Abstract
Objective:
LEAC-102 is an emerging drug extracted from the medicinal fungus Antrodia cinnamomea (AC), which is traditionally used to ameliorate fatigue and liver disorders arising from excessive alcohol consumption. AC has been used as a health product with an immunomodulatory function, but its anticancer effect has not been applied in clinical therapy as a drug. This first-in-human study examined the safety and tolerability of LEAC-102 as a new drug in healthy adults.
Method:
This standard 3 + 3 dose-escalation study included 18 participants administered LEAC-102 at doses of 597.6, 1195.2, 1792.8, 2390.4, or 2988 mg/day for 1 month plus 7 days of safety follow-up. The maximum planned dose was 2988 mg. Dose-limiting toxicity (DLT) was monitored from the start of LEAC-102 administration up to the final visit. The dose of LEAC-102 was escalated to the subsequent cohort as long as there was no DLT in the previous cohort. Tolerability, clinical status, safety (by laboratory parameters), and adverse event occurrence were documented weekly during the treatment and 1 week after the conclusion of the treatment.
Results:
All clinical biochemistry profiles were in the normal range, and no serious adverse effects were observed. The maximum tolerated dose of LEAC-102 was determined to be 2988 mg/day because one participant experienced urticaria. Additionally, our exploratory objectives revealed that LEAC-102 significantly elevated natural killer, natural killer T, and dendritic cells in a dose-dependent manner, activated effector T cells, and upregulated programmed cell death-1 expression.
Conclusions:
The outcomes suggested that LEAC-102 was well tolerated and safe in healthy adults and exhibited potential immunomodulatory function.
Supplemental data for this article is available online at https://doi.org/10.1080/07315724.2022.2032868 .
Introduction
Herbal medicines extracted from natural products have a uniquely diverse composition and comprise a variety of multidimensional chemical structures, leading to the multiplicity of their biological activities and druglike properties. Some natural products have been developed into emerging drugs for the treatment of human diseases and have had a profound impact on biomedicine (Citation1).
Antrodia cinnamomea (AC), a medical mushroom, is a unique edible fungus that grows on Cinnamomum kanehirai in Taiwan and comprises a complex mixture of biological compounds, including terpenoids, polysaccharides, maleic and succinic acid derivatives, benzoquinone derivatives, lignans, benzenoids, and glycoproteins (Citation2). AC is traditionally used by indigenous people to ameliorate fatigue and liver disorders arising from excessive alcohol consumption (Citation3). Gradually, the use of AC as a health food has increased due to its numerous biological activities including liver-protective, anti-inflammatory, antioxidant, antihypertensive (Citation4), cholesterol-lowering (Citation5), anticancer (Citation6), and immunomodulatory effects (Citation7).
The traditional use of AC indicates its protective effects in the liver. Many studies have shown that AC activates nuclear factor erythroid 2–related factor 2 and increases the expression of antioxidant genes to promote liver function and protect against hepatotoxicity in rats (Citation8, Citation9). AC also reverses carbon tetrachloride–induced liver fibrosis in rats by reducing serum glutamic oxaloacetic transaminase and serum glutamate pyruvate transaminase levels (Citation10).
Furthermore, AC also exhibits suppressive effects on tumorigenesis. AC extracts inhibited hepatocellular carcinoma cell survival by upregulating the expression of an inhibitor of nuclear factor kappa B (NF-κB) in the cytoplasm and decreasing the activity of NF-κB in the nucleus (Citation11). Further cancer-related AC research has emerged in recent years in addition to the studies of its role in hepatocellular carcinoma. Several in vitro and in vivo studies have revealed that AC attenuates tumor invasion and migration by inhibiting the self-renewal and proliferation capacities of cancer stem cells in head and neck squamous cell carcinoma and lung, breast, and colorectal cancer (Citation2, Citation12, Citation13).
Recently, the immunomodulatory effect of AC was found to be related to the inhibition of tumor progression. The purified polysaccharide component of AC indirectly blocks tumor progression by enhancing immunomodulatory capacities, promoting a Th1-dominant state and natural killer (NK) cell activity in an animal model (Citation14). A ubiquinone derivative isolated from AC was suggested to promote the antitumor immune response of immature dendritic cells toward liver cancer stem cells (Citation15). A case report also revealed that AC improved quality of life and immune function and reduced the occurrence of adverse effects associated with chemotherapy in patients with small cell lung cancer (Citation16).
