Abstract
Background: Acute ingestion of ketone supplements alters metabolism and potentially exercise performance. No studies to date have evaluated the impact of co-ingestion of ketone salts with caffeine and amino acids on high intensity exercise performance, and no data exists in Keto-Adapted individuals.
Methods: We tested the performance and metabolic effects of a pre-workout supplement containing beta-hydroxybutyrate (BHB) salts, caffeine, and amino acids (KCA) in recreationally-active adults habitually consuming a mixed diet (Keto-Naïve; n = 12) or a ketogenic diet (Keto-Adapted; n = 12). In a randomized and balanced manner, subjects consumed either the KCA consisting of ∼7 g BHB (72% R-BHB and 28% S-BHB) with ∼100 mg of caffeine, and amino acids (leucine and taurine) or Water (control condition) 15 minutes prior to performing a staged cycle ergometer time to exhaustion test followed immediately by a 30 second Wingate test.
Results: Circulating total BHB concentrations increased rapidly after KCA ingestion in KN (154 to 732 μM) and KA (848 to 1,973 μM) subjects and stayed elevated throughout recovery in both groups. Plasma S-BHB increased >20-fold 15 minutes after KCA ingestion in both groups and remained elevated throughout recovery. Compared to Water, KCA ingestion increased time to exhaustion 8.3% in Keto-Naïve and 9.8% in Keto-Adapted subjects (P < 0.001). There was no difference in power output during the Wingate test between trials. Peak lactate immediately after exercise was higher after KCA (∼14.9 vs 12.7 mM).
Conclusion: These results indicate that pre-exercise ingestion of a moderate dose of R- and S-BHB salts combined with caffeine, leucine and taurine improves high-intensity exercise performance to a similar extent in both Keto-Adapted and Keto-Naïve individuals.
Introduction
Beta-hydroxybutyrate (BHB) is the primary circulating ketone body that serves as both an alternative fuel to glucose and a metabolic signal capable of eliciting epigenetic and inflammatory/immune-modulating effects (Citation1). In the context of traditional high-carbohydrate diets recommended for athletes that suppress fatty acid metabolism and ketogenesis (Citation2), resting concentrations of BHB are relatively low (<0.3 mM) and contribute minimally to fueling and signaling. Elevating circulating BHB concentrations to a range from >0.3 to 5.0 mM (i.e., nutritional ketosis) through either dietary carbohydrate restriction or by exogenous ketone supplementation is likely to result in increased use of ketones for fuel (Citation3), increased BHB signaling (Citation4), improved heart function (Citation5), and in the case of low-carbohydrate/ketogenic diets broad-spectrum therapeutic effects, notably reversal of pre-diabetes and type-2 diabetes (Citation6). Elevating circulating concentrations of BHB for the purposes of enhancing exercise performance is a relatively new concept that is increasingly being studied from the perspective of both ketogenic diets (Citation7) and exogenous ketones (Citation8).
The development of exogenous ketones over the last decade has resulted in various forms of BHB esters and salts. Whereas considerable work has been published on the safety, kinetics, and metabolic responses to ketone esters (Citation9, Citation10), only a handful of studies have assessed ketone salts. In respect to exercise performance, ketone salts have been shown to impair (Citation11) or have no effect (Citation12, Citation13) on high-intensity cycling. However, it is hard to glean much from these ketone salt studies given the relatively small sample size and limited disclosure of supplement contents and form of BHB. BHB is a chiral molecule with two enantiomers. Ketone salt products may be racemic mixtures of BHB or be enriched with R-BHB resulting in varying proportions of the enantiomers. Whereas R-BHB is the primary product of endogenous ketogenesis that participates in ATP production, S-BHB may retain the signaling functions attributed to BHB (Citation1). None of the published ketone salt studies validated the chirality of the BHB in the products (Citation11–14), with the exception of one metabolic study (Citation9) that showed ingestion of a racemic BHB mixture resulted in higher and sustained elevation of S-BHB over R-BHB indicating slower metabolism of the S-form (Citation1). Assessment of ketones by means of typical colorimetric and enzymatic techniques does not detect S-BHB so the actual degree of ketosis achieved by ketone salts in exercise performance studies (Citation11–13) remains unknown. Another potentially important factor that has not been examined in any study of exogenous ketones is whether the response varies depending on prior nutritional status. Specifically, individuals who have consumed a ketogenic diet for several months may have maximized their metabolic adaptations (e.g., increased fat oxidation, metabolic/signaling responses attributed to increased availability of ketones) thereby rendering them less responsive to exogenous ketone supplements.
To address these issues, in the current study we chose to examine the effects of a BHB-salt enriched with the R-enantiomer in both Keto-Naïve and Keto-Adapted individuals. The ketone salt supplement also included other potentially active ingredients including amino acids (leucine and taurine), and a modest dose of caffeine that could alone elicit an effect on performance as well as increase lipolysis and ketosis (Citation15). In addition to measuring high-intensity exercise performance, we examined circulating concentrations of R- and S-BHB, metabolic, and hormonal responses to exercise.
Methods
Experimental approach
A two-way crossover design was used to determine the effects of a pre-workout supplement consisting of ketone salts, caffeine, and amino acids (KCA) on exercise performance in a group of individuals habitually consuming a mixed non-ketogenic diet (Keto-Naïve) and a ketogenic diet (Keto-Adapted). Given the difficulties of creating a true placebo for a mixed ingredient supplement that masks the taste and stimulant effects of ketones and caffeine, we chose to simply compare the KCA response to a hydration-matched Water (control) condition. We provided participants with containers labeled ‘A’ or ‘B’ for purposes of blinding them to the appearance, but no attempt was made to make the drinks taste the same. Thus, the design was neither placebo-controlled nor double-blind. Prior to the two experimental trials, participants completed medical history, diet and physical activity questionnaires before signing an informed consent document approved by the Institutional Review Board of the Ohio State University (# 2016H0377). They completed a peak oxygen consumption test and were familiarized with the exercise protocol. The two experimental sessions were performed in a randomized and balanced order; each lasted ∼2 hr, and was separated by 2–7 days to accommodate variable schedules while allowing adequate recovery.
