Abstract
Background
New biomarkers of progression in patients with prostate cancer (PCa) are needed to improve their classification and clinical management. This systematic review investigated the relationship between single nucleotide polymorphisms (SNPs) and PCa progression.
Methods
A keyword search was performed in Pubmed, EMBASE, Scopus, Web of Science, and Cochrane for publications between 2007 and 2022. We included articles with adjusted and significant associations, a median follow-up greater than or equal to 24 months, patients taken to radical prostatectomy (RP) as a first therapeutic option, and results presented based on biochemical recurrence (BCR).
Results
In the 27 articles selected, 73 SNPs were identified in 39 genes, organized in seven functional groups. Of these, 50 and 23 SNPs were significantly associated with a higher and lower risk of PCa progression, respectively. Likewise, four haplotypes were found to have a significant association with PCa progression.
Conclusion
This article highlights the importance of SNPs as potential markers of PCa progression and their possible functional relationship with some genes relevant to its development and progression. However, most variants were identified only in cohorts from two countries; no additional studies reproduce these findings.
Introduction
In 2020, more than 1.4 million new prostate cancer (PCa) cases were diagnosed worldwide, ranking second in cancer incidence in the male population, and It was responsible for 370,000 deaths (Citation1). In 2023 the United States is expected to witness 288,300 new cases of prostate cancer and 34,700 related deaths (Citation2). The implementation of prostate-specific antigen (PSA)-based PCa screening and digital rectal examination have increased the incidence of mainly indolent tumors that can lead to overtreatment. PCa progression can be detected by the presence of biochemical recurrence (BCR), defined as an increase in PSA after treatment (e.g., PSA ≥ 0.2 ng/ml after radical prostatectomy (RP)) (Citation3).
There are different scales to establish the risk of progression, including Gleason score, preoperative PSA, and clinical stratification of tumors, among other factors (Citation4). An example is the D’Amico scale, which classifies the risk of BCR after localized PCa treatment as low, intermediate, or high, presenting a 5-year relapse-free survival rate of 94.5%, 76.6%, and 54.6%, respectively (Citation5,Citation6). In this sense, more than 50% of patients with localized PCa, even at high risk, will not progress, which makes it necessary to identify new elements to assess PCa progression and avoid unnecessary treatments with unwanted side effects.
Some studies have explored the molecular classification of PCa, identifying subtypes and biomarkers that may contribute to a more accurate prognosis (Citation7). Single nucleotide polymorphisms (SNPs) are variations in the DNA sequence that affect a single base and occur in at least 1% of the population (Citation8). Around 100 SNPs have been associated with the risk of developing PCa (Citation9,Citation10), and a significant association has been shown between the presence of these markers and disease progression. That is why the present systematic review examined the relationship between SNPs and PCa progression in patients who underwent RP as their first therapeutic option. In contrast to other studies, here we incorporate not only biologically plausible variables associated with PCa, and a schematic functional integration of the different genes involved was also carried out in prostate cells, androgen-producing cells and hepatocytes.
Methodology
Databases and search
A search was performed for scientific articles published in the last 16 years (2007-2022) in five databases: Pubmed, EMBASE, Scopus, Web of Science, and Cochrane. The search strategy included terms associated with PCa, BCR, and SNPs based on Medical Subject Headings (MeSH) terms located in the title, abstract, or keywords of the studies (Supplementary Table 1).
Inclusion and exclusion criteria
This review considered the following criteria for the inclusion of articles:
Population: Individuals with localized PCa taken to RP without other previous treatments.
Follow-up: Studies with a median follow-up of 24 months or longer.
Results: Only studies presenting their results based on BCR were included.
Likewise, articles meeting any of these criteria were excluded:
They used healthy individuals or patients with benign prostatic hyperplasia as a comparison group.
Articles without a significant association.
They did not evaluate associations using multivariate models, adjusted at least for age, PSA, and Gleason score.
Selection of studies
The selection of articles was made by four reviewers based on the inclusion and exclusion criteria described above: first by title, then by abstract, and finally by complete content. Likewise, articles with inconsistencies in the classification of risks and progression, the analysis of association measures, the genetic models used, and the data presented in the results were excluded.
Data extraction and synthesis
The following data were collected from each article: country of origin of the population, number of participants, type of study performed, participant selection criteria, central tendency measures, type of tissue studied, BCR percentage, and genotyping method. Subsequently, genes and SNPs with significant association with BCR, alleles, the corresponding genotype, and the type of outcome evaluated (differentiating between BCR and BCR-free time or progression) were extracted. Similarly, the gene analysis model, relationship with the outcome (risk or protection), variables included in the multivariate model, hazard ratio (HR), 95% confidence interval (CI 95%), and p-value were also collected.
Results
General characteristics of the articles
The flow chart of this study is shown in . In the initial search, 877 articles were obtained, of which 22 (11–32) were finally selected. Other 5 (33–37) articles were identified by snowball method, reaching a total of 27 articles evaluated. Supplementary Table 2 presents all data collected from these articles. All of them were cohort studies that analyzed a median of 458 (range: 126-846) individuals and had a median follow-up of 54 (range: 24-88.8) months. In all studies, the outcome was measured in BCR or time to BCR, with a median BCR of 36.1% (range: 22.4-42.6%) in the cohorts. Most SNPs were identified in genes with biological plausibility for PCa. Genotyping was performed on blood samples from patients, and in most cases, mass spectrometry was employed; three studies used a Taqman real-time PCR method, one used genotyping arrays, and one used PCR-RFLP.
The 27 articles included in the study were conducted in Canada (n = 4), Taiwan (n = 20), or China (n = 3). Ten cohorts were identified in these 27 articles, so there were different studies that evaluated the same cohort. All included studies performed a multivariate Cox regression model, evaluating variables such as age at diagnosis, PSA value, Gleason score, and staging; Some articles also took into account surgical margin compromise (n = 18), lymph node involvement (n = 12), smoking (n = 4), and the use of adjuvant therapy (n = 4). A single article included extraprostatic extension, vascular and perineural invasion, and tumor multifocality (Supplementary Table 2).
SNPs associated with PCa progression
The analysis of 27 articles identified 73 SNPs in 39 genes, as presented in . Of these 73 SNPs, 50 were associated with a higher and 23 with a lower risk of PCa progression. The 39 identified genes were classified into seven groups according to their primary association with the disease.
Table 1. SNPs associated with PCa progression classified according to the function of the genes in which they are located.
The first group involves genes related to sex steroid hormones, with 45 SNPs in 15 genes. The second one is linked to metabolism unrelated to sex steroid hormones, encompassing 6 SNPs in 5 genes. From the third to the sixth group, genes were classified according to specific cancer traits (Citation37,Citation38). The third group covers signaling pathways, bringing together functions like cell proliferation, apoptosis, immunomodulation, migration, and survival. In this group, 6 SNPs were identified in 6 genes. The fourth group contains genes related to cell proliferation, with 5 SNPs identified in 5 genes. The fifth group corresponds to cell adhesion, with 4 SNPs in 2 genes. The sixth group reports on autophagy, in which 3 SNPs were found in 2 genes. Finally, in the seventh group 4 SNPs were identified in 4 genes, and It includes genes related to functions such as embryonic development, DNA repair, and immune response.
Discussion
Identifying new biomarkers of progression in patients with PCa is very important to improve their clinical management. Although some reviews have studied the association between SNPs and PCa progression (Citation39,Citation40), to our knowledge, this is the first systematic review with a search not restricted to a group of specific variants and which included patients who underwent RP. The latter is relevant since RP does not cause a direct alteration in tumor cell evolution and reduces the heterogeneity of other therapeutic regimens. We exclusively included studies in which the control group consisted of cancers that did not progress, enabling us to effectively evaluate the disease’s progression. Progression was measured as BCR, given that PSA is a widely used follow-up parameter in patients with PCa. The median follow-up ranged from 24 to 88.8 months, which could imply that studies with a shorter follow-up time did not capture all individuals who were to progress. Given the origin of the cohorts, they possibly included almost exclusively Caucasian patients from Canada and Asians from China and Taiwan. There is also no reference of patients with African ancestry, who are underrepresented in these two regions. This is relevant because of differences based on ancestry in incidence, mortality, and mutational profile reported in PCa.
The studies included in this systematic review performed a targeted search for SNPs in genes chosen according to their biological plausibility with PCa progression, and they were conducted by a few groups of researchers. In these studies, there is a clear interest in SNPs located in genes related to sex steroid hormones, which received the most attention and, therefore, where the majority of the associations were found. These types of studies have the limitation that they will only identify associations with pre-selected variants, eliminating the possibility of discovering other novel associations, which could happen in genome-wide association studies (GWAS) instead. Two GWAS studies were found for PCa progression, but with the established inclusion criteria they were discarded. This type of study is frequently used to estimate the susceptibility of different diseases, including PCa. The high costs of these assays and the large sample size they require might have hindered their widespread use in the studies identified in this review.
A total of 73 SNPs were identified in 39 genes, which were organized in seven groups according to their function. The functional integration of the products of the product of these genes can be seen in .