Although AC has been demonstrated to be beneficial in cancer treatment as a synergistic therapy and promoter of immune system efficacy, the clinical safety data of AC are still insufficient. In particular, one challenge regarding the use of AC is that the tolerable dose in humans and the clinical effects remain unclear and controversial. Therefore, we conducted a phase I study to evaluate the physiological profiles of LEAC-102, a novel botanical drug extracted from AC, as a single agent in healthy human participants to determine the recommended dose for patients with cancer in the future. The primary objective of this trial was to determine the maximum tolerated dose (MTD) of LEAC-102 as a single agent based on the dose-limiting toxicity (DLT) observed in healthy volunteers. The secondary study objectives were to evaluate the safety and tolerability profiles of LEAC-102 alone in healthy volunteers and to perform immune assessment as the exploratory objective.
Material and methods
Study overview
This was an open-label, single-arm dose-escalation study of LEAC-102 to evaluate its safety and tolerability. To determine the MTD of LEAC-102, a single arm with traditional 3 + 3 dose-escalation (involving a total of 5 dose levels) was applied in this trial. This prospective study was approved by the ethics committee (ethical approval number: 201802057MSC) and the Institutional Review Board of the National Taiwan University Hospital (Protocol ID: LEAC-102-01), and all study methods were performed in accordance with the relevant guidelines and regulations. All study participants provided informed consent prior to participation in the investigation, and the study was supported by Taiwan Leader Biotech Corp. Ltd.
Participants
Eighteen healthy participants aged 20 to 44 years with a body mass index (BMI) of 18.5 to 24.0 kg/m2 and clinically normal hematology, biochemistry, and urinalysis determinations were included. All participants confirmed to be healthy by the investigator based on medical history, clinical examination, chest x-rays, and electrocardiogram (ECG) were eligible. Participants with a previous or current history of clinically significant allergy, hypersensitivity associated with AC, cardiac failure, autoimmune diseases, psychiatric disorders, HIV infection, or hepatitis B or C were excluded. Those using AC products within 1 month prior to the screening visit were also excluded from this trial. Female volunteers were not pregnant, lactating, or taking contraceptives at the time of recruitment.
Study design
The volunteers were divided into 5 cohorts (dose levels A, B, C, D, E) with up to 6 evaluable healthy volunteers per cohort for the assessment of the MTD. The A through E dose levels of LEAC-102 were 597.6, 1195.2, 1792.8, 2390.4, and 2988 mg/day, respectively. Volunteers who were administered LEAC-102 were sequentially enrolled to receive 1, 2, 3, 4, or 5 LEAC-102 capsules (199.2 mg/capsule) alone before a meal 3 times a day. At least 1 day elapsed between the beginning of LEAC-102 administration in the first 3 healthy volunteers of each cohort.
We arranged visits on days 1, 8, 15, and 22, which were scheduled as visits 1, 2, 3, and 4 for safety observation, and volunteers were required to remain on-site for at least 1 hour after taking LEAC-102. Visit 5 was scheduled on day 29 for checking status of the end of treatment (EOT) and conducted exploratory items.
The starting dose of LEAC-102 was determined based on the results of preclinical toxicity studies (Citation17) and no observed adverse effect level of LEAC-102 was determined as 1700 mg/kg/day in rats of both sexes, leading to human equivalent dose of 274.2 mg/kg/day. Based on these data, a starting dose of LEAC-102 with 597.6 mg/day was considered to be a safe starting dosage for humans.
DLT was defined as any ≥ grade 2 adverse event (AE) causally related to LEAC-102 administration as judged by the investigator according to the National Cancer Institute Common Terminology Criteria for Adverse Events (NCI CTCAE) version 5.0. DLT was monitored from the start of LEAC-102 administration up to visit 6 (final visit after 28 consecutive days of treatment plus 7 days of safety follow-up). The dose of LEAC-102 was escalated to the subsequent cohort as long as there was no DLT in the previous cohort. The MTD was defined as the highest dose level at which <2 of 6 healthy volunteers experienced DLT. Each cohort included up to 6 volunteers according to the 3 + 3 design. The MTD should be tested in 6 healthy volunteers. No volunteer could be assigned to more than one dose level. All dose escalation/de-escalation decisions were made by the safety monitoring committee.
Drug product
LEAC-102 was isolated from AC mycelia and fruit bodies using the extraction technology of the Taiwan Leader Biotech Corp. We analyzed the powdered LEAC-102 material by high-performance liquid chromatography (HPLC) to identify the major compounds (Supplementary material, ).
Measurements
For safety assessments, the incidence of AEs/serious adverse events and vital signs were recorded and physical examination assessments, biochemistry analyses, hematology analyses, urinalysis tests, and 12-lead ECGs were performed for this study. The determination of the MTD was based on the occurrence of DLT.
The changes in lymphocyte activities, innate immune cells, adaptive immune cells, bridge cells, regulatory cells, and cytokine levels were assessed as exploratory end points. In addition, the changes in serum levels of adrenocorticotropin (ACTH), cortisol, progesterone, testosterone, and estradiol (E2) as well as the volume of the adrenal gland as measured by ultrasound were evaluated as exploratory safety end points in this study.