Subjects
Participants were between the ages of 18–50 years and recreationally active performing at least 150 minutes of moderate activity per week. Participants were excluded if they had metabolic or endocrine dysfunction, cardiovascular or respiratory diseases, gastrointestinal disorders, regularly smoked, consumed alcoholic beverages >3 drinks/day, epilepsy, chronic headaches or were pregnant. We enrolled a group of Keto-Naïve (n = 12) individuals who had never used ketone supplements or consumed a ketogenic diet. They were required to habitually consume a mixed diet consisting of moderate to high carbohydrate intake determined by interview and a food frequency questionnaire administered during the consent meeting. We also recruited a Keto-Adapted group (n = 12) who had been consistently consuming a very low-carbohydrate, high-fat, moderate-protein ketogenic diet for at least the past 3 months based on prior evidence an adaptation period is required in athletes consuming a ketogenic diet (Citation16). Keto-adaptation was validated by several measures including, 1) scrutiny of a food frequency questionnaire and food logs for a low carbohydrate content (<50 g/day), 2) a resting respiratory exchange ratio <0.75 indicating enhanced fat oxidation, which is typical in Keto-Adapted athletes (Citation16–18) and 3) fasting ketones >0.5 mM. There were no significant differences at baseline in height, weight, peak aerobic capacity, insulin resistance, or habitual caffeine intake between the Keto-Naïve and Keto-Adapted groups, but the Keto-Adapted group was older (mean ± SD; 36 ± 8 vs 23 ± 2 years) (.
Supplement
According to information provided by the manufacturer, the KCA supplement (KETO//OS MAX CHARGED, Pruvit Ventures, Melissa, TX) contained 8.5 g BHB salts consisting of 7.2 g BHB, 910 mg sodium, 245 mg magnesium, and 189 mg calcium. The supplement also contained 100 mg of caffeine (mean ± SD 1.3 ± 0.2 mg/kg), representing a typical dose in a serving of regular coffee that may mildly elevate ketosis (Citation15). The supplement label also listed L-leucine and L-taurine as ingredients. Total energy, mineral, amino acid and caffeine content were validated by an independent laboratory (Covance, Princeton, NJ) and showed small differences in minerals (all within 25%) and similar caffeine content (4% difference) (). In order to validate the BHB content of the KCA supplement, we developed a method to determine the relative amount of R-BHB and S-BHB using UPLC-MS/MS (details provided in Supplemental methods file). This permitted us to accurately determine the amount of R-BHB and S-BHB in the product. We analyzed three KCA packets and determined that on average one serving of the product contained 72% R-BHB and 28% S-BHB. The KCA supplement was mixed with 473 mL (16 oz) of water and additional flavoring including 4 g erythritol prior to ingestion. During the Water control condition, subjects consumed an equal volume of water with no flavoring to standardize hydration (Citation19).
Table 2. Nutrient content of the ketone-caffeine-amino acid supplement.
Peak oxygen consumption and familiarization
Participants arrived at the laboratory in the morning after at least 8 hr of fasting and no exercise, alcohol, or caffeine the prior day. Height and weight were recorded, and a urine sample was collected to determine urine specific gravity (Reichert TS 400 clinical refractometer); all subjects had values ≤1.025 indicating acceptable hydration status. A urine pregnancy test (Sure-Vue urine hCG strips) was completed in women. Participants were familiarized with the equipment and protocols including seat positions that were replicated for the experimental sessions. A peak oxygen consumption (VO2peak) test was performed on a stationary cycle ergometer (Lode Corival bicycle ergometer, Groningen, Netherlands) and metabolic cart (Parvo Medics TrueOne 2400 Metabolic Measurement System; Sandy, Utah). Participants were fitted with a nose-clip and headgear consisting of a mouthpiece attached to a hose (HANS RUDOLPH 2700 Series non-rebreathing; Shawnee, Kansas) linked with the two-way air chamber. After a 4 minutes warm-up with no resistance, a staged protocol began at 20 W that was increased 20 W every minute. The test was completed when the participants voluntarily stopped pedaling, which occurred within 18 minutes. VO2 and VCO2 gases were recorded every 15 seconds to calculate oxygen consumption and respiratory exchange ratio (RER) and averaged over the last 2 minutes of the stage. Peak VO2 and RER were determined by the highest 15-second interval value. The set resistance (W) during the stage of which the VO2peak occurred was used to calculate the loads for the progressive exercise protocol (i.e., 65, 70, 75, 80, 85, 90% of the wattage at VO2peak). After testing the participant was given a log to record all food and beverages consumed during the 24 hr prior to the upcoming experimental test session, which was scheduled between 2 and 7 days later.
Experimental sessions
Similar to the previous visit, subjects arrived at the laboratory in a fasted and hydrated state determined by urine specific gravity and provided a food log of the 24 hr prior to testing. Capillary blood BHB and glucose were recorded from a finger stick using a lancet with subsequent measurement on a handheld meter and appropriate strips (Precision Xtra Blood Glucose and Ketone Monitoring System, Abbott Nutrition, Columbus, Ohio). A blood draw from an arm vein was taken immediately following the finger stick for measurement of serum BHB, lactate, glycerol, and insulin. The KCA or Water was provided and ingested within 5 minutes. Another finger stick was taken 10 and 15 minutes after beverage consumption followed by another venous blood draw. An exercise protocol consisting of a staged time to exhaustion (TTE) test followed by a 30 second Wingate test was performed. The protocol began with a 4 minute warm-up, followed by 5 minute stages starting at an exercise resistance equal to 65% of the wattage achieved during the last completed stage of the VO2peak test. Each stage increased by 5% for a maximum of 6 stages (i.e., 90%). At the end of each stage perceived exertion was recorded using the 6–20 BORG scale (Citation20). Another finger stick was taken at the end of stage 3. Exhaustion was defined when the cadence fell below 60 revolutions per minute (RPM). The first time the participant fell below 60 RPM, a verbal warning was given and the participant was allowed 5 seconds to get the RPMs above 60. If the participant failed to achieve this cadence, or the second time the RPMs fell below 60, the test was ended. After exhaustion, the mouthpiece and headgear were removed and the participant was immediately moved to a different bike (Monark Ergomedic 894E bicycle ergometer, Vansbro, Sweden) to complete a Wingate test. When subjects reached a cadence of 120 RPM, a resistance equal to 0.075 kg/kg body mass was applied while subjects pedaled as hard as possible for 30 seconds. A finger stick and venous blood draw were obtained immediately after the Wingate test and participants were asked to rate their level of gastrointestinal (GI) distress from 0 (no distress) to 10 (the worst distress you have ever felt). The participant was then seated for 60 minutes to recover. Finger sticks were taken every 10 minutes throughout recovery and a venous blood draw taken at 30 and 60 minutes. Participants were given a copy of their completed food log to replicate the day before their scheduled second test day, which involved the exact same protocol except the other beverage was consumed. A schematic diagram of the experimental session is shown in .
