Critical Reviews in Plant Sciences presents cutting-edge reviews on a broad spectrum of research topics, typically focusing on angiosperms and occasionally covering early diverging lineages such as gymnosperms (Thiagarajan et al., Citation2016) and green algae (Leliaert et al., Citation2012). We recently reported on state-of-the-art genome editing research in plants (Songstad et al., Citation2017; Zhang et al., Citation2017) and have highlighted novel cannabis research (Gray et al., Citation2016). In this Special Issue, we focus on the non-vascular bryophytes, which include hornworts, liverworts, and mosses. These fascinating and largely unnoticed plants are integral components of diverse environments on all continents (von Konrat et al., Citation2010). So, look around – with approximately 20,000 species worldwide (http://www.theplantlist.org/1.1/browse/B/) – just about any parcel of land (and some bodies of fresh water) will reveal the presence of common bryophytes. Because this Special Issue is a collection of reviews focusing on non-vascular plants, which are generally considered to be less complex morphologically and with distinctive life histories compared to vascular plants, this introduction will serve to acquaint and orient readers to some of the general ecological and biological attributes of bryophytes.
Bryophytes were some of the first green plants to successfully colonize land 470–551 million years ago (mya) from aquatic algal ancestors (Morris et al., Citation2018). Traditionally, the term “bryophytes” has been used to describe a paraphyletic assemblage of three phyla, Anthocerotophyta (hornworts), Bryophyta (mosses), and Marchantiophyta (liverworts), whose relationships have long been a source of debate within the scientific community (Goffinet, Citation2000 and references therein). Recent phylogenetic analyses support two main evolutionary hypotheses (Nishiyama et al., Citation2004; Wickett et al. Citation2014; Cox, Citation2018; Puttick et al., Citation2018 in this Special Issue): (1) the liverworts and mosses are a monophyletic clade with the hornworts as an independent lineage and (2) all three bryophyte phyla as members of a single clade, sister to vascular plants.
Although the dominant phase in their life cycle is the haploid gametophyte (), bryophytes represent a significant advancement in morphological and reproductive complexity compared to their green algal ancestors (Kenrick and Crane, Citation1997). Their plant bodies are either organized into dorsoventrally flattened thalli (hornworts and liverworts) or branching stem-like tissues (caulids) with leaf-like structures (phyllids) arranged spirally (mosses) or in ranks (liverworts and a few mosses). Bryophytes lack roots, but have filamentous rhizoids that anchor the plants to the substrate. Water and nutrient uptake occurs through the above-ground gametophyte surfaces. Although some bryophytes carry out internal transport via conducting cells, no bryophytes produce lignin (Ligrone et al., Citation2000; Citation2008). Sexual reproduction shares a common theme in bryophytes: sessile female gametes (eggs) are produced in archegonia and motile male gametes (flagellated sperm) are formed in antheridia. Water is required for sexual reproduction in bryophytes, though moss sperm can survive periods of desiccation (Shortlidge et al., Citation2012), potentially extending their reproductive viability. Bryophyte species are genetically predetermined to produce either hermaphroditic (monoicous) or single-sexed gametophytes (dioicous). The diploid sporophyte is relatively inconspicuous compared to the gametophytic phase and is attached to and nutritionally dependent on the maternal gametophyte throughout its lifespan. The sporophyte produces haploid spores via meiosis that are often wind-dispersed, sometimes across long distances (Muñoz et al., Citation2004) to initiate new gametophyte populations. Bryophytes also reproduce asexually by the physical fragmentation of gametophyte plants or in some species by the formation of gemmae, which are small, multicellular structures that develop in splash cups on the surfaces of mosses and liverworts and grow into clonal plants ().
Figure 1. Life cycle of a moss, which serves to illustrate the generalized life cycle of bryophytes. (A) Green, leafy gametophyte that produces (B) antheridia and (C) archegonia. (D) Sperm from the antheridia fertilize eggs in archegonia to produce the young sporophyte, which is initially completely surrounded by tissues of the maternal gametophyte that later form the calyptra. The maturing sporophyte (E) consist of the foot, seta, and capsule. At the base, the foot is embedded in the gametophyte where it acquires water and nutrients from the maternal plant. The calyptra and operculum dislodge revealing peristome teeth (F). Haploid spores (G) germinate and form protonema (H), which in turn produce the leafy gametophytes.
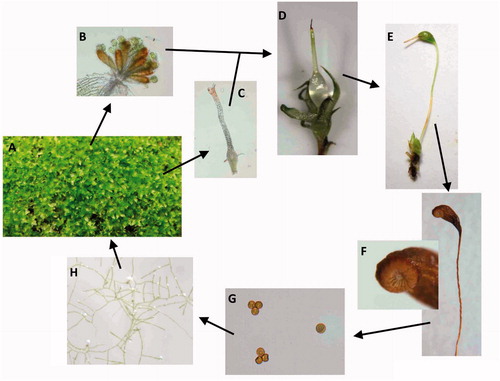
Figure 2. (A) Two gemmae cups of a moss. (B) Microscopic view of two moss gemmae. (C) Longitudinal section through gemma (G) and the gemma cup wall (GCW) of a liverwort, Marchantia species. T: thallus.
