Abstract
MicroRNA (miRNA) has garnered considerable attention due to its diagnostic capabilities, such as in hypoxic cognitive impairment and cancers. However, the existing miRNA detection methods are commonly criticized for the drawbacks of low sensitivity and false-positive detection derived from interfering molecules. Here, we provide a novel, sensitive and portable method for miRNA detection by combining target identification based cyclization of padlocks, immobilized primer-based signal amplification and a personal glucose meter. The proposed method exhibits several advantages, including precise identification of specific sites, exceptional sensitivity and instrument-free feature. These attributes hold great promise for the diagnosis and clinical investigation of various diseases, such as cancer and hypoxic cognitive impairment, enabling a deeper understanding of their pathological and physiological aspects.
METHOD SUMMARY
With miRNA-155 as detective target, the feasibility of the method has been demonstrated. The padlock sequences are cyclized by miRNA-155, which subsequently hybridize with primer sequence that is immobilized on the surface of a 96-well plate, and the interfering molecules are removed. This DNA polymerase triggers a chain extension process on the terminus of primer sequence, activating DNAzyme based cleavage. Consequently, a multitude of linker sequences are generated to facilitate the formation of the ‘e/linker/f/sucrase’ on magnetic bead, thereby enabling the catalysis of sucrose into glucose. This enzymatic reaction may be identified and measured using the personal glucose meter.
Skin and soft tissue infections caused by Pseudomonas aeruginosa is a common acquired disease in postpartum care.
The existing miRNA detection methods are commonly criticized for the drawbacks of low sensitivity and false-positive detection derived from interfering molecules.
Materials & methods
Cyclization of padlock.
The proximity ligation of HP and template sequence.
Analytical performance (e.g. sensitivity and selectivity) of the established approach.
Results & discussion
Cyclization of padlock and immobilization of primer.
The approach exhibits a wide detection range and a low limit of detection of 329 aM.
Conclusion
This technique offers a sensitive and reliable approach for nucleic acid amplification-based detection.
1. Background
MicroRNAs (miRNAs), one kind of noncoding RNA, alters several cellular processes and histopathology [Citation1,Citation2]. For example, miRNA plays a significant role in cerebral ischemia/reperfusion by modifying the function of mitochondrion, including oxidative stress, metabolism and apoptosis [Citation3]. Furthermore, a variety of miRNAs could take roles in the cancer progression, participate in the process of tumor immune and function with miRNA sponges [Citation4]. These understandings lead to emerging studies on miRNA as a diagnostic tool, impacting multiple areas of medicine [Citation5]. The emergence of highly sensitive miRNA assays has shown promising clinical potential as a way for diagnosing and monitoring of a variety of disease [Citation6,Citation7]. Therefore, it is highly desired to develop novel methods for miRNA analysis.
Many technologies have been developed recently in response to the growing need to detect and quantify miRNA [Citation8–10]. The extremely low concentration of miRNA in the blood presents a considerable difficulty for all methods. To detect miRNA with sufficient sensitivity, various signal amplifications are always included. The polymerase chain reaction (PCR) is the most widely used method of amplification because of its high sensitivity and precision [Citation11]. However, PCR-based procedures are highly dependent on the high-priced and incredibly precise heat cycling instruments, limiting their utility for real-time detection. Some isothermal amplification techniques have been proposed as viable replacements for PCR; these include strand displacement amplification (SDA) [Citation12], rolling circle amplification (RCA) [Citation13–15], exponential amplification reaction (EXPAR) [Citation16], recombinase polymerase amplification (RPA) [Citation17] and DNAzyme based strategies [Citation18,Citation19]. The RCA-based approaches in particular have garnered a lot of interest because of its stability and easy-to-operate feature. However, RCA-based miRNA detection methods have limitations, including requiring a complementary 3′ terminal of target sequence for cyclizing the padlock, which precludes their use for long single-stranded DNA sequence analysis, exhibiting poor signal amplification efficiency, being plagued by high background signal from conventional fluorescent probes and interfering molecules. Therefore, it is important to provide an instrument-free and sensitive platform for miRNA detection in light of the scarcity of resources in rural regions and the limitations of RCA-based approaches.