Materials and reagents
Reagents and materials used in this research included Ficoll-Paque PREMIUM (GE Healthcare, Massachusetts, USA), staining buffer (homemade reagent), Pasteur pipettes, 1.5-mL Eppendorf tubes (Volac, Royston, UK), 15 mL and 50-mL plastic centrifuge and FACS™ tubes (Thermo Fisher Scientific, Corning, USA), Thermo Scientific Nunc serological pipettes (Thermo Fisher Scientific, Corning, USA), and 96-well plates (V bottom for cell counting) (Merck, Darmstadt, Germany).
Isolation of peripheral blood mononuclear cells (PBMCs)
Human PBMCs were isolated from buffy coats by the Ficoll density gradient centrifugation technique (Ficoll-Paque™ PREMIUM; GE Healthcare, USA). After centrifugation, PBMCs were washed with 1X PBS and resuspended in staining buffer (2% FBS + 0.02% NaN3 in 1× PBS) for cell surface marker staining or intracellular staining.
Staining and flow cytometry
PBMCs were prepared from the peripheral blood and stained for cell surface markers, including TCR-FITC, CD25-PE, CD45-ECD, PD-1-PE Cy5, CD4-PE Cy7, TCR-APC, CD25-AlexaFluor®488, CTLA-4-PE, CD33-FITC, CD39-PE, CD11c-APC, PD-1-AlexaFluor®488, PD-L1-PE, CD14-PE Cy5.5, and CD11c-APC. Data acquisition was performed using a Navios flow cytometer (Beckman Coulter) and analyzed by Kaluza software version 2.1 (Beckman Coulter).
Statistical analyses
Baseline characteristics are presented using descriptive statistics and displayed by dose level. Additionally, descriptive statistics are provided for all of the end points by dose level. Descriptive statistics, including the number of observations, mean, and standard deviation (SD), are presented for the raw data as well as changes from the baseline. The count and percentages were used to summarize the categorical data. Statistical analysis was performed using SAS for Windows software (Version 9.4, SAS Inc., USA). Paired Student’s t test was applied to analyze the raw numerical results. Unpaired Student’s t test was applied to analyze the percentage changes in the immune analysis.
AEs observed during the study were coded using the up-to-date version of the Medical Dictionary for Regulatory Activities (MedDRA) and were reported by dose level by system organ class and the preferred term classified in the MedDRA as appropriate. The toxicity grades of the AEs were rated according to the NCI CTCAE version 5.0. Net changes from pretreatment laboratory test results and vital signs were analyzed by descriptive statistics.
Results
Baseline characteristics
A standard 3 + 3 dose-escalation study was employed to evaluate the safety and tolerability of LEAC-102. A total of 18 healthy participants were recruited to receive the indicated dose of LEAC-102 (597.6, 1195.2, 1792.8, 2390.4, or 2988 mg/day). We also identified major compounds of LEAC-102 powder by HPLC, including 25S-Antcin H, 25 R-Antcin H, 25S-Antcin B, 25S-Antcin K, and 25 R-Antcin B (). The groups that received dose levels A to D (n = 3) and dose level E (n = 6) were Intention-to-treat (ITT)- and MTD-evaluable populations. Only one participant who received dose level E did not complete the entire trial due to DLT (). The age, body weight, and BMI of the participants were similar among the LEAC-102 dose levels ().
Figure 1. Major compounds of LEAC-102 powder. (A) 25R/S-Antcin B, (B) 25R/S-Antcin K, and (C) 25R/S-Antcin H were primary compounds of LEAC-102 powder and analyzed by high-performance liquid chromatography (HPLC).
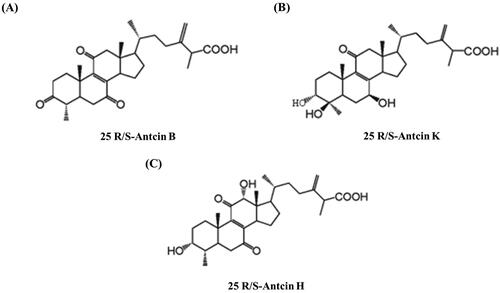
Table 1. Summary of Participant Disposition.
Table 2. Demographic Characteristics of Participants.