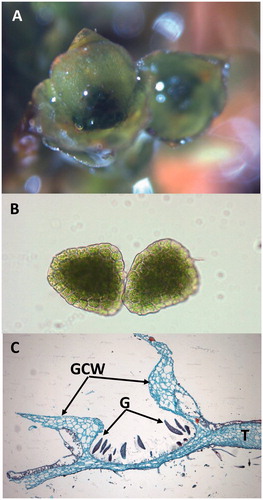
Bryophytes occupy a wide variety of habitats (), with the majority of species able to survive periods of desiccation. These plants can live in deserts (e.g., Grimmia, Tortula), fresh water (e.g., Fontinalis, Riccia), disturbed open soil (e.g., Funaria, Phaeoceros), tropical rainforests (e.g., Calymperes, Drepanolejeunea), and mesic temperate forests (e.g., Bazzania, Hypnum). There are also about 130 species of mosses and 30 species of liverworts found in the Antarctic and these taxa have evolved the capacity to survive the extremely xeric and cold environs of this polar region via desiccation tolerance mechanisms (Bramley-Alves et al., Citation2014), such as poikilohydry (Smith et al., Citation1997; Lewis et al., Citation2017). Hornworts, however, are absent from the Arctic (Lewis et al., Citation2017) and the Antarctic (Bramley-Alves et al., Citation2014) polar regions. The ability of bryophytes to survive and recover from long-term suspended animation was demonstrated to an amazing level when four species of moss were revived after 400–600 years frozen beneath the Tear Drop Glacier in northern Canada (La Farge et al., Citation2013). In addition, these fascinating plants play many important ecological roles as habitats for invertebrates, slowing water movement through systems and thus decreasing erosion, and as seedbeds promoting germination. However, bryophytes are reported to prevent germination of seeds as well (Whitehead et al., Citation2018). They are also “the canaries in the coal mine” and have been used as indicators of environmental health due to their sensitivities to air/rain water pollution (Pescott et al., Citation2015; Gatziolis et al., Citation2016).
Figure 3. Habitats of mosses. (A) Growing between concrete slabs in a sidewalk. (B) Growing on debris in a forest. (C) Growing on the side of tree in a temperate forest. (D) Growing on exposed soil and across a trailing vine in a tropical forest. (E) Growing on vertical rock face. (F) Growing on an old tree stump. (G) Growing in a wet bog. (H) Initially growing on animal dung in a bog in southern Chile.
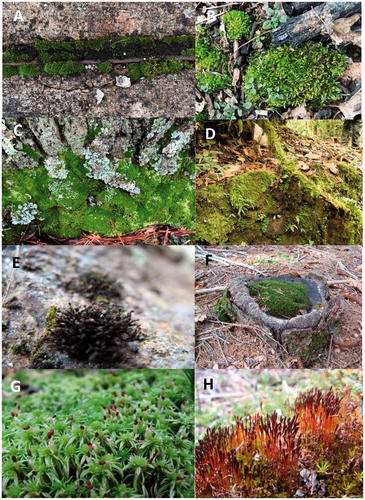
I. Bryophyta (mosses)
Mosses are represented by 9000 (Magill, Citation2010), to 13,000 (Goffinet et al., Citation2008) species. The moss life cycle is distinguished from the other bryophytes by their highly branching, filamentous, or thalloid (e.g., Sphagnum species) protonema that enable the production of multiple leafy stems from a single spore germination event (). Continued growth results in a collection of upright or prostrate caulids covered in phyllids, which can function as a super organism with many individuals forming mats or cushions that can increase water retention and slow water loss (). Water is essential for reproduction and moss sperm, formed in antheridia (), can be dispersed via rain and even insects (Cronberg et al., Citation2006; Rosenstiel et al., Citation2012), where chemical compounds produced by the female archegonia () can attract the insects or the sperm directly. Sperm of the moss Physcomitrella patens may be further oriented to swim through the water-filled neck canal toward the egg via activation of two membrane-bound proteins and changes in intracellular calcium ion levels (Steinhorst and Kudla, Citation2017). The sperm and egg fuse and form the zygote, which in turn divides to form a two-celled embryo proper. These cells form the sporophyte that ultimately consists of the foot (embedded in the maternal gametophyte and functioning in water/nutrient transfer), the seta/stalk, and the capsule (). Throughout early development the sporophyte apex is topped by the calyptra, a maternal cap of gametophyte tissue, that is covered by a relatively thin cuticle that protects the immature sporophyte from water loss (Budke et al., Citation2011; Citation2013). Moss sporophytes are initially green and capable of photosynthesis, but they do not fix sufficient carbon to sustain their entire growth and development (Proctor, Citation1977). The capsule is differentiated into a central sterile columella and surrounded by spore mother cells, which undergo meiosis synchronously to form haploid spores. After meiosis, mature sporophytes dry out and ultimately release the spores via the operculum, a cap of tissue covering the capsule opening during development. After the operculum detaches from the capsule, the peristome teeth in some mosses are responsive to changes in humidity, facilitating spore dispersal from the capsule (Gallenmüller et al., Citation2018). Moss sporophytes are relatively persistent, compared to the sporophytes of liverworts and hornworts and can be found attached to their maternal gametophytes months beyond fertilization, dispersing spores long after the sporophyte itself has turned brown and is dead.
