Abstract
The SPOT-MAS assay “Screening for the Presence Of Tumor by Methylation And Size” detects the five most common cancers in Vietnam by evaluating circulating tumor DNA in the blood. Here, we validated its performance in a prospective multi-center clinical trial, K-DETEK. Our analysis of 2795 participants from 14 sites across Vietnam demonstrates its ability to detect cancers in asymptomatic individuals with a positive predictive value of 60%, with 83.3% accuracy in detecting tumor location. We present a case report to support further using SPOT-MAS as a complementary method to achieve early cancer detection and provide the opportunity for early treatment.
Introduction
Cancer is a major public health and economic issue and is the leading cause of death worldwide (Citation1). According to GLOBOCAN 2020, the number of new cancer cases globally was 19.3 million, and approximately 10 million cancer-related deaths were reported (Citation2). In Vietnam, GLOBOCAN 2020 reported over 182,500 newly diagnosed cases and 122,690 cancer-related deaths (Citation3). Of these, the top five most common types of cancer in Vietnam were liver (14.5%), lung (14.4%), breast (11.8%), gastric (9.8%), and colorectal cancer (9%). Up to 80% of cancer patients in Vietnam were diagnosed at stage III or stage IV, leading to a high rate of 1-year mortality (25%) and a low 5-year survival rate compared to other countries (Citation4). Early cancer screening assays capable of identifying early-stage cancer patients among high-risk or asymptomatic individuals are an essential component of the cancer control program recommended by the World Health Organization (WHO), aiming to achieve better treatment outcomes and reduced mortality (Citation5). Cancer screening programs for breast (mammography), cervical (cervical cytology and/or high-risk human papillomavirus), and colorectal cancers (stool-based tests or endoscopy), and targeted screening for lung cancer (low-dose computed tomography) and prostate cancer (prostate-specific antigen [PSA] based test) for high-risk populations is recommended by The United States Preventive Services Task Force (USPSTF) (Citation6–10) and WHO guidelines (Citation5). While these routine screening tests reduce cancer-specific mortality (Citation11–14), they are associated with invasiveness, low accessibility, and high false positive rates when used sequentially, resulting in overdiagnosis and overtreatment.
Liquid biopsy (LB) has emerged as a potential approach for early cancer detection due to its non-invasiveness and the ability to detect multiple cancer types simultaneously. Most LB assays are based on detecting circulating tumor DNA (ctDNA) in blood. Cell-free tumor DNA (ctDNA) is, theoretically, cell-free DNA (cfDNA) that originates from tumor cells and could contain tumor-specific genetic and epigenetic aberrations (Citation15,Citation16). In practice, no DNA extraction methods is capable of separating ctDNA from cfDNA (circulating DNA comes from other cells), and therefore ctDNA comprises only a minor proportion of total cfDNA when extracted from liquid biopsy samples. The characterization of ctDNA could open the door to many applications such as profiling tumor mutational landscapes (Citation17), monitoring treatment response (Citation18), and especially early cancer screening for asymptomatic individuals (Citation19). In 2020, the Food and Drug Administration (FDA) approved the use of two liquid biopsy-based assays (FoundationOne Liquid CDx and Guardant360 CDx) for detecting genetic changes in ctDNA to assist doctors in selecting the best targeted therapies for patients with lung and prostate cancer (Citation20).
There are several assays of early cancer screening based on LB, each uses different signatures on ctDNA. The CancerSEEK test can detect eight types of cancer, including ovarian, liver, stomach, pancreatic, esophageal, colon, lung, and breast cancer (Citation21). It is based on detecting mutations in 16 specific cancer genes combined with eight biochemical markers to give insights into cancer risk. The average sensitivity of the CancerSEEK test for 8 cancer types ranged from 33% to 98%, and the specificity was 99% (Citation21). However, we and others have shown that this mutation-based approach requires prior knowledge of a tumor and cannot easily address tumor heterogeneity (Citation22–24). In addition, this approach is further hindered by the presence of clonal haematopoiesis of indeterminant potential (CHIP) mutations, which are mutations often associated with cancer in blood cells that can result in high false-positive rates (Citation24). The DELFI test moved away from mutation-based approach and used genome-wide fragment length variations of ctDNA in an attempt to increase sensitivity (Citation25). DELFI reported an overall sensitivity of 80% and specificity of 95% for detecting 7 cancer types, including breast, colorectal, lung, ovarian, prostate, stomach, and gallbladder cancer (Citation25). The GALLERI test used yet another approach based on specific methylation variations of tumor DNA released into the bloodstream to screen for >50 early-stage cancers (Citation26,Citation27). Overall, this test simultaneously detected multiple cancer types (from 8 to 50), showing specificity above 95% with varied sensitivity from 44% to 98% depending on cancer types and disease stages.
We previously developed a multimodal LB-based assay named Screening for the Presence Of Tumor by Methylation And Size (SPOT-MAS) to detect the five most common cancer types in Vietnam (liver, breast, colorectal, gastric, and lung cancer) (Citation28). The SPOT-MAS test detected cancer patients in a cohort of 285 patients and 222 healthy individuals, with a sensitivity of 73.9% (73.2–74.6%) and a specificity of 95.9% (95.8–96.0%) (Citation28). Furthermore, another study that further validate the performance of SPOT-MAS in a larger cohort of 833 cancer patients and 787 healthy individuals has been completed and the data are being prepared for publication.
This study aimed to assess the feasibility and performance of SPOT-MAS in a multi-center clinical trial setting. To do so, we launched a prospective cohort study named K-DETEK at 13 major hospitals and one research institute in Vietnam in April 2022. Here, we present an interim report for the K-DETEK study 6 months from initiation.
Materials and methods
Study design
K-DETEK is a multi-center, prospective cohort study recruiting participants aged 40 or older who present at the Outpatient Clinic of one of the 13 hospitals and research institutes in five regions in Vietnam for periodic follow-up visits for their chronic conditions including, hypertension, diabetes, dyslipidemia, and ischemic heart diseases or for routine annual health check-ups beginning in April 2022. The trial was registered with the U.S. National Institutes of Health (ClinicalTrials.gov identifier: NCT05227261). The study was reviewed and approved by the institutional ethics committee of the University of Medicine and Pharmacy, Ho Chi Minh City, Vietnam (approval number: 192/HĐĐĐ-ĐHYD) ().