Safety
There were no significant changes in body weight, diastolic blood pressure, or pulse rate in any participant after LEAC-102 administration. Although systolic blood pressure was elevated at dose levels A and C (108.0 and 120.0 mm Hg) compared with baseline, no clinically relevant effects were noted (). The mean total bilirubin of all participants was significantly decreased at the end point, but the value was within the clinically normal range (). Biochemistry parameters, including renal function (), total cholesterol, triglycerides, C-reactive protein, fasting glucose, amylase, lipase (), and electrolyte profiles (), were normal according to standard clinical safety references. Hematological profiles () were within the normal range, except for eosinophils and basophils, which exhibited changes compared with the baseline levels; however, these differences were not clinically significant. Of all AEs observed, 2 were mild and 2 were moderate (data not shown) with headache and urticaria. No serious AEs occurred. Moreover, the AE incidences at all dose levels (A to E) were 0.0%, 33.3%, 33.3%, 0.0%, and 33.3%, with no dose-dependent increase in incidence associated with LEAC-102 administration ().
Table 3. Body Weight and Blood Pressure Profiles in Participants
Table 4. Plasma Liver Function Profiles in Participants
Table 5. Plasma Renal Function Profiles in Participants
Table 6. Specific Chemistry Profiles in Participants
Table 7. Electrolyte Profiles in Participants
Table 8. Hematology Profiles in Participants
Table 9. AEs in Participants Receiving the Dose of LEAC-102
Exploratory profiles
The exploratory safety end points, including ACTH, progesterone, testosterone, and E2 levels, were within the normal range according to standard clinical safety references. Only cortisol at dose level E changed from baseline (10.78 vs 8.17 µg/dL); however, this change was not clinically significant ().
Table 10. Specific Hormones in Participants
We focused on the possible immunomodulatory activities of LEAC-102 by characterizing the immune profiles of participants in the indicated cohorts (C to E), including protective immune effector cells and suppressive regulatory cells, before treatment and 1 month after LEAC-102 administration. All immune profiling analyses depicted the difference in each dose group in terms of the percentage change between baseline and EOT. The number of NK cells was significantly increased in a dose-dependent (level C to E) manner after LEAC-102 treatment (−26.0%, −1.6%, and 12.1%, respectively [p = 0.04]) (). Cohorts D and E also demonstrated a trend of monocyte (10.2%, 6.3%), natural killer T (NKT) cell (−2.8%, 17.9%), and dendritic cell (DC) (−13.1%, −1.9%) induction compared to that of cohort C (). Furthermore, increased levels of programmed cell death-1 (PD-1) expressed on naive CD4+ T cells (27.2%, 61.9%), naive CD8+ T cells (56.2%, 67.0%), and activated CD4+ T cells (103.1%, 235.6%) were noted upon administration of doses D and E of LEAC-102 ().
Figure 2. Distribution of immune cells during LEAC-102 treatment in participants at doses C to E. (A) B cells, (B) monocytes, (C) dendritic cells, (D) natural killer (NK) cells, (E) NKT cells, and (F) T cells in peripheral blood mononuclear cells (PBMCs) were quantified by flow cytometry using antibodies against specific epitopes. The percentages of the indicated cell subsets and statistical significance between groups were analyzed and calculated using Kazula and Prism (*p < 0.05, **p < 0.01, ***p < 0.001).
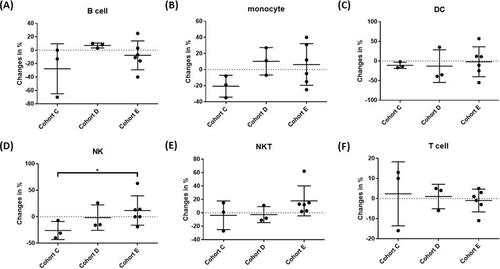
Figure 3. LEAC-102 upregulates programmed cell death-1 (PD-1) expression on CD4+ and CD8+ T cells in the peripheral blood. Participants were administered LEAC-102 at doses of C, D, or E (1792.8, 2390.4, or 2988 mg/day) for 1 month. (A) Naive PD-1+ CD4+ T cells, (B) activated PD-1+ CD4+ T cells, (C) naive PD-1+ CD8+ T cells, and (D) activated PD-1+ CD8+ T cells were quantified by flow cytometry using antibodies against specific epitopes. The percent changes in the indicated cell subsets and statistical significance between groups were analyzed and calculated by Kazula and Prism (*p < 0.05, **p < 0.01, ***p < 0.001).