Figure 4. Moss gametophyte structures. (A) (C–F) Polytrichium species. (B) Leucobryum species. (A) Protonema. (B) Leafy gametophytes clustered together in a cushion. (C) Antheridia (arrows) surrounded by perigonial bracts at the terminus of the male leafy gametophyte. (D) Enlargement of antheridia exhibiting mitotically dividing cells, which will give rise to sperm. (E) Archegonia (A) surrounded by paraphyses (P) at the terminus of the female leafy gametophyte. (F) Enlargement of the archegonium (A), neck (N), neck canal (NC) and egg (E). All images except (B) were obtained from slides and used with permission from Carolina Biological Supply, Whitsett, North Carolina, Copyright Carolina Biological Supply.
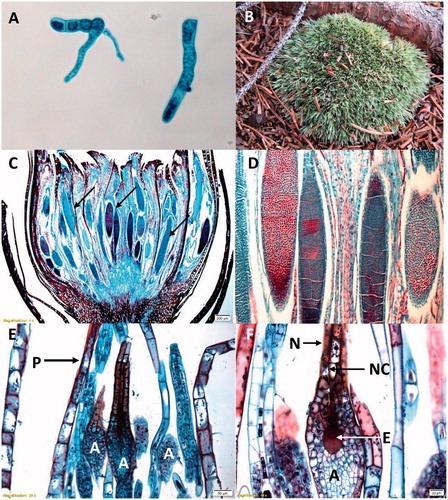
Figure 5. Mosses. (A) A mass of leafy green gametophytes and brown sporophytes of Funaria hygrometrica. Note the long tapering calyptra (arrows) covering the sporophyte apex and the expanding capsules. Insert is a single gametophyte (G) with an attached green sporophyte (GS). S: seta; C: calyptra covering the capsule; Line: about 8 mm. (B) Longitudinal section through the sporophyte capsule of a Polytrichium species. S: seta wall, O: operculum; CO: columella; SP: haloid spores; and P: peristome. The section does not show the calyptra. Image was obtained from a slide and used with permission from Carolina Biological Supply, Whitsett, North Carolina, Copyright Carolina Biological Supply.
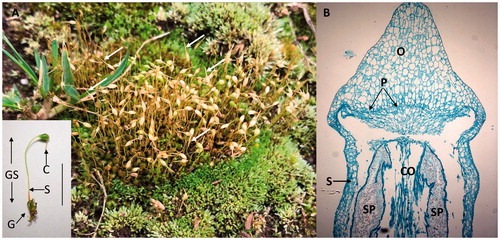
II. Marchantiophyta (liverworts)
There are 5000 to 7500 species of liverworts worldwide (Söderström et al., Citation2016; von Konrat et al., Citation2010) making this the second largest phylum of bryophytes. Morphologically the liverwort gametophytes are diverse with both simple and complex thalloid species that are flattened dorsoventrally () and leafy liverworts that have a three-ranked caulid and phyllid arrangement, which are somewhat similar to mosses. Marchantia, belongs to the complex, thalloid liverworts, which include about 5% of all liverworts and has often been used to represent the morphology of liverworts. In Marchantia photosynthesis only occurs in a defined cell layer on the dorsal or upper surface bounded by epidermal cells with pores for gas exchange (). Rhizoids and scales are located on the lower surface and serve to absorb water and anchor the plant to the substrate. The cells between the upper and lower epidermis are mostly thin-walled and used for storage of oils and other products. In Marchantia, male and female gametangia are produced on umbrella-like antheridiophores and archegoniophores () on separate plants. The archegonia are inverted and hang downward from the bottom of the structure (), whereas the male gametangia are located on the upper surface of the gametophores (). Sperm dispersal is similar to mosses in that they are facilitated by water, though no tests have been made to determine whether animals may contribute to their dispersal. Marchantia sporophytes () are composed of a foot embedded in the female gametophyte, setae, and a capsule and as in the mosses the sporophyte is dependent on the gametophyte for water and nutrition. The capsules of some, but not all, liverworts contain elaters (), which are diploid structures intermixed with the haploid spores. Elaters are long, narrow cells with secondary wall thickenings thought to have two functions. First, they may serve as nutrition for the developing spores and second, may be involved in spore dispersal in response to changes in moisture and humidity (Schuster, Citation1966; Kremer and Drinnan, Citation2003).