Figure 1. Overall flow chart of study subject selection and follow-up of K-DETEK. K-DETEK is a multi-center, prospective cohort study recruiting participants aged 40 or older who present at the Outpatient Clinic of one of the 13 hospitals and research institutes in five regions in Vietnam for periodic follow-up visits for their chronic conditions or for annual health check-ups beginning in April 2022. The estimated number of participants to be enrolled in the study is 3000. The inclusion criteria include: (i) 40 years of age or older, (ii) have neither clinical suspicion of cancer nor history of confirmed cancer, (iii) agree to be contacted at 6 months and 12 months following enrollment to collect information about general health status and progress of cancer, (iv) have no history of blood transfusion or bone marrow transplantation within the past 3 years, and (v) have no clinical manifestation of dementia. Eligible participants provided 10 ml of blood for analyzing cfDNA by SPOT-MAS assay. After 30 days, each participant received the results of either “ctDNA signal detected” or “ctDNA signal not detected.” The study participants were scheduled for follow-up visits at 6 and 12 months. This study reported interim results of 2795 participants 6 months from study initiation.
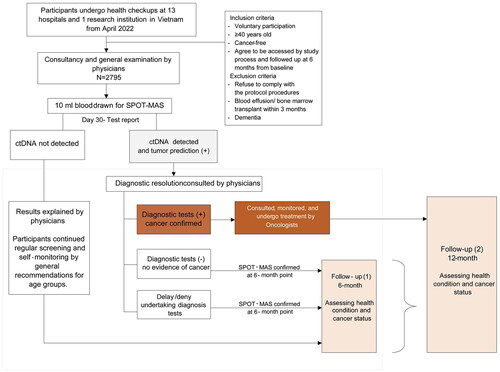
The estimated number of participants to be enrolled in the study is 3000. Physicians screened all participants for inclusion criteria including: (i) 40 years of age or older, (ii) have neither clinical suspicion of cancer nor history of confirmed cancer, (iii) agree to be contacted at 6 months and 12 months following enrollment to collect information about general health status and progress of cancer, (iv) have no history of blood transfusion or bone marrow transplantation within the past 3 years, and (v) have no clinical manifestations of dementia. Eligible participants provided 10 ml of blood for analyzing cfDNA by SPOT-MAS assay. Each participant received the results of either “ctDNA signal detected” or “ctDNA signal not detected.” For cases with detectable ctDNA signals, tumor origin was predicted by SPOT-MAS algorithms.
The study participants were scheduled for follow-up visits at 6 and 12 months. This study reported interim results from the K-DETEK prospective cohort study 6 months from initiation.
Collection of clinical information data
At enrollment, each participant was provided with clear information about the study purpose and process and a questionnaire about demographic information, personal medical history, family history, lifestyle habits, past major medical history, and clinical warning signs of cancers (Supplementary Appendix 1).
Collection of blood samples
The blood (10 ml) was collected from each participant in Streck Cell-Free DNA BCT tubes (Streck, USA) by trained physicians at different clinical sites and then transported at room temperature to the central lab within 5 days of collection (median: 2 days, range: 0–5 days). All samples were assigned a study identification number and were anonymized before being given to the investigators conducting the testing. Upon arrival at the lab, the blood hemolysis rate was assessed by trained personnels who performed visual inspection to compare the plasma with a color scale after centrifuging the samples. The hemolysis rates were measured as hemolysis indexes which correspond to serum hemoglobin (Hb) concentration. There were 5 cases with hemolysis index >100, which were rejected and blood resampling was requested. All resampled samples met the quality control and no participant was excluded from this study due to hemolysis.
Plasma cell-free DNA isolation
Blood samples were centrifuged twice (1600×g for 10 min at 4 °C and 16,000×g for 10 min at 4 °C) to separate plasma from cellular components. Plasma was then collected and stored at −80 °C in 2 ml aliquots. CfDNA was extracted using the MagMAX Cell-free DNA Isolation kit (Thermo Fisher, USA) on the KingFisher Flex Magnetic 96DW automated system following the manufacturer’s instructions. The resulting cfDNA was recovered and stored in a DNA Lobind tube (Eppendorf AG), held at −20 °C if not used immediately, and the cfDNA concentration was measured using the QuantiFluor dsDNA system (Promega, USA).
SPOT-MAS assay
The isolated cfDNA samples were subjected to the SPOT-MAS assay (patent pending), which was previously described (Citation28). The principle of SPOT-MAS assay was previously described (Citation29). Briefly, SPOT-MAS simultaneously analyze multiple signatures of ctDNA including methylation changes at 450 target regions (mostly derived from Chen et al. (Citation30)), genome-wide methylation profiles, and fragment length patterns. The details of SPOT-MAS procedure have been included in supplementary methods.
The performance of SPOT-MAS was assessed by computing the number of cases with a signal-detected (n, %), including true-positive values, false-positive values, cases without current diagnostic resolution, the number of negative cases (n, %), and positive predictive value (PPV) for cancer signal detection (%). TOO predictions were also reported, shown by its return rate (%) and overall prediction accuracy (%).
Informing participants of test results
The SPOS-MAS test result was released and sent to participants within 30 days of their return for their next check-up appointment. The reports would show whether they had “ctDNA-detected” or “ctDNA not detected.”
For participants with “ctDNA-detected,” physicians at the hospitals would consult with the participants regarding appropriate diagnostic tests related to the five cancer types, depending on the TOO probability values across five tumors of interest: liver, lung, breast, and colorectal and/or gastric. Predictions of cancer types not covered by the SPOT-MAS test were reported as “other cancers.” The proposed tests suggested by The National Comprehensive Cancer Network (NCCN) for cancer screening (Citation31–34) to confirm cancer origin are listed in . Participants who received an “other tumors” result were advised to undergo a health check-up with full-body CT scans as recommended by their physicians, and further decisions would be the responsibility of the clinical physicians (). For patients for whom 2 different types of cancer were predicted, the diagnostic test was recommended in a sequential manner based on the prediction probabilities.
Table 1. Imaging tests for “signal-detected” cases.
After being confirmed for cancer diagnosis, cancer patients were then followed-up at their 12-month (±1 week) point from baseline to collect information about their general health and cancer status.
The “ctDNA-detected” cases where imaging tests detected no abnormal results were also consulted about their results. Additionally, it was recommended that they re-take the SPOT-MAS test 6 months (±1 week) after their previous test to re-confirm the ctDNA cancer hallmarks due to the possible presence of a small tumor that could not be identified via imaging as well as to eliminate the chance of a false-positive signal due to changes in body physiology with rare diseases and/or infections. An interview was also conducted at 12 months (±1 week) post-test to assess participants’ cancer status and health condition.