Figure 2. Functional integration of the gene products identified in the review in a prostatic epithelial cell, a steroid hormone-producing cell, and a hepatocyte. The seven groups the genes were classified into were identified with different colors, and their location in the different cell types was based on data reported in The Human Protein Atlas (https://www.proteinatlas.org). The group of genes related to sex steroid hormones (orange) is found in all three cell types. In the steroid hormone-producing cell, CYP17A1 and the HSD17B family are involved in testosterone synthesis, SULT2B1 decreases DHEA levels, and CYP19A1, which encodes for aromatase, facilitates the conversion of testosterone to estrogen; the NPAS family could regulate the circadian cycle of the adrenal gland or influence AR activity. In the prostatic epithelial cell, estrogen can bind to ESR1, which travels to the nucleus and activates gene transcription; in the endoplasmic reticulum, it can be metabolized into carcinogenic species by CYP1B1, which can be inactivated by UGT1, COMT, and the NQO family. UGT1 is also involved in the glucuronidation (G) of estrogen and testosterone, inactivating them and marking them for excretion. UGT1 and COMT are mostly expressed in the liver and are responsible for endobiotic and xenobiotic metabolism; however, their relationship with PCa is not entirely clear. As a consequence of the function of these genes, the transcriptional activity of these hormones is regulated in genes related to cell proliferation, growth, and survival. In the group of metabolism genes unrelated to steroid hormones (turquoise), all the products of these genes are in the prostatic epithelial cell, except for CES1, which is located in the hepatic cell and is related to endobiotic and xenobiotic metabolism. In the endoplasmic reticulum, PTGS2 participates in the synthesis of prostaglandins, which can induce an autocrine activation of the EP4 receptor activating the PI3K/Akt pathway, which, in turn, can activate mTOR and inhibit p53, among many other actions. This pathway has been related to cell proliferation and angiogenesis. Through glycosylation, SLC35B4 participates in the activation of oncoproteins such as YAP and Myc, which target the nucleus and activate the transcription of genes associated with proliferation and migration. MTHFR participates in folate metabolism, which could influence the methylation of genes associated with DNA repair and increased genomic instability. In the cell membrane, ADIPOQ binds to its receptor, activating AMPK, which is able to inhibit mTOR that normally can activate S6K1, promoting protein translation in ribosomes and cell growth. HFE regulates intracellular iron levels and may activate HIF1A, which activates EMT-related gene transcription. In the following groups, all functional integration of gene products was located only in the prostate cell. In the signaling pathway gene group (purple), FGFR4 binds to its ligand and can activate the mTOR pathway or the MAPK pathway, activating different TFs in the nucleus and promoting cell proliferation. TNFRSF13B binds to its ligand and activates NF-KB, which promotes the transcription of genes related to proliferation and EMT. LncRNA CASC8 could favor Myc expression, and Mir-605 can inactivate MDM2, favoring p53 activity, which can control cell cycle and apoptosis. STK3 prevents YAP activation through the Hippo suppressor pathway; it could promote AR activity and favors autophagosome binding to lysosomes, promoting cell proliferation. YWHAZ can also activate the Akt pathway sustaining growth signaling. In the cell proliferation group (red wine), CCND1 binds with CDK4 in the nucleus, inhibiting RB function, resulting in gene activation by E2F, which favors cell cycle progression. Anoctamins are membrane channels that help regulate intracellular calcium levels, which could regulate apoptosis. In the group of cell adhesion (green), when SHH is not present, CDON promotes apoptosis. CDH2 interacts with the membrane proteins of prostatic stromal cells and could promote tumor dissemination. In the autophagy group (blue), ATG16L1 and MAP1LC3 participate in phagosome synthesis, which, on the one hand, can reduce cellular oxidative stress and, on the other hand, can promote cell proliferation by facilitating protein recycling. In the group of other functions (pink), POU5F1 is a transcription factor that activates genes related to stem cell pluripotency, XRCC1 is involved in DNA repair, and MSMB is secreted by prostatic epithelial cells and may have autocrine and paracrine functions related to apoptosis and cell proliferation. CDB1 can interact with the T lymphocyte receptor participating in antigen presentation. AMPK: AMP-activated protein kinase; mTOR: mammalian target of rapamycin; EMT: epithelial-mesenchymal transition; HIF-1a: hypoxia-inducible factor-1a; NF-kB: nuclear factor kappa B; S6K1: Ribosomal protein S6 kinase beta-1; AR: androgen receptor; TCR: T cell receptor; TF: transcription factor; ADIPOR: adiponectin receptor; β2-m: beta-2-microglobulin; APRIL: proliferation-inducing ligand; DHEA: dehydroepiandrosterone; EP4: prostaglandin E receptor 4; LATS: large tumor suppressor kinase 1; TEAD: TEA domain transcription factor 1; FGF: fibroblast growth factor; SHH: Sonic hedgehog; MDM2: murine double minute 2; PI3K: phosphatidylinositol 3'-kinase; CDK4: cyclin-dependent kinase 4. *HSD17B2, B3, B12; ** NQO1, 2; ***NPAS2, 3.
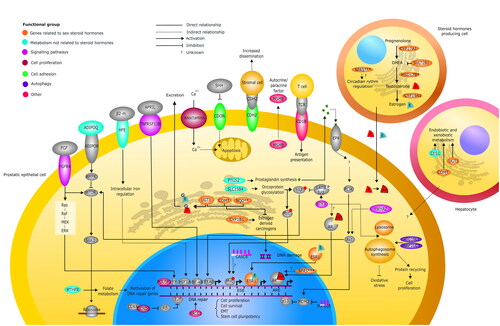
1. Genes related to sex steroid hormones
Variants were identified in three genes of the HSD17B family.
HSD17B2: It encodes a protein that inactivates estradiol and decreases androgen levels; it has been reported to decrease its expression as PCa progresses (Citation41). This review found six SNPs, one protective and five risk SNPs, associated with PCa progression (Citation11). It is not yet known how these variants are connected to PCa progression, but alterations in their function may lead to reduced androgen inactivation, potentially contributing to PCa progression.
HSD17B3: It encodes a protein that increases testosterone synthesis; however, its expression was found reduced in PCa (Citation42). Three SNPs were identified: two protective and one risk SNP for PCa progression. Risk SNP rs1810711 has been reported as part of a seven-SNP signature associated with increased susceptibility to PCa (Citation43). One of the protective SNPs (rs2257157) has also been associated with increased susceptibility to PCa (Citation44). On the other hand, an expression signature that included HSD17B2 and HSD17B3 was able to identify patients at high risk for PCa progression (Citation45).
HSD17B12: Its product converts estrone to estradiol and is involved in the elongation of fatty acids (Citation46). Three SNPs were identified: two protective and one risk SNP for PCa progression (Citation11). Protective SNP rs11037662 has been associated with a low response to androgen deprivation therapy (ADT) (Citation47). The other identified variables have not been linked to PCa in other studies. Another variant not identified in this review (HSD17B12 rs7932905) has been associated with increased tumor aggressiveness in PCa (Citation48).
Variants were identified in three genes of the cytochrome P450 (CYP) family involved in steroid synthesis.
CYP1B1: Its product catalyzes reactions involved in drug metabolism and the synthesis of cholesterol, steroids and other lipids. It also activates different estrogen-derived procarcinogens, and It has been reported to be overexpressed in PCa (Citation49). Two CYP1B1 SNPs associated with PCa progression were identified (Citation13,Citation28): rs1800440, which is risk SNP, and rs1056836, which is protective. They have been linked to increased susceptibility to PCa (Citation50–52).
CYP17A1: Its product promotes androgen synthesis and is expressed in about 50% of PCa cases (Citation53). Four SNPs associated with an increased risk of PCa progression were identified: rs743572, rs6162, and rs6163 have been associated with increased tumor aggressiveness, and rs6163 with lower BCR-free survival in PCa (Citation54); rs2486758 has been related to a worse response to ADT (Citation55).
CYP19A1: Its product codes for aromatase, which produces estrogens from androgens. Four SNPs were identified: three risk and one protective SNP (Citation14). Risk SNP rs1870050 has been associated with an increased response to ADT (Citation56). The other variables identified have not been linked to PCa in other studies. Another SNP not identified in this review (CYP19A1 rs4775936) has been related to lower survival in PCa (Citation57).
The products of NPAS2 and NPAS3 regulate functions like neurogenesis and the circadian cycle.
NPAS2: It is suggested that the product of this gene may regulate the circadian rhythm of the adrenal gland and the androgen receptor (AR) in PCa (Citation58,Citation59). This review found that SNP NPAS2 rs6542993 has been associated with an increased risk of progression (Citation15); this variant has not otherwise been related to PCa. Three other variants not identified in this review (NPAS2 rs895521, rs23051560, and rs746924) have been linked to increased susceptibility to PCa (Citation60–62).
NPAS3: It has a low expression in castration-resistant PCa (Citation63). NPAS3 rs8004379 was found to increase the expression of its product and is associated with a lower risk of PCa progression (Citation16). This is consistent with its reported tumor suppressor role in astrocytoma, regulating the cell cycle, apoptosis, and cell invasion (Citation64). Likewise, some oncogenic pathways demonstrate increased activity when NPAS3 expression is absent (Citation64).
The products of NQO1 and NQO2 prevent DNA damage by metabolizing compounds such as quinones, which are derived from estrogens (Citation65).
NQO1: SNP rs2917670 was associated with an increased risk of PCa progression (Citation13). Other variants not identified in this review, such as rs4986998, which reduces the activity of its product, and rs1800566 have been connected to increased susceptibility to PCa. Thus, it could be speculated that rs2917670 decreases the activity of the NQO1 product (Citation65,Citation66).
NQO2: Three SNPs associated with an increased risk of PCa progression were identified (Citation13). Of these, rs1143684 decreases the activity of the NQO2 product, so it could increase DNA damage (Citation67). The other variants identified have not been linked to PCa in other studies.
Other genes related to estrogen metabolism and action with variants related to PCa progression were also identified.