Figure 7. Transverse section through the thallus of Marchantia species. The upper epidermis (UE) is composed of small cells covered by a cuticle and has numerous barrel-shaped air pores (AP) or channels for gas exchange. Beneath the upper epidermis are air chambers (AC) containing photosynthetic cells or filaments also known as the photosynthetic zone (PZ) or upper assimilatory region (see insert in upper right). The majority of the thallus is composed of storage cells (SC) that contain various substances including oils. The lower surface has an epidermis (LE) with numerous unicellular rhizoids (R; appear unattached due to section orientation) and multicellular scales (S) that serve to anchor the thallus to the substrate. Images were obtained from slides and used with permission from Carolina Biological Supply, Whitsett, North Carolina, Copyright Carolina Biological Supply.
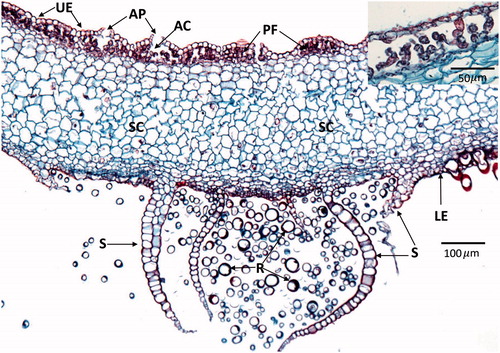
Figure 8. Images of Marchantia species with gametangiophores. (A) Mostly archegoniophores (arrows). (B) Mostly antheridiophores (arrows).
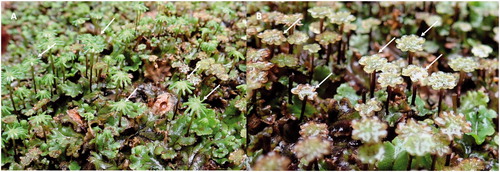
Figure 9. Gametangiophores of Marchantia. (A) Longitudinal section though an archegoniophore (ARP) showing several archegonia (AR). T: thallus; S: scales; R: rhizoids. (B) Longitudinal section through an antheridiophore (ANP) and male antheridia (AN). T: thallus. Images were obtained from slides and used with permission from Carolina Biological Supply, Whitsett, North Carolina, Copyright Carolina Biological Supply.
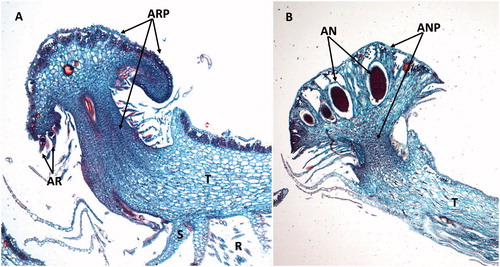
Figure 10. Sporophytes of Marchantia. (A) Sporophyte (hanging downward) with foot (F) embedded in the archegoniophore (ARP). The seta (S) supports the capsule (C) containing spores. (A) Enlargement of the capsule showing thread-like elaters (arrows). Images were obtained from slides and used with permission from Carolina Biological Supply, Whitsett, North Carolina, Copyright Carolina Biological Supply.
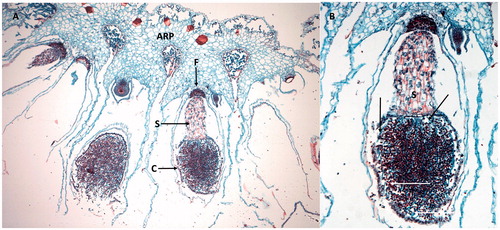
III. Anthocerotophyta (hornworts)
There are only an estimated 215 species of hornworts worldwide (Söderström et al., Citation2016), making them the smallest bryophyte phylum. These plants, like some of the liverworts, have flattened thalli. Both antheridia and archegonia develop within the gametophytic tissues of hornworts, whereas the same structures in mosses and liverworts are formed on the gametophyte surface (Renzaglia et al., Citation2007). At the cellular level, hornworts contain only a single chloroplast per cell, compared to multiple chloroplasts per cell in mosses and liverworts. These solitary chloroplasts typically contain proteinaceous bodies called pyrenoids that are a critical carbon-concentrating mechanism (Villarreal and Renner, Citation2012) a feature shared by hornworts and green algae. Species in all 13 hornwort genera form endosymbiotic relationships with nitrogen-fixing cyanobacteria (Renzaglia et al., Citation2007), typically Nostoc species (Costa et al., Citation2001). These bacteria invade hortwort tissue through pores (mucilage clefts) on the underside of the thallus and then grow internally in canals formed from the separation of the middle lamella between cells. It was thought that only a few mosses have a symbiotic relationship with cyanobacteria and these are mostly epiphytic, except for two Sphagnum species in which there exists endophytic symbiosis (Solheim and Zielke, Citation2002). However, recent evidence revealed that cyanobacteria are common symbionts with boreal forest floor mosses such as Sphagnum, Pleurozium, and Hylocomium species (e.g., DeLuca et al., Citation2002, Citation2008; Turetsky et al. Citation2012;). In contrast, relatively few liverworts have this relationship with the notable exceptions of Marchantia and Porella (see review: Adams and Duggan, Citation2008). Certainly, the association of nitrogen-fixing bacteria with hornworts offers a nutritional advantage to the plants. Hornworts have a unique sporophyte development wherein this diploid structure is produced from a basal meristem and splits longitudinally into two halves, forming the characteristic horns that inspired the common name (). The sporophytes also have stomata located in their epidermis, which are thought to be involved in sporophyte drying and dehiscence (Villarreal and Renzaglia, Citation2015).