Participants who received a “signal not detected” result were carefully consulted about their lack of risk for the five types of cancer investigated, as well as the risk for other cancer types not covered by the SPOT-MAS test. They were also advised to continue following the regular cancer screening programs and follow-up interviews conducted by our specialized physicians to confirm noncancer status at 6 months (±1 week) after enrollment.
Descriptive and statistical analysis
The participants’ characteristics at baseline are described in . Participants with high-risk factors (smoking, drinking, hepatitis B/C, FDR (First-Degree Relatives) diagnosed with two cancer types, FDR diagnosed with cancer at the age of younger than 45 or identified mutant carriers) were defined as “high-risk.” The remaining individuals were considered “moderate-risk” ().
Table 2. Clinical characteristics of 2795 study participants.
The effect of hemolysis rate, cfDNA concentration, and different location sites on the sequencing quality of the cfDNA in the plasma was analyzed using a two-sided Mann–Whitney–Wilcoxon test with Bonferroni correction (). The median cfDNA concentration across different patient groups, such as gender (male or female), age (<51 or ≥51), and level at risk (medium-risk or high-risk), were compared using a Wilcoxon Rank Sum test with p-values under 0.05 denoting a significant difference.
All statistical analyses were carried out using R (4.1.0) with standard data analysis packages and the ggplot2 package for visualization.
Results
Clinical characteristics of study participants
K-DETEK is a prospective, multi-center clinical study in which 3000 participants were recruited from 13 hospitals and one research institute in Vietnam between April 2022 and July 2022 (). At the time of the interim analysis, 2795 enrolled participants satisfied all criteria specified in the methods section. The clinical characteristics of eligible participants are summarized in , with a higher percentage of females than males (57.8% versus 42%) and a median age of 51 years, ranging from 40 to 88 years. High-risk individuals accounted for 31.7% (n = 887) of all participants. The remaining 68.3% (n = 1908) individuals were considered moderate risk.
Figure 2. Geographical location of participating centers and their contribution to the total participants in K-DETEK study. (A) K-DETEK is a prospective, multi-center clinical study that enrolled 2795 participants from 13 hospitals and 1 research institute in Vietnam between April 2022 and July 2022. These research centers were grouped into five regions including two major cities (Hanoi and HCMC) and three remote regions (Central, Southeast and Mekong Delta). (B) Pie chart showing the distribution of participants across five regions.
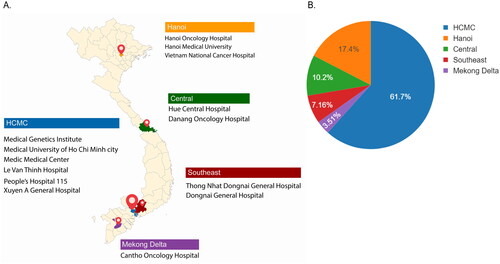
The study centers were grouped into five major regions: two metropolitan cities (Ho Chi Minh City [HCMC] and Hanoi) and three remote regions (Central, Southeast, and Mekong Delta). The majority of eligible participants were recruited from the two cities: HCMC (61.70%) and Hanoi (17.40%). The remaining participants were recruited from the central (10.20%), Southeast (7.16%), and Mekong Delta (3.51%) areas ().
Variations in logistic time and hemolysis rates of blood samples collected from different clinical sites and their impacts on cfDNA concentration and sequencing quality
All blood samples were collected in Streck Cell-Free DNA BCT tubes and transported to a central laboratory located in HCMC for cfDNA isolation, library preparation and sequencing. Due to variations in distance from collection sites to the central laboratory, the time from sample collection to processing varied from zero to five days for HCMC and Hanoi, while most samples (>70%) collected from the other regions (Central, Southeast, and Mekong Delta) were processed within three days of collection ().
Figure 3. The influences of sample processing time and plasma hemolysis on sequencing library yield of cfDNA. (A,B) Bar plots showing the differences in logistic time (A) and plasma hemolysis rate (B). (C,D) Violin plots showing the impact logistic time (C) and plasma hemolysis rate (D) on cfDNA amount per ml plasma. (E,F) Violin plots showing the impacts of sample processing time (E) and hemolysis rates (F) on the efficiency of library yields. Mann–Whitney–Wilcoxon test two-sided with Bonferroni correction was performed to compare the fold changes in DNA library yields across different patient groups. ns: not significant; *, p < 0.05; ****, p < 0.00001.
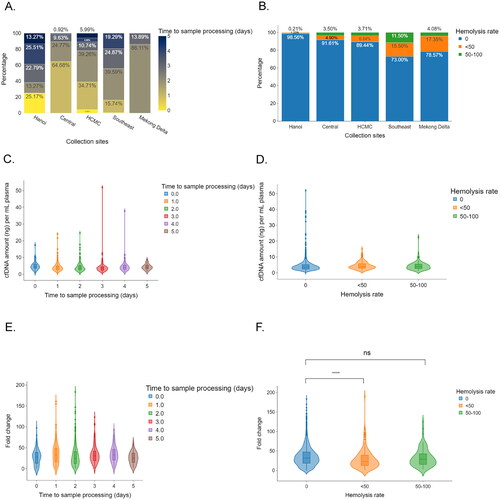
We noted that samples collected from remote areas, including Southeast and Mekong Delta, had higher hemolysis rates than those collected from HCMC (Southeast (27.00%) and Mekong Delta (21.43%) versus 10.56% for HCMC; ). Despite being distant from the central laboratory, Hanoi and central areas had lower proportions of hemolyzed samples than HCMC; 1.44% from Hanoi and 8.39% in central regions. ().
Delayed sample processing and increased hemolysis rate could negatively impact cfDNA yield and sequencing library preparation’s success rate. We did not observe any significant differences in cfDNA concentration among samples processed on the day of collection and those processed from day one post-collection onward (range, 3.6–4.5 ng/ml, p > 0.05, ). Likewise, there was no association between hemolysis rates and cfDNA concentration (, range, 3.6–3.9 ng/ml, p > 0.05). Next, the impact of sample processing time and hemolysis rate on sequencing library preparation efficiency was examined by computing fold changes in DNA library yield relative to input cfDNA amount. Although there was no correlation between sample processing time and cfDNA library preparation efficiency (, range, 24.5–33.1, p > 0.05), plasma quality showed varied impacts on library yields depending on hemolysis rates (, range, 23.2–31.9, p > 0.05,). Samples with a hemolysis rate of <50% had significantly lower fold-change in library yield compared to those without hemolysis (, 31.9 ng/ml vs. 23.2 ng/ml, p < 0.0001). This observation indicated that the plasma quality of collected samples, particularly the hemolysis rate, is an important determinant of DNA library efficacy.