In this study, we provide a new approach that utilizes target recognition cyclized padlock, functional primer initiated signal cycles and personal glucose meter (PGM) for highly sensitive and portable analysis of miRNA. In the presence of miRNA, it forms duplex with the two ends of padlock, resulting in the cyclization of the padlock under the assistance of T4 DNA ligase. Subsequently, the cyclized padlock sequences are amplified by a signal cycle initiated by functionalized primers. The signal cycles release numerous linker sequences to facilitate the binding of sucrase to streptavidin magnetic beads (MBs). The suggested approach effectively differentiates site-specific mutations in miRNA with a high level of sensitivity. Furthermore, the system has been seamlessly included into the PGM-based results readout mode, so creating a sensitive, versatile and portable platform for the on-site identification of miRNA.
2. Materials & methods
2.1. Materials & reagents
All sequences and modifications of oligonucleotides are summarized in Supplementary Table S1 and were purchased from Tsingke Biotechnology Co., Ltd Target and padlock probes were purified with polyacrylamide gel electrophoresis. All other modified oligonucleotides were purified with HPLC. The DNA concentration was determined by measuring the absorbance at 260 nm. Phi29 DNA polymerase and T4 DNA ligase were purchased from New England Biolabs (Beijing, China). The HAuCl4, streptavidin (SA), bovine serum albumin (BSA), N-ethyl-N‘-(3-di-methylaminopropyl) carbodiimide (EDC), sodium acetate and dimethyl sulfoxide (DMSO) were purchased from Heowns Biochemical Technology Co., Ltd (Tianjin, China). Heowns Biochemical Technology Co., Ltd (Tianjin, China). Yuwell personal glucose meter (580) was used in this experiment.
2.2. Cyclization of padlock
10 μl target miRNA (10 μM) and 10 μl padlock sequence (10 μM) were added to a tube containing 30 μl buffer solution (20 mM Tris-HCl, 100 mM NaCl, 10 mM MgCl2, 5 mM KCl, pH 7.4). The mixture was heated to 90°C for 5 min and gradually cooled to room temperature. Then, 10 U of T4 DNA ligase was added. The ligation mixture incubated at 30°C for 30 min. Then the mixture was heated to 75°C for 5 min to inactivate the T4 DNA ligase.
2.3. Primer initiated signal cycle
10 μl cyclized padlock (10 μM) was added to the 96-well plates which is immobilized with primer sequences, and were incubated at room temperature for 10 min. Afterward, 1× phi 29 DNA polymerase buffer, 8 U phi29 DNA polymerase, 1 mM dNTPs and 5 μg BSA were added to the well, which was incubated at 35°C for 45 min and inactivated at 75°C for 10 min. In addition, 10 μl H probe (150 nM), 10 μl f-sucrase, and 10 μl e@MBs complex were added to the mixture. The mixture was incubated at room temperature for 15 min.
2.4. Target analysis via the proposed approach
The supernatant was collected via magnetic separation. Afterward, the supernatant was added with 1 μl of a sucrose solution (0.5 M). The mixture was incubated at 55°C for 30 min, and then 5 μl of the solution was detected by PGM to obtain the glucose signal.
3. Results & discussion
3.1. The working mechanism of the proposed method for miRNA analysis
The operational mechanism of the approach is illustrated in . The current study focuses on the detection of miRNA-155, which constitute miR-155/NRF2/HO-1 axis that plays a significant role in hypoxic cognitive impairment. The binding interaction between the target miRNA and the padlock sequence is primarily observed in the ‘c’ and ‘d’ regions. The padlock sequence forms an intact circular ring with the aid of T4 DNA ligase. The primer sequence, initially labeled on the surface of the 96-well plate, hybridizes with the ‘b’ section of circular ring. This primer serves as a catalyst for initiating the chain extension process with the aid of DNA polymerase, transcribing multiple ‘a’’ sections. Subsequently, the ‘a’’ segment hybridizes with the two complementary tails of the H probe, thereby activating the DNAzyme. Subsequently, the DNAzyme cleaves the substrate sequence at the ‘rA’ site with the assistance of Mg2+ in a buffer solution. This cleavage results in the liberation of linker sequence, and the regeneration of the ‘a'’ section. The linker sequence assembles with ‘e’ at the MB (e@MB) and ‘f’ labeled with sucrase (sucrase-f). Following the elimination of the unbound sucrase-f, the “e/linker/f/sucrase”@MB complex is employed to facilitate the catalysis of sucrose into glucose, hence leading to an amplified PGM signal. The PGM signal accurately indicates the variations in concentration of target miRNA, hence offering a convenient and precise quantitative method for detecting miRNA.