UGT1: Its product regulates estrogen excretion and metabolism, inactivating derived carcinogenic metabolites; its inhibition contributes to androgen receptor (AR) activation in PCa (Citation68). This study found seven associated SNPs (3 protective and 4 risk SNPs) (Citation17), which have not been linked to cancer in other studies. Another variant not identified in our study (UGT1 rs3064744) has been associated with increased susceptibility to PCa (Citation69).
COMT: Its product inactivates estrogen-derived reactive compounds, which can induce the neoplastic transformation of prostate cells (Citation70). Its inhibition may increase cell proliferation in PCa (Citation71). This review found three SNPs: one protective and two risk SNPs (Citation13). The two risk SNPs (rs9332377 and rs165849) have been linked to increased susceptibility to stomach and thyroid cancer (Citation72). The other identified variant has not been linked to cancer in other studies. Another variant not found in this review (COMT rs4680) has been associated with lower susceptibility to PCa (Citation73).
ESR1: Encodes for estrogen receptor 1, wich regulates the expression of genes related to cell proliferation and contributes to the development of PCa. Its high expression has been linked to metastases refractory to ADT, which has been connected to worse prognosis (Citation74–76). This review found three ESR1 SNPs associated with an increased risk of PCa progression (Citation14). Of these, rs1062577 increases ESR1 expression; it has been related to more aggressive PCa, and it results in the loss of miR-186 binding site, an inhibitor of cell proliferation in PCa (Citation54,Citation77). SNP rs9341016 has been associated with serum estrone levels, and rs488133 has been linked to an increased number of chromosomal aberrations in patients exposed to occupational radiation (Citation78,Citation79). Another variant not identified in this review (rs9340799) has been associated with increased susceptibility to PCa (Citation80).
Variants in other genes related to androgen and other lipid metabolism.
SULT2B1: Its product decreases the levels of androgenic precursor dehydroepiandrosterone (DHEA). SULT2B1 expression is reduced in PCa, and its inhibition promotes cell proliferation in this type of cancer (Citation81,Citation82). This review found two SULT2B1 SNPs associated with PCa progression: one risk and one protective SNP. These variants have not been linked to PCa in other studies.
2. Metabolism unrelated to steroid hormones
Variants were identified in genes related to the metabolism of carbohydrates, lipids, vitamins, and iron.
CES1: Its product is involved in endobiotic and xenobiotic metabolism, including toxins and drugs. CES1 is also a transcription factor target gene whose activity has been associated with lipid metabolism, lower androgen levels, and decreased cell proliferation in PCa (Citation83). Likewise, CES1 expression has been associated with decreased lipid accumulation and the induction of apoptosis in PCa (Citation84). In this review, two SNPs were found that decrease their expression and are associated with an increased risk of PCa progression: rs8192950 and rs8192935 (Citation18).
SLC35B4: Through its product, it transports nucleotide sugars from the cytoplasm to the Golgi apparatus. Studies have linked its function to changes in glycosylation of oncoproteins such as YAP1 and c-Myc, and its expression is elevated in different cancer types (Citation85,Citation86). SLC35B4 is overexpressed in PCa, where it is linked to greater aggressiveness and worse prognosis (Citation19). This review found two SNPs associated with an increased risk of progression (Citation19). Of these, rs1646724 increases the expression of SLC35B4 (Citation19). The other variant identified has not been connected to PCa in other studies.
PTGS2: Its product participates in prostaglandin biosynthesis and is overexpressed in PCa, which is associated with an increased risk of BCR (Citation87). Likewise, PTGS2 inhibition suppresses cell proliferation in PCa by decreasing angiogenesis and increasing apoptosis (Citation88). Prostaglandins could also activate the PI3K/AKT pathway resulting in increased cell proliferation in CaP. The present review identified the protective rs4648302 variant, which decreases the expression of this gene (Citation20).
MTHFR: Through its product, it participates in folate metabolism and DNA synthesis, methylation, and repair. MTHFR is overexpressed in PCa, which has been linked to lower BCR-free survival (Citation21). Likewise, low MTHFR expression has been associated with lower levels of hypermethylation in genes involved in DNA repair (Citation89). This review identified the rs9651118 variant, which decreases the expression of this gene and is associated with a lower risk of PCa progression (Citation21).
ADIPOQ: It encodes for adiponectin, which has pleiotropic functions in the metabolism of lipids and glucose. Adiponectin reduces cell proliferation and epithelial-mesenchymal transition in PCa, where low ADIPOQ expression has been seen in metastatic lesions (Citation90). Binding of ADIPOQ to its receptor can activate AMPK, which can inactivate mTOR. This review observed that rs182052 is associated with an increased risk of PCa progression (Citation22), and it correlates with lower serum adiponectin levels and increased susceptibility to PCa (Citation91).
HFE: Its product can form a complex with β2-microglobulin, which promotes cell activation and metastasis in PCa by regulating the intracellular iron level, which can activate the transcription factor 1-alpha inducible by hypoxia (HIF1A) (Citation92,Citation93). Likewise, HFE inhibition has been shown to increase sensitivity to radiotherapy in PCa (Citation94). This review found that rs9393682 was a risk factor, which has been reported to increase the expression of this gene (Citation23).
3. Signaling pathways
Variants were identified in genes related to the regulation of signaling pathways controlling functions like cell proliferation and survival.
MIR605: It encodes a miRNA that suppresses a p53 inhibitor and may reduce tumor proliferation and invasion in PCa, where its expression is decreased (Citation95). This review found a risk SNP (rs2043556) (Citation24), which has been reported to decrease the expression of this gene and which could explain this association (Citation96). This SNP has also been linked to increased susceptibility to different cancer types (Citation97).
CASC8: It encodes a long non-coding RNA located at 8q24.1, where other non-coding RNAs promote the interaction between the MYC promoter and its enhancers (Citation98). MYC is a proto-oncogene that regulates cell cycle progression, apoptosis, and cell transformation (Citation99). This review found a risk SNP for PCa progression (rs1447295) (Citation25), which has also been linked to increased susceptibility to PCa (Citation100).
TNFRSF13B: Through its product, it activates the signaling pathway of nuclear factor kappa B (NF-κB), promoting cell proliferation (Citation101). In PCa, NF-κB plays a key role in the development of castration resistance (Citation102). This review found that TNFRSF13B rs4792800 increases its expression and is associated with an increased risk of PCa progression (Citation26).
YWHAZ: Its product activates the phosphatidylinositol 3-kinase (PI3K)/AKT pathway, promoting cell survival (Citation103). YWHAZ is overexpressed in PCa cell lines associated with high Gleason score and BCR (Citation104). This review found that rs2290291 increases the expression of this gene and the risk of PCa progression (Citation27).
STK3: Although its product is part of the Hippo tumor suppressor pathway, it also has a non-canonical protumor function in PCa (Citation105). Likewise, its product has proven to be important for the binding of the autophagosome to the lysosome. STK3 is amplified, its expression has been associated with AR activity, and it promotes cell proliferation under conditions similar to castration in PCa (Citation105). This review found that STK3 rs7827435 decreases its expression and is linked to a lower risk of PCa progression (Citation28).
FGFR4: The binding of its product to a growth factor activates the MAPK/ERK pathway, which is involved in the regulation of cell proliferation and differentiation. This review found that FGFR4 rs351855 increases the risk of PCa progression (Citation29), and it has been associated with increased PCa invasiveness, increased stability, sustained AR activation, and increased susceptibility to PCa (Citation106–108).
4. Cell proliferation
Products of the ANO family can activate cell proliferation pathways by increasing intracellular calcium levels (Citation109).
ANO4: Low ANO4 expression may predict increased BCR-free survival (Citation110). This review identified a protective variant in ANO4 (Citation30); however, no other studies were found to link this SNP to cancer.
ANO5: This review found that ANO5 rs4622263 increases the risk of progression in PCa (Citation30); rs4622263 can regulate a transcription factor associated with cell regeneration in cancer (Citation30,Citation111).
ANO7: Its expression is reduced in PCa, which has been associated with early BCR (Citation112). This review identified a protective variant in ANO7 (Citation30); however, no other studies were found to link this SNP to cancer.
ANO10: This gene plays an important role in apoptosis (Citation113). This review identified a protective variant in ANO10 (Citation30); however, no other studies were found to link this SNP to cancer.
Likewise, a variant was identified in another gene involved in the regulation of cell proliferation.
CCND1: It encodes cyclin D1, which induces cell cycle transition from G1 phase to S phase promoting cell proliferation. Its high expression has been related to perineural invasion in PCa (Citation114). This review found that CCND1 rs9344 increases the risk of PCa progression (Citation31); it regulates the alternative splicing of CCND1 mRNA, resulting in a longer half-life of its product (Citation115). Likewise, rs9344 has been linked to higher CCND1 expression and a worse prognosis in esophageal cancer (Citation116). Interestingly, in the included article, association with progression was found in the G allele, which, unlike the A allele, has not been frequently linked to cancer.
5. Cell adhesion
Variants were identified in two genes associated with cell adhesion.
CDON: It encodes a membrane protein that regulates cellular interactions during myogenic differentiation. Likewise, when sonic hedgehog (SHH) is present, the proapoptotic activity of the product of CDON is inhibited, which promotes cell proliferation in PCa (Citation117). It has been evidenced that CDON is overexpressed in PCa, and its silencing reduces invasive capacity (Citation118). This review found that CDON rs3737336 increases the expression of this gene and is associated with an increased risk of PCa progression (Citation24). In contrast to these findings, rs3737336 has also been linked to a lower risk of PCa progression in patients with ADT (Citation119).