Blood processing and analyses
A butterfly needle was inserted into the antecubital vein of the subject. Approximately 12 mL of blood was drawn at each time point into serum and EDTA tubes. Venous blood was centrifuged to obtain serum/plasma, and aliquoted into appropriately labeled microcentrifuge tubes. Samples reserved for lactate measurement were deproteinated using meta-phosphoric acid according to the assay kit recommended protocol prior to freezing and storage. All samples were snap frozen in liquid nitrogen and stored at -80 °C. Samples were thawed one time and analyzed in duplicate. Lactate was measured using a fluorometric kit (Cayman Chemical, Ann Arbor, MI) with a mean coefficient of variation (CV) of 10.1%. Serum insulin was analyzed by fluorometric enzyme-linked immunosorbent assay (ELISA) (Calbiotech, Spring Valley, CA) with a CV of 12.1%. Plasma glycerol was measured using a kit according to the manufacturer’s recommended protocol (Cayman Chemical, Ann Arbor, MI) with a CV of 6.4%. Fasting glucose and insulin values at baseline were used to calculate an index of insulin resistance [HOMA-IR; calculated as Glucose (mmol/L)·Insulin (µIU/mL)/22.5] (Citation21).
We developed a UPLC-MS/MS method to quantify R-BHB and S-BHB in the KS and from plasma samples using the same derivatization as Tsutsui et al (Citation22) and validated the plasma method according to the 2018 guidelines for industry from the FDA (Citation23). Details for the method can be found in the Supporting Information; details for the validation will be published elsewhere.
Statistics
Using the same TTE protocol, the coefficient of variation on repeated trials in 4 moderately-trained individuals was 1.2%, which translated to 9.6 seconds. We aimed to detect a two-fold greater increase, which translated to a sample size of 9 subjects per cohort assuming a mean difference of 20 seconds, SD of 13 seconds, 90% power and alpha level 0.05. To provide perspective on the practical relevance of the difference in TTE between trials, we estimated the smallest worthwhile difference (SWD) as 69 seconds (calculated as 0.2 multiplied by the SD of the best TTE of the two trials for 24 subjects). Independent t-tests were used to compare characteristics of Keto-Naive and Keto-Adapted groups. For primary performance data, a two-way analysis of variance (ANOVA) was used with group (Keto-Naïve and Keto-Adapted) as a between and supplement condition (KS and Water) as a within factor. Dependent t-tests were also performed on primary data based on sequence of trials independent of supplement. No significant effects were detected indicating lack of an order effect. For blood variables, a two-way repeated measures ANOVA with supplement condition and time (11 time points for ketones and glucose, and 5 time points for other metabolic/hormonal markers) as within factors was used. Fisher’s least significant difference post hoc was used to examine comparisons between conditions when significant main or interaction effects were present. Relations among outcome variables were determined by simple regression using Pearson correlation coefficients. Effect sizes (Cohen’s d) were calculated for main outcome variables and interpreted as small (0.2), medium (0.5) and large (0.8). The alpha level for significance was set at P ≤ 0.05.
Results
Gastrointestinal distress
The KCA was well tolerated. Self-reported gastrointestinal distress on a scale of zero to ten determined immediately post-exercise were not significantly different between KCA (KN: 1.2; KA:1) and water (KN: 2.9; KA: 0) conditions. There were three instances of vomiting post-exercise during the Water condition in Keto-Naïve subjects and only one instance of minor gastrointestinal stress after KS due to eructation of the product in a Keto-Adapted subject. In all cases, subjects reporting higher than a 0 completed the exercise trial and testing as scheduled.
Performance
Compared to Water, TTE was 9% longer (100 seconds) after KCA with no difference between groups. Twenty-one of twenty-four participants increased TTE after the KCA. The three who showed a decrease in performance (1 woman and 2 men) exhibited times that were <5% different between trials (). The magnitude of improvement after KCA exceeded the SWD in half of the subjects (6 of 12) for both groups (). Average and peak power over 30 seconds during the Wingate test were not different between trials ().
Figure 2. Individual time to exhaustion responses. Bars represent the difference in time to fatigue between the Ketone-Caffeine-Amino Acid (KCA) and Water trials. 10 of 12 Keto-Naïve and 11 of 12 Keto-Adapted participants cycled longer during the KCA trial. Trial (Citation2) x Group (Citation2) ANOVA indicated a significant (P < 0.001) effect of trial.
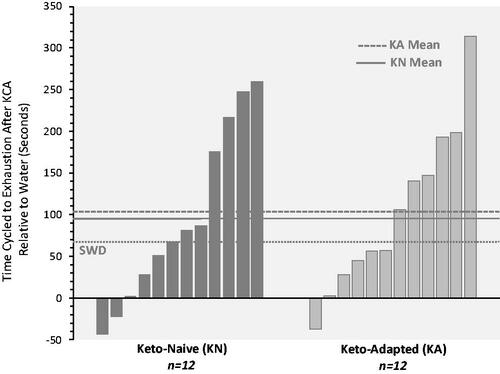
Table 3. Exercise performance responses.
Ketones
Resting capillary blood R-BHB concentrations in the Keto-Adapted group were 4-fold higher than Keto-Naïve subjects. After ingestion of the KCA, R-BHB concentrations were higher at all time points during and after exercise compared to Water (). The highest R-BHB concentrations occurred 15 minutes after KCA ingestion, with the net change from baseline two-fold higher in the Keto-Adapted (0.70 mM) versus the Keto-Naïve (0.33 mM) group. Capillary R-BHB returned to concentrations similar to baseline 20 minutes into recovery in Keto-Adapted subjects, whereas R-BHB remained significantly higher than baseline throughout 60 minutes of recovery in the Keto-Naïve group. In both groups, capillary R-BHB after Water ingestion decreased below baseline mid-exercise and stayed lower than pre-exercise concentrations throughout recovery. There were no significant correlations between ketone concentrations and performance.
Figure 3. Capillary blood R-beta-hydroxybutyrate (R-BHB) responses to ingestion of a Ketone-Caffeine-Amino Acid (KCA) supplement or Water in 12 Keto-Adapted (A) and 12 Keto-Naïve (B) subjects. Trial (Citation2) x Time (Citation11) ANOVA indicated time and time x trial interaction effects <0.000 for both groups. *P ≤ 0.05 from corresponding baseline value. #P ≤ 0.05 from corresponding Water time point. BL = baseline, MP = Exercise mid-point, IP = immediate post-exercise. Values are mean ± SE.