Associations between participants’ clinical features and cfDNA levels
Previous studies have reported that differences in participant-specific physiological conditions could result in varying levels of circulating cfDNA, influencing library yield and sequencing quality (Citation35,Citation36). However, the results are often inconsistent across different studies (Citation36). Here, we examined such associations in the K-DETEK study. Male participants had significantly higher cfDNA levels than their female counterparts (, 3.9 ng/ml, IQR: 2.7–5.4 versus 3.3 ng/ml, IQR: 2.4–4.8 p < 0.00001, ). We also observed significantly higher plasma cfDNA levels in participants above the age of 51 compared to their younger counterparts (, 3.9 ng/ml, IQR: 2.7–5.7 versus 3.3 ng/ml, IQR: 2.4–4.5, p < 0.0001, ). These differences suggested that the baseline levels of cfDNA could vary by gender and age. When stratifying the samples by age and gender, we observed that female participants younger than 51 had a median cfDNA level of 3.0 ng/ml plasma (IQR: 2.1–4.2), the lowest cfDNA level among the participant groups ().
Figure 4. Association between participants’ clinical features and plasma cfDNA concentration. (A,B) Box plots showing the differences in cfDNA amount stratified by gender (A) and median age (B). (C) Box plots showing the differences in cfDNA stratified by both age and gender. (D) Box plots showing the differences in cfDNA concentration between medium risk and high-risk participants. Wilcoxon rank sum test performed to compare the median of cell free DNA amount across different patient groups. ***, p < 0.0001; ****, p < 0.00001.
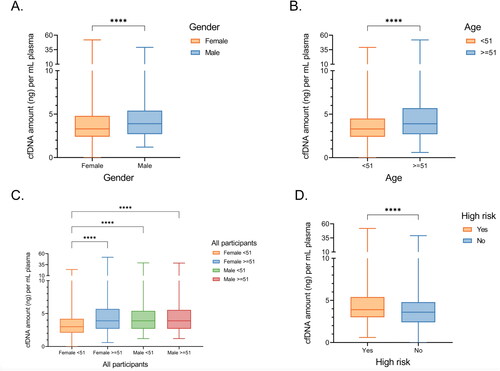
Table 3. Associations between participants’ clinical features and amount of cfDNA per ml plasma.
We also found that high-risk participants with one of the recorded cancer risk factors, including alcohol consumption, smoking, and chronic infection with HBV/HCV, had higher levels of cfDNA than those without these risk factors (, 3.9 ng/ml versus 3.6 ng/ml, p < 0.0001, ). Among those risk factors, we found that liver hepatitis was significantly correlated with differences in cfDNA levels. In contrast, other risk factors, including alcohol consumption or smoking, were not significantly associated with cfDNA levels (3.9 ng/ml versus 3.6 ng/ml, p < 0.00001, ). Thus, the present data showed that participants’ gender, age, and HBV/HCV infection status are important contributors to the variations in cfDNA levels.
SPOT-MAS test performance
To detect ctDNA and identify its TOO, the SPOT-MAS test simultaneously interrogates ctDNA specific signatures, including aberrant methylation, DNA copy number changes, and fragment length profiles using both targeted and genome-wide sequencing data. SPOT-MAS test results were obtained from all 2795 study participants ( and ).
Table 4. Cases with signal-detected reports.
Table 5. SPOT-MAS performance and tumor origin accuracy.
We identified 13/2795 cases (0.47%) having a signal of ctDNA by the SPOT-MAS test (). Of those cases, 10 participants agreed to undertake diagnostic tests for cancer types based on the prediction of TOO provided in the SPOT-MAS test report within 12 months, while 3 did not have diagnostic results at the time of writing this report due to delays in taking the diagnostic tests (n = 1), or declining to take the tests (n = 2). Among the ten participants with confirmed diagnostic results, six (60%) had six different types of cancers, including endometrial cancer, colorectal carcinoma, stomach adenocarcinoma, breast adenocarcinoma, cholangiocarcinoma, and hepatocellular carcinoma (HCC). All six confirmed cancer cases belonged to the moderate-risk group of participants. Five out of six had tumor types matched with either the first or second cancer type predicted by SPOT-MAS, suggesting an overall accuracy of 83.3% for TOO. Four cases with detectable ctDNA were confirmed to be cancer-free by diagnostic tests. Despite having negative results by diagnostic tests, we noted that two of the four cases with detected ctDNA displayed some abnormal lesions, with participant 7 having a hyperplastic sigmoid colon polyp and participant 10 having a right hepatic cyst and a left functional ovarian cyst (). Thus, follow-ups at 6 months and 12 months following diagnosis will be required to confirm their noncancer status. Together, our real-world analysis conducted on 2795 SPOT-MAS results revealed a detection rate of 0.47%, PPV of 60% for cancer detection, and an accuracy of 83.3% for TOO identification in a population with previously unknown cancer status ().
Case report
A 60-year-old male came to Medical Genetics Institute and volunteered to participate in the K-DETEK study. He reported a history of hypertension and type 2 diabetes, both well-controlled. The patient had no warning symptoms of cancer, no history of cancer, and no family history related to cancer. He rarely underwent health check-ups and self-reported being a heavy drinker.
He was screened by a physician and determined to satisfy all inclusion and exclusion criteria of the K-DETEK study. Then he was invited to voluntarily join the study in June 2022 and undertake the SPOT-MAS test. One month after undergoing SPOT-MAS, his report showed “ctDNA signal-detected,” predicting the possibility of ctDNA from liver cancer (75%) and/or colorectal cancer (23%) (). The participant was informed of his SPOT-MAS test results and underwent diagnostic tests for liver cancer within a month. The ultrasound presented in revealed a tumor measuring 3.5 × 3.3 cm in the lower segment VIII of the liver, suggesting Stage IB (T1b-N0-M0) hepatocellular carcinoma (HCC). His CT scan image identified a space-occupying lesion in segment VIII, strongly supporting the diagnosis of early-stage HCC (). After these results were carefully reviewed and discussed, the patient was transferred to an oncologist for minimally invasive treatment by a combination of TACE and RFA, a non-surgical optimized method with good efficacy and safety suitable only for patients with early-stage tumors. After receiving treatment, he was scheduled for a follow-up at 12 months from the study baseline. Thus, our case report supports using SPOT-MAS as a complementary method to achieve early detection and tumor location prediction and provide early cancer treatment opportunities.
