting process, uniform sampling was carried out on the whole windrow, on the surface and at different depths, to obtain a homogeneous and representative sample to be used for physicochemical analyses.
The compost obtained from green waste (quack grass) was applied at a rate of 5% (w/w) and its physicochemical characteristics were as follows: pH: 7.80, electrical conductivity: 8.50 mS cm−1, total organic carbon: 20.64%, total nitrogen: 1.32%, C/N: 15.64, available phosphorus: 0.27 mg g−1.
The rhizobacterial strain ER10 was isolated from the rhizosphere of date palm in the Erfoud region (31° 26’ 19.1” N, 04° 14’ 10.1” W). This rhizobial strain was tested for plant growth promoting traits and biochemical characteristics by standard protocols, including phosphate and potassium solubilization as described by Alikhani et al. (Citation2006) as well as production of siderophores (Schwyn and Neilands Citation1987), exopolysaccharides (Lee et al. Citation2007), and indole acetic acid (Raklami et al. Citation2019). The characteristics of ER10 are reported in . The inoculation of tomato (cv. Campbell 33) plants (two-leaf stage) was performed by preparing an ER10 suspension in Tryptic Soy Broth medium that was incubated for 2 days at 28°C to obtain an optical density (OD = 1) at 600 nm (approximately 109 CFU mL−1).
Table 1. PGPR properties of the tested rhizobacteria.
Inoculation of plants was performed on roots by spraying 4 mL of the ER10 bacterial suspension in equal volumes and using a micropipette following transplanting of the plants. A second inoculation was performed as a booster after 15 days by spraying 8 mL of the bacterial suspension on the roots, to increase the level of the bacteria in the soil and ensure the infection of newly formed roots.
In vitro antagonistic activity
The bacterial strain was tested for its ability to inhibit growth of V. dahliae. The tests were completed in sterile Petri dishes containing potato dextrose agar (PDA). Bacterial cultures of ER10 were adjusted to a final concentration of 106 CFU mL−1 (OD = 0.8) and 10 µL of the bacterial culture was placed in the centre of the plate. A V. dahliae suspension of 1.5 × 106 conidia mL−1 (counted using a haemocytometer) was sprayed onto the plates. Bacterial and fungal cultures were also plated separately as controls. Plates were incubated at 30°C for 7 days, and the antifungal activity of the bacteria was assessed by the presence of growth inhibition halos surrounding each bacterial colony. The test was performed in triplicate using independent Petri dishes.
Fungal pathogen
The Verticillium isolate OMV5 was provided by The Laboratory of Botany, Biotechnology and Plant Protection, Faculty of Science, Ibn Tofail University, Kenitra, Morocco. It was found to be very aggressive against tomatoes (Ouazzani et al. Citation2014).
Experimental setup and design
The experiment was conducted for 5 months in a controlled greenhouse (light/dark photoperiod of 16/8 h, average temperature of 27°C and relative humidity 68.5%). The soil used was collected from the nursery of the Urban Municipality of Marrakesh, Morocco, and had the following physicochemical characteristics: pH: 8.2; electrical conductivity: 0.14 mS cm−1; organic matter: 1.02%; total organic carbon: 0.6%; available phosphorus: 8 mg kg−1; total nitrogen: 0.9 mg g−1; calcium: 2.4 g kg−1 and potassium: 0.57 mg g−1.
Tomato seeds (cv. Campbell 33) susceptible to V. dahliae, of homogeneous size, were selected and disinfected with sodium hypochlorite (5% v/v) for 5 min. They were rinsed three times with distilled water and germination was performed in commercial peat at 28°C. Then, the tomato seedlings were transplanted into perforated plastic pots (one per pot) containing 2 kg of agricultural soil that had been previously sterilized for 3 h at 180°C.
Half of the plants (80 tomato plants) were inoculated with a conidial suspension of V. dahliae and adjusted to 1 × 106 conidia mL−1 using a haemocytometer. The roots of the tomato seedlings were lightly wounded 6 cm under the upper part of the stem with a pointed stick. Then, 10 mL of the conidial suspension was inoculated into the wound using a test tube. The other half of the plants was treated under the same conditions with sterile distilled water (10 mL).
The experiment was arranged in a randomized complete block design with eight treatments: 1) Control treatment: control plants without application of compost or PGPR; 2) Compost treatment (C): plants amended with compost alone; 3) PGPR Treatment (B): plants inoculated with PGPR bacteria; 4) Combined compost and PGPR treatment (CB); 5) V. dahliae treatment (V); 6) Compost and V. dahliae treatment (CV); 7) PGPR and V. dahliae treatment (BV); and 8) Combined compost, PGPR and V. dahliae treatment (CBV).
Plant growth and physiological parameters
After 5 months of cultivation, growth parameters (plant height, number of leaves, and total fresh and dry weight of the plant) were measured. Photosystem II efficiency was assessed by measuring chlorophyll fluorescence using a portable fluorometer (Opti-sciences OSI 30p) for dark-acclimated leaves. Leaves were acclimated to darkness for 30 min using forceps before measurements were made. Chlorophyll fluorescence variables were recorded: initial (F0), maximum (Fm), variable (Fv) fluorescence as well as the Fv/Fm ratio. Stomatal conductance (gs) was measured on five replicates per treatment on a sunny day before harvest using a porometer system (Leaf Porometer LP1989, Decagon Device, Inc., Washington, USA) following the instructions in the user manual. The third youngest leaf was selected for measurements.
Evaluation of disease response in tomato plants
One month after inoculation with isolate OMV5, the evolution of disease symptoms was observed for 3 months. Disease severity was recorded visually. A scale from 0 to 5 was used to assess disease progression according (El Said et al. Citation2012, Formula 1). Disease incidence was calculated as the percentage of infected plants out of the total plants examined according to Formula 2 (Boutaj et al. Citation2019) and the leaf alteration index (LAI) was then established for each plant according to the scale of Douira et al. (Citation1995) (Formula 3).
Where, A: healthy plant or no symptoms; B: onset of dieback, no defoliation; C: average dieback, average wilting; D: severe dieback, severe wilting and E: dead plant.
Where, i: leaves appearance notes 0–5; xi: number of leaves with the note i; and Nt F: total number of leaves. Leaf appearance notes were recorded as: 0: healthy looking leaves; 1: cotyledonary leaves: wilting or yellowing; 2: cotyledonary leaves: dropped; 3: true leaves: wilting or yellowing; 4: true leaves: necrotic; and 5: true leaves: fallen.
The area under the disease progress curve (AUDPC) originally defined by Campbell and Madden (Citation1990) was used to summarize the progression of the disease. The standardized AUDPC was calculated according to the following formula 4:
Where, t: time between observation; n: total number of observations; Si: final mean severity (FMS); and 4: maximum disease rating.
Determination of mineral content
Dry tomato leaf samples (0.5 g) were placed in porcelain melting pots and calcined at 555°C in an oven for 6 h. The ash obtained was taken up by 3 mL of HCl (6 N) with dry evaporation in the sandbox and then recovered with hot distilled water. The solutions obtained were filtered and the extracts collected. To determine the concentrations of P, Na+, K+, Ca2+and Mg2+ in the solution, inductively coupled plasma-atomic emission spectrometry (ICP-AES) (Varian AA240, Varian, Inc., Palo Alto, CA) was used.
Malondialdehyde content
Lipid peroxidation was assessed by measuring leaf and root malondialdehyde (MDA) content following Wu et al. (Citation2016). Fresh leaf and root samples (100 mg) were ground in the presence of liquid nitrogen, 10% trichloroacetic acid (TCA) and 1 mL cold acetone. The homogenate was centrifuged at 8000 × g for 15 min at 4°C and the supernatant was added to 0.1% H3PO4 and 0.6% thiobarbituric acid (TBA). The mixture was incubated for 30 min at 100°C and then cooled in an ice bath to stop the reaction. The optical density was recorded at 532 nm and 600 nm using the molar extinction coefficient of 155 mM−1 cm−1.
Hydrogen peroxide content
The concentration of hydrogen peroxide (H2O2) in leaves and roots samples was determined by the method of Velikova et al. (Citation2000). Powdered frozen sample (0.5 g) was homogenized with 5 mL of 10% (w/v) TCA and centrifuged at 15 000 × g for 15 min at 4°C. After recovery of the supernatant, 0.5 mL of potassium phosphate buffer (10 mM, pH7) and 1 mL of potassium iodide (1 M) were added. The absorbance at 390 nm was then recorded after 1 h of incubation in the dark.
Antioxidant enzyme assay
The optimized protocol for the antioxidant enzyme assay was described by Ait-El-Mokhtar et al. Citation2020). Tomato leaf and root samples (100 mg) were frozen in liquid nitrogen and homogenized in 5 mL of phosphate buffer (0.1 M, pH 7), 0.1 g of polyvinylpolypyrrolidone (PVPP) and 0.1 mmol ethylenediaminetetraacetic acid (EDTA). The homogenate was then centrifuged at 15 000 × g for 15 min at 4°C and the supernatants were stored at 20°C for determination of enzyme activity.
The activity of superoxide dismutase (SOD) was determined according to the method of Beyer and Fridovich (Citation1987) by monitoring its ability to inhibit the photochemical reduction of p-nitroblue-tetrazolium (NBT) at 560 nm. The reaction mixture contains 50 μL of enzymatic extract, 1750 μL of phosphate buffer (0.1 M, pH 7), 50 μL of riboflavin, 100 μL of methionine and 50 μL of NBT. SOD activity was expressed in unit per mg protein. The peroxidase (POX) activity was determined and measured for 3 min using the method of Tejera García et al. (Citation2004). The reaction solution consisted of 100 mM K2HPO4/KH2PO4 buffer (pH 6.5), 40 mM guaiacol, 10 mM H2O2 and the enzyme extract (0.1 mL). The absorbance was recorded at 436 nm and results were expressed in µmol Gaïacolmin−1 mg−1 protein using an extinction coefficient of 25.5 mM−1 cm−1. The polyphenol oxidase (PPO) activity was estimated by monitoring catechol oxidation at 410 nm according to Hori et al. (Citation1997). The test mixture contained 100 mM K2HPO4/KH2PO4 buffer (pH 6), catechol 50 mM and enzyme extract. The activity of PPO was expressed in μmol catechol min−1mg−1protein. Catalase activity was determined by monitoring the decrease in absorbance at 240 nm for 3 min following Aebi (Citation1984). The reaction mixture consisted of 50 mM K2HPO4, (pH 7.0), 10 mM H2O2 and 0.1 mL enzyme extract. The results were expressed in µmol H2O2 min−1 mg−1protein.