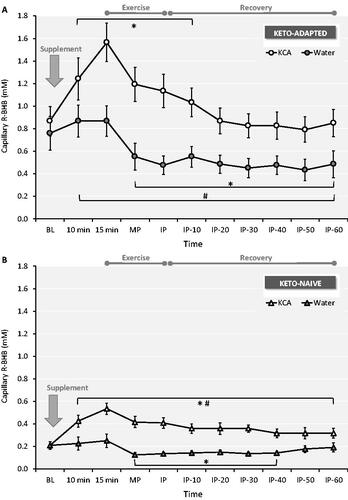
Because the KCA supplement contained both enantiomers of BHB and the device for measuring capillary ketones is specific for R-BHB, the true increase in circulating total BHB is underestimated by traditional ketone assay systems. The results of our enantiomer-specific plasma BHB analysis confirm that both R-BHB and S-BHB increased after KCA ingestion (). The plasma R-BHB response () was remarkably similar to the R-BHB results obtained in capillary blood, reflecting the high correlation between the two methods (r2=0.92). Fasting plasma S-BHB concentrations were more than 3-fold higher in the Keto-Adapted than the Keto-Naïve subjects (14 ± 6 vs 4 ± 3 μM; P < 0.001), and remained stable throughout exercise and recovery during the Water trial in both groups (). Fasting S-BHB concentrations in the Keto-Naïve group were below the lower limit of quantification (10 μM). Ingestion of KCA rapidly increased plasma S-BHB in both groups with concentrations peaking immediately post-exercise and staying elevated throughout 60 minutes of recovery. There was a trend for a more rapid increase in plasma S-BHB in the Keto-Adapted subjects with higher values 15 minutes after ingestion of KCA (trial x time interaction effect P = 0.07).
Figure 4. Plasma R-beta-hydroxybutyrate (R-BHB) and S-BHB responses to ingestion of a Ketone-Caffeine-Amino Acid (KCA) supplement or Water in 12 Keto-Adapted (A and C) and 12 Keto-Naïve (B and D) subjects. Trial (Citation2) x Time (Citation5) ANOVA indicated time and time x trial interaction effects <0.001 for all comparisons. *P ≤ 0.05 from corresponding baseline value. #P ≤ 0.05 from corresponding Water time point. BL = baseline, IP = immediate post-exercise. Values are mean ± SE.
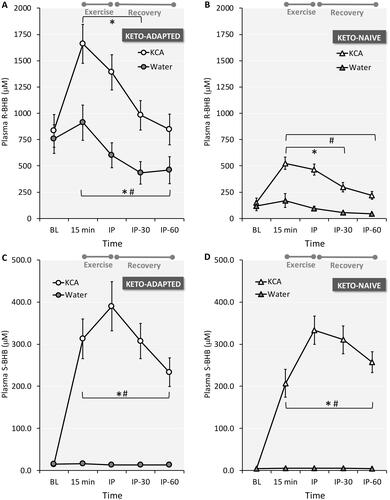
Metabolic and hormonal responses
In both groups and trials, capillary glucose peaked immediately post-exercise, gradually returning to baseline levels during recovery (). Plasma lactate peaked immediately after exercise and gradually returned toward resting levels after 60 minutes of recovery (). Peak lactate was higher after KCA ingestion in Keto-Naïve subjects, with a trend (Trial x time interaction effect P = 0.086) noted in Keto-Adapted subjects. Lactate concentrations were higher at several time points for Keto-Adapted versus Keto-Naïve subjects. Plasma glycerol peaked immediately after exercise and gradually returned toward resting levels after 60 minutes of recovery (). Peak glycerol was lower after KCA ingestion compared to Water in the Ketone-Naïve group. Post-exercise glycerol concentrations were higher in Keto-Adapted versus Keto-Naïve subjects. Plasma insulin remained stable in response to supplement ingestion and exercise in Keto-Adapted subjects (), whereas responses were more variable in Keto-Naïve subjects ().
Figure 5. Capillary blood glucose responses to ingestion of a Ketone-Caffeine-Amino Acid (KCA) supplement or Water in 12 Keto-Adapted (A) and 12 Keto-Naïve (B) subjects. Trial (Citation2) x Time (Citation11) ANOVA indicated a main effect of time (<0.001) for both groups. *P ≤ 0.05 from corresponding baseline value. BL = baseline, MP = Exercise mid-point, IP = immediate post-exercise. Values are mean ± SE.
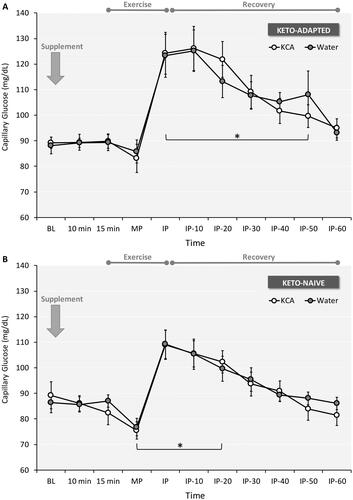
Figure 6. Plasma lactate (A and D), glycerol (B and D), and insulin (C and E) responses to ingestion of a Ketone-Caffeine-Amino Acid (KCA) supplement or Water in 12 Keto-Adapted and 12 Keto-Naïve subjects. Trial (Citation2) x Time (Citation5) ANOVA indicated time effects <0.001 for lactate and glycerol. For lactate, there were significant interaction effects in Keto-Naïve (P = 0.040) and a trend in Keto-Adapted (0.086) subjects. For glycerol, there was a significant interaction effect in Keto-Naïve (P = 0.005) subjects. *P ≤ 0.05 from corresponding baseline value. #P ≤ 0.05 from corresponding Water time point. BL = baseline, IP = immediate post-exercise. Values are mean ± SE.
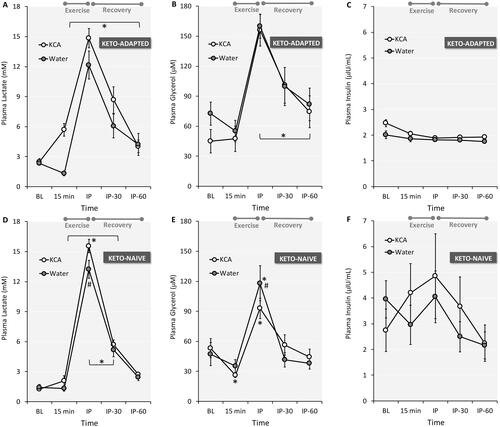
Oxygen consumption, RER, and RPE
Peak VO2 levels achieved during exercise were higher after KCA ingestion than water in Keto-Adapted but not Keto-Naïve subjects (). Substrate oxidation from indirect calorimetry showed lower RER in the Keto-Adapted group during stages 2 and 4, but there were no differences between trials at any stage (). The RPE scores increased steadily from stage 1–4 with significantly lower values for the KCA condition at stage 1 and a trend at stage 2 (). Stages 5 and 6 were not analyzed due to too few participants completing those stages.