Figure 5. SPOT-MAS and confirmed diagnosis tests result of a case with detectable ctDNA in K-DETEK study. (A) A 60-year-old male (case#5) with unknown cancer status or symptoms was enrolled in K-DETEK study. His SPOT-MAS test was “ctDNA detected” and predicted to have liver cancer or colorectal cancer with probabilities of 75% and 25%, respectively. (B) After receiving the SPOT-MAS test result, the patient was recommended to undertake an ultrasound test, which revealed a hypo-echoic mass of 3.5 × 3.3 cm located in right lobe segment VIII of the liver, suspecting of hepatocellular carcinoma. His following CT-scan confirmed the presence of tumor in the same location.
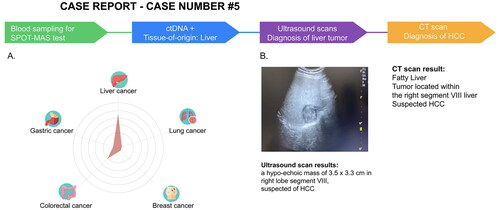
Discussion
K-DETEK is a prospective trial aiming to recruit 3000 participants from 13 major hospitals and one research institute in Vietnam to validate the performance of the SPOT-MAS test in clinical settings. Our interim analysis of 2795 eligible participants showed the feasibility of SPOT-MAS for testing samples collected from multiple clinical sites and its ability to detect cancer in asymptomatic participants in early stages when they may benefit from early treatment.
For a multi-center trial, it is essential to ensure the consistency of sample quality across different study sites. Hence, we first investigated factors that could contribute to variations in sample quality, which was assessed by plasma cfDNA yield and sequencing library efficacy. We did not observe any noticeable impact of sample processing time, from 0 to 5 days, on cfDNA concentrations or sequencing library yields. However, variations in the hemolysis rate of collected samples across research sites, possibly due to poor blood collection techniques, significantly affect library efficacy and subsequent quality of genome-wide sequencing. This suggested that homogeneity of blood sampling techniques across different clinical sites is required for consistency of sequencing quality in large-scale, multi-center screening and that appropriate training for clinicians on how to perform venipuncture using Streck cfDNA BCT tubes is important for our future extended K-DETEK study.
Consistent with previous studies, we observed significant associations between participants’ gender and age and cfDNA levels (Citation37,Citation38). Interestingly, we found that participants with chronic infection with HBV or HCV had significantly higher levels of cfDNA. Our findings suggested that future studies are required to assess the influence of patients’ clinical information on the performance of SPOT-MAS.
At the time of this interim analysis of K-DETEK, we detected 13 (0.47%) cases with a ctDNA signal in their blood out of 2795 healthy participants. Of those, the number of true positive cases was 6, indicating a PPV of 60%. At the time of receiving SPOT-MAS test result, case 3 had epigastric pain and his physician advised him to undertake endoscopy to clarify the symptom of gastric pain first instead of undergoing a CT-scan with contrast for cancer detection. His endoscopy results confirmed the stomach cancer status. For the four participants with the imaging diagnosis tests unable to confirm the presence of tumors, we noted that two displayed some abnormal lesions, with participant 7 having a hyperplastic sigmoid colon polyp and participant 10 having a right hepatic cyst and a left functional ovarian cyst (). At the time of submission, four participants with detectable ctDNA but not being confirmed by diagnostic tests have not reached the 6 month follow-up. Hence, the reevaluation data of those cases are not currently available. We acknowledge that the missing of confirmed diagnosed tests for these four cases is a limitation of our study. In addition, the number of false-positive cases was too small to draw a reliable statistical conclusion. However, we did not observe any apparent association between the false-positive status and the tested factors including hemolysis rate, cfDNA concentration and collection sites (Supplementary Table S1).
Most conventional methods for cancer screening to date have focused on a particular cancer type, leading to higher cumulative false-positive rates (Citation39). By contrast, incorporating multi-cancer screening in one test could increase the overall PPV of the screening method (Citation40). Other trial studies, such as DETECT-A and PATHFINDER, have been conducted to validate the clinical performance of multi-cancer screening tests. The DETECT-A study evaluated the combination of the CancerSEEK blood test for detecting ctDNA and subsequent PET-CT test for TOO identification on 10,006 participants and reported 26 cases with detectable ctDNA signal and a PPV of 28.3%. In the PATHFINDER study, the multi-cancer early detection (MCED) blood test that can detect >50 types of cancers was validated on 6621 healthy individuals (Citation41). The interim analysis of the PATHFINDER study reported a detection rate of 1.4% (92 cases) and a PPV of 43.1%. Thus, our research and others consistently show that an LB test based on the detection of ctDNA could prospectively identify cancers in asymptomatic individuals whose cancer status was previously unknown. Furthermore, the case reported in the K-DETEK study provided important support for the clinical relevance of the SPOT-MAS assay in detecting cancer at an early stage when the patient can benefit from early treatment.
We reported a lower detection rate but higher PPV than other studies. The differences in detection rates across the three studies could be due to the differences in study recruitment criteria. The K-DETEK study recruited all participants from the age of 40, with a median age of 51, while in other studies, the inclusion criteria of participants’ age were over 50 (MCED) (Citation42,Citation43) or from 65 to 75 years (CancerSEEK) (Citation44), populations that might have a higher incidence of cancers. Additionally, our study achieved a higher PPV (60%) compared to CancerSEEK (28.3%) and PATHFINDER (38.0%). The higher PPV found in the SPOT-MAS assay used in the K-DETEK study could be because this study interrogates multiple features of ctDNA, including methylation and fragment length profile in a single library reaction, while CancerSEEK and GALLERI focus on either mutations or methylation markers. The strategy of integrating different signatures of ctDNA in an ensemble learning model to improve cancer prediction has been shown to be effective in many types of cancer. It was shown that the integration of fragment length profile and DNA copy number aberration (CNA) or methylation and CNA in a prediction model improved liver cancer detection (Citation45,Citation46). Consistently, we previously showed that a combination of genome-wide methylation and fragment length profiles into a machine learning model could enhance the performance in detecting early-stage colorectal cancer (Citation29) or breast cancers (manuscript under review). Hence, a multimodal approach involving simultaneous analysis of multiple ctDNA signatures may overcome the challenges of low abundance and molecular heterogeneity of ctDNA in the bloodstream. Additionally, compared to CancerSEEK, which relies on other companion assays using serum biomarkers to determine the tissue location of cancers, SPOT-MAS could correctly predict TOO for five out of six cases with detectable ctDNA based on the top two prediction labels of SPOT-MAS.