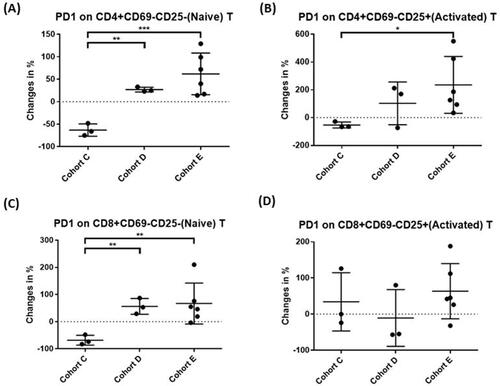
Discussion
This was a first-in-human study of LEAC-102, an emerging botanical drug extracted from the fruiting bodies and solid-state cultivated mycelia of AC. The results demonstrated that oral administration of LEAC-102 at dosages up to 2988 mg/day was well tolerated by healthy adults. A similar observation was made in a preliminary study, in which healthy adults ingested AC products at a dosage of 1440 mg daily for 3 months with normal clinical biochemistry profiles and no AEs (Citation5). A few changes were noted overall during our trial. The systolic blood pressure in dose groups A and C (equivalent to 597.6 and 1792.8 mg/day) was significantly higher than at baseline, but it was within the clinical normal range and was not dose-dependent. A pilot study also revealed that AC significantly reduced systolic blood pressure (from 144.86 to 133.10 mm Hg, p < 0.05) and diastolic blood pressure (from 96.19 to 91.38 mm Hg, p < 0.05) in mildly hypertensive patients who were administered AC for 2 months with no abnormal laboratory findings (Citation18). Furthermore, the changes in parameters, such as total bilirubin (from 0.85 to 0.67 mg/dL) in liver function assessments and lymphocytes (from 30.60% to 29.43%), eosinophils (from 2.20% to 4.60%), and basophils (from 0.70% to 0.87%) in hematological assessments, were also not clinically significant. This finding is consistent with the toxicological research results of AC administration to date, and even when used as a health food, AC can protect the liver and enhance immunity (Citation15, Citation19–21).
Although AC is regarded as a Chinese herbal medicine with biological effects, its clinical safety is still unclear. Thus, the therapeutic application of AC needs to be evaluated in formal and government-approved human clinical trials to determine its safety and tolerability. Several toxicological tests indicated that AC doses up to 4000 mg/kg body weight/day in rats (Citation22, Citation23) and 2500 mg/kg body weight/day in mice were safe and tolerable as no significant abnormalities were observed in body weight, organ weight gain, hematological parameters, and liver and renal function (Citation23, Citation24). In our study, we strictly monitored the effect of all LEAC-102 doses on renal function. Only in terms of adrenal function did the cortisol level at dose E increase to 10.78 µg/dL at the end point; however, this was not a clinically significant finding. The remaining parameters, including ultrasound of the adrenal gland (data not shown) and serum hormone and electrolyte profiling, all confirmed the safety of LEAC-102.
Single dosing with LEAC-102 up to 2988 mg/day has no serious adverse effects on healthy adults; however, one of the participants experienced urticaria and DLT. This participant was a 32-year-old man and was enrolled on September 4, 2019; no medical history was noted for this person at the screening. Then, the participant was assigned to cohort E from September 17 to 26, 2019. The participant experienced moderate urticaria on September 25, 2019, and the dosing of LEAC-102 was held from the evening on September 26, 2019. Without additional medication given to treat this event, this event was recovered on September 30, 2019, after stopping the dosing of LEAC-102. This participant was then withdrawn from the study on October 1, 2019, by the investigator. The dosing of LEAC-102 was interrupted and then withdrawn in this participant due to this event; this event was possibly related to LEAC-102 as judged by the investigator and was considered a DLT.
Therefore, the MTD in this study was determined to be dose level E, in which participants were administered 2988 mg/day of LEAC-102 for 28 consecutive days. A total of 4 AEs were observed in 4 participants in this study, including increased blood bilirubin, first-degree atrioventricular block, urticaria, and headache. All AEs were resolved, except for one in a participant who had a high bilirubin level at enrollment that appeared to decrease at the end of the study. However, the cause of preexisting high bilirubin in this participant was not clear.
To investigate the potential immunomodulatory effects of LEAC-102, we used epitope-specific antibodies and flow cytometric analysis to identify immune cell subsets in the peripheral blood. Immune analysis revealed that LEAC-102 dramatically promoted the induction of NK cells, NKT cells, and DCs in a dose-dependent manner. Previous studies have shown that AC enhances NK cell cytolytic activity and macrophage phagocytic activity and increases splenic lymphocyte proliferation by activating the c-JUN N-terminal kinase, p38, and extracellular signal-regulated kinase signaling pathways (Citation25–27). Another study revealed that polysaccharides extracted from AC play a crucial role in the activation of DCs, critical leukocytes for initiating immune responses, in mice (Citation28). AC treatment also increased the capacity of activated DCs to suppress allergen-specific type 2 T helper cell polarization in an allogeneic mixed lymphocyte reaction (Citation29). Moreover, our study shows for the first time that LEAC-102 promotes CD4+ T and CD8+ T cell activation accompanied by the upregulation of PD-1 expression by CD4+ and +CD8+ T cells. Immune checkpoint inhibitors targeting PD-1 have been widely investigated, and PD-1+CD4+ and PD-1+CD8+ effector cells play a crucial role in advanced cancer therapy (Citation30, Citation31). Thus, taking LEAC-102 increases naive CD4+ T cells and activates effector CD4+ T cells, then stimulates naive CD8+ T cells, meaning that LEAC-102 may have an education effect on immune system () and exhibits multiple effects important for adjuvant cancer treatment.