Tomato yield assessment
Mature tomato fruits were harvested from each treatment. The number, weight and diameter of the fruits were measured.
Fruit titratable acidity and total soluble solids assessment
Titratable acidity (TA) was determined according to Formula 5 described by Pastori et al. (Citation2017):
Where, Vs: Volume of NaOH solution (mL) used for titration; and 10: Volume (mL) of juice used.
Total soluble solids (TSS) content was assessed using the method described by Tigist et al. Citation2011). The juice was obtained from the fruit of each treatment and then the TSS was measured with a digital refractometer that reported the amount of sugar in °Brix.
The maturity index (MI) was calculated using Formula 6:
Where, TSS: total soluble solids; and TA: titratable activity.
Qualitative analyses of fruits
Carotenoid analysis was performed according to Arnon (Citation1949). Finely ground fruit (200 mg) was mixed with 80% (v/v) acetone in 2 mL cryotubes containing glass beads, and then centrifuged at 10 000 rpm for 10 min. During the extraction process, tubes were wrapped in aluminium foil to prevent the oxidation of pigments. The extraction was repeated two to four times until the solid residue was colourless. The absorbance of the supernatant and acetone was measured at wavelengths of 663 nm, 645 nm, and 470 nm. Finally, the total carotenoid level was determined using the following equations:
Where, A is the absorbance at a given wavelength; V is the volume of the supernatant (cm3); and m is the mass of the fruit sample (g).
The level of lycopene was determined using the method described by Sadler et al. (Citation1990). Frozen ground material was extracted with 12.5 mL of ethanol: hexane solution at a volume ratio of 4:3 for 10 min. Then, 2 mL of distilled water was added. Afterwards, the mixture was vortexed for 10s and left to stand on ice for 10 min. The absorbance of the upper phase was measured at 502 nm using a UV/visible spectrophotometer. Finally, the lycopene level was calculated using the following equation (Hilares et al. Citation2019):
Where, MW represents the molecular weight of lycopene (537 g mole−1); V represents the volume of the hexane layer (L); m represents sample mass (g); and ξ represents the molar extinction coefficient of lycopene in hexane (1.72 x 105 L mol−1 cm−1) at 502 nm.
The water content (WC) was determined as per the following method: fully ripened fruits of each plant were weighed to determine their fresh weight (FW), cut into pieces, and placed in an oven (70°C) to measure their dry weight (DW) (Chafi et al. Citation2015). Finally, WC was determined using the formula:
Statistical analysis
Experimental data are given as mean ± standard deviation (SD) based on five replicates for each analysis. Statistical analysis was performed with COSTAT 6.4 for Windows. All results were analysed using three-way analysis of variance (ANOVA) (pathogen inoculation, compost and PGPR interactions). Comparisons between means were evaluated using Tukey’s HSD test calculated at p < 0.05.
Results
Impact of compost, ER10 and/or Verticillium infection on tomato plant growth
Infection with Verticillium showed a significant (P < 0.001, ) negative effect on the growth of tomato plants (). However, biostimulant application significantly increased growth parameters. In the presence of V. dahliae, the largest increases in height, and total fresh and dry weights were recorded in CV plants at 68%, 97% and 71%, respectively (Fig. A, B and C). For CBV treated plants, the number of leaves was 104% compared with V plants (Figure D). Interactions among CBV treatments had a significant positive effect (P < 0.01, ) on total fresh weight.
Table 2. Result of three-way ANOVA test for independent variables including compost (C), PGPR (B), Verticillium dahliae (V) treatments and their interaction.
Fig. 1 (a) Plant height, (b) Number of leaves, (c) Total dry weight, (d) Total fresh weight of tomato plants infected by Verticillium dahliae or not in the presence of different biostimulant combination after 5 months of cultivation. Control; C: Compost; B: Plant growth-promoting Rhizobacteria; CB: Compost + Plant growth-promoting Rhizobacteria; V: V. dahliae; CV: Compost + V. dahliae; BV: Plant growth-promoting Rhizobacteria + V. dahliae; CBV: Compost + Plant Growth-promoting Rhizobacteria + V. dahliae. Data are based on five replicates; bars with different letters significantly differ according to Tukey’s HSD test at p < 0.05.
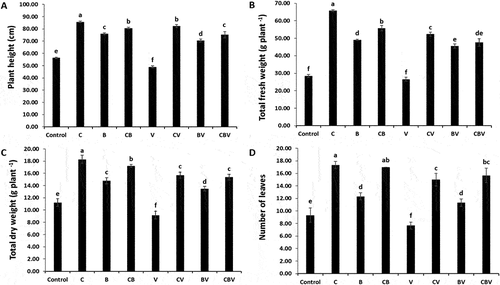
Impact of compost, ER10 and/or Verticillium infection on tomato plant physiology
Physiological traits of tomato plants were significantly (P < 0.001, ) affected by the pathogen, however, the application of biofertilizers improved these parameters. The highest increase in chlorophyll fluorescence (27%) was recorded for the CV treatment in comparison with V plants, followed by the BV and CBV treatments (). The use of compost or ER10 alone or in combination increased stomatal conductance as compared with V plants (). Interactions among CBV treatment had a significant positive effect (P < 0.001, ) on photosynthetic efficiency.
Fig. 2 (a) Photosynthetic efficiency and (b) stomatal conductance of tomato plants infected by Verticillium dahliae or not in the presence of different biostimulant combination after 5 months of cultivation. Control; C: Compost; B: Plant growth-promoting Rhizobacteria; CB: Compost + Plant growth-promoting Rhizobacteria; V: V. dahliae; CV: Compost + V. dahliae; BV: Plant growth-promoting Rhizobacteria + V. dahliae; CBV: Compost + plant growth-promoting Rhizobacteria + V. dahliae. Data are based on five replicates; bars with different letters significantly differ according to Tukey’s HSD test at p < 0.05.
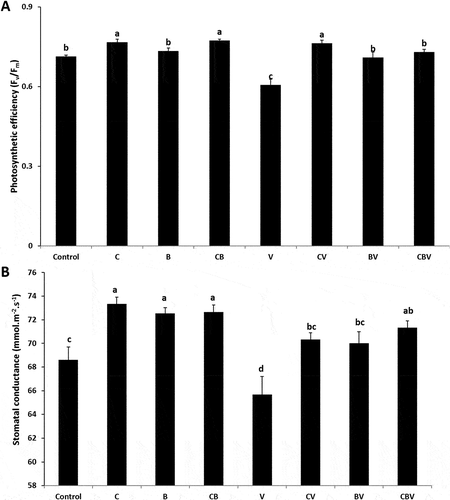
Impact of compost, ER10 and/or Verticillium infection on the mineral composition of tomato plants
We analysed P, K+, Mg2+, Ca2+and Na+ content in tomato leaves treated or not with biofertilizers in the absence and presence of V. dahliae, as the degree of tolerance depends on their absorption and translocation (Raklami et al. Citation2019). In the absence of V. dahliae, the highest values of K+, Mg2+, Ca2+ and Na+ were recorded in the CB bipartite combination (), at 462%, 50%, 70% and 344%, respectively, relative to the control plants. The nutrient status of tomato leaves (P, K+, Mg2+, Ca2+ and Na+) was significantly increased by all of the biofertilizers applied, independent of the presence or absence of the pathogen, compared with non-treated plants (). Application of the pathogen increased K+, Mg2+, Ca2+ and Na+ concentrations in V plants compared with the control. In addition, biofertilizers further increased these nutrients.
Fig. 3 (a) phosphorous, (b) potassium, (c) magnesium, (d) calcium, (e) sodium in the leaves of tomato plants infected by Verticillium dahliae or not in the presence of different biostimulants combination after 5 months of cultivation. Control; C: Compost; B: Plant growth-promoting Rhizobacteria; CB: Compost + Plant growth-promoting Rhizobacteria; V: V. dahliae; CV: Compost + V. dahliae; BV: Plant growth-promoting Rhizobacteria + V. dahliae; CBV: Compost + plant growth-promoting Rhizobacteria + V. dahliae. Data are based on five replicates; bars with different letters significantly differ according to Tukey’s HSD test at p < 0.05.
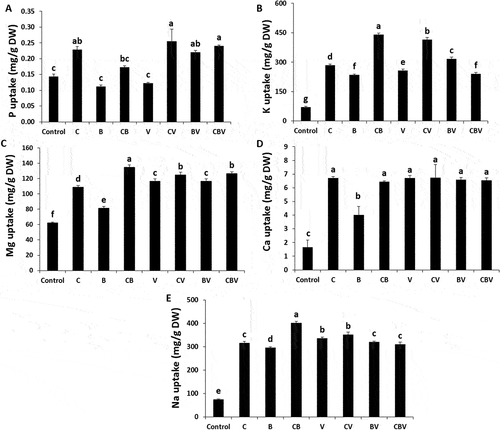
Impact of compost, ER10 and/or Verticillium infection on the evolution of disease symptoms of tomato plants
The protective potential of ER10 against V. dahliae was evaluated individually and/or in combination with compost. The results indicated that the CBV treatment significantly reduced disease severity by 27% after 49 days from inoculation with the pathogen compared with the V. dahliae inoculated control (). However, the CV and BV treatments reduced the disease severity by 24% and 18%, respectively.
Fig. 4 Severity of the disease caused by Verticillium dahliae on tomato plants infected by V. dahliae in the presence of different biostimulant combination after 5 months of cultivation. V: V. dahliae; CV: Compost + V. dahliae; BV: Plant growth-promoting Rhizobacteria + V. dahliae; CBV: Compost + Plant growth-promoting Rhizobacteria + V. dahliae. Data are based on five replicates.
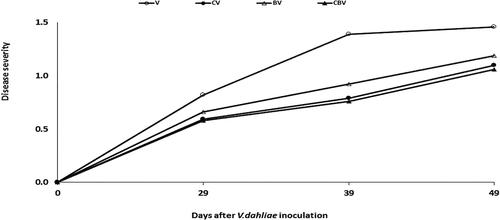
Similarly, disease incidence was reduced by 25% in both the CBV and CV treatments and by 22% in BV treated plants compared with the control 49 days after inoculation with the pathogen (). The CBV treatment was very effective in reducing the foliar alteration index by 73%, followed by CV (71%) and BV (61%) (). In contrast, the CBV treatment reduced AUDPC and FMS by 33% and 35%, respectively, followed by the CV and then BV treatments, relative to V plants ()). The interactions between C × B × V were significant (P < 0.001, ) for the symptom parameters.