CDH2: Its product participates in tumor cell migration and invasion, and its expression has been linked to increased tumor aggressiveness (Citation120). In PCa, its expression has been associated with metastasis and resistance to castration (Citation121). This review identified three CDH2 SNPs associated with PCa progression: two risk and one protective SNPs (Citation32). Risk SNP rs643555 increases CDH2 expression, which has been associated with reduced BCR-free survival (Citation32). The other identified variants have not been linked to PCa in other studies.
6. Autophagy
Autophagy can decrease oxidative stress and DNA damage, but It also can promote cell proliferation through protein recycling (Citation122).
ATG16L1: Its product is involved in autophagosome synthesis and its loss increases the production of inflammatory cytokines (Citation123). Reduced ATG16L1 expression has been associated with lower relapse-free survival in PCa (Citation33). This review identified two variants in ATG16L1 associated with PCa progression (Citation33). Protective rs78835907 is associated with increased ATG16L1 expression in PCa (Citation33). The other identified variant has not been linked to PCa in other studies.
MAP1LC3: Its product also participates in autophagosome synthesis. To supply the nutrients needed for their growth, cancer cells increase autophagy to recycle damaged proteins and organelles (Citation124). In PCa, MAP1LC3 is overexpressed and is associated with a higher Gleason score (Citation125). This review found that MAP1LC3 rs8044820 increases the risk of PCa progression (Citation33). This variant has not been linked to PCa in other studies.
7. Other functions
Variants were identified in genes associated with cell differentiation, DNA repair, the immune system, and prostatic secretions.
POU5F1: Its product is a transcription factor that maintains the pluripotency of embryonic stem cells. POU5F1 expression is associated with the self-renewal capacity of cancer stem cells (Citation126). In PCa, these cells have a high level of self-renewal, can differentiate into heterogeneous tumor cells, and can be resistant to chemotherapy (Citation127). POU5F1 is overexpressed in 25% of castration-resistant tumors and in less than 11% of primary PCa tumors (Citation128). This review found that POU5F1 rs2394882 decreases the expression of this gene and reduces the risk of PCa progression (Citation34).
XRCC1: It participates through its product in cancer control by acting as a scaffolding protein for DNA repair enzymes (Citation129). This review found that XRCC1 Arg399Gln increases the risk of PCa progression (Citation35). Arg399Gln has been associated with increased DNA damage, possibly due to the decreased function of the XRCC1 product (Citation130). Likewise, it has been related to increased susceptibility to PCa (Citation131).
CB1B: Through its product, it participates in the presentation of antigens to T lymphocytes. It has been demonstrated that CBD1 is important for the immune system to recognize tumor neoantigens, and its low expression is associated with lower BCR-free survival in PCa (Citation36,Citation132). This review found that CDB1 rs3181082 decreases the expression of this gene while increasing the risk of PCa progression (Citation36).
MSMB: It has been expressed mostly in benign rather than malignant prostate tissue (Citation133). Its expression has been associated with a favorable prognosis in PCa, and the T allele of rs10993994, which reduces MSMB expression, has been linked to increased susceptibility to PCa (Citation134,Citation135). However, this review found that the C allele of rs10993994, which increases MSMB activity and expression, increases the risk of PCa progression (Citation25). Some studies support this finding as the T allele of rs10993994 is not always associated with more aggressive PCa; MSMB expression has also been linked to a shorter time to BCR (Citation136,Citation137).
Finally, four haplotypes were found to be associated with an increased risk of PCa progression (Supplementary Table 3). One haplotype with seven variants in UGT1A (Citation17); one haplotype with eight variants in CYP1B1, SULT2B1, and HSD17B2 (Citation13); one haplotype with four variants in CCND1 (Citation30); and one haplotype with three variants in TCF7L2 (Citation138). The TCF7L2 product promotes cell proliferation and regulates the expression of AR by being an effector of the Wnt pathway (Citation139). The variants in TCF7L2 (rs7094463, rs10749127, and rs11196224) are located close to enhancers of this gene, so they could have an effect on its activity (Citation138).
Conclusions
This systematic review examines the relationship of SNPs with PCa progression. The organization of genes in subgroups stands out as a strength since it allows for a functional perspective of the identified variants. As evidenced, some of these SNPs have been linked to changes in the activity or expression of genes with a significant role in PCa development and progression. Despite this, the conclusions of our study also have limitations. The mechanism by which some of these variants are associated with PCa is not entirely clear. Likewise, the data focused on specific polymorphisms; thus, it is possible that other variants with a significant association were not identified. On the other hand, SNPs were mainly described in two cohorts from Taiwan and Canada, so these findings have not been confirmed in patients from other populations. Similarly, as they are retrospective studies, factors like information quality and selection bias may have influenced the results. Lastly, excluding articles that used patients under PCa medications may have limited the amount of SNPs that were found in our revision. It is important to continue exploring the relevance of SNPs in PCa progression and prognosis to expand the molecular understanding of the disease and find a repertoire of potential biomarkers with a clinical impact on different populations. Also, incorporating additional variables like PSA levels and Gleason scores could enhance clinicians’ decision-making and potentially pave the way for future research studies, however, because of the lack of data from individual patients in these publications we couldn’t perform this analysis.
Ethics and integrity statements
Data availability statement: The authors confirm that data supporting the findings of this study are available in the article and its supplementary materials.
Funding statement: No funding was received for the conduct of this study.
Conflict of interest statement: The authors declare that they have no conflicts of interest.
Ethical approval statement: Not applicable.
Patient consent statement: Not applicable.
Permission to reproduce material from other sources: Not applicable.
Clinical trial registration: Not applicable.
Supplemental Material
Download PDF (3.5 MB)Acknowledgments
The researchers thank Paula Daniela Morales for her contribution to this article.
Disclosure statement
The authors report no conflicts of interest. The authors alone are responsible for the content and writing of the article.
Correction Statement
This article has been republished with minor changes. These changes do not impact the academic content of the article.
Additional information
Funding
References
- Ferlay J, Colombet M, Soerjomataram I, Parkin DM, Piñeros M, Znaor A, et al. Cancer statistics for the year 2020: An overview. Int J Cancer. 2021;149(4):778–789. doi:10.1002/ijc.33588.
- Siegel RL, Miller KD, Wagle NS, Jemal A. Cancer statistics, 2023. CA Cancer J Clin. 2023;73(1):17–48. doi:10.3322/caac.21763.
- Mottet N, van den Bergh RCN, Briers E, Van den Broeck T, Cumberbatch MG, De Santis M, et al. EAU-EANM-ESTRO-ESUR-SIOG Guidelines on prostate cancer-2020 update. Part 1: screening, diagnosis, and local treatment with curative intent. Eur Urol. 2021;79(2):243–262. doi:10.1016/j.eururo.2020.09.042.
- Rebello RJ, Oing C, Knudsen KE, Loeb S, Johnson DC, Reiter RE, et al. Prostate cancer. Nat Rev Dis Primers. 2021;7(1):9. doi:10.1038/s41572-020-00243-0.
- Hernandez DJ, Nielsen ME, Han M, Partin AW. Contemporary evaluation of the D’amico risk classification of prostate cancer. Urology. 2007;70(5):931–935. doi:10.1016/j.urology.2007.08.055.
- D’Amico AV, Whittington R, Malkowicz SB, Schultz D, Blank K, Broderick GA, et al. Biochemical outcome after radical prostatectomy, external beam radiation therapy, or interstitial radiation therapy for clinically localized prostate cancer. JAMA. 1998;280(11):969–974. doi:10.1001/jama.280.11.969.
- The Cancer Genome Atlas Research Network. The molecular taxonomy of primary prostate cancer. Cell. 2015;163(4):1011–1025. doi:10.1016/j.cell.2015.10.025.
- Sachidanandam R, Weissman D, Schmidt SC, Kakol JM, Stein LD, Marth G, et al. A map of human genome sequence variation containing 1.42 million single nucleotide polymorphisms. Nature. 2001;409(6822):928–933. doi:10.1038/35057149.
- Eeles RA, Olama AAA, Benlloch S, Saunders EJ, Leongamornlert DA, Tymrakiewicz M, et al. Identification of 23 new prostate cancer susceptibility loci using the iCOGS custom genotyping array. Nat Genet. 2013;45(4):385–391. 391e1-2. doi:10.1038/ng.2560.
- Eeles R, Goh C, Castro E, Bancroft E, Guy M, Olama AAA, et al. The genetic epidemiology of prostate cancer and its clinical implications. Nat Rev Urol. 2014;11(1):18–31. doi:10.1038/nrurol.2013.266.
- Audet-Walsh É, Bellemare J, Lacombe L, Fradet Y, Fradet V, Douville P, et al. The impact of germline genetic variations in hydroxysteroid (17-beta) dehydrogenases on prostate cancer outcomes after prostatectomy. Eur Urol. 2012;62(1):88–96. doi:10.1016/j.eururo.2011.12.021.
- Gu CY, Qin XJ, Qu YY, Zhu Y, Wan FN, Zhang GM, et al. Genetic variants of the CYP1B1 gene as predictors of biochemical recurrence after radical prostatectomy in localized prostate cancer patients. Medicine). 2016;95(27):e4066. doi:10.1097/MD.0000000000004066.
- Lévesque E, Laverdière I, Audet-Walsh E, Caron P, Rouleau M, Fradet Y, et al. Steroidogenic germline polymorphism predictors of prostate cancer progression in the estradiol pathway. Clin Cancer Res. 2014;20(11):2971–2983. doi:10.1158/1078-0432.CCR-13-2567.