Discussion
The primary circulating ketone R-BHB is both a metabolic fuel and signaling molecule whose full effects in humans is still under active investigation. Multiple lines of evidence suggest elevating BHB in the circulation via endogenous production from ketogenesis and/or exogenous ketones is associated with metabolic, therapeutic, and potentially performance-enhancing effects (Citation7, Citation10). In this investigation we tested the acute effects of ingesting 7.2 g BHB (72% R-BHB/28% S-BHB) in the salt form combined with a 100 mg dose of caffeine and the amino acids leucine and taurine versus a hydration-matched water control. Since KCA was a multi-ingredient supplement, we cannot isolate outcomes to any one component. We tested this KCA supplement in a group of recreationally active Keto-Naïve and Keto-Adapted individuals. The primary findings were that ingestion of the KCA supplement: 1) increased high-intensity performance by 9%, 2) resulted in rapid plasma increases and clearance of R-BHB, 3) resulted in rapid and sustained elevations of plasma S-BHB, 4) was well tolerated, and 5) altered metabolic responses to exercise as evidenced by increased peak lactate and decreased glycerol (Keto-Naïve only) responses. A unique aspect of this work that has not been performed in any other exogenous ketone study was inclusion of a captive group of Keto-Adapted individuals. We demonstrated that the consistent performance enhancement after KCA supplementation was evident in both the Keto-Naïve and Keto-Adapted participants, indicating a robust ergogenic effect irrespective of ketone concentration and duration of keto-adaptation.
The most salient finding was that compared to water, pre-workout ingestion of KCA during a progressive cycling test to exhaustion designed to produce fatigue in ∼20 minutes improved 8.3% in Keto-Naïve and 9.8% in Keto-Adapted individuals. All but 3 out of 24 individuals cycled longer after KCA than water, indicating a consistent ergogenic effect. Improved performance was irrespective of starting concentrations of ketones and prior adaptation to ketones as evidenced by similar improvements in both groups. The results stand in contrast to the three published exogenous ketone salt studies that that showed impaired (Citation11) or no impact (Citation12, Citation13) of ketone salts on high-intensity cycling. Several possible reasons may explain the disparity between our results and these other studies including use of a different BHB formulation that varied in mineral content and the addition of caffeine and amino acids.
The KCA supplement rapidly elevated circulating ketone concentrations in every participant, but the delta change (peak R-BHB minus baseline pre-ingestion values) varied widely from 0.2 to 1.5 mM. Even though Keto-Adapted individuals started with higher R-BHB concentrations (0.9 vs 0.2 mM), they had more than a two-fold greater increase in R-BHB after supplement ingestion (0.7 vs 0.3 mM). We used a standard dose of 7.2 g BHB as opposed to other studies that scaled it to body weight (Citation11, Citation14), but this unlikely explains differences in ketosis between people owing to the relatively homogenous weight status of our cohort. Rather, the individual BHB responses to ingestion of the KCA supplement underscore the high degree of variance among people in R-BHB absorptive capacity and/or clearance via renal loss or cellular/tissue uptake. The relatively small change in circulating R-BHB, especially in the Keto-Naïve group, who also started with lower BHB concentrations, suggests other aspects of the supplement may have contributed to improved exercise performance. However, before considering those elements it is important to note that the KCA supplement examined in this study contained approximately 2 g S-BHB, which is not detected in the blood using conventional assay methods and not measured in other ketone salt performance studies. Thus, the true level of ketosis would be underestimated unless S-BHB is specifically quantified.
Little is known regarding the function of S-BHB since R-BHB is the normal product of ketogenesis and the only form readily utilized to produce ATP owing to the chiral specificity of BHB dehydrogenase (Citation1). Nevertheless, we detected plasma S-BHB in all subjects in the fasted state, with concentrations more than 3-fold higher in Keto-Adapted subjects. In the fasted state plasma S-BHB was about 2–3% of total BHB (i.e., R-BHB + S-BHB) for both groups. S-BHB-CoA is a minor transient intermediate during beta-oxidation of fatty acids. Thus, the higher fasting S-BHB in Keto-Adapted subjects may indicate that some S-BHB escapes the mitochondria and enters the circulation as a result of increased fatty acid flux through beta-oxidation. It is noteworthy that in the Keto-Naïve group the increase in plasma S-BHB was similar in magnitude to R-BHB despite S-BHB being present in the KCA supplement at less than one half the R-BHB dose (i.e., 2.0 vs 5.2 g). The kinetics of plasma S-BHB were also different than R-BHB in that concentrations peaked slightly later than R-BHB and stayed elevated through 60 minutes of recovery, consistent with other findings (Citation9) that showed ingestion of a 50:50 racemic ketone salt (∼20 g) resulted in higher and more sustained elevation of plasma S-BHB compared to R-BHB. In our study, from 30 to 60 minute post-exercise, plasma S-BHB represented over one-half of total plasma BHB in the Keto-Naïve group and nearly one-fourth of total plasma BHB in the Keto-Adapted group, likely reflecting a delayed clearance of S-BHB. When considering just plasma R-BHB there were 3 of 12 subjects who failed to exceed 0.5 mM, but when considering total plasma BHB all subjects in the Keto-Naïve group exceeded 0.5 mM. The significance of the 28-fold (Keto-Adapted) to 78-fold (Keto-Naïve) increase in plasma S-BHB remains unknown. Animal studies indicate S-BHB may be a preferred substrate for sterol synthesis (Citation24, Citation25). S-BHB may also participate in signaling functions attributed to BHB as they do not appear to demonstrate chiral specificity (Citation1).