Fig. 5 (a) Disease incidence, (b) Leaf alteration index, (c) AUDPC, (d) FMS caused by Verticillium dahliae on tomato plants infected by V. dahliae in the presence of different biostimulant combination after 5 months of cultivation. V: V. dahliae; CV: Compost + V. dahliae; BV: Plant Growth-Promoting Rhizobacteria + V. dahliae; CBV: Compost + Plant Growth-Promoting Rhizobacteria + V. dahliae. Data are based on five replicates; bars with different letters significantly differ according to Tukey’s HSD test at p < 0.05.
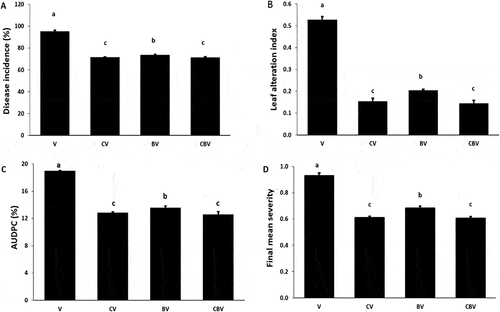
Impact of compost, ER10 and/or Verticillium infection on lipid peroxidation and hydrogen peroxide content of tomato plants
In the presence of V. dahliae, leaf and root H2O2 and MDA content increased considerably in control tomato plants (). However, the application of biostimulants was effective in lowering these indicators of oxidative damage. The BV and CBV treatments reduced leaf MDA content in a similar manner (47% compared with V plants), while the lowest value of leaf H2O2 content was recorded in the CBV treatment (77% relative to V plants).
Fig. 6 (a) (H2O2) in the leaves, (b) in the root; Malondialdehyde MDA (c) in the leaves and (d) in the root of tomato plants infected by Verticillium dahliae or not in the presence of different biostimulant combination after 5 months of cultivation. Control; C: Compost; B: Plant Growth-Promoting Rhizobacteria; CB: Compost + Plant Growth-Promoting Rhizobacteria; V: V. dahliae; CV: Compost + V. dahliae; BV: Plant Growth-Promoting Rhizobacteria + V. dahliae; CBV: Compost + Plant Growth-Promoting Rhizobacteria + V. dahliae. Data are based on five replicates; bars with different letters significantly differ according to Tukey’s HSD test at p < 0.05.
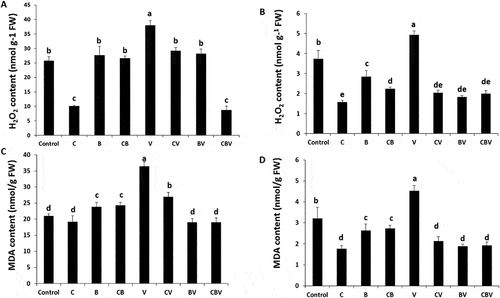
Impact of compost, ER10 and/or Verticillium infection on protein content and antioxidant enzyme activity
Shoot protein content was significantly improved in plants treated with compost compared with the control or the B and CB treatments in the absence of V. dahliae (). Under the same conditions, the root protein content was significantly enhanced by all treatments except the control, especially in the case of treatments CB and C. However, in the presence of V. dahliae, shoot protein content was mostly enhanced in CV (95%) followed by CBV (84%) and BV (25%) treatments compared with V plants, while protein content in roots was significantly improved in the BV treatment (67%) compared with the others treatments.
Fig. 7 (a) Peroxidase (POX), (b) Polyphenoloxidase (PPO), (c) Superoxide dismutase (SOD), (d) Catalase (CAT) in the leaves and roots of tomato plants infected or not by the pathogen Verticillium dahliae after 5 months. Data are based on five replicates; bars with different (n = 5) letters significantly differ according to Tukey’s HSD test at p < 0.05.
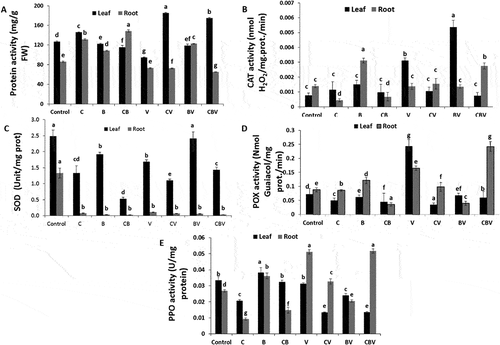
Application of V. dahliae induced an increase in antioxidant activities in leaves and roots (). Incorporation of compost alone or combined with ER10 reduced CAT, SOD, POX and PPO activities at the leaf level by 77%, 15%, 79% and 67%, respectively, for the CBV treatment and by 67%, 34%, 87%, and 67%, respectively, in the CV treatment relative to V plants. However, inoculation of ER10 alone increased CAT and SOD activities within the shoot by 67% and 43%, respectively. As for the roots, the bipartite combinations of compost and ER10 significantly increased CAT and POX activities by 100% and 47% compared with control plants in the presence of the pathogen ().
Impact of compost, ER10 and/or Verticillium infection on tomato yield and quality
In order to verify the effects of biostimulants on fruit production as well as their influence on disease severity, we determined the weight, number, and diameter of fruits and measured some important metabolites indicative of fruit maturity and quality ().
Fig. 8 (a) Total weight of tomato fruits; (b) Fruit number; (c): Fruit diameter of tomato plants infected by Verticillium dahliae or not in the presence of different biostimulant combination after 5 months of cultivation. Control; C: Compost; B: Plant Growth-Promoting Rhizobacteria; CB: Compost+ Plant Growth-Promoting Rhizobacteria; V: V. dahliae; CV: Compost + V. dahliae; BV: Plant Growth-Promoting Rhizobacteria + V. dahliae; CBV: Compost + Plant Growth-Promoting Rhizobacteria + V. dahliae. Data are based on five replicates; bars with different letters significantly differ according to Tukey’s HSD test at p < 0.05.
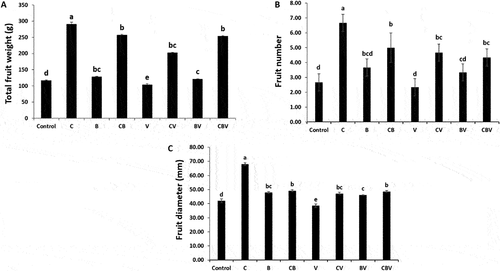
With respect to fruit weight, multifactorial analysis of variance showed that the interactions between all the biostimulants and V. dahliae were significant (P < 0.01), except those between ER10 and compost (). In addition, biostimulants applied separately or in combination had a positive effect on fruit weight, number and diameter either in the absence or presence of the pathogen (). The C and CB treatments significantly increased these parameters in comparison with the controls. However, a significant decrease was observed in these traits with the application of V. dahliae. Interestingly, the application of compost alone or combined with ER10 suppressed the negative effect of V. dahliae on fruit weight, number and diameter, while the application of ER10 had a low impact. The interactions between all biostimulants and V. dahliae had significant effects on these parameters except for fruit number ().
The data showed that V. dahliae significantly (P < 0.001) increased total acidity (TA) and decreased total soluble sugar (TSS) and maturity index (MI) ( and ). The application of biostimulants had a considerable beneficial effect on the parameters measured in fruit from both infected and non-infected plants (). Moreover, tomatoes from plants treated only with compost or ER10 had a greater increase in TA, TSS, and MI. Additionally, in infected plants, the greatest TA and TSS values were in fruit from plants treated with compost, while the greatest MI occurred in tomatoes from plants that received combined treatments (). There were significant (P < 0.05) differences between fruit from plants treated or not treated with B in terms of water content (WC) ( and ). A notable decrease in WC level was observed in fruits from plants treated with B, CB, and CBV, however, a significant increase was observed in fruits from plants treated with BV relative to control plants. All treatments (application of pathogen, biostimulants, and their combination) increased lycopene and carotenoid content ()). The highest value of lycopene was observed in fruit from plants that received compost (12.37 mg kg−1 FW) and CBV (11.60 mg kg−1 FW). Moreover, carotenoid content was elevated in fruit of the CV (1.46 mg g−1 FW) and CBV (1.41 mg g−1 FW) treatments.
Fig. 9 (a) Total fruit weight, (b) Fruit number, (c) Fruit diameter, (d) Maturity index of tomato plants infected by Verticillium dahliae or not in the presence of different biostimulant combination after 5 months of cultivation. Control; C: Compost; B: Plant Growth-Promoting Rhizobacteria; CB: Compost+ Plant Growth-Promoting Rhizobacteria; V: V. dahliae; CV: Compost + V. dahliae; BV: Plant Growth-Promoting Rhizobacteria + V. dahliae; CBV: Compost + Plant Growth-Promoting Rhizobacteria + V. dahliae. Data are based on five replicates; bars with different letters significantly differ according to Tukey’s HSD test at p < 0.05.
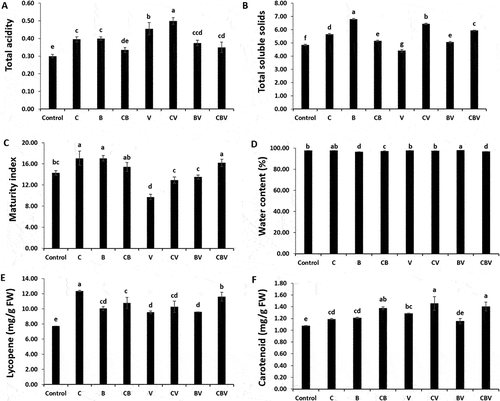
Discussion
The application of biostimulants such as compost and PGPR may represent suitable substitutes for synthetic chemical fungicides and genetically resistant plants (Bensidhoum et al. Citation2016; Tabli et al. Citation2018). Although most biostimulants used as biocontrol agents demonstrate an inhibitory effect on plant pathogens and at the level of many plant pathological systems (Koné et al. Citation2010), this study indicates, for the first time, the synergistic effect of green waste compost application alone and/or combined with ER10 on the improvement of tomato tolerance to V. dahliae. The tomato plants infected with the pathogen showed reduced growth compared with the uninfected plants. In addition, the use of compost alone and/or combined with ER10 improved the growth of tomato plants in the presence of V. dahliae. Several studies have shown that treating plants with compost improves their growth in the presence of pathogens (Akhtar et al. Citation2016; Shi et al. Citation2016; Samet et al. Citation2018). Furthermore, the application of ER10 improved the growth parameters of plants infected with the pathogen compared to uninfected control plants (Akhtar et al. Citation2016). The improved growth of tomato plants infected with V. dahliae in the presence of organic amendment (C) and/or the bacteria inoculum (B) may be due to improved uptake of mineral elements, changes in root system architecture, and the activation of the plant defence system, which may be implicated in the protection of the plant root tissues against the pathogen (Diagne et al. Citation2020). The application of compost alone and/or combined with PGPR resulted in an improvement in the growth parameters of plants affected by V. dahliae, which may also be due to the reduction of the stress caused by the pathogen. These results corroborate previous work showing that incorporation of PGPR with organic soil amendments resulted in improved plant health and protection against plant pathogens (Antoniou et al. Citation2017). Indeed, On et al. (Citation2015) reported that the incorporation of Bacillus subtilis inhibited the mycelial growth of A. solani, potentially due to the production of biosurfactants like iturin and fengycin causing antibiosis to fungal pathogens.