- Lévesque É, Huang SP, Audet-Walsh É, Lacombe L, Bao BY, Fradet Y, et al. Molecular markers in key steroidogenic pathways, circulating steroid levels, and prostate cancer progression. Clin Cancer Res. 2013;19(3):699–709. doi:10.1158/1078-0432.CCR-12-2812.
- Yu CC, Chen LC, Chiou CY, Chang YJ, Lin VC, Huang CY, et al. Genetic variants in the circadian rhythm pathway as indicators of prostate cancer progression. Cancer Cell Int. 2019;19(1):87. doi:10.1186/s12935-019-0811-4.
- Bao BY, Lin VC, Yu CC, Yin HL, Chang TY, Lu TL, et al. Genetic variants in ultraconserved regions associate with prostate cancer recurrence and survival. Sci Rep. 2016;6(1):22124. doi:10.1038/srep22124.
- Laverdière I, Flageole C, Audet-Walsh É, Caron P, Fradet Y, Lacombe L, et al. The UGT1 locus is a determinant of prostate cancer recurrence after prostatectomy. Endocr Relat Cancer. 2015;22(1):77–85. doi:10.1530/ERC-14-0423.
- Ke CC, Chen LC, Yu CC, Cheng WC, Huang CY, Lin VC, et al. Genetic analysis reveals a significant contribution of CES1 to prostate cancer progression in Taiwanese Men. Cancers. 2020;12(5):1346. doi:10.3390/cancers12051346.
- Huang EY, Chang YJ, Huang SP, Lin VC, Yu CC, Huang CY, et al. A common regulatory variant in SLC35B4 influences the recurrence and survival of prostate cancer. J Cell Mol Med. 2018;22(7):3661–3670. doi:10.1111/jcmm.13649.
- Lee CH, Pao JB, Lu TL, Lee HZ, Lee YC, Liu CC, et al. Prognostic value of prostaglandin-endoperoxide synthase 2 polymorphisms in prostate cancer recurrence after radical prostatectomy. Int J Med Sci. 2016;13(9):696–700. doi:10.7150/ijms.16259.
- Lin VC, Lu TL, Yin HL, Yang SF, Lee YC, Liu CC, et al. Prognostic relevance of methylenetetrahydrofolate reductase polymorphisms for prostate cancer. Int J Mol Sci. 2016;17(12):1996. doi:10.3390/ijms17121996.
- Gu C, Qu Y, Zhang G, Sun L, Zhu Y, Ye D. A single nucleotide polymorphism in ADIPOQ predicts biochemical recurrence after radical prostatectomy in localized prostate cancer. Oncotarget. 2015;6(31):32205–32211. doi:10.18632/oncotarget.4980.
- Lin VC, Huang SP, Ting HJ, Ma WL, Yu CC, Huang CY, et al. Vitamin D receptor-binding site variants affect prostate cancer progression. Oncotarget. 2017;8(43):74119–74128. doi:10.18632/oncotarget.18271.
- Huang SP, Lévesque E, Guillemette C, Yu CC, Huang CY, Lin VC, et al. Genetic variants in microRNAs and microRNA target sites predict biochemical recurrence after radical prostatectomy in localized prostate cancer. Int J Cancer. 2014;135(11):2661–2667. doi:10.1002/ijc.28904.
- Huang SP, Huang LC, Ting WC, Chen LM, Chang TY, Lu TL, et al. Prognostic significance of prostate cancer susceptibility variants on prostate-specific antigen recurrence after radical prostatectomy. Cancer Epidemiol Biomarkers Prev. 2009;18(11):3068–3074. doi:10.1158/1055-9965.EPI-09-0665.
- Li CY, Huang SP, Chen YT, Wu HE, Cheng WC, Huang CY, et al. TNFRSF13B is a potential contributor to prostate cancer. Cancer Cell Int. 2022;22(1):180. doi:10.1186/s12935-022-02590-2.
- Yu CC, Chen LC, Lin WH, Lin VC, Huang CY, Lu TL, et al. Genetic association analysis of cell cycle regulators reveals YWHAZ has prognostic significance in prostate cancer. Cancer Genomics Proteomics. 2020;17(2):209–216. doi:10.21873/cgp.20181.
- Huang CY, Huang SP, Lin VC, Yu CC, Chang TY, Juang SH, et al. Genetic variants in the Hippo pathway predict biochemical recurrence after radical prostatectomy for localized prostate cancer. Sci Rep. 2015;5(1):8556. doi:10.1038/srep08556.
- Chen L, Lei Z, Ma X, Huang Q, Zhang X, Zhang Y, et al. Prognostic significance of fibroblast growth factor receptor 4 polymorphisms on biochemical recurrence after radical prostatectomy in a Chinese population. Sci Rep. 2016;6(1):33604. doi:10.1038/srep33604.
- Yu CC, Chen LC, Huang CY, Lin VC, Lu TL, Lee CH, et al. Genetic association analysis identifies a role for ANO5 in prostate cancer progression. Cancer Med. 2020;9(7):2372–2378. doi:10.1002/cam4.2909.
- Yu CC, Lin VC, Huang CY, Liu CC, Wang JS, Wu TT, et al. Prognostic significance of cyclin D1 polymorphisms on prostate-specific antigen recurrence after radical prostatectomy. Ann Surg Oncol. 2013;20(S3):492–499. doi:10.1245/s10434-013-2869-x.
- Yu CC, Chen LC, Lin VC, Huang CY, Cheng WC, Hsieh AR, et al. Effect of genetic variants in cell adhesion pathways on the biochemical recurrence in prostate cancer patients with radical prostatectomy. Cancer Med. 2019;8(6):2777–2783. doi:10.1002/cam4.2163.
- Huang CY, Huang SP, Lin VC, Yu CC, Chang TY, Lu TL, et al. Genetic variants of the autophagy pathway as prognostic indicators for prostate cancer. Sci Rep. 2015;5(1):14045. doi:10.1038/srep14045.
- Lin VC, Huang SP, Huang CY, Yu CC, Yin HL, Huang TY, et al. Cancer stem cell gene variants predict disease recurrence in Patients treated with radical prostatectomy for prostate cancer. Int J Med Sci. 2017;14(12):1301–1306. doi:10.7150/ijms.21428.
- Huang SP, Huang CY, Wang JS, Liu CC, Pu YS, Yu HJ, et al. Prognostic significance of p53 and X-ray repair cross-complementing group 1 polymorphisms on prostate-specific antigen recurrence in prostate cancer post radical prostatectomy. Clin Cancer Res. 2007;13(22 Pt 1):6632–6638. doi:10.1158/1078-0432.CCR-07-1437.
- Lee CH, Chen LC, Yu CC, Lin WH, Lin VC, Huang CY, et al. Prognostic value of CD1B in localised prostate cancer. Int J Environ Res Public Health. 2019;16(23):4723. doi:10.3390/ijerph16234723.
- Hanahan D, Weinberg RA. The hallmarks of cancer. Cell. 2000;100(1):57–70. doi:10.1016/s0092-8674(00)81683-9.
- Hanahan D. Hallmarks of Cancer: New Dimensions. Cancer Discov. 2022;12(1):31–46. doi:10.1158/2159-8290.CD-21-1059.
- Huang TY, Chien TM, Liu CC, Lee HY, Yu CC, Huang CY, et al. Prognostic significance of genetic polymorphisms in disease progression and survival in prostate cancer after androgen deprivation therapy. Urol Sci. 2015;26(2):81–84. doi:10.1016/j.urols.2014.11.002.
- Swift SL, Lang SH, White H, Misso K, Kleijnen J, Quek RG. Effect of DNA damage response mutations on prostate cancer prognosis: a systematic review. Future Oncol. 2019;15(28):3283–3303. doi:10.2217/fon-2019-0298.
- Gao X, Dai C, Huang S, Tang J, Chen G, Li J, et al. Functional silencing of HSD17B2 in prostate cancer promotes disease progression. Clin Cancer Res. 2019;25(4):1291–1301. doi:10.1158/1078-0432.CCR-18-2392.
- Khvostova EP, Otpuschennikov AA, Pustylnyak VO, Gulyaeva LF. Gene expression of androgen metabolising enzymes in benign and malignant prostatic tissues. Horm Metab Res. 2015;47(2):119–124. doi:10.1055/s-0034-1374631.
- Beuten J, Gelfond JAL, Franke JL, Weldon KS, Crandall AC, Johnson-Pais TL, et al. Single and multigenic analysis of the association between variants in 12 steroid hormone metabolism genes and risk of prostate cancer. Cancer Epidemiol Biomarkers Prev. 2009;18(6):1869–1880. doi:10.1158/1055-9965.EPI-09-0076.
- Kwon EM, Holt SK, Fu R, Kolb S, Williams G, Stanford JL, et al. Androgen metabolism and JAK/STAT pathway genes and prostate cancer risk. Cancer Epidemiol. 2012;36(4):347–353. doi:10.1016/j.canep.2012.04.002.
- Bamodu OA, Tzou KY, Lin CD, Hu SW, Wang YH, Wu WL, et al. Differential but concerted expression of HSD17B2, HSD17B3, SHBG and SRD5A1 testosterone tetrad modulate therapy response and susceptibility to disease relapse in patients with prostate cancer. Cancers. 2021;13(14):3478. doi:10.3390/cancers13143478.
- Labrie F, Luu-The V, Lin SX, Labrie C, Simard J, Breton R, et al. The key role of 17 beta-hydroxysteroid dehydrogenases in sex steroid biology. Steroids. 1997;62(1):148–158. doi:10.1016/s0039-128x(96)00174-2.