Figure 4. A potential effect on immune regulation during LEAC-102 treatment in healthy participants. LEAC-102 increases naive CD4+ T cells, effector CD4+ T cells, and naive CD8+ T cells, then effector CD4+ T cells also stimulate naive CD8+ T cells. Moreover, CD4+ and CD8+ T cell activation is accompanied by the upregulation of programmed cell death-1 (PD-1) expression by CD4+ and CD8+ T cells, both indicating that taking LEAC-102 may have an education effect on the immune system in healthy participants.
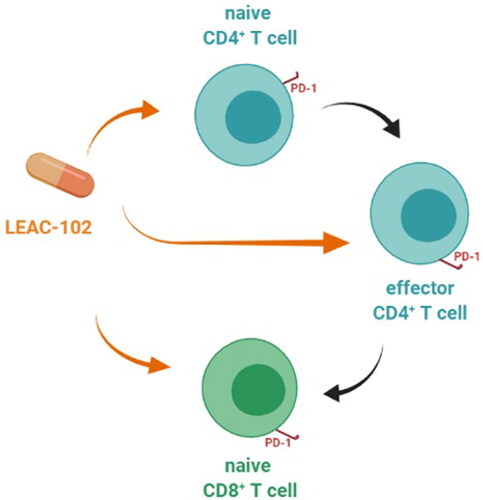
Although this study provides important data on the clinical safety and potential immune efficacy profile of LEAC-102, there are still some limitations. All data were obtained from healthy participants who only received an oral dose of up to 2988 mg/day, which limits the generalizability of findings. Moreover, the small sample size with the 3 + 3 dose-escalated design may limit the evaluation of immunomodulatory potential.
The purpose of this study was to evaluate the tolerability of LEAC-102 in healthy participants and observe DLT. If LEAC-102 is to be used in cancer adjuvant applications, further research is needed on the safety, tolerability, pharmacokinetics, pharmacodynamics, and efficacy of LEAC-102 in patients with cancer.
Conclusions
This first-in-human phase I study of LEAC-102 demonstrated an acceptable safety and tolerability profile at doses up to 2988 mg/day with no serious adverse effects in healthy adults. Our results suggested that LEAC-102 may exhibit novel immunomodulatory activities by promoting adaptive T-cell activation and simultaneously upregulating PD-1 expression in a dose-dependent manner. Based on these findings, LEAC-102 is expected to be applicable for cancer treatment and has the potential to shed light on mechanisms targetable by future immunotherapies.
Role of the funding source
The funding source had no involvement in the study design; data collection, analysis, or interpretation; writing of the report; or the decision to submit the article for publication. The role of the funding source is the provision of test reagents and funds for the clinical trial.
Authors’ contributions
Conception and design: Kai-Wen Huang.
Development of methodology: Tzung-Hsien Lai.
Acquisition of data (acquired and managed patients, provided facilities, etc.): Yu-Tso Liao.
Analysis and interpretation of data (e.g., statistical analysis, biostatistics, computational analysis): Tzung-Hsien Lai, Wan-Jing Chen.
Writing, review, and/or revision of the manuscript: Wan-Jing Chen, Yu-Tso Liao, Kai-Wen Huang.
Administrative, technical, or material support (i.e., reporting or organizing data, constructing databases): Yu-Tso Liao.
Study supervision: Kai-Wen Huang.
Abbreviations | ||
AC | = | Antrodia cinnamomea |
ACTH | = | adrenocorticotropin |
AE | = | adverse event |
BMI | = | body mass index |
DC | = | dendritic cell |
DLT | = | dose-limiting toxicity |
E2 | = | estradiol |
ECG | = | electrocardiogram |
EOT | = | end of treatment |
HPLC | = | high-performance liquid chromatography |
MTD | = | maximum tolerated dose |
NF-κB | = | nuclear factor kappa B |
NK | = | natural killer |
PBMC | = | peripheral blood mononuclear cells |
PD-1 | = | programmed cell death-1 |
Supplementary_Figure_S1.docx
Download MS Word (620.5 KB)Acknowledgements
The authors would like to thank the participating volunteers and their families for taking part in the study, all coinvestigators, and the clinical and nursing staff for conducting the study. None of the authors of this paper has a financial or personal relationship with other individuals or organizations that could inappropriately influence or bias the content of this paper.
Disclosure statement
We confirm that there are no known conflicts of interest associated with this publication and there has been no significant financial support for this work that could have influenced its outcome.