There are several limitations in this study. First, the sample size of this study was relatively small compared to other trials, such as PATHFINDER and DETECT-A (Citation42–44). Second, at the time of this paper’s submission, three participants with detectable ctDNA signals had declined or postponed cancer diagnosis tests. This hesitation by participants highlights potential psychological impacts among positive cases, which was not investigated in this study. Third, it is important to note that current liquid biopsy tests should only be used as a complementary approach to the available diagnosis tests to increase rates of cancer detection, especially in early stages. To be used as a stand-alone test, further work is required to improve its performance, with more focus on increasing sensitivity while maintaining high specificity. Last but not least, most participants have not reached the 12-month follow-up point. Thus, a final report is underway to confirm the cancer status for cases with “signal not detected” or those with detectable ctDNA but not yet confirmed by diagnostic tests to precisely estimate the PPV and NPV. However, this interim report is to timely present our important findings to researchers and clinicians working in the oncology field. These findings have major implications for incorporating a multi-cancer blood test into a national cancer screening program which is currently not available in many low- and middle-income countries.
Finally, the cancer types detected by SPOT-MAS were limited to the top five common cancer types in Vietnam. Patients with rarer cancer types would be missed by our assay. To address these limitations, we are conducting an extended K-DETEK study, which aims to recruit 10,000 additional participants to further validate the performance of SPOT-MAS in a larger cohort. Furthermore, the patients’ attitude toward cancer screening, the psychological impacts of receiving positive results, the cost-effectiveness, and the clinical benefit to patients’ survival rates will also be investigated in this larger study.
In conclusion, our study has demonstrated the clinical application of a multi-cancer blood test in a low- and middle-income country like Vietnam, where a nationwide cancer screening program is urgently needed but currently not available. Beyond detecting the presence of cancer signals, our test was able to predict the tumor location, allowing clinicians to fast-track the follow-up diagnostic and guide any necessary treatment. Our experience led us to believe that it is feasible to implement a multi-cancer screening assay such as one reported here in a national screening program to provide early detection and better prognosis for cancer patients in Vietnam. However, more work is needed before such program becomes a reality. First, data from larger trials are required to fully assess the accuracy and cost effectiveness of our test. Second, future studies will be needed to determine who will be best benefit from the test and how frequent the test should be carried out. Finally, we will need to demonstrate the clinical benefits of our test outweigh potential psychological and behavioral sequelae associated with positive results. Despite these challenges, we are optimistic that within the next 5 years our test, along with other multi-cancer early detection tests, will be available in clinical practices and implemented in screening programs across multiple nations.
Author contributions
Conceptualization, Van Hoi Le, Vinh Quang Bui, Lan Hieu Nguyen, Nhu Hiep Pham, Thanh Hai Phan, Huu Thinh Nguyen, Van Song Tran, Chi Viet Bui, Van Kha Vo, Nhan Pham Thanh Nguyen, Ha Huu Phuoc Dang, Van Dung Pham, Van Thinh Cao, Huu Nguyen Nguyen, Duy Sinh Nguyen, Thi Quynh Tho Nguyen, Thanh-Thuy Thi Do, Dinh-Kiet Truong, Hung Sang Tang, Minh Duy Phan, Hoa Giang, Hoai Nghia Nguyen, Le Son Tran, Van Tung Nguyen, Thi Le Quyen Le, Thi Lan Anh Luong, Van Bau Phan, Quang Binh Truong, Thi Huong Ly Tran, Minh Thien Huynh, Tu Quy Tran, Si Tuan Nguyen, Vu Tran, Van Khanh Tran; Patient consultancy and screening, Van Hoi Le, Vinh Quang Bui, Lan Hieu Nguyen, Nhu Hiep Pham, Thanh Hai Phan, Huu Thinh Nguyen, Van Song Tran, Chi Viet Bui, Van Kha Vo, Nhan Pham Thanh Nguyen, Ha Huu Phuoc Dang, Van Dung Pham, Van Thinh Cao, Van Tung Nguyen, Thi Le Quyen Le, Thi Lan Anh Luong, Van Bau Phan, Quang Binh Truong, Thi Huong Ly Tran, Minh Thien Huynh, Tu Quy Tran, Si Tuan Nguyen, Vu Tran, Van Khanh Chan, Thi Kim Phuong Doan, Thi Trang Dao, Canh Duy Phan, Thanh Xuan Nguyen, Nguyen Tuong Pham, Bao Toan Nguyen, Thi Thu Thuy Pham, Huu Linh Le, Cong Thanh Truong, Thanh Xuan Jasmine, Minh Chi Le; Formal analysis, Thanh Dat Nguyen, Luu Hong Dang Nguyen, Ngoc Minh Phan, Trong Hieu Nguyen, Thien Chi Van Nguyen, Thi Mong Quynh Pham, Vu Uyen Tran, Minh Phong Le, Dac Ho Vo, Thi Minh Thu Tran, Minh Nguyen Nguyen, Thi Thanh Nguyen, Ba Linh Tieu; Data curation, Thi Van Phan, Huu Tam Phuc Nguyen, Dinh Yen An Truong, Chi Thuy Tien Cao. Funding acquisition, Hoa Giang, Hoai Nghia Nguyen; Writing manuscript draft and editing, Thi Hue Hanh Nguyen, Y-Thanh Lu; Le Son Tran, Minh Duy Phan.
Supplemental Material
Download PDF (320.9 KB)Acknowledgments
We thank our K-DETEK participants for their courage and generosity. We thank Angela Marie Jansen, PhD, MHS of Angela Jansen and Associates, for her help in editing this manuscript.