- Kohli M, Riska SM, Mahoney DW, Chai HS, Hillman DW, Rider DN, et al. Germline predictors of androgen deprivation therapy response in advanced prostate cancer. Mayo Clin Proc. 2012;87(3):240–246. doi:10.1016/j.mayocp.2011.09.009.
- Sun T, Oh WK, Jacobus S, Regan M, Pomerantz M, Freedman ML, et al. The impact of common genetic variations in genes of the sex hormone metabolic pathways on steroid hormone levels and prostate cancer aggressiveness. Cancer Prev Res). 2011;4(12):2044–2050. doi:10.1158/1940-6207.CAPR-11-0283.
- Beuten J, Gelfond JAL, Byrne JJ, Balic I, Crandall AC, Johnson-Pais TL, et al. CYP1B1 variants are associated with prostate cancer in non-hispanic and hispanic caucasians. Carcinogenesis. 2008;29(9):1751–1757. doi:10.1093/carcin/bgm300.
- Zhang H, Li L, Xu Y. CYP1B1 polymorphisms and susceptibility to prostate cancer: a meta-analysis. PLOS One. 2013;8(7):e68634. doi:10.1371/journal.pone.0068634.
- Liu JY, Yang Y, Liu ZZ, Xie JJ, Du YP, Wang W. Association between the CYP1B1 polymorphisms and risk of cancer: a meta-analysis. Mol Genet Genomics. 2015;290(2):739–765. doi:10.1007/s00438-014-0946-x.
- Cui L, Dillehay K, Chen W, Shen D, Dong Z, Li W. Association of the CYP1B1 Leu432Val polymorphism with the risk of prostate cancer: a meta-analysis. Mol Biol Rep. 2012;39(7):7465–7471. doi:10.1007/s11033-012-1579-y.
- Giatromanolaki A, Fasoulaki V, Kalamida D, Mitrakas A, Kakouratos C, Lialiaris T, et al. CYP17A1 and androgen-receptor Expression in prostate carcinoma tissues and cancer cell lines. Curr Urol. 2019;13(3):157–165. doi:10.1159/000499276.
- Robles-Fernandez I, Martinez-Gonzalez LJ, Pascual-Geler M, Cozar JM, Puche-Sanz I, Serrano MJ, et al. Association between polymorphisms in sex hormones synthesis and metabolism and prostate cancer aggressiveness. PLOS One. 2017;12(10):e0185447. doi:10.1371/journal.pone.0185447.
- Crucitta S, Del Re M, Paolieri F, Bloise F, Sbrana A, Sammarco E, et al. CYP17A1 polymorphism c.-362T > C predicts clinical outcome in metastatic castration-resistance prostate cancer patients treated with abiraterone. Cancer Chemother Pharmacol. 2020;86(4):527–533. doi:10.1007/s00280-020-04133-w.
- Ross RW, Oh WK, Xie W, Pomerantz M, Nakabayashi M, Sartor O, et al. Inherited variation in the androgen pathway is associated with the efficacy of androgen-deprivation therapy in men with prostate cancer. J Clin Oncol. 2008;26(6):842–847. doi:10.1200/JCO.2007.13.6804.
- Kanda S, Tsuchiya N, Narita S, Inoue T, Huang M, Chiba S, et al. Effects of functional genetic polymorphisms in the CYP19A1 gene on prostate cancer risk and survival. Int J Cancer. 2015;136(1):74–82. doi:10.1002/ijc.28952.
- Oster H, Damerow S, Hut RA, Eichele G. Transcriptional profiling in the adrenal gland reveals circadian regulation of hormone biosynthesis genes and nucleosome assembly genes. J Biol Rhythms. 2006;21(5):350–361. doi:10.1177/0748730406293053.
- Mukhopadhyay NK, Ferdinand AS, Mukhopadhyay L, Cinar B, Lutchman M, Richie JP, et al. Unraveling androgen receptor interactomes by an array-based method: discovery of proto-oncoprotein c-Rel as a negative regulator of androgen receptor. Exp Cell Res. 2006;312(19):3782–3795. doi:10.1016/j.yexcr.2006.07.017.
- Zhu Y, Stevens RG, Hoffman AE, Fitzgerald LM, Kwon EM, Ostrander EA, et al. Testing the circadian gene hypothesis in prostate cancer: a population-based case-control study. Cancer Res. 2009;69(24):9315–9322. doi:10.1158/0008-5472.CAN-09-0648.
- Chu LW, Till C, Yang B, Tangen CM, Goodman PJ, Yu K, et al. Circadian genes and risk of prostate cancer in the prostate cancer prevention trial. Mol Carcinog. 2018;57(3):462–466. doi:10.1002/mc.22770.
- Chu LW, Zhu Y, Yu K, Zheng T, Yu H, Zhang Y, et al. Variants in circadian genes and prostate cancer risk: a population-based study in China. Prostate Cancer Prostatic Dis. 2008;11(4):342–348. doi:10.1038/sj.pcan.4501024.
- Sicotte H, Kalari KR, Qin S, Dehm SM, Bhargava V, Gormley M, et al. Molecular profile changes in patients with castrate-resistant prostate cancer pre- and post-abiraterone/prednisone treatment. Mol Cancer Res. 2022;20(12):1739–1750. doi:10.1158/1541-7786.MCR-22-0099.
- Moreira F, Kiehl TR, So K, Ajeawung NF, Honculada C, Gould P, et al. NPAS3 demonstrates features of a tumor suppressive role in driving the progression of astrocytomas. Am J Pathol. 2011;179(1):462–476. doi:10.1016/j.ajpath.2011.03.044.
- Mandal RK, Nissar K, Mittal RD. Genetic variants in metabolizing genes NQO1, NQO2, MTHFR and risk of prostate cancer: a study from North India. Mol Biol Rep. 2012;39(12):11145–11152. doi:10.1007/s11033-012-2023-z.
- Chen S, Knox R, Lewis AD, Friedlos F, Workman P, Deng PS, et al. Catalytic properties of NAD(P)H:quinone acceptor oxidoreductase: study involving mouse, rat, human, and mouse-rat chimeric enzymes. Mol Pharmacol. 1995;47(5):934–939.
- Jamieson D, Wilson K, Pridgeon S, Margetts JP, Edmondson RJ, Leung HY, et al. NAD(P)H:quinone oxidoreductase 1 and nrh:quinone oxidoreductase 2 activity and expression in bladder and ovarian cancer and lower NRH:quinone oxidoreductase 2 activity associated with an NQO2 exon 3 single-nucleotide polymorphism. Clin Cancer Res. 2007;13(5):1584–1590. doi:10.1158/1078-0432.CCR-06-1416.
- Williamson SC, Mitter R, Hepburn AC, Wilson L, Mantilla A, Leung HY, et al. Characterisations of human prostate stem cells reveal deficiency in class I UGT enzymes as a novel mechanism for castration-resistant prostate cancer. Br J Cancer. 2013;109(4):950–956. doi:10.1038/bjc.2013.399.
- Tang L, Yao S, Till C, Goodman PJ, Tangen CM, Wu Y, et al. Repeat polymorphisms in estrogen metabolism genes and prostate cancer risk: results from the prostate cancer prevention trial. Carcinogenesis. 2011;32(10):1500–1506. doi:10.1093/carcin/bgr139.
- Mosli HA, Tolba MF, Al-Abd AM, Abdel-Naim AB. Catechol estrogens induce proliferation and malignant transformation in prostate epithelial cells. Toxicol Lett. 2013;220(3):247–258. doi:10.1016/j.toxlet.2013.05.002.
- Tolba MF, Omar HA, Hersi F, Nunes ACF, Noreddin AM. The impact of catechol-O-methyl transferase knockdown on the cell proliferation of hormone-responsive cancers. Mol Cell Endocrinol. 2019;488:79–88. doi:10.1016/j.mce.2019.03.007.
- Ruiz-Llorente S, Montero-Conde C, Milne RL, Moya CM, Cebrián A, Letón R, et al. Association study of 69 genes in the ret pathway identifies low-penetrance loci in sporadic medullary thyroid carcinoma. Cancer Res. 2007;67(19):9561–9567. doi:10.1158/0008-5472.CAN-07-1638.
- Brureau L, Moningo D, Emeville E, Ferdinand S, Punga A, Lufuma S, et al. Polymorphisms of estrogen metabolism-related genes and prostate cancer risk in two populations of African Ancestry. PLOS One. 2016;11(4):e0153609. doi:10.1371/journal.pone.0153609.
- Ricke WA, McPherson SJ, Bianco JJ, Cunha GR, Wang Y, Risbridger GP. Prostatic hormonal carcinogenesis is mediated by in situ estrogen production and estrogen receptor alpha signaling. Faseb J. 2008;22(5):1512–1520. doi:10.1096/fj.07-9526com.
- Ravenna L, Sale P, Vito MD, Russo A, Salvatori L, Tafani M, et al. Up-regulation of the inflammatory-reparative phenotype in human prostate carcinoma. Prostate. 2009;69(11):1245–1255. doi:10.1002/pros.20966.
- Semenas J, Wang T, Sajid Syed Khaja A, Firoj Mahmud A, Simoulis A, Grundström T, et al. Targeted inhibition of ERα signaling and PIP5K1α/Akt pathways in castration-resistant prostate cancer. Mol Oncol. 2021;15(4):968–986. doi:10.1002/1878-0261.12873.