Funding
The study was supported by Taiwan Leader Biotech Corp. Ltd.
References
- Yuan H, Ma Q, Ye L, Piao G. The traditional medicine and modern medicine from natural products. Molecules. 2016;21(5):559. doi:10.3390/molecules21050559.
- Ganesan N, Baskaran R, Velmurugan BK, Thanh NC. Antrodia cinnamomea—an updated minireview of its bioactive components and biological activity. J Food Biochem. 2019;43(8):e12936.
- Wu SH, Ryvarden L, Chang TT. Antrodia camphorata (" niu-chang-chih"), new combination of a medicinal fungus in Taiwan. Bot Bull Acad Sin Taipei. 1997;38:273–6.
- Geethangili M, Tzeng YM. Review of pharmacological effects of Antrodia camphorata and its bioactive compounds. Evid Based Complement Alternat Med. 2011;2011:212641.
- Chen WJ, Chang FW. A pilot study to assess food safety and potential cholesterol-lowering efficacy of Antrodia cinnamomea solid-state cultivated mycelium in healthy adults. Evid Based Complement Alternat Med. 2020;2020:5865764.
- Huang T-T, Lan Y-W, Chen C-M, Ko Y-F, Ojcius DM, Martel J, Young JD, Chong K-Y. Antrodia cinnamomea induces anti-tumor activity by inhibiting the STAT3 signaling pathway in lung cancer cells. Sci Rep. 2019;9(1):5145.
- Lin I-Y, Pan M-H, Lai C-S, Lin T-T, Chen C-T, Chung T-S, Chen C-L, Lin C-H, Chuang W-C, Lee M-C, et al. CCM111, the water extract of Antrodia cinnamomea, regulates immune-related activity through STAT3 and NF-κB pathways. Sci Rep. 2017;7(1):4862.
- Huang C-H, Chang Y-Y, Liu C-W, Kang W-Y, Lin Y-L, Chang H-C, Chen Y-C. Fruiting body of Niuchangchih (Antrodia camphorata) protects livers against chronic alcohol consumption damage. J Agric Food Chem. 2010;58(6):3859–66. doi:10.1021/jf100530c.
- Liu YW, Lu KH, Ho CT, Sheen LY. Protective effects of Antrodia cinnamomea against liver injury. J Tradit Complement Med. 2012;2(4):284–94.
- Shih YL, Wu MF, Lee CH, et al. Antrodia Cinnamomea reduces carbon tetrachloride-induced hepatotoxicity in male wister rats. In Vivo (Athens, Greece). 2017;31:877–84.
- Hsu YL, Kuo YC, Kuo PL, Ng LT, Kuo YH, Lin CC. Apoptotic effects of extract from Antrodia camphorata fruiting bodies in human hepatocellular carcinoma cell lines. Cancer Lett. 2005;221(1):77–89. doi:10.1016/j.canlet.2004.08.012.
- Huang Y-J, Yadav VK, Srivastava P, Wu AT, Huynh T-T, Wei P-L, Huang C-YF, Huang T-H. Antrodia cinnamomea enhances chemo-sensitivity of 5-FU and suppresses colon tumorigenesis and cancer stemness via up-regulation of tumor suppressor miR-142-3p. Biomolecules. 2019;9(8):306. doi:10.3390/biom9080306.
- Su Y-K, Shih P-H, Lee W-H, Bamodu OA, Wu ATH, Huang C-C, Tzeng Y-M, Hsiao M, Yeh C-T, Lin C-M, et al. Antrodia cinnamomea sensitizes radio-/chemo-therapy of cancer stem-like cells by modulating microRNA expression. J Ethnopharmacol. 2017;207:47–56. doi:10.1016/j.jep.2017.06.004.
- Liu J-J, Huang T-S, Hsu M-L, Chen C-C, Lin W-S, Lu F-J, Chang W-H. Antitumor effects of the partially purified polysaccharides from Antrodia camphorata and the mechanism of its action. Toxicol Appl Pharmacol. 2004;201(2):186–93. doi:10.1016/j.taap.2004.05.016.
- Li TY, Chiang BH. 4-Acetylantroquinonol B from Antrodia cinnamomea enhances immune function of dendritic cells against liver cancer stem cells. Biomed Pharmacother. 2019;109:2262–9.
- Long H, Hu CT, Weng CF. Antrodia Cinnamomea prolongs survival in a patient with small cell lung cancer. Medicina (Kaunas, Lithuania. 2019;55(10):640. doi:10.3390/medicina55100640.
- Lin J-Y, Chen M-C, Chiu E. Genotoxicity and subchronic toxicity studies of Taiwanofungus camphoratus extract. Fundam Toxicol Sci. 2019;6(3):81–106. doi:10.2131/fts.6.81.