Figure 11. Hornworts. (A) Gametophyte on the forest floor with mature sporophytes (arrows) that have released spores. (B) Gametophyte thallus with immature sporophytes (arrows) that have not split to release the spores. (C) Gametophyte thallus with mature sporophytes (arrows) that have split at the apex into the distinctive “horns” to release the spores.
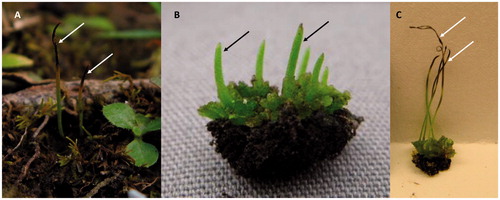
A. Special issue research articles
The focus of this Special Issue are aspects of the bryophytes. Each of the review articles is written by noted experts in their respective fields of study. The titles of the four main manuscripts of the isue are the following: (1) Land plant molecular phylogenetics: a review with comments on evaluating incongruence among phylogenies (Cox, Citation2018); (2) The evolutionary diversity of mosses – taxic heterogeneity and its ecological drivers (Huttunen et al., Citation2018); (3) Bryophyte biogeography (Patiño and Vanderpoorten, Citation2018); and (4) Terpenoid secondary metabolites in bryophytes: chemical diversity, biosynthesis and biological functions (Chen et al., Citation2018). A fifth paper detailing liverworts was withdrawn from this Special Issue and will appear in a latter issue of Critical Reviews in Plant Sciences.
Cox (Citation2018) reviews the relationships between the three major bryophyte lineages relative to tracheophytes. Analyses of morphological and molecular characters for phylogenetic reconstructions are summarized in a table of 43 unique studies that describes their findings related to the relationships among these lineages. A thorough discussion of the impact of data sampling and model choice on the analyses is also presented. The author highlights the two best-supported hypotheses, which are “monophyletic bryophytes” and "liverworts plus mosses-basal" and the fact that both of these hypothesis support mosses and liverworts as sister lineages. These two hypotheses are favored based on data from slower evolving amino-acids that are preferred over nucleotide data and better fitting models that account for composition heterogeneity. Ultimately the author discusses his reasoning behind favoring the monophyly of bryophytes hypothesis and concludes by discussing the impact of that hypothesis on our understanding of plant evolution and the morphology of the most recent common ancestor of land plants.
Huttunen et al. (Citation2018) explore changes in habitat and their connection to changes in morphology in a contemporary phylogenetic framework for the 12,500 moss species, by mapping habitat evolution and seven morphological characters onto a time-calibrated phylogeny. They determined that in mosses shifts between terrestrial, epiphytic, and aquatic habitats have occurred at least 180 times with derived lineages most often containing epiphytic and aquatic taxa. Shifts to aquatic habitats occurred most commonly in cool biomes of the Northern Hemisphere. Reversals to other habitats occurred in several epiphytic clades, but were not detected in clades composed of aquatic species. Additionally, they discuss how the position of the female gametangia is often linked to growth habit with transitions to cladocarpy and to a lesser degree pleurocarpy, occurring frequently during moss evolution. Sporophyte morphology is also explored. At classification levels above subclass dehiscence mechanisms and the peristome are phylogenetically stable, whereas below the ordinal level sporophyte characters vary based on environmental conditions. Reduced sporophyte structures are associated with taxa growing in open, frequently disturbed habitats and epiphytic lineages. This article shows how molecular phylogenies provide a powerful tool for testing the hypothesized relationships between morphological evolution and ecological characters and lays the foundation for future studies of additional morphological structures across mosses.
Patiño and Vanderpoorten (Citation2018) explore the ecological and evolutionary biogeography of bryophytes. They highlight research examining the relationships between spore and vegetative propagule dispersal, sexual systems, and species distributions. Recent evidence suggests that vegetative propagules may also contribute to long distance dispersal (LDD), especially for species that are not reproducing sexually. They review the literature reporting transoceanic disjunctions among bryophyte species, finding that the disjunctions largely predate continental split events, indicating that repeated events of LDD most likely explain the disjunction patterns. Bryophyte endemism is discussed in comparison to angiosperms in light of the lack of overlap in the biodiversity hotspots for these two groups and the lower levels of endemism found in bryophytes. Lower levels of bryophyte endemism can be attributed to widely distributed taxa that are actually multiple species with narrower distribution ranges, lower speciation rates, and higher rates of post-speciation dispersal. They highlight the recent publication of the worldwide checklist of liverworts and hornworts that has enabled the demonstration of latitudinal diversity gradients in liverworts based on analyses of species richness distribution patterns. The authors conclude with a call to action for continued research in bryophyte taxonomy and floristics that can inform conservation, especially focusing on the assembly of a worldwide moss checklist that would enable similar explorations of latitudinal diversity gradients in these bryophytes.