- Hua X, Xiao Y, Pan W, Li M, Huang X, Liao Z, et al. miR-186 inhibits cell proliferation of prostate cancer by targeting GOLPH3. Am J Cancer Res. 2016;6(8):1650–1660.
- Litviakov NV, Goncharik OO, Freĭdin MB, Sazonov AE, Vasil’eva EO, Mezheritskiĭ SA, et al. [The estimate of association between gene polymorphisms and the frequency and spectrum of cytogenetic abnormalities in the cohort of Siberian group of chemical enterprises employees exposed to professional irradiation (microarray studies)]. Radiats Biol Radioecol. 2013;53(2):137–150. doi:10.7868/s0869803113020069.
- Beckmann L, Hüsing A, Setiawan VW, Amiano P, Clavel-Chapelon F, Chanock SJ, et al. Comprehensive analysis of hormone and genetic variation in 36 genes related to steroid hormone metabolism in pre- and postmenopausal women from the breast and prostate cancer cohort consortium (BPC3). J Clin Endocrinol Metab. 2011;96(2):E360–7. doi:10.1210/jc.2010-0912.
- Hernández J, Balic I, Johnson-Pais TL, Higgins BA, Torkko KC, Thompson IM, et al. Association between an estrogen receptor alpha gene polymorphism and the risk of prostate cancer in black men. J Urol. 2006;175(2):523–527. doi:10.1016/S0022-5347(05)00240-5.
- Seo YK, Mirkheshti N, Song CS, Kim S, Dodds S, Ahn SC, et al. SULT2B1b sulfotransferase: induction by vitamin D receptor and reduced expression in prostate cancer. Mol Endocrinol. 2013;27(6):925–939. doi:10.1210/me.2012-1369.
- He D, Falany CN. Inhibition of SULT2B1b expression alters effects of 3beta-hydroxysteroids on cell proliferation and steroid hormone receptor expression in human LNCaP prostate cancer cells. Prostate. 2007;67(12):1318–1329. doi:10.1002/pros.20615.
- Zhang B, Cheng Q, Ou Z, Lee JH, Xu M, Kochhar U, et al. Pregnane X receptor as a therapeutic target to inhibit androgen activity. Endocrinology. 2010;151(12):5721–5729. doi:10.1210/en.2010-0708.
- Zhou L, Zhang C, Yang X, Liu L, Hu J, Hou Y, et al. Melatonin inhibits lipid accumulation to repress prostate cancer progression by mediating the epigenetic modification of CES1. Clin Transl Med. 2021;11(6):e449. doi:10.1002/ctm2.449.
- Liu J, Zhao X, Wang K, Zhang X, Yu Y, Lv Y, et al. A novel YAP1/SLC35B4 regulatory axis contributes to proliferation and progression of gastric carcinoma. Cell Death Dis. 2019;10(6):452. doi:10.1038/s41419-019-1674-2.
- Rosa-Fernandes L, Oba-Shinjo SM, Macedo-da-Silva J, Marie SKN, Palmisano G. Aberrant protein glycosylation in brain cancers, with emphasis on glioblastoma. Adv Exp Med Biol. 2022;1382:39–70. doi:10.1007/978-3-031-05460-0_4.
- Khor LY, Bae K, Pollack A, Hammond MEH, Grignon DJ, Venkatesan VM, et al. COX-2 expression predicts prostate-cancer outcome: analysis of data from the RTOG 92-02 trial. Lancet Oncol. 2007;8(10):912–920. doi:10.1016/S1470-2045(07)70280-2.
- Liu XH, Kirschenbaum A, Yao S, Lee R, Holland JF, Levine AC. Inhibition of cyclooxygenase-2 suppresses angiogenesis and the growth of prostate cancer in vivo. J Urol. 2000;164(3 Pt 1):820–825. doi:10.1097/00005392-200009010-00056.
- Kang S, Kim JW, Kang GH, Park NH, Song YS, Kang SB, et al. Polymorphism in folate- and methionine-metabolizing enzyme and aberrant CpG island hypermethylation in uterine cervical cancer. Gynecol Oncol. 2005;96(1):173–180. doi:10.1016/j.ygyno.2004.09.031.
- Tan W, Wang L, Ma Q, Qi M, Lu N, Zhang L, et al. Adiponectin as a potential tumor suppressor inhibiting epithelial-to-mesenchymal transition but frequently silenced in prostate cancer by promoter methylation. Prostate. 2015;75(11):1197–1205. doi:10.1002/pros.23002.
- Dhillon PK, Penney KL, Schumacher F, Rider JR, Sesso HD, Pollak M, et al. Common polymorphisms in the adiponectin and its receptor genes, adiponectin levels and the risk of prostate cancer. Cancer Epidemiol Biomarkers Prev. 2011;20(12):2618–2627. doi:10.1158/1055-9965.EPI-11-0434.
- Josson S, Nomura T, Lin JT, Huang WC, Wu D, Zhau HE, et al. β2-microglobulin induces epithelial to mesenchymal transition and confers cancer lethality and bone metastasis in human cancer cells. Cancer Res. 2011;71(7):2600–2610. doi:10.1158/0008-5472.CAN-10-3382.
- Gross M, Top I, Laux I, Katz J, Curran J, Tindell C, et al. Beta-2-microglobulin is an androgen-regulated secreted protein elevated in serum of patients with advanced prostate cancer. Clin Cancer Res. 2007;13(7):1979–1986. doi:10.1158/1078-0432.CCR-06-1156.
- Josson S, Matsuoka Y, Gururajan M, Nomura T, Huang WC, Yang X, et al. Inhibition of β2-microglobulin/hemochromatosis enhances Radiation Sensitivity by Induction of Iron Overload in Prostate Cancer Cells. PLoS One. 2013;8(7):e68366. doi:10.1371/journal.pone.0068366.
- Zhou YJ, Yang HQ, Xia W, Cui L, Xu RF, Lu H, et al. Down-regulation of miR-605 promotes the proliferation and invasion of prostate cancer cells by up-regulating EN2. Life Sci. 2017;190:7–14. doi:10.1016/j.lfs.2017.09.028.
- Zhang MW, Jin MJ, Yu YX, Zhang SC, Liu B, Jiang X, et al. Associations of lifestyle-related factors, hsa-miR-149 and hsa-miR-605 gene polymorphisms with gastrointestinal cancer risk. Mol Carcinog. 2012;51 Suppl 1:21–31. doi:10.1002/mc.20863.
- Hu Y, Yu CY, Wang JL, Guan J, Chen HY, Fang JY. MicroRNA sequence polymorphisms and the risk of different types of cancer. Sci Rep. 2014;4(1):3648. doi:10.1038/srep03648.
- Kim T, Cui R, Jeon YJ, Lee JH, Lee JH, Sim H, et al. Long-range interaction and correlation between MYC enhancer and oncogenic long noncoding RNA CARLo-5. Proc Natl Acad Sci U S A. 2014;111(11):4173–4178. doi:10.1073/pnas.1400350111.
- Xiang JF, Yang L, Chen LL. The long noncoding RNA regulation at the MYC locus. Curr Opin Genet Dev. 2015;33:41–48. doi:10.1016/j.gde.2015.07.001.
- Amundadottir LT, Sulem P, Gudmundsson J, Helgason A, Baker A, Agnarsson BA, et al. A common variant associated with prostate cancer in European and African populations. Nat Genet. 2006;38(6):652–658. doi:10.1038/ng1808.
- Guttridge DC, Albanese C, Reuther JY, Pestell RG, Baldwin AS. NF-kappaB controls cell growth and differentiation through transcriptional regulation of cyclin D1. Mol Cell Biol. 1999;19(8):5785–5799. doi:10.1128/MCB.19.8.5785.
- Jin R, Yamashita H, Yu X, Wang J, Franco OE, Wang Y, et al. Inhibition of NF-kappa B signaling restores responsiveness of castrate-resistant prostate cancer cells to anti-androgen treatment by decreasing androgen receptor-variant expression. Oncogene. 2015;34(28):3700–3710. doi:10.1038/onc.2014.302.
- Neal CL, Xu J, Li P, Mori S, Yang J, Neal NN, et al. Overexpression of 14-3-3ζ in cancer cells activates PI3K via binding the p85 regulatory subunit. Oncogene. 2012;31(7):897–906. doi:10.1038/onc.2011.284.
- Rüenauver K, Menon R, Svensson MA, Carlsson J, Vogel W, Andrén O, et al. Prognostic significance of YWHAZ expression in localized prostate cancer. Prostate Cancer Prostatic Dis. 2014;17(4):310–314. doi:10.1038/pcan.2014.32.
- Schirmer AU, Driver LM, Zhao MT, Wells CI, Pickett JE, O'Bryne SN, et al. Non-canonical role of Hippo tumor suppressor serine/threonine kinase 3 STK3 in prostate cancer. Mol Ther. 2022;30(1):485–500. doi:10.1016/j.ymthe.2021.08.029.
- Xu W, Li Y, Wang X, Chen B, Wang Y, Liu S, et al. FGFR4 transmembrane domain polymorphism and cancer risk: a meta-analysis including 8555 subjects. Eur J Cancer. 2010;46(18):3332–3338. doi:10.1016/j.ejca.2010.06.017.
- Wang J, Stockton DW, Ittmann M. The fibroblast growth factor receptor-4 Arg388 allele is associated with prostate cancer initiation and progression. Clin Cancer Res. 2004;10(18 Pt 1):6169–6178. doi:10.1158/1078-0432.CCR-04-0408.