Alfano et al. (Citation2011) and Markakis et al. (Citation2016) have demonstrated that phenolics are among the abiotic factors that contribute to the suppressive activity of compost amendments against soil pathogens. In addition, PGPR such as Bacillus spp. and Pseudomonas spp. also can minimize pathogen damage by producing a diverse range of antibiotics and are among the most effective biocontrol mechanisms (Haldar and Sanghamitra Citation2015). The PGPR exert their antagonism towards several plant pathogens by using secreted siderophores that function by sequestering iron in the root zone, making it unavailable to pathogens and inhibiting their growth. Siderophores increase iron uptake by plants that can utilize the bacterial iron-siderophore complex (Murali Sankar et al. Citation2021). Furthermore, siderophores have the ability to suppress several fungal pathogens and improve the growth of many crops (Bensidhoum et al. Citation2016; Tabli et al. Citation2018; Singh et al. Citation2019). Several studies have reported that PGPR have the ability to suppress pathogen growth and activity by secreting plant growth regulators such as lytic enzymes like 1-aminocyclopropane-1-carboxylic acid (ACC-deaminase), cellulases, chitinase, lipases, proteases and β-1,3-glucanase that are involved in lysis of the fungal cell wall (Goswami et al. Citation2016). On the other hand, β-1,3-glucanase and chitinase producing bacteria have the capacity to destroy the fungal cell wall, which consists of glucans, chitin and polysaccharides. Therefore, the expression of lytic enzymes by PGPR is effective in enhancing pathogen suppression (Singh et al. Citation2019).
This study has confirmed the beneficial effect of biostimulants applied alone or in combination in improving tomato plant growth when infected by V. dahliae disease. This improvement of growth parameters is mainly associated with the improvement of physiological parameters of tomato plants affected by the pathogen, amended with compost and/or inoculated with ER10. The results indicated that treated tomato plants had increased stomatal conductance and chlorophyll fluorescence, in contrast with decreases in these parameters in non-inoculated and non-amended tomato plants. Verticillium dahliae reduced stomatal conductance in tomato plants primarily due to stomatal closure, which accounts for decreased leaf conductance and transpiration rates (Zarco-Tejada et al. Citation2021). This result is in agreement with data reported for other plant species (Pascual et al. Citation2009; Mauro et al. Citation2020). The pathogen decreases stomatal conductance in plants because the fungus obstructs xylem vessels, which leads to leaf water deficits (Sadras et al. Citation2000; Pascual et al. Citation2009; Mauro et al. Citation2020). The alteration in chlorophyll fluorescence observed in tomato plants affected by V. dahliae is a consequence of the plant’s regulatory response to fungal attack, which results in damage to the photosynthetic apparatus (Bermúdez-Cardona et al. Citation2015). Plants inoculated with PGPR and/or amended with compost compensated for decreases in chlorophyll fluorescence by relatively unchanged dark and light respiration, a process that consumes electrons generated in the photosynthetic electron transport chain, by maintaining or increasing the substomatal CO2 concentration (Bulgari et al. Citation2019).
In addition, chlorophyll fluorescence was virtually unaffected by the pathogen, suggesting the absence of permanent photoinhibition and subsequent photodamage. This improvement could also be explained by the ability of compost alone and/or combined with ER10 to increase CO2 uptake and the photosynthetic activity of tomato. There are two possibilities for the improvement in chlorophyll fluorescence of tomato plants inoculated with V. dahliae grown in the presence of ER10 and/or compost. This might be explained by both the key role of PGPR in solubilizing unavailable mineral elements via the production of organic acids (Kaur et al. Citation2021) and by the increased content of available nutrients, which could be due to better nutrient retention, reduced efflux, and/or decreased catabolism due to the compost (Anli et al. Citation2020; Mauro et al. Citation2020). Moreover, the improvement in growth and physiology of pathogen-infected tomato inoculated with PGPR and/or amended with compost may be due to the ability of these biostimulants to produce several enzymes such as amylase, cellulase, pectinase, and protease, which could degrade the cell walls of harmful microorganisms and thus reduce biotic stress (Geetha et al. Citation2014; Akhtar et al. Citation2016; Yu et al. Citation2019).
Our data showed that the use of compost supplemented or not with ER10 had a positive effect on Na+, K+, Ca2+, P and Mg2+ uptake both in the absence or presence of V. dahliae. Previous studies with olive (López-Moral et al. Citation2021) and eggplant (Ali et al. Citation2021) indicated that the application of biostimulants resulted in a higher level of nutrient uptake as well as higher biomass yield and induced defence responses against V. dahliae. Improved absorption, efficiency and transport of mineral elements, particularly Mg2+, is necessary for the synthesis of chlorophyll and is a cofactor for several critical cellular processes, including photosynthesis (Baslam et al. Citation2020).
This study highlighted the interactions between pathogen, plant and biostimulants to modulate the effects of fungal invasion. Moreover, our results showed that the application of compost alone and/or combined with ER10 significantly reduced the stress on tomato plants caused by the pathogen. The application of soil amendments with organic materials originating from agro-industrial co-products and green plant waste (green composts) is an innovative strategy to improve soil quality and carbon sequestration while improving the efficiency of disease suppression (Lehmann et al. Citation2011; De Corato et al. Citation2019). It is also very well known that the addition of compost plays a crucial role through various mechanisms, including ISR in order to improve the plant defence system (Yogev et al. Citation2010). There are many reports of the positive effects of compost inoculated with bio-control agents such as Trichoderma hamatum or B. subtilis on disease suppression, as it contains multifaceted microbial consortia. Previous studies showed that application of biocontrol agents and compost reduced pathogen populations in the soil (Cucu et al. Citation2018; Shen et al. Citation2021). Similarly, results confirmed that some Bacillus strains have a potential effect against Phytophthora blight caused by P. capsici on squash (Gilardi et al. Citation2015). Thus, these authors confirmed that the release of volatile compounds contained in the organic amendments were able to inhibit the mycelial growth of V. dahliae (Vitullo et al. Citation2013; Kowalska et al. Citation2021). In addition, Zhang et al. (Citation2018) showed that root colonization of tomato plants by AMF strongly stimulated the expression of Ve genes involved in the inhibition of V. dahliae. Our study shows strong root colonization in the combination of AMF with compost, which may explain the effect of AMF on the expression of Ve genes. Furthermore, Panno et al. (Citation2021) reported that the use of organic amendments in combination with biocontrol agents allowed tomato plants to resist V. dahliae through the expression of Ve genes.
Antoniou et al. (Citation2017) reported that disease suppression by compost application in tomato plants could be due to the presence of microbial communities, especially Bacillus species, in the compost. Similar results indicated that inoculation with the biocontrol agent Enterobacter cloacae into compost metabolized fatty acids and inhibited germination of Pythium ultimum zoospores, thereby reducing disease incidence (McKellar et al. Citation2003). PGPRs behave as a dynamic network that inhibits invasion, infection and disease severity (Hacquard et al. Citation2017). Inoculation with Bacillus amyloliquefaciens enhances disease suppression through the production of antifungal compounds such as Bacillomycin D, secretion of proteins like acetolactate synthase (Kierul et al. Citation2015; Gu et al. Citation2017) and induction of systemic resistance by producing several enzymes and antibiotics, which compete for niches with pathogens in the rhizosphere. Previous studies have confirmed the positive effects of PGPRs, under biotic stress, via the production of siderophores (pyovidin) and organic acids that mitigate damage and protect plants through several mechanisms (Waqas et al. Citation2015; Köhl et al. Citation2019).
Plants can employ several defence mechanisms to counteract the detrimental effects of biotic stress by accumulating suitable osmolytes in the cytosol. Furthermore, compost application and/or PGPR inoculation were found to positively influence the production of key osmolytes such as protein, proline, and glycine betaine, which can promote photosynthesis by improving the chloroplast cellular functions (McNeece et al. Citation2019; Aalipour et al. Citation2020; Abdel Latef et al. Citation2021). In this study, V. dahliae inoculation resulted in the accumulation of protein content in tomato plants. The enhanced protein content in the presence of V. dahliae might be attributed to enhanced biosynthesis related to biotic stress to preserve cell homoeostasis. Plants treated with compost and/or PGPR can accumulate greater amounts of osmolytes, which might preserve and increase photosynthetic activity in harsh environments (McNeece et al. Citation2019; Meena et al. Citation2020). The accumulation of osmoprotectants such as protein can induce physicochemical changes by producing defence compounds in stressed plants (Chung Citation2012). It is well known that increasing cellular osmolytes can help the plant maintain cellular functions to ensure physiological stability against stress (Ullah et al. Citation2019).
In this study, there was an early and rapid increase in H2O2 accumulation after inoculation of the pathogen, whereas plants inoculated with PGPR or amended with compost showed lower levels of H2O2 and MDA when attacked by the pathogen, which explains an important characteristic of plant resistance to pathogens. These results are in agreement with the observations of Gupta et al. (Citation2015) and Lian et al. (Citation2017), who noticed that H2O2 and MDA levels increased within infected plants. Indeed, the exposure of plants to biotic stress causes the production of reactive oxygen species (ROS) that trigger the stress response and defence pathways (Talaat et al. Citation2019). These reactive species cause oxidative damage, imbalance of cell functions, inactivation of enzymes, slowing of metabolic activities and consequently cell death (Gupta et al. Citation2015; Khanna et al. Citation2019). The application of PGPR and compost could limit the production of ROS, successfully reducing cellular damage under oxidative stress conditions. Furthermore, H2O2 induces activation of a 46-kD mitogen-activated protein kinase (MAPK) and increases the expression of antioxidant genes (Zhang et al. Citation2006).