Chen et al. (Citation2018) provide an informative and timely overview of terpenes present in bryophytes. As a starting point, the authors describe the chemical diversity of terpenes, indicating that in bryophytes the underlying molecular basis so far remain unaddressed. In the past, higher plants were thoroughly investigated regarding the genetic and biochemical capabilities of terpene metabolism and many plant terpene synthases, including mono-, sesqui- and di-terpene synthases, were isolated and characterized; for instance at least 90 monoterpene synthases are known. The plant terpene gene family is subdivided into seven families, tps a-g. Based on the reaction mechanism, terpene synthases either employ ionization-induced carbocation formation (class I), protonation-induced carbocation formation (class II), or are bifunctional enzymes that use both mechanisms. The aspartate-rich motif plays an important role in coordinating the three-magnesium cluster, which is involved in binding the diphosphate moiety of the substrate, e.g., geranyl pyrophosphate (GPP), farnesyl pyrophosphate (FPP), or geranylgeranyl pyrophosphate (GGPP). Interestingly, bryophyte terpene synthases are distinct from typical plant terpene synthases and share more homology with microbial terpene synthases. This is a novel and significant finding and subsequently, the terpene synthases of bryophytes are called microbial terpene synthases-like (MTPSL). State of the art research on MTPSL is presented here and furthermore, the authors give an overview of the possible functions of terpenes in the environment and in plant-plant interactions. The new results regarding the terpene synthesis gene “equipment” of bryophytes fit together nicely and support the earlier finding of the terpene compounds. To our knowledge, a publication with similar content and combination of topics has never been published.
References
- Adams, D. G. and Duggan, P. S. 2008. Cyanobacteria-bryophyte symbioses. J. Exp. Bot. 59: 1047–1058.
- Bramley-Alves, J., King, D. H., Robinson, S. A., and Miller, R. E. 2014. Dominating the Antarctic environment: bryophytes in a time of change. Adv. Photosynth. Resp. 37: 309–324.
- Budke, J. M., Goffinet, B., and Jones, C. S. 2011. A hundred-year-old question: is the moss calyptra covered by a cuticle? A case study of Funaria hygrometrica. Ann. Bot. 107: 1259–1277
- Budke, J. M., Goffinet, B., and Jones, C. S. 2013. Dehydration protection provided by a maternal cuticle improves offspring fitness in the moss. Funaria hygrometrica. Ann. Bot. 111: 781–789.
- Chen, F., Ludwiczuk, A., Wei, G., Chen, X., Crandall-Stotler, B., and Bowman, J. L. 2018. Terpenoid secondary metabolites in bryophytes: chemical diversity, biosynthesis and biological functions. Crit. Rev. Plant Sci. 37.
- Costa, J.-L., Paulsrud, P., Rikkinen, J., and Lindblad, P. 2001. Genetic diversity of Nostoc symbionts endophytically associated with two bryophyte species. Appl. Environ. Microbiol. 67: 4393–4396.
- Cox, C. J. 2018. Land plant molecular phylogenetics: a review with comments on evaluating incongruence among phylogenies. Crit. Rev. Plant Sci. 37.
- Cronberg, N., Natcheva, R., and Hedlund, K. 2006. Microarthropods mediate sperm transfer in mosses. Science 313: 1255.
- DeLuca, T. H., Zackrisson, O., Gundale, M. J., and Nilsson, M.-C. 2008. Ecosystem feedbacks and nitrogen fixation in boreal forests. Science 320: 1181.
- DeLuca, T. H., Zackrisson, O., Nilssson, M. C., and Sellstedt, A. 2002 Quantifying nitrogen-fixation in feather moss carpets of boreal forests. Nature 419: 917–920.
- Gallenmüller, F., Langer, M., Poppinga, S., Kassemeyer, H.-K., and Speck, T. 2018. Spore liberation in mosses revisited. AoB Plants 10: plx075. doi:10.1093/aobpla/plx07
- Gatziolis, D., Jovan, S., Donovan, G., Amacher, M., and Monleon, V. 2016. Elemental atmospheric pollution assessment via moss-based measurements in Portland, Oregon. Gen. Tech. Rep. PNW-GTR-938. Portland, OR: U.S. Department of Agriculture, Forest Service, Pacific Northwest Research Station. p.55.