- Chen CC, Li IC, Lin TW, Chang HL, Lin WH, Shen YC. Efficacy and safety of oral Antrodia cinnamomea mycelium in mildly hypertensive adults: a randomized controlled pilot clinical study. Eur J Integr Med. 2016;8(5):654–60. doi:10.1016/j.eujim.2016.06.001.
- Chen C-Y, Chien S-C, Tsao N-W, Lai C-S, Wang Y-Y, Hsiao W-W, Chu F-H, Kuo Y-H, Wang S-Y. Metabolite profiling and comparison of bioactivity in Antrodia cinnamomea and Antrodia salmonea fruiting bodies. Planta Med. 2016;82(3):244–9.
- Chen TI, Chen CC, Lin TW, Tsai YT, Nam MK. A 90-day subchronic toxicological assessment of Antrodia cinnamomea in Sprague-Dawley rats. Food Chem Toxicol. 2011a;49(2):429–33. doi:10.1016/j.fct.2010.11.018.
- Chiou YL, Chyau CC, Li TJ, et al. Hepatoprotective effect of Antrodia cinnamomea mycelium in patients with nonalcoholic steatohepatitis: a randomized, double-blind, placebo-controlled trial. J Am Coll. Nutr. 2021;40(4):349–357.
- Chen TI, Chen CW, Lin TW, Wang DS, Chen CC. Developmental toxicity assessment of medicinal mushroom Antrodia cinnamomea T.T. Chang et W.N. Chou (higher Basidiomycetes) submerged culture mycelium in rats. Int J Med Mushrooms. 2011b;13(6):505–11. doi:10.1615/intjmedmushr.v13.i6.20.
- Lin CC, Kumar KJS, Liao JW, Kuo YH, Wang SY. Genotoxic, teratotoxic and oral toxic assessments of Antrodia cinnamomea health food product (Leader Deluxe Antrodia cinnamomea®). Toxicol Rep. 2015a;2:1409–17. doi:10.1016/j.toxrep.2015.10.007.
- Chang JB, Wu MF, Lu HF, et al. Toxicological evaluation of Antrodia cinnamomea in BALB/c mice. In Vivo (Athens, Greece). 2013;27:739–45.
- Chen YY, Lo CP, Lin CC, Hsieh YH. Effects of Taiwanofungus camphoratus on non-specific and specific immune activities in mice. Mycology. 2018;9(2):129–35. doi:10.1080/21501203.2018.1437837.
- Lin Y-H, Kuo J-T, Chen Y-Y, Kumar KJS, Lo C-P, Lin C-C, Wang S-Y. Immunomodulatory effects of the stout camphor medicinal mushroom, Taiwanofungus camphoratus (Agaricomycetes)-based health food product in mice. Int J Med Mushrooms. 2018;20(9):849–58. doi:10.1615/IntJMedMushrooms.2018027389.
- Lu C-C, Hsu Y-J, Chang C-J, Lin C-S, Martel J, Ojcius DM, Ko Y-F, Lai H-C, Young JD. Immunomodulatory properties of medicinal mushrooms: differential effects of water and ethanol extracts on NK cell-mediated cytotoxicity. Innate Immun. 2016;22(7):522–33. doi:10.1177/1753425916661402.
- Lin C-C, Pan I-H, Li Y-R, Pan Y-G, Lin M-K, Lu Y-H, Wu H-C, Chu C-L. The adjuvant effects of high-molecule-weight polysaccharides purified from Antrodia cinnamomea on dendritic cell function and DNA vaccines. PLoS One. 2015b;10(2):e0116191. doi:10.1371/journal.pone.0116191.
- Liu KJ, Leu SJ, Su CH, Chiang BL, Chen YL, Lee YL. Administration of polysaccharides from Antrodia camphorata modulates dendritic cell function and alleviates allergen-induced T helper type 2 responses in a mouse model of asthma. Immunology. 2010;129(3):351–62. doi:10.1111/j.1365-2567.2009.03175.x.
- Aldarouish M, Su X, Qiao J, Gao C, Chen Y, Dai A, Zhang T, Shu Y, Wang C. Immunomodulatory effects of chemotherapy on blood lymphocytes and survival of patients with advanced non-small cell lung cancer. Int J Immunopathol Pharmacol. 2019;33:2058738419839592.
- Zheng B, Wang D, Qiu X, Luo G, Wu T, Yang S, Li Z, Zhu Y, Wang S, Wu R, et al. Trajectory and functional analysis of PD-1(high) CD4+ CD8+ T cells in hepatocellular carcinoma by single-cell cytometry and transcriptome sequencing. Adv Sci (Weinh)). 2020;7(13):2000224.