Environmental stresses such as microbial pathogen infection cause oxidative stress in plants by increasing the production of ROS (Xie et al. Citation2019). To protect themselves from this harm, plant species have developed enzymatic and non-enzymatic antioxidant systems that work together to detoxify ROS (Hasanuzzaman et al. Citation2020a). In this study, compost application and/or bacteria inoculation significantly improved antioxidant enzyme activity, except for SOD activity, compared with the controls. Antioxidant enzymes such as POX, PPO, CAT and SOD are effective as defence enzymes against abiotic and biotic stresses as they modulate ROS detoxification (Helaly et al. Citation2018; Hasanuzzaman et al. Citation2020b) and processes of cell lignification (Lee et al. Citation2007; Lanna-Filho et al. Citation2017). Furthermore, metalloenzymes like PPO, SOD, and CAT are influenced by the concentration of minerals such as Cu, Fe and Mn as well as Zn in plants (Tang et al. Citation2015; Gong et al. Citation2018; Jha Citation2019). These mineral micronutrients could be improved in inoculated plants with beneficial microbes and/or amended ones with organic fertilizers (Shen et al. Citation2013; Xiong et al. Citation2017). In addition, organic fertilizer increased the antioxidant content of the plants, which improved their resistance to pests and diseases (Shafique et al. Citation2015). The capacity of organic fertilizer to reduce oxidative stress in plants could be due to the presence of humic compounds, which promote plant development by enhancing soil bioavailability of specific nutrients, namely Fe2+ and Zn2+, acting as plant growth regulators, or influencing metabolic processes (Samet et al. Citation2018). Compost can also work by upregulating phytohormones including abscisic acid, gibberellins, and cytokinin, which help to reduce oxidative stress (Pant et al. Citation2012). Moreover, tomato plant defence system modulation by compost application and/or beneficial bacteria inoculation might have a beneficial effect against V. dahliae.
In this study, plants treated with compost and/or ER10 had the best results with respect to TFW, NF, DF, and TSS as well as lycopene and carotenoid content. Thus, the application of biostimulants (bacteria and compost) significantly increased fruit production with concurrent acceleration in maturation and improvement in antioxidant levels, compared with fruit from non-treated plants. These results are in agreement with the findings of previous researchers (Almaghrabi et al. Citation2013; Chandrasekaran et al. Citation2019; Rahi et al. Citation2021). González-Hernández et al. (Citation2021) showed that the application of compost tea increased pepper production and resistance to pathogens. Moreover, the impact of bacteria was very pronounced with regard to fruit development and maturation, whereas the application of compost had the opposite effect. Even beneficial bacteria stimulate cell division, expansion, and differentiation through the secretion of phytohormones (e.g. indole acetic acid) and help plants to obtain nitrogen from the atmosphere or other nutrients available in the soil; however, it cannot not make up for those unavailable in the soil (Ahemad and Kibret Citation2014). The effect of compost application could be attributed to increasing the quantity of nutrients (i.e. NO3− and K2O) that are involved in many physiological and biochemical processes, thereby promoting plant growth and fruit development. In addition, compost application may have resulted in the production of phytohormones as well as growth promoters (i.e. humic substances and beneficial microbia) (Abd El-Razek et al. Citation2020; González-Hernández et al. Citation2021). Thus, there are a number of reasons for the positive effect of compost application on fruit growth and lycopene content. These include the improvement of soil biochemical properties, increases in the expression of genes and activity of enzymes involved in nutrient assimilation, as well as the stimulation of cell division and fruit development (Nardi et al. Citation2021). This is the first report showing that the combined application of compost with beneficial bacteria increased yield as well as fruit maturity and quality markers. These results might be explained by the fact that the application of bacteria competes for food with the beneficial microorganisms existing in the compost, thus decreasing the effect of the compost. Moreover, we note that the application of biostimulants (C, B, and C + B) had a pivotal role in preventing tomato fruit yield losses caused by V. dahliae.
The experiments conducted in this study confirmed that the application of a combination of compost with ER10 can be a successful strategy to reduce the damage caused by V. dahliae on greenhouse tomato by more than 50%. Furthermore, this combination showed beneficial effects on the growth, physiological and mineral parameters of the plants. The inoculation with ER10 and amendment with compost stimulated antioxidant activities and decreased stress markers in the presence of the pathogen, while improving the growth and maturity of tomato fruits. Thus, the application of compost and ER10 protected tomato plants against V. dahliae, increasing yield and quality, suggesting the potential for adoption by the industry to improve agricultural sustainability.
Supplementary_May_05__2022.docx
Download MS Word (479.4 KB)Disclosure statement
No potential conflict of interest was reported by the author(s).
Supplementary material
Supplemental data for this article can be accessed online here: https://doi.org/10.1080/07060661.2022.2089235
Additional information
Funding
References
- Aalipour H, Nikbakht A, Etemadi N, Rejali F, Soleimani M. 2020. Biochemical response and interactions between arbuscular mycorrhizal fungi and plant growth promoting rhizobacteria during establishment and stimulating growth of Arizona cypress (Cupressus arizonica G.) under drought stress. Sci Hortic. 261:108923. doi:10.1016/j.scienta.2019.108923.
- Abd El-Razek E, Haggag LF, El-Hady ES, Shahin MFM. 2020. Effect of soil application of humic acid and bio-humic on yield and fruit quality of “Kalamata” olive trees. Bullet Nat Res Centre. 44(1):1–8. doi:10.1186/s42269-020-00318-8.
- Abdel Latef AAH, Tahjib-Ul-Arif M, Rhaman MS. 2021. Exogenous Auxin-mediated salt stress Alleviation in Faba Bean (Vicia faba L.). Agronomy. 11(3):547. doi:10.3390/agronomy11030547.
- Aebi H. 1984. Catalase in vitro. Meth Enzymol. 105:121–126. doi:10.1016/s0076-6879(84)05016-3.
- Ahemad M, Kibret M. 2014. Mechanisms and applications of plant growth promoting rhizobacteria: current perspective. J King Saud Univ Sci. 26(1):1–20. doi:10.1016/j.jksus.2013.05.001.
- Ait Rahou Y, Ait-El-Mokhtar M, Anli M, Boutasknit A, Ben-Laouane R, Douira A, Benkirane R, El Modafar C, Meddich A. 2021. Use of mycorrhizal fungi and compost for improving the growth and yield of tomato and its resistance to Verticillium dahliae. Arch Phytopathol Plant Prot. 1:26. doi:10.1080/03235408.2020.1854938.
- Ait-El-Mokhtar M, Baslam M, Ben-Laouane R, Anli M, Boutasknit A, Mitsui T, Wahbi S, Meddich A. 2020. Alleviation of detrimental effects of salt stress on date palm (Phoenix dactylifera L.) by the application of arbuscular mycorrhizal fungi and/or compost. Front Sustain Food Syst. 4:131. doi:10.3389/fsufs.2020.00131.
- Akhtar N, Naveed M, Iqbal MZ, Khalid M, Waraich EA. 2016. Effect of consortium of plant growth promoting and compost inhabiting bacteria on physicochemical changes and defense response of maize in fungus infested soil. Pak J Agric Sci. 53(1):59–68. doi:10.21162/PAKJAS/16.4700.
- Alfano G, Lustrato G, Lima G, Vitullo D, Ranalli G. 2011. Characterization of composted olive mill wastes to predict potential plant disease suppressiveness. Biological Control. 58(3):199–207. doi:10.1016/j.biocontrol.2011.05.001.
- Ali M, Ahmad H, Hayat S, Ghani MI, Amin B, Atif MJ, Wali K, Cheng Z. 2021. Application of garlic allelochemicals improves growth and induces defense responses in eggplant (Solanum melongena) against Verticillium dahliae. Ecotoxicol Environ Saf. 215:112132. doi:10.1016/j.ecoenv.2021.112132.
- Alikhani HA, Saleh-Rastin N, Antoun H. 2006. Phosphate solubilization activity of rhizobia native to Iranian soils. Plant Soil. 287(1–2):35–41. doi:10.1007/s11104-006-9059-6.
- Almaghrabi OA, Massoud SI, Abdelmoneim TS. 2013. Influence of inoculation with plant growth promoting rhizobacteria (PGPR) on tomato plant growth and nematode reproduction under greenhouse conditions. Saudi J Biol Sci. 20(1):57–61. doi:10.1016/j.sjbs.2012.10.004.
- Anli M, Baslam M, Tahiri A, Raklami A, Symanczik S, Boutasknit A, Ait-El-Mokhtar M, Ben-Laouane R, Toubali S, Ait-Rahou Y, et al. 2020. Biofertilizers as strategies to improve photosynthetic apparatus, growth, and drought stress tolerance in the date palm. Front Plant Sci. 11:516818. doi:10.3389/fpls.2020.516818.
- Antoniou A, Tsolakidou MD, Stringlis IA, Pantelides IS. 2017. Rhizosphere microbiome recruited from a suppressive compost improves plant fitness and increases protection against vascular wilt pathogens of tomato. Front Plant Sci. 8:2022. doi:10.3389/fpls.2017.02022.
- Arnon DI. 1949. Copper enzymes in isolated chloroplasts. Polyphenoloxidase in Beta vulgaris. J Plant Physiol. 24(1):1. doi:10.1104/pp.24.1.1.
- Attia MS, El-Sayyad GS, Abd Elkodous M, El-Batal AI. 2020. The effective antagonistic potential of plant growth-promoting rhizobacteria against Alternaria solani causing early blight disease in tomato plant. Sci Hortic. 266:109289. doi:10.1016/j.scienta.2020.109289.
- Baslam M, Mitsui T, Hodges M, Priesack E, Herritt MT, Aranjuelo I, Sanz-Sáez Á. 2020. Photosynthesis in a changing global climate: scaling up and scaling down in crops. Front Plant Sci. 11:882. doi:10.3389/fpls.2020.00882.
- Bensidhoum L, Nabti E, Tabli N, Kupferschmied P, Weiss A, Rothballer M, Schmid M, Keel C, Hartmann A. 2016. Heavy metal tolerant Pseudomonas protegens isolates from agricultural well water in northeastern Algeria with plant growth promoting, insecticidal and antifungal activities. Eur J Soil Biol. 75:38–46. doi:10.1016/j.ejsobi.2016.04.006.
- Bermúdez-Cardona MB, Filho JAW, Rodrigues FA. 2015. Leaf gas exchange and chlorophyll a fluorescence in maize leaves infected with Stenocarpella macrospora. Phytopathology. 105(1):26–34. doi:10.1094/PHYTO-04-14-0096-R.
- Beyer WF, Fridovich I. 1987. Assaying for superoxide dismutase activity: some large consequences of minor changes in conditions. Anal Biochem. 161(2):559–566. doi:10.1016/0003-2697(87)90489-1.