Disclosure statement
The authors including LST, HNN, HG, MDP, HHN and DSN hold equity in Gene Solutions. The funder Gene Solutions provided support in the form of salaries for authors THHN, YTL, TDN, LHDN, NMP, THN, TCVN, TMQP, VUT, MPL, DHV, TMTT, MNN, TTN, BLT, HTPN, DYAT, CTTC, HNN, DSN, TQTN, TVP, TTTD, DKT, HST, MDP, HG, HNN and LST. NHN, HG, MDP and LST are inventors on the patent application (USPTO 17930705). We also confirm that this does not alter our adherence to Cancer Investigation policies on sharing data and materials.
Data availability statement
Sequencing data will be deposited in a public portal database (NCBI SRA) upon acceptance and are available on request from the corresponding author, LST. The data are not publicly available due to ethical restrictions.
Additional information
Funding
References
- Organization WH. Fact sheets – cancer 2022. [updated 2022 Feb 3; cited 2022 Nov 23]. Available from: https://www.who.int/news-room/fact-sheets/detail/cancer.
- Sung H, Ferlay J, Siegel RL, Laversanne M, Soerjomataram I, Jemal A, Bray F. Global cancer statistics 2020: GLOBOCAN estimates of incidence and mortality worldwide for 36 cancers in 185 countries. CA Cancer J Clin. 2021;71(3):209–249. doi:10.3322/caac.21660.
- WHO. Global cancer observatory: cancer today. 2020. [cited 2022 Jul 25]. https://gco.iarc.fr/
- Pham T, Bui L, Kim G, Hoang D, Tran T, Hoang M. Cancers in Vietnam-burden and control efforts: a narrative scoping review. Cancer Control. 2019;26(1):1073274819863802.
- WHO. Cancer control: knowledge into action: WHO guide for effective programmes: module 3: early detection. Geneva: World Health Organization; 2007.
- Davidson KW, Barry MJ, Mangione CM, Cabana M, Caughey AB, Davis EM, et al. Screening for colorectal cancer: US Preventive Services Task Force recommendation statement. JAMA. 2021;325(19):1965–1977.
- Curry SJ, Krist AH, Owens DK, Barry MJ, Caughey AB, Davidson KW, et al. Screening for cervical cancer: US Preventive Services Task Force recommendation statement. IAMA. 2018;320(7):674–686.
- Screening for breast cancer: U.S. Preventive Services Task Force recommendation statement. Ann Intern Med. 2016;164(4):279–296. doi:10.7326/M15-2886.
- Force USPST. Screening for lung cancer: US Preventive Services Task Force recommendation statement. JAMA. 2021;325(10):962–970.
- Grossman DC, Curry SJ, Owens DK, Bibbins-Domingo K, Caughey AB, Davidson KW, et al. Screening for prostate cancer: US Preventive Services Task Force recommendation statement. JAMA. 2018;319(18):1901–1913. doi:10.1001/jama.2018.3710.
- Ebell MH, Bentivegna M, Hulme C. Cancer-specific mortality, all-cause mortality, and overdiagnosis in lung cancer screening trials: a meta-analysis. Ann Fam Med. 2020;18(6):545–552. doi:10.1370/afm.2582.
- Schoenborn NL, Sheehan OC, Roth DL, Cidav T, Huang J, Chung SE, et al. Association between receipt of cancer screening and all-cause mortality in older adults. JAMA Netw Open. 2021;4(6):e2112062. doi:10.1001/jamanetworkopen.2021.12062.
- Stang A, Jöckel KH. The impact of cancer screening on all-cause mortality. Dtsch Arztebl Int. 2018;115(29-30):481–486.
- Gini A, Jansen EEL, Zielonke N, Meester RGS, Senore C, Anttila A, et al. Impact of colorectal cancer screening on cancer-specific mortality in Europe: A systematic review. Eur J Cancer. 2020;127:224–235. doi:10.1016/j.ejca.2019.12.014.
- Yan YY, Guo QR, Wang FH, Adhikari R, Zhu ZY, Zhang HY, et al. Cell-free DNA: hope and potential application in cancer. Front Cell Dev Biol. 2021;9:639233.
- Campos-Carrillo A, Weitzel JN, Sahoo P, Rockne R, Mokhnatkin JV, Murtaza M, et al. Circulating tumor DNA as an early cancer detection tool. Pharmacol Ther. 2020;207:107458. doi:10.1016/j.pharmthera.2019.107458.
- Tran LS, Nguyen QT, Nguyen CV, Tran VU, Nguyen TT, Le HT, et al. Ultra-deep massive parallel sequencing of plasma cell-free DNA enables large-scale profiling of driver mutations in vietnamese patients with advanced non-small cell lung cancer. Front Oncol. 2020;10:1351. doi:10.3389/fonc.2020.01351.
- Nguyen Hoang V-A, Nguyen ST, Nguyen TV, Pham TH, Doan PL, Nguyen Thi NT, et al. Genetic landscape and personalized tracking of tumor mutations in Vietnamese women with breast cancer. Mol Oncol. 2023. doi:10.1002/1878-0261.13356.
- Alix-Panabières C, Pantel K. Clinical applications of circulating tumor cells and circulating tumor DNA as liquid biopsy. Cancer Discov. 2016;6(5):479–491. doi:10.1158/2159-8290.CD-15-1483.
- Use of circulating tumor DNA for EarlyStage solid tumor drug development guidance for industry. 2022. Available from: https://www.fda.gov/regulatory-information/search-fda-guidance-documents/use-circulating-tumor-deoxyribonucleic-acid-early-stage-solid-tumor-drug-development-draft-guidance.
- Cohen JD, Li L, Wang Y, Thoburn C, Afsari B, Danilova L, et al. Detection and localization of surgically resectable cancers with a multi-analyte blood test. Science. 2018;359(6378):926–930. doi:10.1126/science.aar3247.
- Nguyen HT, Luong BA, Tran DH, Nguyen TH, Ngo QD, Le LGH, et al. Ultra-deep sequencing of plasma-circulating DNA for the detection of tumor-derived mutations in patients with nonmetastatic colorectal cancer. Cancer Invest. 2022;40(4):354–365. doi:10.1080/07357907.2021.2017951.
- Croessmann S, Park BH. Circulating tumor DNA in early-stage breast cancer: new directions and potential clinical applications. Clin Adv Hematol Oncol. 2021;19(3):155–161.
- Razavi P, Li BT, Brown DN, Jung B, Hubbell E, Shen R, et al. High-intensity sequencing reveals the sources of plasma circulating cell-free DNA variants. Nat Med. 2019;25(12):1928–1937. doi:10.1038/s41591-019-0652-7.