3.2. Cyclization of padlock & immobilization of primer
To validate the practicality of the suggested sensing approach, fluorescence experiments were conducted to verify the cyclization of the padlock and the immobilization of the functional primer on the surface of 96-well plate. In order to confirm the cyclization of the padlock by miRNA, fluorescent moiety (FAM) and the corresponding quenching moiety (BHQ) were utilized to label the two terminals of the padlock. In instances where miRNA was not existed in the sensing system, the fluorophore FAM became dissociated from the BHQ molecule, resulting in a heightened FAM signal. Upon the introduction of miRNA, it hybridizes with the two ends of the padlock, resulting in the quenching of the fluorescence signal emitted by the FAM. According to the data presented in A, the fluorescence spectra exhibited a peak value at 520 nm with a measurement of 3454 a.u. This value experienced a significant drop upon hybridization of the padlock with the miRNA, suggesting successful cyclization. The proper immobilization of the primer onto the plate's surface is of utmost importance in ensuring the effectiveness of succeeding signal cycles and reducing the interfering substances, hence minimizing background signals. Consequently, the primer was appropriately tagged with a fluorescent FAM moiety. Following the immobilization of the FAM-primer onto the plate, a PBS buffer was utilized to eliminate any unbound primer sequences. Subsequently, the fluorescent signals emitted by the FAM-primer@plate were documented (B).
3.3. DNAzyme cleavage & assembly of “e/linker/f/sucrase”@MB
To evaluate the cleavage activity of the DNAzyme, FAM and BHQ were attached to the two ends of the H probe. In the linear condition, the FAM signal of the H probe exhibited a high intensity, which experienced a significant reduction upon assembly of the H probe into a hairpin structure. Upon the addition of the H probe to the plate, it was observed that the FAM signal was restored. This observation suggests that the H probe has successfully bound to the single-stranded DNA (ssDNA) product, thereby initiating the cleavage of the DNAzyme. Consequently, the linker sequence that was tagged with FAM was dissociated from the ssDNA/H@plate complex (A). The findings presented in B demonstrate the viability of utilizing PGM as a readout tool for obtaining results across various combinations of experimental components. The significant enhancement of the PGM signal observed in the presence of target miRNA, e@MB and f-sucrase indicates the potential viability of the entire sensing system.
3.4. Optimization & selection of optimal experimental conditions
Different parameters were optimized to determine the best possible experimental conditions for this sensing system. In the study, the signal-to-background noise ratio (P/P0) was performed in order to demonstrate the impact of the experimental conditions, where P represents the PGM value with miRNA and P0 represents the background noise without a target. The P/P0 value exhibited a positive correlation with the concentration of H probe, ranging from 0 to 150 nM. However, a subsequent drop in the P/P0 value was obtained beyond the concentration of 150 nM, as depicted in A. Therefore, a concentration of 150 nM H probe was utilized for the following experiments. Additionally, it is worth considering that the concentration of the phi29 polymerase may exert an impact on the outcomes of miRNA detection. In B, it was observed that the optimal P/P0 value was achieved at an enzyme concentration of 8 U/L when the concentration of phi29 polymerase was varied from 2 U/l to 10 U/l. This particular concentration of 8 U/l was chosen for further investigations. In order to get the most favorable reaction time, the experiment was conducted by employing several incubation durations ranging from 30 to 180 min. The P/P0 exhibited no significant variations when the reaction time was extended to 120 min, as indicated by the results presented in C. Hence, a reaction time of 120 min was determined to be the optimal duration for the approach.
3.5. Analytical performance of the fluorescent biosensor
Following optimal experimental conditions, experiments were conducted with varying concentrations of miRNA while the PGM signals in response to the target molecules were monitored. As illustrated in A, a progressive increase in PGM signals was observed as the concentration of miRNA rose from 1 fM to 100 pM. Furthermore, B & C demonstrate a linear relationship between the PGM signals and the logarithmic value of miRNA concentration, which spans from 1 fM to 100 pM. The calibration curve's regression equation can be represented as Y = 1.233 × lgC + 5.275, where R2 = 0.9931. The limit of detection for miRNA was determined to be 329 aM using a 3σ approach. This finding demonstrates a significant enhancement in comparison to alternative methods that include intricate material preparations and assay procedures. To assess the selectivity of the developed approach, a set of additional DNA sequences were utilized as control molecules. These sequences contained one to three mismatched base pairs with the target miRNA and were denoted as mis1, mis2, mis3, let-7a and miRNA-211. The selectivity of the designed sensing method was evaluated by measuring its response to the target miRNA at a concentration of 100 fM. The contrasts depicted in D clearly demonstrate the strong selectivity of this sensing approach toward target miRNA in comparison to other interfering molecules. Specifically, only the samples containing target miRNA exhibit noticeable enhancements in fluorescence intensity when compared with the control species.