- Yu W, Feng S, Dakhova O, Creighton CJ, Cai Y, Wang J, et al. FGFR-4 Arg388 enhances prostate cancer progression via extracellular signal-related kinase and serum response factor signaling. Clin Cancer Res. 2011;17(13):4355–4366. doi:10.1158/1078-0432.CCR-10-2858.
- Kunzelmann K. TMEM16, LRRC8A, bestrophin: chloride channels controlled by Ca(2+) and cell volume. Trends Biochem Sci. 2015;40(9):535–543. doi:10.1016/j.tibs.2015.07.005.
- Yuan P, Ling L, Fan Q, Gao X, Sun T, Miao J, et al. A four-gene signature associated with clinical features can better predict prognosis in prostate cancer. Cancer Med. 2020;9(21):8202–8215. doi:10.1002/cam4.3453.
- Tun HW, Marlow LA, Roemeling C. v, Cooper SJ, Kreinest P, Wu K, et al. Pathway Signature and Cellular Differentiation in Clear Cell Renal Cell Carcinoma. PLoS One. 2010;5(5):e10696. doi:10.1371/journal.pone.0010696.
- Marx A, Koopmann L, Höflmayer D, Büscheck F, Hube-Magg C, Steurer S, et al. Reduced anoctamin 7 (ANO7) expression is a strong and independent predictor of poor prognosis in prostate cancer. Cancer Biol Med. 2021;18(1):245–255. doi:10.20892/j.issn.2095-3941.2019.0324.
- Wanitchakool P, Ousingsawat J, Sirianant L, Cabrita I, Faria D, Schreiber R, et al. Cellular defects by deletion of ANO10 are due to deregulated local calcium signaling. Cell Signal. 2017;30:41–49. doi:10.1016/j.cellsig.2016.11.006.
- Nakamura Y, Felizola SJA, Kurotaki Y, Fujishima F, McNamara KM, Suzuki T, et al. Cyclin D1 (CCND1) expression is involved in estrogen receptor beta (ERβ) in human prostate cancer. Prostate. 2013;73(6):590–595. doi:10.1002/pros.22599.
- Betticher DC, Thatcher N, Altermatt HJ, Hoban P, Ryder WD, Heighway J. Alternate splicing produces a novel cyclin D1 transcript. Oncogene. 1995;11(5):1005–1011.
- Izzo JG, Wu TT, Wu X, Ensor J, Luthra R, Pan J, et al. Cyclin D1 guanine/adenine 870 polymorphism with altered protein expression is associated with genomic instability and aggressive clinical biology of esophageal adenocarcinoma. J Clin Oncol. 2007;25(6):698–707. doi:10.1200/JCO.2006.08.0283.
- Sanchez P, Hernández AM, Stecca B, Kahler AJ, DeGueme AM, Barrett A, et al. Inhibition of prostate cancer proliferation by interference with SONIC HEDGEHOG-GLI1 signaling. Proc Natl Acad Sci U S A. 2004;101(34):12561–12566. doi:10.1073/pnas.0404956101.
- Hayashi T, Oue N, Sakamoto N, Anami K, Oo HZ, Sentani K, et al. Identification of transmembrane protein in prostate cancer by the Escherichia coli ampicillin secretion trap: expression of CDON is involved in tumor cell growth and invasion. Pathobiology. 2011;78(5):277–284. doi:10.1159/000329588.
- Bao BY, Pao JB, Huang CN, Pu YS, Chang TY, Lan YH, et al. Polymorphisms inside microRNAs and microRNA target sites predict clinical outcomes in prostate cancer patients receiving androgen-deprivation therapy. Clin Cancer Res. 2011;17(4):928–936. doi:10.1158/1078-0432.CCR-10-2648.
- Hazan RB, Phillips GR, Qiao RF, Norton L, Aaronson SA. Exogenous expression of N-cadherin in breast cancer cells induces cell migration, invasion, and metastasis. J Cell Biol. 2000;148(4):779–790. doi:10.1083/jcb.148.4.779.
- Jennbacken K, Tesan T, Wang W, Gustavsson H, Damber JE, Welén K. N-cadherin increases after androgen deprivation and is associated with metastasis in prostate cancer. Endocr Relat Cancer. 2010;17(2):469–479. doi:10.1677/ERC-10-0015.
- Karantza-Wadsworth V, Patel S, Kravchuk O, Chen G, Mathew R, Jin S, et al. Autophagy mitigates metabolic stress and genome damage in mammary tumorigenesis. Genes Dev. 2007;21(13):1621–1635. doi:10.1101/gad.1565707.
- Saitoh T, Fujita N, Jang MH, Uematsu S, Yang BG, Satoh T, et al. Loss of the autophagy protein Atg16L1 enhances endotoxin-induced IL-1beta production. Nature. 2008;456(7219):264–268. doi:10.1038/nature07383.
- Hu YL, DeLay M, Jahangiri A, Molinaro AM, Rose SD, Carbonell WS, et al. Hypoxia-induced autophagy promotes tumor cell survival and adaptation to antiangiogenic treatment in glioblastoma. Cancer Res. 2012;72(7):1773–1783. doi:10.1158/0008-5472.CAN-11-3831.
- Zhong C, Wu K, Wang S, Long Z, Yang T, Zhong W, et al. Autophagy-related circRNA evaluation reveals hsa_circ_0001747 as a potential favorable prognostic factor for biochemical recurrence in patients with prostate cancer. Cell Death Dis. 2021;12(8):726. doi:10.1038/s41419-021-04015-w.
- Vaddi PK, Stamnes MA, Cao H, Chen S. Elimination of SOX2/OCT4-associated prostate cancer stem cells blocks tumor development and enhances therapeutic response. Cancers. 2019;11(9):1331. doi:10.3390/cancers11091331.
- Hurt EM, Kawasaki BT, Klarmann GJ, Thomas SB, Farrar WL. CD44+ CD24(-) prostate cells are early cancer progenitor/stem cells that provide a model for patients with poor prognosis. Br J Cancer. 2008;98(4):756–765. doi:10.1038/sj.bjc.6604242.
- Taylor BS, Schultz N, Hieronymus H, Gopalan A, Xiao Y, Carver BS, et al. Integrative genomic profiling of human prostate cancer. Cancer Cell. 2010;18(1):11–22. doi:10.1016/j.ccr.2010.05.026.
- Caldecott KW, Aoufouchi S, Johnson P, Shall S. XRCC1 polypeptide interacts with DNA polymerase beta and possibly poly (ADP-ribose) polymerase, and DNA ligase III is a novel molecular “nick-sensor” in vitro. Nucleic Acids Res. 1996;24(22):4387–4394. doi:10.1093/nar/24.22.4387.
- Duell EJ, Wiencke JK, Cheng TJ, Varkonyi A, Zuo ZF, Ashok TD, et al. Polymorphisms in the DNA repair genes XRCC1 and ERCC2 and biomarkers of DNA damage in human blood mononuclear cells. Carcinogenesis. 2000;21(5):965–971. doi:10.1093/carcin/21.5.965.
- Chen L, Ambrosone CB, Lee J, Sellers TA, Pow-Sang J, Park JY. Association between polymorphisms in the DNA repair genes XRCC1 and APE1, and the risk of prostate cancer in white and black Americans. J Urol. 2006;175(1):108–112; discussion 112. doi:10.1016/S0022-5347(05)00042-X.
- Kain L, Webb B, Anderson BL, Deng S, Holt M, Costanzo A, et al. The identification of the endogenous ligands of natural killer T cells reveals the presence of mammalian α-linked glycosylceramides. Immunity. 2014;41(4):543–554. doi:10.1016/j.immuni.2014.08.017.
- Liu AY, Bradner RC, Vessella RL. Decreased expression of prostatic secretory protein PSP94 in prostate cancer. Cancer Lett. 1993;74(1-2):91–99. doi:10.1016/0304-3835(93)90049-f.
- Hyakutake H, Sakai H, Yogi Y, Tsuda R, Minami Y, Yushita Y, et al. Beta-microseminoprotein immunoreactivity as a new prognostic indicator of prostatic carcinoma. Prostate. 1993;22(4):347–355. doi:10.1002/pros.2990220409.
- Lou H, Yeager M, Li H, Bosquet JG, Hayes RB, Orr N, et al. Fine mapping and functional analysis of a common variant in MSMB on chromosome 10q11.2 associated with prostate cancer susceptibility. Proc Natl Acad Sci U S A. 2009;106(19):7933–7938. doi:10.1073/pnas.0902104106.
- Xu J, Isaacs SD, Sun J, Li G, Wiley KE, Zhu Y, et al. Association of prostate cancer risk variants with clinicopathologic characteristics of the disease. Clin Cancer Res. 2008;14(18):5819–5824. doi:10.1158/1078-0432.CCR-08-0934.
- Girvan AR, Chang P, van Huizen I, Moussa M, Xuan JW, Stitt L, et al. Increased intratumoral expression of prostate secretory protein of 94 amino acids predicts for worse disease recurrence and progression after radical prostatectomy in patients with prostate cancer. Urology. 2005;65(4):719–723. doi:10.1016/j.urology.2004.10.058.
- Chen CS, Huang CY, Huang SP, Lin VC, Yu CC, Chang TY, et al. Genetic interaction analysis of TCF7L2 for biochemical recurrence after radical prostatectomy in localized prostate cancer. Int J Med Sci. 2015;12(3):243–247. doi:10.7150/ijms.10953.
- Yang X, Chen MW, Terry S, Vacherot F, Bemis DL, Capodice J, et al. Complex regulation of human androgen receptor expression by Wnt signaling in prostate cancer cells. Oncogene. 2006;25(24):3436–3444. doi:10.1038/sj.onc.1209366.