- Bonilla N, Gutiérrez-Barranquero JA, De Vicente A, Cazorla FM. 2012. Enhancing soil quality and plant health through suppressive organic amendments. Diversity. 4(4):475–491. doi:10.3390/d4040475.
- Boutaj H, Meddich A, Wahbi S, Moukhli A, El Alaoui-Talibi Z, Douira A, Filali-Maltouf A, El Modafar C. 2019. Effect of Arbuscular Mycorrhizal Fungi on Verticillium wilt development of olive trees caused by Verticillium dahliae. J Biotechnol. 14(8):79-–88.
- Bulgari R, Franzoni G, Ferrante A. 2019. Biostimulants application in horticultural crops under abiotic stress conditions. Agronomy. 9(6):306. doi:10.3390/agronomy9060306.
- Campbell CL, Madden LV. 1990. Introduction to plant disease epidemiology. New York (NY): John Wiley & Sons.
- Campestrini LH, Melo PS, Peres LEP, Calhelha RC, Ferreira ICFR, Alencar SM. 2019. A new variety of purple tomato as a rich source of bioactive carotenoids and its potential health benefits. Heliyon. 5(11):e02831. doi:10.1016/j.heliyon.2019.e02831.
- Chafi A, Benabbes R, Bouakka M, Hakkou A, Kouddane N, Berrichi A. 2015. Pomological study of dates of some date palm varieties cultivated in Figuig oasis. J Mater Environ Sci. 6:1266–1275.
- Chandrasekaran M, Chun SC, Oh JW, Paramasivan M, Saini RK, Sahayarayan JJ. 2019. Bacillus subtilis CBR05 for tomato (Solanum lycopersicum) fruits in South Korea as a novel plant probiotic bacterium (PPB): implications from total phenolics, flavonoids, and carotenoids content for fruit quality. Agronomy. 9(12):838. doi:10.3390/agronomy9120838.
- Chavan VA, Yumlembam RA, Sewakram K, Borkar S. 2017. Fungicide resistance in Alternaria leaf blight pathogen in tomato crop grown in Satara District. J Pharmacogn Phytochem. 6:1736–1739.
- Chen JY, Klosterman SJ, Hu XP, Dai XF, Subbarao KV. 2021. Key insights and research prospects at the Dawn of the population genomics era for Verticillium dahliae. Annu Rev Phytopathol. 59(1):31–51. doi:10.1146/annurev-phyto-020620-121925.
- Chung KR. 2012. Stress response and pathogenicity of the necrotrophic fungal pathogen Alternaria alternata. Scientifica. 2012:1–17. doi:10.6064/2012/635431.
- Çolak NG, Eken NT, Ülger M, Frary A, Doganlar S. 2020. Mapping of quantitative trait loci for antioxidant molecules in tomato fruit: carotenoids, vitamins C and E, glutathione and phenolic acids. Plant Sci. 292:110393. doi:10.1016/j.plantsci.2019.110393.
- Couto D, Zipfel C. 2016. Regulation of pattern recognition receptor signalling in plants. Nat Rev Immunol. 16(9):537–552. doi:10.1038/nri.2016.77.
- Cucu MA, Gilardi G, Pugliese M, Matic S, Ulrich G, Gullino ML, Garibaldi A. 2018. Influence of different biological control agents and compost on total and nitrification driving microbial communities at rhizosphere and soil level in a lettuce- Fusarium oxysporum f. sp. Lactucae pathosystem. J App Microb. 126(3):905–918. doi:10.1111/jam.14153.
- De Corato U, Patruno L, Avella N, Lacolla G, Cucci G. 2019. Composts from green sources show an increased suppressiveness to soil borne plant pathogenic fungi: relationships between physicochemical properties, disease suppression, and the microbiome. Crop Prot. 124:104870. doi:10.1016/j.cropro.2019.104870.
- De Jonge R, van Esse HP, Maruthachalam K, Bolton MD, Santhanam P, Saber MK, Thomma BP. 2012. Tomato immune receptor Ve1 recognizes effector of multiple fungal pathogens uncovered by genome and RNA sequencing. Proc Natl Acad Sci USA. 109(13):5110–5115. doi:10.1073/pnas.1119623109.
- Diagne N, Ngom M, Djighaly PI, Fall D, Hocher V, Svistoonoff S. 2020. Roles of arbuscular mycorrhizal fungi on plant growth and performance: importance in biotic and abiotic stressed regulation. Diversity. 12(10):370. doi:10.3390/d12100370.
- Douira A, Ben Kirane R, Ouazzani Touhami A, Okeke B, Elhaloui NE. 1995. Verticillium wilt of pepper (Capsicum annuum) in Morocco. J Phytopathol. 143(8):467–470. doi:10.1111/j.1439-0434.1995.tb04556.x.
- El Said SH, Hegazi AA, Allatif AMA. 2012. Resistance of some olive cultivars to Verticillium wilt. J Appl Sci Res. 8:2758–2765.
- FAOSTAT. 2021. Crops. [accessed 2021 Sept 20]. http://www.fao.org/faostat/en/#data/QC.
- Fradin EF, Thomma BPHJ. 2006. Physiology and molecular aspects of Verticillium wilt diseases caused by V. dahliae and V. albo-atrum. Mol Plant Pathol. 7(2):71–86. doi:10.1111/j.1364-3703.2006.00323.x.
- Fradin EF, Zhang Z, Juarez Ayala JC, Castroverde CD, Nazar RN, Robb J, Thomma BP. 2009. Genetic dissection of Verticillium wilt resistance mediated by tomato Ve1. Plant Physiol. 150(1):320–332. doi:10.1104/pp.109.136762.
- Geetha K, Rajithasri AB, Bhadraiah B. 2014. Isolation of plant growth promoting rhizobacteria from rhizosphere soils of green gram, biochemical characterization and screening for antifungal activity against pathogenic fungi. J Pharm Sci Invent. 3:47–54.
- Gilardi G, Demarchi S, Gullino ML, Garibaldi A. 2015. Nursery treatments with non-conventional products against crown and root rot, caused by Phytophthora capsici, on zucchini. Phytoparasitica. 43(4):501–508. doi:10.1007/s12600-015-0461-6.
- Gong S, Wang C, Jiang P, Hu L, Lei H, Chen Q. 2018. Designing highly efficient dual-metal single-atom electrocatalysts for the oxygen reduction reaction inspired by biological enzyme systems. J Mater Chem A. 6(27):13254–13262. doi:10.1039/c8ta04564j.
- González-Hernández AI, Suárez-Fernández MB, Pérez-Sánchez R, Gómez-Sánchez MÁ, Morales-Corts MR. 2021. Compost tea induces growth and resistance against Rhizoctonia solani and Phytophthora capsici in pepper. Agronomy. 11(4):781. doi:10.3390/agronomy11040781.
- Goswami D, Thakker JN, Dhandhukia PC. 2016. Portraying mechanics of plant growth promoting rhizobacteria (PGPR): a review. Cogent Food Agric. 2:1–19. doi:10.1080/23311932.2015.1127500.
- Gu Q, Yang Y, Yuan Q, Shi G, Wu L, Lou Z, Huo R, Wu H, Borriss R, Gao X. 2017. Bacillomycin D produced by Bacillus amyloliquefaciens is involved in the antagonistic interaction with the plant-pathogenic fungus Fusarium graminearum. Appl Environ Microbiol. 83(19):e01075–17. doi:10.1128/aem.01075-17.
- Gupta DK, Palma JM, Corpas FJ. 2015. Reactive oxygen species and oxidative damage in plants under stress. Heidelberg (Germany): Springer.
- Hacquard S, Spaepen S, Garrido-Oter R, Schulze-Lefert P. 2017. Interplay between innate immunity and the plant microbiota. Annu Rev Phytopathol. 55(1):565–589. doi:10.1146/annurev-phyto-080516-035623.
- Haldar S, Sanghamitra S. 2015. Plant-microbe cross-talk in the rhizosphere: insight and biotechnological potential. Open Microbiol J. 9(1):1–7. doi:10.2174/1874285801509010001.
- Hasanuzzaman M, Bhuyan MHMB, Parvin K, Bhuiyan TF, Anee TI, Nahar K, Hossen MS, Zulfiqar F, Alam MM, Fujita M. 2020a. Regulation of ROS metabolism in plants under environmental stress: a review of recent experimental evidence. Int J Mol Sci. 21(22):8695. doi:10.3390/ijms21228695.
- Hasanuzzaman M, Bhuyan MHMB, Zulfiqar F, Raza A, Mohsin SM, Mahmud JA, Fujita M, Fotopoulos V. 2020b. Reactive oxygen species and antioxidant defense in plants under abiotic stress: revisiting the crucial role of a universal defense regulator. Antioxidants. 9(8):681. doi:10.3390/antiox9080681.
- Helaly MN, El-Sheery NI, El-Hoseiny H, Rastogi A, Kalaji HM, Zabochnicka-Świątek M. 2018. Impact of treated wastewater and salicylic acid on physiological performance, malformation and yield of two Mango cultivars. Sci Hortic. 233:159–177. doi:10.1016/j.scienta.2018.01.001.
- Hilares RT, Dos Santos JG, Shiguematsu NB, Ajaz AM, da Silva SS, Dos Santos JC. 2019. Low-pressure homogenization of tomato juice using hydrodynamic cavitation technology: effects on physical properties and stability of bioactive compounds. Ultrason Sonochem. 54:192–197. doi:10.1016/j.ultsonch.2019.01.039.
- Hori K, Wada A, Shibuta T. 1997. Changes in phenoloxidase activities of the galls on leaves of Ulmus davidana formed by Tetraneura Fusiformis (Homoptera: Eriosomatidae). Appl Entomol Zool. 32(2):365–371. doi:10.1303/aez.32.365.
- Inderbitzin P, Subbarao KV. 2014. Verticillium systematics and evolution: how confusion impedes Verticillium wilt management and how to resolve it. Phytopathology. 104(6):564–574. doi:10.1094/phyto-11-13-0315-ia.
- Jha Y. 2019. The importance of zinc-mobilizing rhizosphere bacteria to the enhancement of physiology and growth parameters for Paddy under salt-stress conditions. Jordan J Biol Sci. 12:167–173.
- Jones JB, Zitter TA, Momol TM, Miller SA. 2016. Compendium of tomato diseases and ests. 2nd ed. St. Paul (MN): APS Press.
- Kaur T, Devi R, Kour D, Yadav A, Yadav AN, Dikilitas M, Abdel-Azeem AM, Ahluwalia AS, Saxena AK. 2021. Plant growth promoting soil microbiomes and their potential implications for agricultural and environmental sustainability. Biologia. 76(9):2687–2709. doi:10.1007/s11756-021-00806-w.