Figure 5. Analytical performance of the proposed method for miRNA analysis. (A) PGM values of the approach when detecting different concentrations of miRNA. (B) Correlation between the PGM value and the concentrations of miRNA. (C) linear correlation between the PGM values and the logarithmic concentrations of miRNA. (D) PGM values of the approach when detecting target and interfering sequences.
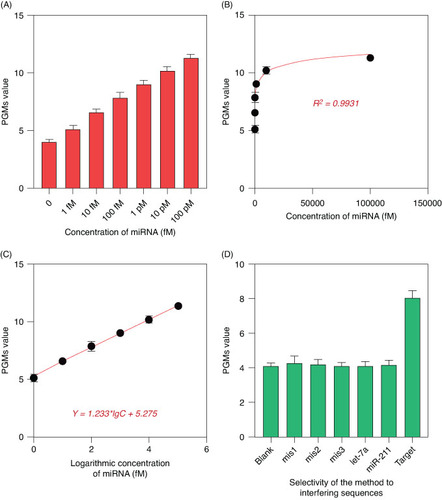
3.6. Analysis in complicate sample
Subsequently, a test was conducted to assess the impact of complex samples, including Bovine Serum Albumin (BSA), Dulbecco's Modified Eagle Medium (DMEM) and Phosphate-Buffered Saline (PBS) buffer, on the suggested approach for the detection of miRNA (10 and 100 pM). Through the introduction of various solutions to the miRNA, it was noted that the aforementioned procedure resulted in a minimal increase in PGM value (A). This finding suggests that the potential interference arising from complex samples can be effectively mitigated. The repeatability of the proposed method was assessed by conducting multiple detections of miRNA for 10 consecutive duplicates. The resulting PGM values (8.56 ± 0.312) exhibited a coefficient of variation (CV) of 4.04%. This suggests that the reproducibility of the proposed approach is satisfactory for clinical detection, as depicted in B.
Figure 6. Analytical performance of the proposed method in complicated samples. (A) PGM value of the approach when detecting 100 fM miRNA from different samples. (B) PGM value of the approach when detecting 100 fM miRNA from 10 sample duplicates.
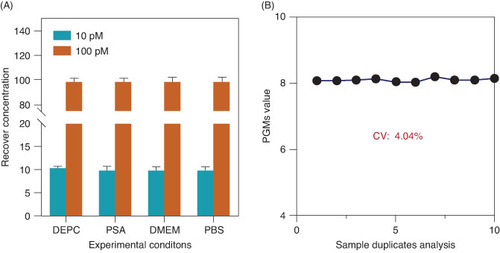
The miRNA-155/NRF2/HO-1 axis plays a crucial role in preventing neuronal cell damage induced by hypoxia/reoxygenation. Subsequently, we studied the expression level of miRNA-155 in the blood of rats with cognitive function impairment caused by hypoxia. Five groups of mice with hypoxia-induced cognitive impairment (test group) and five groups of normal rats (normal group) were included in this study. Peripheral blood of all these rats was extracted, and the differences of miRNA-155 expression levels in peripheral blood of rats in the two groups were studied by using the constructed method. As shown in Supplementary Figure S1, miRNA-155 concentration in peripheral blood of rats with cognitive impairment was significantly higher than that of rats in the normal group, suggesting that miRNA-155/NRF2/HO-1 axis possibly plays an important role in hypoxic-induced cognitive impairment in rats.