Ingestion of the KCA supplement did not alter the glycemic response to high-intensity exercise, indicating a net increase in availability of circulating energy substrates (BHB + glucose). Previous studies have shown that ketone salts have no significant impact (Citation12, Citation13) or a small transient glucose-lowering effect (Citation11, Citation14). Ingestion of higher doses of BHB esters (Citation10) or infusion of BHB (Citation26), both of which result in greater delta increases in circulating BHB, decrease circulating glucose. The lack of difference in the glucose response in this study may be attributed to the lower delta increase in circulating BHB and/or the glucose-enhancing effect of high-intensity exercise that may have dominated over the more subtle effect of ketosis on glucose flux. The KCA supplement also had minimal impact on plasma insulin, especially in the Keto-Adapted group with all subjects showing no evidence of any insulin response. The Keto-Naïve group included four individuals with a HOMA-IR over 1.0, whereas the highest value in the Keto-Adapted group was 0.7. These same four individuals demonstrated an exaggerated insulin response to ingestion of the KCA supplement that resulted in greater variability. Glycerol peaked immediately post-exercise in both conditions indicating accelerated adipose tissue lipolysis, but consistent with previous work (Citation10) the response was attenuated by the KCA supplement in the Keto-Naïve group only. Lipolysis is exquisitely sensitive to small changes in insulin, and therefore the slightly higher insulin may have inhibited release of glycerol from adipose tissue in the Keto-Naïve group. Ketones also directly inhibit the rate of glycerol appearance into the circulation (Citation26) by binding directly to receptors on adipocytes (Citation27), which may explain lower concentrations after KCA.
Immediate post-exercise lactate concentrations were ∼15 mM reflecting the high-intensity nature of the exercise protocol, with peak concentrations higher after KCA supplementation. Other ketone salt studies that involved high-intensity exercise showed no effect on lactate (Citation11, Citation12, Citation14). In fact, ketone ester supplementation resulted in significantly decreased lactate accumulation during a lower intensity bout of exercise, an effect attributed to a ketosis-induced inhibition on skeletal muscle glycolysis (Citation10). The slightly higher peak lactate in this study may be attributed to the greater cumulative work output after KCA supplementation as reflected by the fact that participants were able to cycle for a longer duration during the time trial. Also, caffeine ingestion (∼250 to 375 mg) has been shown in some studies to increase muscle and blood lactate concentrations during high-intensity exercise (Citation28), suggesting that the caffeine content of the KCA supplement may have contributed to the observed increase in lactate.
Although our aim was not to study a single nutrient or focus on mechanisms, it is worthwhile to consider plausible reasons for the ergogenic effect. The improvement in high-intensity performance after KCA ingestion could relate to direct or indirect effects of the BHB-salts, or the caffeine and amino acids present in the formula. Specific to the BHB salts, an ergogenic effect could have been mediated by enhanced substrate availability, BHB signaling (Citation1), improved efficiency (Citation29), and/or enhanced buffering. Regarding buffering, we did not measure blood pH but previous work has shown that ketone salts increase blood and urine pH due to dissociation of inorganic cations resulting in a BHB- conjugate base capable of buffering hydrogen ions (Citation9). Since fatigue during high-intensity exercise is well known to be partially mediated by accumulation of hydrogen ions, enhanced buffering after KCA seems plausible as a possible contributor to enhanced performance.
A large body of literature has shown that caffeine ingestion improves exercise performance through both central and peripheral mechanisms (Citation28, Citation30) although most of this work has involved moderate to high doses of caffeine (>3 mg/kg) well above the 100 mg dose (1.3 mg/kg) used in this study. However, as reviewed by Spriet (Citation31) there is some evidence that lower doses of caffeine (<3 mg/kg) may exert ergogenic effects, but the results are less consistent especially at the 100 mg dose used in the present study. For example, high intensity cycling performance was improved after ingesting 2 and 3 mg/kg caffeine, but not a 1 mg/kg dose (Citation32). Other studies also failed to show that caffeine at a dose <2 mg/kg before exercise improved cycling or running performance (Citation33, Citation34). We still believe that the caffeine in the KCA supplement partially contributed to the results, but likely through central as opposed to metabolic effects (Citation31, Citation35). The leucine and taurine in the KCA supplement may have also contributed to the observed increase in performance, especially taurine which according to our chemical analysis was provided at a dose of 2.75 g. Although studies are inconsistent, there is evidence from a meta-analysis that taurine supplementation ranging from 1 to 6 g/day can improve endurance performance (Citation36) and a single dose of just less than 4 g increased high-intensity exercise performance (Citation37).
The important issues of placebo effect and the fasting state of the control (Water) condition also warrant consideration. The placebo effect is a favorable outcome attributable only to the belief that one has received a beneficial treatment, or in the context of this experiment an improvement in exercise performance arising from ingesting the KCA supplement versus the comparison Water trial. Although we did blind participants by providing similar appearing drinks, they were aware of the treatments on the basis of the distinct flavor and taste of the KCA supplement. Specific studies addressing the placebo effect of caffeine on cycling performance indicate the performance benefit is on the order of 1–3% (Citation38). In a recent review of placebo effects associated with various nutritional ergogenic aids, Hurst et al (Citation39) conclude the overall effects are small to moderate on exercise performance (Cohen’s d = 0.35). When considering placebos most relevant to this study, it was reported in 8 studies of caffeine and 5 studies of fictitious sport supplements that Cohen’s d were 0.40 and 0.21, respectively (Citation39). Thus, it is possible or even likely that participants in our study exercised longer because of a belief the KCA supplement worked better than Water, but the magnitude of improvement after KCA supplementation (9%, Cohen’s d = 1.00) suggests that additional factors contributed to the ergogenic effect.
Practical application and limitations
The KCA supplement was well tolerated with no indications of severe distress as has been reported with ketone esters (Citation40, Citation41). Unexpectedly, three Keto-Naïve subjects vomited immediately after exercise during the Water trial. The most likely explanation is the acid stress of high-intensity exercise raising hydrogen ion levels (Citation42). Two of the participants vomited during the first experimental session and the other during the second session suggesting the GI stress cannot solely be accounted for by an adaptation to the specific exercise stress. Our results warrant further investigation into the metabolic and performance effects of ketone salts and caffeine, specifically studies that isolate individual effects of ketone salts with caffeine and potentially other nutrients (e.g., amino acids). Both trials were performed in the morning after an overnight fast, which may not represent an optimal state to perform the exercise protocol. It should be recognized that results obtained on a cycle ergometer in a laboratory setting may not translate to sporting events where enhanced endurance and power at the end of events is relevant. Future studies may examine more real-world performance outcomes relevant to athletes and consider fed versus fasted conditions. Further work is necessary to determine the active components, optimal dosages, underlying mechanisms and translational value for different types of athletes.