- Goffinet, B. 2000. The origin and phylogenetic relationships of bryophytes. In Bryophyte Biology, 1st ed.; Shaw, A. J. and Goffinet, B., Eds. Cambridge University Press: England.
- Goffinet, B., Buck, W. R., and Shaw, A. J. 2008. Morphology, anatomy and classification of the Bryophyta. In Bryophyte Biology, 2nd ed.; Goffinet, B and Shaw, A. J. Eds. Cambridge University Press: England, pp 55–138.
- Gray, D. J., Clarke, R. C., and Trigiano, R. N. 2016. Introduction to the special issue on cannabis. Crit. Rev. Plant Sci. 35: 289–292.
- Huttunen, S., Bell, N., and Hedenäs, L. 2018. The evolutionary diversity of mosses – taxic heterogeneity and its ecological drivers. Crit. Rev. Plant Sci. 37.
- Kenrick, P. and Crane, P. 1997. The origin and early evolution of plants on land. Nature 389: 33–39.
- Kremer, C. and Drinnan, A. 2003. Secondary wall formation in elaters of liverworts and the hornwort Megaceros. Intl. J. Plant Sci. 164: 823–834.
- La Farge, C., Williams, K. H., and England, J. H. 2013. Regeneration of little ice age bryophytes emerging from a polar glacier with implications of totipotency in extreme environments. Proc. Natl. Acad. Sci. USA 110: 9839–9844.
- Leliaert, F., Smith, D. R., Moreau, H., Herron, M. D., Verbruggen, H., Delwiche, C. F., and De Clerck, O. 2012. Phylogeny and molecular evolution of the green algae. Crit. Rev. Plant Sci. 31: 1–46.
- Lewis, L. R., Ickert-Bond, S. M., Biersma, E. M., Convery, P., Goffinet, B., Hassel, K., Kruijer, H. J. D., La Farge, C., Metzgar, J., Stech, M., Villarreal, J. C., and McDaniel, S.F. 2017. Future directions and priorities of Arctic bryophyte research. Arctic Sci. 3: 475–497
- Ligrone, R., Duckett, J. G., and Renzaglia, K. S. 2000. Conducting tissues and phyletic relationships of bryophytes. Phil. Trans. Royal Soc. B 355: 795–813.
- Ligrone, R., Carafa, A., Duckett, J. G., Renzaglia, K. S., and Ruel, K. 2008. Immunocytochemical detection of lignin-related epitopes in cell walls in bryophytes and the charalean alga Nitella. Plant System. Evol. 270: 257–272.
- Magill, R.E. 2010. Moss diversity: new look at old numbers. Phytotaxa 9: 167–174.
- Morris, J. L., Puttick, M. N., Clark, J. W., Edwards, D., Kenrick, P. Pressel, S., Wellman, C. H., Yang, Z., Schneider, H., and Donoghue, P. C. J. 2018. The timescale of early land plant evolution. Proc. Natl. Acad. Sci. USA 115: E2274–E2283. doi:10.1073/pnas.1719588115
- Muñoz, J., Felicisímo, A. M., Cabezas, F., Burgaz, A. R., and Martínez. I. 2004. Wind as a long-distance dispersal vehicle in the Southern hemisphere. Science 304: 1144–1147.
- Nishiyama, T., Wolf, P. G., Kugita, M., Sinclair, R. B., Sugita, M., Sugiura, C., Wakasugi, T., Yamada, K., Yoshinaga, K., Yamaguchi, K., Ueda, K., and Hasebe, M. 2004. Chloroplast phylogeny indicates that bryophytes are monophyletic. Molec. Biol. Evol. 21: 1813–1819.
- Patiño, J., and Vanderpoorten, A. 2018. Bryophyte biogeography. Crit. Rev. Plant Sci. 37.
- Pescott, O. L., Simkin, J. M., August, T. A., Randle, Z., DoreMarc, A. J., and Botham, S. 2015. Air pollution and its effects on lichens, bryophytes, and lichen-feeding Lepidoptera: review and evidence from biological records. Biol. J. Linnean Soc. 115: 611–635
- Proctor, M. C. F. 1977. Evidence on the carbon nutrition of moss sporophytes from 14CO2 uptake and the subsequent movement of labelled assimilate. J. Bryology 9: 375–386.
- Puttick, M. N., Morris, J. L., Williams, T. A., Cox, C. J., Edwards, D., Kenrick, P., Pressel, S., Wellman, C. H., Schneider, H., Pisani, D., and Donoghue, P. C. J. 2018. The interrelationships of land plants and the nature of the ancestral embryophyte. Curr. Biol. 28: 733–745.
- Renzaglia, K. S., Schuette, S., Duff, R. J., Ligrone, R., Shaw, A. J., Mishler, B. D., and Duckett, J.G. 2007. Bryophyte phylogeny: advancing the molecular and morphological frontiers. Bryologist 110: 179–213.