- Kawchuk LM, Hachey J, Lynch DR, Kulcsar F, Van Rooijen G, Waterer DR, Prüfer D. 2001. Tomato Ve disease resistance genes encode cell surface-like receptors. Proc Natl Acad Sci USA. 98(11):6511–6515. doi:10.1073/pnas.091114198.
- Khanna K, Jamwal VL, Sharma A, Gandhi SG, Ohri P, Bhardwaj R, Al-Huqail AA, Siddiqui MH, Ali HM, Ahmad P. 2019. Supplementation with plant growth promoting rhizobacteria (PGPR) alleviates Cadmium toxicity in Solanum lycopersicum by modulating the expression of secondary metabolites. Chemosphere. 230:628–639. doi:10.1016/j1.
- Kierul K, Borriss R, Chen X-H, Voigt B, Carvalhais LC, Albrecht D. 2015. Influence of root exudates on the extracellular proteome of the plant growth-promoting bacterium Bacillus amyloliquefaciens FZB42. Microbiology. 161(1):131–147. doi:10.1099/mic.0.083576-0.
- Kim JH, Castroverde CDM. 2020. Diversity, function and regulation of cell surface and intracellular immune receptors in Solanaceae. Plants. 9(4):434. doi:10.3390/plants9040434.
- Klimes A, Dobinson KF, Thomma BPHJ, Klosterman SJ. 2015. Genomics spurs rapid advances in our understanding of the biology of vascular wilt pathogens in the genus Verticillium. Annu Rev Phytopathol. 53(1):181–198. doi:10.1146/annurev-phyto-080614-120224.
- Klosterman SJ, Atallah ZK, Vallad GE, Subbarao KV. 2009. Diversity, pathogenicity, and management of Verticillium species. Annu Rev Phytopathol. 47(1):39–62. doi:10.1146/annurev-phyto-080508-081748.
- Köhl J, Kolnaar R, Ravensberg WJ. 2019. Mode of action of microbial biological control agents against plant diseases: relevance beyond efficacy. Front Plant Sci. 10:845. doi:10.3389/fpls.2019.00845.
- Koné SB, Dionne A, Tweddell RJ, Antoun H, Avis TJ. 2010. Suppressive effect of non-aerated compost teas on foliar fungal pathogens of tomato. Biol Control. 52(2):167–173. doi:10.1016/j.biocontrol.2009.10.
- Kowalska B. 2021. Management of the soil-borne fungal pathogen–Verticillium dahlia Kleb. causing vascular wilt diseases. Plant Pathol. 103(4):1185–1194. doi:10.1007/s42161-021-00937-8.
- Lanna-Filho R, Souza RM, Alves E. 2017. Induced resistance in tomato plants promoted by two endophytic bacilli against bacterial speck. Trop Plant Pathol. 42(2):96–108. doi:10.1007/s40858-017-0141-9.
- Lee BR, Kim KY, Jung WJ, Avice JC, Ourry A, Kim TH. 2007a. Peroxidases and lignification in relation to the intensity of water-deficit stress in white clover (Trifolium repens L.). J Exp Bot. 58(6):1271–1279. doi:10.1093/jxb/erl280.
- Lee VT, Matewish JM, Kessler JL, Hyodo M, Hayakawa Y, Lory S. 2007b. A cyclic-di-GMP receptor required for bacterial exopolysaccharide production. Mol Microbiol. 65(6):1474–1484. doi:10.1111/j.1365-2958.2007.05879.x.
- Lehmann J, Rillig MC, Thies J, Masiello CA, Hockaday WC, Crowley D. 2011. Biochar effects on soil biota – a review. Soil Biol Biochem. 43(9):1812–1836. doi:10.1016/j.soilbio.2011.04.022.
- Lian Q, Zhang J, Gan L, Ma Q, Zong Z, Wang Y. 2017. The biocontrol efficacy of Streptomyces pratensis LMM15 on Botrytis cinerea in tomato. Bio Med Res Int. 2017:1–11. doi:10.1155/2017/9486794.
- López-Moral A, Agustí-Brisach C, Trapero A. 2021. Plant biostimulants: new insights into the biological control of Verticillium Wilt of olive. Front Plant Sci. 12:782. doi:10.3389/fpls.2021.662178.
- Macho AP, Zipfel C. 2014. Plant PRRs and the activation of innate immune signalling. Mol Cell. 54(2):263–272. doi:10.1016/j.molcel.2014.03.028.
- Markakis EA, Fountoulakis MS, Daskalakis GC, Kokkinis M, Ligoxigakis EK. 2016. The suppressive effect of compost amendments on Fusarium oxysporum f.sp. radicis-cucumerinum in cucumber and Verticillium dahliae in eggplant. Crop Prot. 79:70–79. doi:10.1016/j.cropro.2015.10.015.
- Mauro RP, Agnello M, Distefano M, Sabatino L, San Bautista Primo A, Leonardi C, Giuffrida F. 2020. Chlorophyll fluorescence, photosynthesis and growth of tomato plants as affected by long-term oxygen root zone deprivation and grafting. Agronomy. 10(1):137. doi:10.3390/agronomy10010137.
- McKellar ME, Nelson EB. 2003. Compost-induced suppression of Pythium damping-off is mediated by fatty-acid-metabolizing seed-colonizing microbial communities. Appl Environ Microbiol. 69(1):452–460. doi:10.1128/AEM.69.1.452-460.2003.
- McNeece BT, Sharma K, Lawrence GW, Lawrence KS, Klink VP. 2019. The mitogen activated protein kinase (MAPK) gene family functions as a cohort during the Glycine max defense response to Heteroderaglycines. Plant Physiol Biochem. 137:25–41. doi:10.1016/j.plaphy.2019.01.018.
- Meena M, Swapnil P, Divyanshu K, Kumar S, Harish TYN, Zehra A, Marwal A, Upadhyay RS. 2020. PGPR-mediated induction of systemic resistance and physiochemical alterations in plants against the pathogens: current perspectives. J Basic Microbiol. 60(10):828–861. doi:10.1002/jobm.202000370.
- Mekonnen H, Kibret M. 2021. The roles of plant growth promoting rhizobacteria in sustainable vegetable production in Ethiopia. Chem Biol Technol Agric. 8(1):1–11. doi:10.1186/s40538-021-00213-y.
- Mhlongo MI, Piater LA, Madala NE, Labuschagne N, Dubery IA. 2018. The chemistry of plant–microbe interactions in the rhizosphere and the potential for metabolomics to reveal signaling related to defense priming and induced systemic resistance. Front Plant Sci. 9:112. doi:10.3389/fpls.2018.00112.
- Mishra S, Singh A, Keswani C, Saxena A, Sarma BK, Singh HB. 2015. Harnessing plant-microbe interactions for enhanced protection against phytopathogens. In: Arora NK, editor. Plant microbes symbiosis: applied facets. New Delhi (India): Springer; p. 111–125.
- Miya A, Albert P, Shinya T, Desaki Y, Ichimura K, Shirasu K, Narusaka Y, Kawakami N, Kaku H, Shibuya N. 2007. CERK1, a LysM receptor kinase, is essential for chitin elicitor signaling in Arabidopsis. Proc Natl Acad Sci. 104(49):19613–19618. doi:10.1073/pnas.0705147104.
- Murali Sankar P, Shreedevasena S, Kaviyarathinam T, Syamala M. 2021. A multi-trait mechanisms of PGPR in plant disease management. Biotica Research Today. 3(5):382–385.
- Nardi S, Schiavon M, Francioso O. 2021. Chemical structure and biological activity of humic substances define their role as plant growth promoters. Molecules. 26(8):2256. doi:10.3390/molecules26082256.
- Okatan V. 2020. Antioxidant properties and phenolic profile of the most widely appreciated cultivated berry species: a comparative study. Folia Hort. 32(1):79–85. doi:10.2478/fhort-2020-0008.
- Oleńska E, Małek W, Wójcik M, Swiecicka I, Thijs S, Vangronsveld J. 2020. Beneficial features of plant growth-promoting rhizobacteria for improving plant growth and health in challenging conditions: a methodical review. Sci Total Environ. 140682. doi:10.1016/j.scitotenv.2020.140.
- On A, Wong F, Ko Q, Tweddell RJ, Antoun H, Avis TJ. 2015. Antifungal effects of compost tea microorganisms on tomato pathogens. Biol Control. 80:63–69. doi:10.1016/j.biocontrol.2014.09.017.
- Ouazzani CA, Chliyeh M, Mouria B, Dahmani J, Ouazzani Touhami A, Benkirane R, Achbani EH, Douira A. 2014. Vitro and in vivo effect of salinity on the antagonist potential of Trichoderma harzianum and sensitivity of tomato to Verticillium wilt. Int J Recent Sci Res. 5:780–791.
- Panno S, Davino S, Caruso AG, Bertacca S, Crnogorac A, Mandić A, Matić S. 2021. A review of the most common and economically important diseases that undermine the cultivation of tomato crop in the Mediterranean Basin. Agronomy. 11(11):2188. doi:10.3390/agronomy11112188.
- Pant AP, Radovich TJK, Hue NV, Paull RE. 2012. Biochemical properties of compost tea associated with compost quality and effects on pakchoi growth. Sci Hortic. 148:138–146. doi:10.1016/j.scienta.2012.09.019.
- Pascual I, Azcona I, Morales F, Aguirreolea J, Sánchez-Díaz M. 2009. Growth, yield and physiology of Verticillium-inoculated pepper plants treated with ATAD and composted sewage sludge. Plant Soil. 319(1–2):291–306. doi:10.1007/s11104-008-9870-3.
- Pastori PL, Figueiras RMC, Oster AH, Barbosa MG, Silveira MRSD, Paiva LGG. 2017. Postharvest quality of tomato fruits bagged with nonwoven fabric (TNT). Rev Colomb Cienc Hortícolas. 11(1):80–88. doi:10.17584/rcch.2017v11i1.5839.
- Pathania P, Rajta A, Singh PC, Bhatia R. 2020. Role of plant growth-promoting bacteria in sustainable agriculture. Biocatal Agric Biotechnol. 101842. doi:10.1016/j.bcab.2020.101842.
- Pieterse CM, Zamioudis C, Berendsen RL, Weller DM, Van Wees SC, Bakker PA. 2014. Induced systemic resistance by beneficial microbes. Annu Rev Phytopathol. 52(1):347–375. doi:10.1146/annurev-phyto-082712-102340.