- Cristiano S, Leal A, Phallen J, Fiksel J, Adleff V, Bruhm DC, et al. Genome-wide cell-free DNA fragmentation in patients with cancer. Nature. 2019;570(7761):385–389. doi:10.1038/s41586-019-1272-6.
- Liu MC, Oxnard GR, Klein EA, Swanton C, Seiden MV, Liu MC, et al. Sensitive and specific multi-cancer detection and localization using methylation signatures in cell-free DNA. Ann Oncol. 2020;31(6):745–759. doi:10.1016/j.annonc.2020.02.011.
- Klein EA, Richards D, Cohn A, Tummala M, Lapham R, Cosgrove D, et al. Clinical validation of a targeted methylation-based multi-cancer early detection test using an independent validation set. Ann Oncol. 2021;32(9):1167–1177. doi:10.1016/j.annonc.2021.05.806.
- Hieu NT, Hai PT, Thuy PTT, Xuan JT, Chu NV, Huong TTT, et al. ESTABLISHMENT OF THE SPOT-MAS LISTENCE PROCESS TO Detect Many Types of Cancer FROM EARLY STAGE. VMJ [Internet]. April 27, 2022 [cited 2023 Feb 1]; 513(1). Available from: https://tapchiyhocvietnam.vn/index.php/vmj/article/view/2360.
- Nguyen HT, Khoa Huynh LA, Nguyen TV, Tran DH, Thu Tran TT, Khang Le ND, et al. Multimodal analysis of ctDNA methylation and fragmentomic profiles enhances detection of nonmetastatic colorectal cancer. Future Oncol. 2022;18(35):3895–3912. doi:10.2217/fon-2022-1041.
- Chen X, Gole J, Gore A, He Q, Lu M, Min J, et al. Non-invasive early detection of cancer four years before conventional diagnosis using a blood test. Nat Commun. 2020;11(1):3475. doi:10.1038/s41467-020-17316-z.
- Wood DE, Kazerooni EA, Baum SL, Eapen GA, Ettinger DS, Hou L, et al. Lung cancer screening, version 3.2018, NCCN clinical practice guidelines in oncology. J Natl Compr Canc Netw. 2018;16(4):412–441. doi:10.6004/jnccn.2018.0020.
- Bevers TB, Helvie M, Bonaccio E, Calhoun KE, Daly MB, Farrar WB, et al. Breast cancer screening and diagnosis, version 3.2018, NCCN clinical practice guidelines in oncology. J Natl Compr Canc Netw. 2018;16(11):1362–1389. doi:10.6004/jnccn.2018.0083.
- Benson AB, Venook AP, Al-Hawary MM, Cederquist L, Chen YJ, Ciombor KK, et al. Rectal cancer, version 2.2018, NCCN clinical practice guidelines in oncology. J Natl Compr Canc Netw. 2018;16(7):874–901. doi:10.6004/jnccn.2018.0061.
- Benson AB, 3rd, Venook AP, Cederquist L, Chan E, Chen YJ, Cooper HS, et al. Colon cancer, version 1.2017, NCCN clinical practice guidelines in oncology. J Natl Compr Canc Netw. 2017;15(3):370–398. doi:10.6004/jnccn.2017.0036.
- Hummel EM, Hessas E, Müller S, Beiter T, Fisch M, Eibl A, et al. Cell-free DNA release under psychosocial and physical stress conditions. Transl Psychiatry. 2018;8(1):236. doi:10.1038/s41398-018-0264-x.
- Yuwono NL, Warton K, Ford CE. The influence of biological and lifestyle factors on circulating cell-free DNA in blood plasma. Elife. 2021; 10:10. doi:10.7554/eLife.69679.
- Alghofaili L, Almubarak H, Gassem K, Islam SS, Coskun S, Kaya N, Karakas B. Cell-free DNA levels of twins and sibling pairs indicate individuality and possible use as a personalized biomarker. PLoS One. 2019;14(10):e0223470. doi:10.1371/journal.pone.0223470.
- Jylhävä J, Kotipelto T, Raitala A, Jylhä M, Hervonen A, Hurme M. Aging is associated with quantitative and qualitative changes in circulating cell-free DNA: the vitality 90+ study. Mech Ageing Dev. 2011;132(1-2):20–26. doi:10.1016/j.mad.2010.11.001.
- Braunstein GD, Ofman JJ. Criteria for evaluating multi-cancer early detection tests. touchREVIEWS Oncol Haematol. 2021;17(1):3–6. doi:10.17925/OHR.2021.17.1.3.
- Ahlquist DA. Universal cancer screening: revolutionary, rational, and realizable. NPJ Precis Oncol. 2018;2:23. doi:10.1038/s41698-018-0066-x.
- Liu MC. Transforming the landscape of early cancer detection using blood tests—commentary on current methodologies and future prospects. Br J Cancer. 2021;124(9):1475–1477. doi:10.1038/s41416-020-01223-7.
- Nadauld LD, McDonnell CH, Beer TM, Liu MC, Klein EA, Hudnut A, et al. The PATHFINDER study: assessment of the implementation of an investigational multi-cancer early detection test into clinical practice. Cancers. 2021; 13(14):3501. doi:10.3390/cancers13143501.
- Abstract 903O ‘A prospective study of a multi-cancer early detection blood test’ was presented by Deb Schrag during the proffered paper session “Basic science and translational research”: Annals of Oncology. 2022. [cited 2022 Oct]. Available from: https://oncologypro.esmo.org/meeting-resources/esmo-congress/a-prospective-study-of-a-multi-cancer-early-detection-blood-test.
- Lennon AM, Buchanan AH, Kinde I, Warren A, Honushefsky A, Cohain AT, et al. Feasibility of blood testing combined with PET-CT to screen for cancer and guide intervention. Science. 2020;369(6499):eabb9601. doi:10.1126/science.abb9601.
- Meng Z, Ren Q, Zhong G, Li S, Chen Y, Wu W, et al. Noninvasive detection of hepatocellular carcinoma with circulating tumor DNA features and α-fetoprotein. J Mol Diagn. 2021;23(9):1174–1184.
- Mouliere F, Chandrananda D, Piskorz AM, Moore EK, Morris J, Ahlborn LB, et al. Enhanced detection of circulating tumor DNA by fragment size analysis. Sci Transl Med. 2018;10(466):eaat4921.