Conclusion
Compared to the comparison condition (water ingested after an overnight fast), ingestion of a moderate dose of ketone salts enriched in R-BHB with a relatively small dose of caffeine and amino acids was well tolerated and significantly extended time to fatigue during high-intensity cycling exercise. The performance benefit cannot solely be attributed to BHB or any individual nutrient in the KCA supplement. Improved performance was consistently observed in both a Keto-Naïve and Keto-Adapted cohort indicating a robust ergogenic effect irrespective of ketone levels and habituation to nutritional ketosis.
Abbreviations | ||
BHB | = | beta-hydroxybutyrate |
GI | = | gastrointestinal |
KA | = | Keto-Adapted |
KN | = | Keto-Naïve |
KCA | = | ketone salt, caffeine, and amino acids |
RPE | = | rate of perceived exertion |
RER | = | respiratory exchange ratio |
TTE | = | time to exhaustion |
Supplemental Material
Download PDF (134.4 KB)Disclosure statement
Dr. Volek has received royalties for books on ketogenic diets, he has served on scientific advisor boards for Virta Health, UCAN, Advancing Ketogenic Therapies, Axcess Global and Atkins Nutritionals, and is a founder and holds stock in Virta Health. Dr. Kraemer is a compensated consultant for the American College of Nutrition and a compensated member of the AdvoCare’s scientific and medical advisory board.
Table 1. Characteristics of groups at baseline.
Additional information
Funding
References
- Newman JC , Verdin E . β-Hydroxybutyrate: a signaling metabolite. Annu Rev Nutr. 2017;37(1):51–76. doi:10.1146/annurev-nutr-071816-064916.
- Thomas D , Erdman K , Burke L . American College of Sports Medicine Joint Position Statement. Nutrition and athletic performance. Med Sci Sports Exerc. 2016;48(3):543–68. doi:10.1249/MSS.0000000000000852.
- Cunnane S , Nugent S , Roy M , Courchesne-Loyer A , Croteau E , Tremblay S , Castellano A , Pifferi F , Bocti C , Paquet N , et al. Brain fuel metabolism, aging, and Alzheimer’s disease. Nutrition. 2011;27(1):3–20. doi:10.1016/j.nut.2010.07.021.
- Shimazu T , Hirschey MD , Newman J , He W , Shirakawa K , Le Moan N , Grueter CA , Lim H , Saunders LR , Stevens RD , et al. Suppression of oxidative stress and β-OHB as endogenous histone deactetylase. Science. 2013;339(6116):211–4. doi:10.1126/science.1227166.Suppression.
- Nielsen R , Møller N , Gormsen LC , Tolbod LP , Hansson NH , Sorensen J , Harms HJ , Frøkiaer J , Eiskjaer H , Jespersen NR , et al. Cardiovascular effects of treatment with the ketone body 3-hydroxybutyrate in chronic heart failure patients. Circulation. 2019;139(18):2129–41. doi:10.1161/CIRCULATIONAHA.118.036459.
- Athinarayanan SJ , Adams RN , Hallberg SJ , et al. Long-term effects of a novel continuous remote care intervention including nutritional ketosis for the management of type 2 diabetes: a 2-year nonrandomized clinical trial. Front Endocrinol (Lausanne). 2019;10:38. doi:10.3389/fendo.2019.00348.
- Volek JS , Noakes TD , Phinney SD . Rethinking fat as a performance fuel. Eur J Sport Sci. 2015;15(1):13–20. doi:10.1080/17461391.2014.959564.
- Evans M , Cogan KE , Egan B . Metabolism of ketone bodies during exercise and training: physiological basis for exogenous supplementation. J Physiol. 2017;595(9):2857–71. doi:10.1113/JP273185.
- Stubbs BJ, Cox PJ, Evans RD, Santer P, Miller JJ, Faull OK, Magor-Elliott S, Hiyama S, Stirling M, Clarke K. On the metabolism of exogenous ketones in humans. Front Physiol. 2017;8:1–13. doi:10.3389/fphys.2017.00848.
- Cox PJ , Clarke K . Acute nutritional ketosis: implications for exercise performance and metabolism. Extrem Physiol Med. 2014;3(1):1–9. doi:10.1186/2046-7648-3-17.
- O’Malley T , Myette-Cote E , Durrer C , Little JP . Nutritional ketone salts increase fat oxidation but impair high-intensity exercise performance in healthy adult males. Appl Physiol Nutr Metab. 2017;42(10):1031–5. doi:10.1139/apnm-2016-0641.
- Waldman HS , Basham SA , Price FG , Smith JW , Chander H , Knight AC , Krings BM , McAllister MJ . Exogenous ketone salts do not improve cognitive responses after a high-intensity exercise protocol in healthy college-aged males. Appl Physiol Nutr Metab. 2018;43(7):711–7. doi:10.1139/apnm-2017-0724.
- Rodger S , Plews D , Laursen P , Driller M . Oral β-hydroxybutyrate salt fails to improve 4-minute cycling performance following submaximal exercise. J Sci Cycl. 2017;6(1):26–31.
- Evans M , Patchett E , Nally R , Kearns R , Larney M , Egan B . Effect of acute ingestion of β-hydroxybutyrate salts on the response to graded exercise in trained cyclists. Eur J Sport Sci. 2018;18(3):376–86. doi:10.1080/17461391.2017.1421711.
- Vandenberghe C , St-Pierre V , Courchesne-Loyer A , Hennebelle M , Castellano CA , Cunnane SC . Caffeine intake increases plasma ketones: an acute metabolic study in humans. Can J Physiol Pharmacol. 2017;95(4):455–8. doi:10.1139/cjpp-2016-0338.
- Phinney SD , Bistrian BR , Evans WJ , Gervino E , Blackburn GL . The human metabolic response to chronic ketosis without caloric restriction: preservation of submaximal exercise capability with reduced carbohydrate oxidation. Metabolism. 1983;32(8):769–76. doi:10.1016/0026-0495(83)90106-3.
- Volek JS , Freidenreich DJ , Saenz C , Kunces LJ , Creighton BC , Bartley JM , Davitt PM , Munoz CX , Anderson JM , Maresh CM , et al. Metabolic characteristics of keto-adapted ultra-endurance runners. Metabolism. 2016;65(3):100–10. doi:10.1016/j.metabol.2015.10.028.
- Zajac A , Poprzecki S , Maszczyk A , Czuba M , Michalczyk M , Zydek G . The effects of a ketogenic diet on exercise metabolism and physical performance in off-road cyclists. Nutrients. 2014;6(7):2493–508. doi:10.3390/nu6072493.
- Riebl SK , Davy BM . The hydration equation: update on water balance and cognitive performance. ACSMs Health Fit J. 2013;17(6):21–8. doi:10.1249/FIT.0b013e3182a9570f. PMID: 25346594; PMCID: PMC4207053.