- Prasad M, Srinivasan R, Chaudhary M, Choudhary M, Jat LK. 2019. Plant growth promoting rhizobacteria (PGPR) for sustainable agriculture. In: Singh AK, Kumar A, Singh PK, editors. PGPR amelioration in sustainable agriculture. Sawston, UK: Woodhead Publishing; p. 129–157.
- Raaijmakers JM, Mazzola M. 2012. Diversity and natural functions of antibiotics produced by beneficial and plant pathogenic bacteria. Annu Rev Phytopathol. 50(1):403–424. doi:10.1146/annurev-phyto-081211-172908.
- Rahi AA, Anjum MA, Iqbal Mirza J, Ahmad Ali S, Marfo TD, Fahad S, Datta R. 2021. Yield enhancement and better micronutrients uptake in tomato fruit through potassium humate combined with micronutrients mixture. Agriculture. 11(4):357. doi:10.3390/agriculture11040357.
- Raklami A, Bechtaoui N, Tahiri A, Anli M, Meddich A, Oufdou K. 2019. Use of rhizobacteria and mycorrhizae consortium in the open field as a strategy for improving crop nutrition, productivity and soil fertility. Front Microbiol. 10:1106. doi:10.3389/fmicb.2019.01106.
- Sadler G, Davis J, Dezman D. 1990. Rapid extraction of lycopene and β-carotene from reconstituted tomato paste and pink grapefruit homogenates. J Food Sci. 55(5):1460–1461. doi:10.1111/j.1365-2621.1990.tb03958.x.
- Sadras VO, Quiroz F, Echarte L, Escande A, Pereyra VR. 2000. Effect of Verticillium dahliae on photosynthesis, leaf expansion and senescence of field-grown sunflower. Ann Bot. 86(5):1007–1015. doi:10.1006/anbo.2000.1267.
- Salehi B, Sharifi-Rad R, Sharopov F, Namiesnik J, Roointan A, Kamle M, Kumar P, Martins N, Sharifi-Rad J. 2019. Beneficial effects and potential risks of tomatoes consumption for human health: an overview. Nutrition. 62:201–208. doi:10.1016/j.nut.2019.01.012.
- Samet M, Charfeddine M, Kamoun L, Nouri-Ellouze O, Gargouri-Bouzid R. 2018. Effect of compost tea containing phosphogypsum on potato plant growth and protection against Fusarium solani infection. Environ Sci Pollut Res. 25(19):18921–18937. doi:10.1007/s11356-018-1960-z.
- Schwyn B, Neilands JB. 1987. Universal chemical assay for the detection and determination of siderophores. Anal Biochem. 160(1):47–56. doi:10.1016/0003-2697(87)90612-9.
- Shafique HA, Sultana V, Ara J, Ehteshamul-Haque S, Athar M. 2015. Role of antagonistic microorganisms and organic amendment in stimulating the defense system of okra against root rotting fungi. Polish J Microbiol. 64(2):157–162. doi:10.33073/pjm-2015-023.
- Shen Z, Zhong S, Wang Y, Wang B, Mei X, Li R, Ruan Y, Shen Q. 2013. Induced soil microbial suppression of banana fusarium wilt disease using compost and biofertilizers to improve yield and quality. Eur J Soil Biol. 57:1–8. doi:10.1016/j.ejsobi.2013.03.006.
- Shen T, Lei Y, Pu X, Zhang S, Du Y. 2021. Identification and application of Streptomyces microflavus G33 in compost to suppress tomato bacterial wilt disease. Appl Soil Ecol. 157:103724. doi:10.1016/j.apsoil.2020.103724.
- Shi L, Du N, Yuan Y, Shu S, Sun J, Guo S. 2016. Vinegar residue compost as a growth substrate enhances cucumber resistance against the Fusarium wilt pathogen Fusarium oxysporum by regulating physiological and biochemical responses. Environ Sci Pollut Res. 23(18):18277–18287. doi:10.1007/s11356-016-6798-7.
- Singh JS, Strong PJ. 2016. Biologically derived fertilizer: a multifaceted bio-tool in methane mitigation. Ecotoxicol Environ Saf. 124:267–276. doi:10.1016/j.ecoenv.2015.10.018.
- SinghD, Ghosh P, Kumar J, Kumar A. 2019. Rhizosphere, microbiome and agroecology. In: SinghD, Gupta V, Prabha R, editors. Microbia interventions in agriculture and environment. Singapore: Springer Nature; p. 205–227.
- Sreenivasulu R, Reddy MSP, Tomar D, Sanjay MSS, Reddy BB. 2019. Managing of early blight of tomato caused by Alternaria solani through fungicides and bioagents. Int J Curr Microbiol. App Sci. 8(6):1442–1452. doi:10.20546/ijcmas.2019.806.175.
- Tabli N, Rai A, Bensidhoum L, Palmieri G, Gogliettino M, Cocca E, Consiglio C, Cillo F, Bubici G, Nabti E. 2018. Plant growth promoting and inducible antifungal activities of irrigation well water-bacteria. Biol Control. 117:78–86. doi:10.1016/j.biocontrol.2017.10.010.
- Talaat NB. 2019. Role of reactive oxygen species signaling in plant growth and development. In: Hasanuzzaman M, Fotopoulos V, Nahar K, Fujita M, editors. Reactive oxygen nitrogen and sulfur species in plants: production, metabolism, signaling and defense mechanisms. Hoboken (NJ): John Wiley & Sons Ltd; p. 225–266.
- Tang F, Fu YY, Ye JR. 2015. The effect of methyl salicylate on the induction of direct and indirect plant defense mechanisms in poplar (Populus euramericana “Nanlin 895”). Plant Interact. 10(1):93–100. doi:10.1080/17429145.2015.1020024.
- Tejera García NA, Olivera M, Iribarne C, Lluch C. 2004. Partial purification and characterization of a non-specific acid phosphatase in leaves and root nodules of Phaseolus vulgaris. Plant Physiol Biochem. 42(7–8):585–591. doi:10.1016/j.plaphy.2004.04.004.
- Tigist M, Workneh TS, Woldetsadik K. 2011. Effects of variety on the quality of tomato stored under ambient conditions. J Food Sci Technol. 50(3):477–486. doi:10.1007/s13197-011-0378-0.
- Tubeileh AM, Stephenson GT. 2020. Soil amendment by composted plant wastes reduces the Verticillium dahliae abundance and changes soil chemical properties in a bell pepper cropping system. Curr Plant Biol. 22:100148. doi:10.1016/j.cpb.2020.100148.
- Ullah S, Ashraf M, Asghar HN, Iqbal Z, Ali R. 2019. Plant growth promoting rhizobacteria mediated amelioration of drought in crop plants: a review. Plant Soil Environ. 38(1):1–20. doi:10.25252/SE/19/71760.
- Vallad GE, Subbarao KV. 2008. Colonization of resistant and susceptible lettuce cultivars by a green fluorescent protein-tagged isolate of Verticillium dahliae. Phytopathology. 98(8):871–885. doi:10.1094/phyto-98-8-0871.
- Van Ooijen G, van den Burg HA, Cornelissen BJ, Takken FL. 2007. Structure and function of resistance proteins in solanaceous plants. Annu Rev Phytopathol. 45:43–72. doi:10.1146/annurev.phyto.45.062806.094430.
- Velikova V, Yordanov I, Edreva A 2000. Oxidative stress and some antioxidant systems in acid rain-treated bean plants: Protective role of exogenous polyamines. Plant Sci. 151:59–66. doi:10.1016/S01689452(99)00197-1.
- Vitullo D, Altieri R, Esposito A, Nigro F, Ferrara M, Alfano G, Ranalli G, De Cicco V, Lima G. 2013. Suppressive biomasses and antagonist bacteria for an eco-compatible control of Verticilliumdahliae on nursery-grown olive plants. International Journal of Environmental Science and Technology. 10(2):209–220. doi:10.1007/s13762-012-0145-4.
- Waqas M, Khan AL, Hamayun M, Shahzad R, Kim YH, Choi KS, Lee IJ. 2015. Endophytic infection alleviates biotic stress in sunflower through regulation of defence hormones, antioxidants and functional amino acids. Eur J Plant Pathol. 141(4):803–824. doi:10.1007/s10658-014-0581-8.
- Wu X, Song X, Qiu Z, He Y. 2016. Mapping of TBARS distribution in frozen–thawed pork using NIR hyperspectral imaging. Meat Sci. 113:92–96. doi:10.1016/j.meatsci.2015.11.008.
- Xie X, He Z, Chen N, Tang Z, Wang Q, Cai Y. 2019. The roles of environmental factors in regulation of oxidative stress in plant. Biomed Res Int. 2019:1–11. doi:10.1155/2019/9732325.
- Xiong W, Guo S, Jousset A, Zhao Q, Wu H, Li R, Kowalchuk GA, Shen Q. 2017. Bio-fertilizer application induces soil suppressiveness against Fusarium wilt disease by reshaping the soil microbiome. Soil Biol Biochem. 114:238–247. doi:10.1016/j.soilbio.2017.07.016.
- Yogev A, Raviv M, Hadar Y, Cohen R, Wolf S, Gil L, Katan J. 2010. Induced resistance as a putative component of compost suppressiveness. Biol Control. 54(1):46–51. doi:10.1016/j.biocontrol.2010.03.004.
- Yu YY, Li SM, Qiu JP, Li JG, Luo YM, Guo JH. 2019. Combination of agricultural waste compost and biofertilizer improves yield and enhances the sustainability of a pepper field. J Plant Nutr Soil Sci. 182(4):560–569. doi:10.1002/jpln.201800223.
- Zarco-Tejada PJ, Poblete T, Camino C, Gonzalez-Dugo V, Calderon R, Hornero A, Hernandez-Clemente R, Román-Écija M, Velasco-Amo MP, Landa BB, et al. 2021. Divergent abiotic spectral pathways unravel pathogen stress signals across species. Nat Commun. 12(1):1–11. doi:10.1038/s41467-021-26335-3.
- Zhang A. 2006. Mitogen-activated protein kinase is involved in abscisic acid-induced antioxidant defense and acts downstream of reactive oxygen species production in leaves of maize plants. J Plant Physiol. 141(2):475–487. doi:10.1104/pp.105.075416.
- Zhang Q, Gao X, Ren Y, Ding X, Qiu J, Li N, Chu Z. 2018. Improvement of Verticillium wilt resistance by applying arbuscular mycorrhizal fungi to a cotton variety with high symbiotic efficiency under field conditions. Int J Mol Sci. 19(1):241. doi:10.3390/ijms19010241.