- Rosenstiel, T. N., Shortlidge, E. E., Melnychenko, A. N., Pankow, J. F., and Eppley, S M. 2012. Sex-specific volatile compounds influence microarthropod-mediated fertilization of moss. Nature 489: 431–43.
- Schuster R. M. 1966. The Hepaticae and Anthocerotae of North America: East of the Hundredth Meridian. Vol 1. Columbia University Press, New York.
- Shortlidge, E. E., Rosenstiel, T. N., and Eppley, S. M. 2012. Tolerance to environmental desiccation in moss sperm. New Phytologist 194: 741–750.
- Smith, S, D., Monson, R. K., and Anderson, J. E. 1997. Poikilohydric plants. In Physiological Ecology of North American Desert Plants; Smith, S. D., Monson, R. K., and Anderson, J. E., Ed(s).; Springer-Verlag, Berlin, pp. 191–198
- Söderström, L., Hagborg, A., von Konrat, M., Bartholomew-Began, S., Bell, D., Briscoe, L., Brown, E., Cargill, D. C., Costa, D. P., Crandal-Stotler, B. J., Cooper, E. D., Dauphin, G., Engel, J. J., Feldberg, D. G., Gradstein, S. R., He, X., Heinrichs, J., Hentschel, J., Ilkiu-Gorges, A. L., Katagiri, T., Konstantinova, N. A., Larraín, J., Long, D. G., Nebel, M., Pócs, T., Puche, F., Reiner-Drehwald, E., Renner, M. A. M. m Sass-Gyarmati, A., Schafer-Verwimp, A., Moragues, J. G. S., Stotler, R. E., Sukkharak, P., Thiers, B. M., Uribe, J., Váňa, J., Villarreal, J. C., Wigginton, M., Zhang, L., and Zhu R.-L. 2016. World checklist of hornworts and liverworts. PhytoKeys 59: 1–828.
- Songstad, D. D., Petolino, J. F., Voytas, D. F., and Reichert, N.A. 2017. Genome editing of plants. Crit. Rev. Plant Sci. 36: 1–23.
- Solheim, B. and Zielke, M. 2002. Associations between cyanobacteria and mosses. In Cyanobacteria in Symbiosis; Rai A. N., Bergman B., and Rasmussen, Eds.; Dordrecht: Kluwer Academic Publishers, pp 37–152.
- Steinhorst, L. and Kudla, J. 2017. Plant science: sexual attraction channelled in moss. Nature 549: 35–36.
- Thiagarajan, A., MacDonald, M. T., and Lada, R. 2016. Environmental and hormonal physiology of postharvest needle abscission in christmas trees. Crit. Rev. Plant Sci. 35: 1–17
- Turetsky, M. J., Bond-Lamberty, B., Euskirchen, E., Talbot, J., Frolking, S., McGuire, A. D., and Tuittila, E.-S. 2012. The resilience and functional role of moss in boreal and arctic ecosystems. New Phytologist 196 (1): 49–67.
- Villarreal, J. C., and Renner, S. S. 2012. Hornwort pyrenoids, carbon-concentrating structures, evolved and were lost at least five times during the last 100 million years. Proc. Natl. Acad. Sci. 109: 18873–18878.
- Villarreal, J. C. and Renzaglia, K. S. 2015. The hornworts: important advancements in early land plant evolution, J. Bryology 37: 157–170.
- von Konrat, M., Shaw, A. J., and Renzaglia, K. S. 2010. A special issue of Phytotaxa dedicated to Bryophytes: the closest living relatives of early land plants. Phytotaxa 9: 5–10.
- Whitehead, J., Wittemann, M., and Cronberg, N. 2018. Allelopathy in bryophytes – a review. Lindbergia 41: linbg.01097. doi:10.25227/linbg.01097
- Wickett, N. J., J., Mirarab, S., Nguyen, N., Warnow, T., Carpenter, E., Matasci, N., Ayyampalayam, S., Barker, M. S., Burleigh, J. G., Gitzendanner, M. A., Ruhfel, B. R., Wafula, E., Der, J. P., Graham, S. W., Mathews, S., Melkonian, M., Soltis, D. E., Soltis, P. S., Miles, N. W., Rothfels, C. J., Pokorny, L., Shaw, A. J., DeGironimo, L., Stevenson, D. W., Surek, B., Villarreal, J. C., Roure, B., Philippe, H., dePamphilis, C. W., Chen, T., Deyholos, M. K., Baucom, R. S., Kutchan, T. M., Augustin, M. M., Wang, J., Zhang, Y., Tian, Z., Yan, X., Wu, X. Sun, G.K.-S., Wong, and Leebens-Mack, J. 2014. Phylotranscriptomic analysis of streptophytes. Proc. Natll Acad. Sci. (USA) 111: E4859–E4868.
- Zhang, H., Zhang, J., Lang, Z., Bottella, J. R., and Zhu, J.-K. 2017. Genome editing—Principles and applications for functional genomics research and crop improvement. Crit. Rev. Plant Sci. 36: 291–309.