- Borg G . Perceived exertion as an indicator of somatic stress. Scand J Rehab Med. 1970;2:92–8.
- Matthews DR , Hosker JP , Rudenski AS , Naylor BA , Treacher DF , Turner RC . Homeostasis model assessment: insulin resistance and β-cell function from fasting plasma glucose and insulin concentrations in man. Diabetologia. 1985;28(7):412–9. doi:10.1007/BF00280883.
- Tsutsui H , Mochizuki T , Maeda T , Noge I , Kitagawa Y , Min JZ , Todoroki K , Inoue K , Toyo’oka T . Simultaneous determination of DL-lactic acid and DL-3-hydroxybutyric acid enantiomers in saliva of diabetes mellitus patients by high-throughput LC-ESI-MS/MS. Anal Bioanal Chem. 2012;404(6–7):1925–34. doi:10.1007/s00216-012-6320-0.
- Bioanalytical Method Validation - Guidance for Industry . Rockville, MD: U.S. Department of Health and Human Services, Food and Drug Administration, Center for Drug Evaluation and Research, Center for Veterinary Medicine; 2018 [cited 2020 April 9]. https://www.fda.gov/media/70858/download.
- Webber RJ , Edmond J . Utilization of L(+)-3-hydroxybutyrate, D(-)-3-hydroxybutyrate, acetoacetate, and glucose for respiration and lipid synthesis in the 18-day-old rat. J Biol Chem. 1977;252(15):5222–6.
- Lincoln BC , Des Rosiers C , Brunengraber H . Metabolism of S-3-hydroxybutyrate in the perfused rat liver. Arch Biochem Biophys. 1987;259(1):149–56. doi:10.1016/0003-9861(87)90480-2.
- Mikkelsen KH , Seifert T , Secher NH , Grøndal T , Van Hall G . Systemic, cerebral and skeletal muscle ketone body and energy metabolism during acute hyper-D-β-hydroxybutyratemia in post-absorptive healthy males. J Clin Endocrinol Metab. 2015;100(2):636–43. doi:10.1210/jc.2014-2608.
- Taggart AK , Kero J , Gan X , Cai TQ , Cheng K , Ippolito M , Ren N , Kaplan R , Wu K , Wu TJ , et al. (D)-beta-hydroxybutyrate inhibits adipocyte lipolysis via the nicotinic acid receptor PUMA-G. J Biol Chem. 2005;280(29):26649–52. doi:10.1074/jbc.C500213200.
- Doherty M , Smith PM . Effects of caffeine ingestion on rating of perceived exertion during and after exercise: a meta-analysis. Scand J Med Sci Sports. 2005;15(2):69–78. doi:10.1111/j.1600-0838.2005.00445.x.
- Sato K , Kashiwaya Y , Keon CA , Tsuchiya N , King MT , Radda GK , Chance B , Clarke K , Veech RL . Insulin, ketone bodies, and mitochondrial energy transduction. FASEB J. 1995;9(8):651–8. doi:10.1096/fasebj.9.8.7768357.
- McLellan TM , Caldwell JA , Lieberman HR . A review of caffeine’s effects on cognitive, physical and occupational performance. Neurosci Biobehav Rev. 2016;71:294–312. doi:10.1016/j.neubiorev.2016.09.001.
- Spriet LL . Exercise and sport performance with low doses of caffeine. Sports Med. 2014;44(S2):175–84. doi:10.1007/s40279-014-0257-8.
- Jenkins NT , Trilk JL , Singhal A , O’Connor PJ , Cureton KJ . Ergogenic effects of low doses of caffeine on cycling performance. Int J Sport Nutr Exerc Metab. 2008;18(3):328–42. doi:10.1123/ijsnem.18.3.328.
- Desbrow B , Barrett CM , Minahan CL , Grant GD , Leveritt MD . Caffeine, cycling performance, and exogenous CHO oxidation: a dose-response study. Med Sci Sports Exerc. 2009;41(9):1744–51. doi:10.1249/MSS.0b013e3181a16cf7.
- Lutsch DJ , Camic CL , Jagim AR , Johnston NJ , Musgjerd TL . Acute effects of a multi-ingredient pre-workout supplement on 5-km running performance in recreationally-trained athletes. Int J Exerc Sci. 2019;12(2):1045–56.
- Graham TE , Spriet LL . Metabolic, catecholamine, and exercise performance responses to various doses of caffeine. J Appl Physiol. 1995;78(3):867–74. doi:10.1152/jappl.1995.78.3.867.
- Waldron M , Patterson SD , Tallent J , Jeffries O . The effects of an oral taurine dose and supplementation period on endurance exercise performance in humans: a meta-analysis. Sports Med. 2018;48(5):1247–53. doi:10.1007/s40279-018-0896-2.
- Waldron M , Patterson SD , Jeffries O . Oral taurine improves critical power and severe-intensity exercise tolerance. Amino Acids. 2019;51(10–12):1433–41. doi:10.1007/s00726-019-02775-6.
- Beedie CJ , Stuart EM , Coleman DA , Foad AJ , Placebo effects of caffeine on cycling performance. Med Sci Sports Exerc. 2006;38(12):2159–64. doi:10.1249/01.mss.0000233805.56315.a9.
- Hurst P , Schipof-Godart L , Szabo A , Raglin J , Hettinga F , Roelands B , Lane A , Foad A , Coleman D , Beedie C . The Placebo and Nocebo effect on sports performance: a systematic review. Eur J Sport Sci. 2019;18(10):1–14. doi:10.1080/17461391.2019.1655098.
- Clarke K, Tchabanenko K, Pawlosky R, Carter E, Todd King M, Musa-Veloso K, Ho M, Roberts A, Robertson J, Vanitallie TB, & Veech, RL. Kinetics, safety and tolerability of (R)-3-hydroxybutyl (R)-3-hydroxybutyrate in healthy adult subjects. Regul Toxicol Pharmacol. 2012;63(3):401–408. doi:10.1016/j.yrtph.2012.04.008.
- Leckey JJ , Ross ML , Quod M , Hawley JA , Burke LM . Ketone diester ingestion impairs time-trial performance in professional cyclists. Front Physiol. 2017;8:1–10. doi:10.3389/fphys.2017.00806.
- Samborski P , Chmielarz‐Czarnocińska A , Grzymisławski M . 2013; Exercise‐induced vomiting. Prz Gastroenterol. (6)8:396–400. doi:10.5114/pg.2013.39924