4. Conclusion
A proposed amplification technique for sensitive analysis of miRNA involves the utilization of Mg2+-DNAzyme and PGM. This strategy aims to increase the signal while minimizing background signals by effectively eliminating interfering molecules. By implementing integrated signal recycling cycles, it is possible to obtain a detection limit as low as 329 aM for miRNA. The utilization of functionalized primer-based signal cycles enhances the sensor's specificity in distinguishing the target analyte from complicated matrices found in real samples. The assay for miRNA presented in this study exhibits significant potential for the advancement of various sensors designed for the convenient and sensitive detection of nucleic acid sequences. The miRNA-155/NRF2/HO-1 axis plays a crucial role in preventing neuronal cell damage induced by hypoxia/re-oxygenation. Therefore, the proposed method has been utilized in analyzing the miRNA-155 from the in the blood of rats with cognitive function impairment caused by hypoxia. The result demonstrated a higher expression level of miRNA-155 in the blood of rats with cognitive function impairment caused by hypoxia. Additionally, it holds promise for the diagnosis of a range of disorders, including atherosclerosis and malignancies.
5. Future perspective
MicroRNAs have emerged as diagnostic biomarkers due to their crucial roles in the progression of disease, such as cancers. However, the existing miRNA detection methods are commonly criticized for the drawbacks of low sensitivity and false-positive detection derived from interfering molecules. In this study, we provide a new approach that utilizes target recognition cyclized padlock, functional primer initiated signal cycles and personal glucose meter (PGM) for highly sensitive and portable analysis of miRNA. The advantages of the proposed method could be concluded as: the functionalized primer mediated RCA process enables the removal of interfering molecules, thus endowing the method a low background signals; DNAzyme assisted signal amplification constitutes attached signal amplification and thus enables highly-sensitive miRNA analysis (Supplementary Table S2). However, multiple enzymes are essential for the signal amplification, including the T4 DNA ligase, phi29 DNA polymerase and sucrose, which may induce a higher background signals. Thus, the newly developed functionalized primer initiated signal cycles may be applied for sensitive and portable miRNA analysis and assist the early-diagnosis of postpartum care skin infections.
Author contributions
H Ding was the supervisor of the team in all research steps including designing, data analysis and manuscript writing. Y Xu has the main role for experimental data collection, data gathering, preparation of results and data analysis. F Fang, H Wang and A Chen assisted data analysis. All authors approved the submission of the manuscript.
Financial disclosure
The research was financially supported by the Health Science and Technology Plan of Zhejiang Province (project number: 2022RC265). The authors have no other relevant affiliations or financial involvement with any organization or entity with a financial interest in or financial conflict with the subject matter or materials discussed in the manuscript apart from those disclosed.
Writing disclosure
No writing assistance was utilized in the production of this manuscript.
Ethical conduct of research
The authors state that they have obtained appropriate institutional review board approval from Experimental Animal Center Experimental Animal Welfare Ethics Committee of Zhejiang Province (ZJCLA-IACUC-20050068) and/or have followed the principles outlined in the Declaration of Helsinki for all human or animal experimental investigations.
Supplementary Materials
Download MS Word (62 KB)Supplemental material
Supplemental data for this article can be accessed at https://doi.org/10.1080/07366205.2024.2348347
Competing interests disclosure
The authors have no competing interests or relevant affiliations with any organization or entity with the subject matter or materials discussed in the manuscript. This includes employment, consultancies, stock ownership or options and expert testimony.
Additional information
Funding
References
- Fang Z, Dou G, Wang L. MicroRNAs in the pathogenesis of nonalcoholic fatty liver disease. Int J Biol Sci. 2021;17(7):1851–1863. doi:10.7150/ijbs.59588
- Hochreuter MY, Dall M, Treebak JT, Barres R. MicroRNAs in non-alcoholic fatty liver disease: progress and perspectives. Mol Metab. 2022;65:101581. doi:10.1016/j.molmet.2022.101581
- Yuan L, Chen W, Xiang J, et al. Advances of circRNA-miRNA-mRNA regulatory network in cerebral ischemia/reperfusion injury. Exp Cell Res. 2022;419(2):113302. doi:10.1016/j.yexcr.2022.113302
- He B, Zhao Z, Cai Q, et al. miRNA-based biomarkers, therapies, and resistance in Cancer. Int J Biol Sci. 2020;16(14):2628–2647. doi:10.7150/ijbs.47203
- Ali Syeda Z, Langden SSS, Munkhzul C, et al. Regulatory mechanism of MicroRNA expression in cancer. Int J Mol Sci. 2020;21(5):1723. doi:10.3390/ijms21051723
- Cerne D, Bajalo JL. Cell-free nucleic acids as a non-invasive route for investigating atherosclerosis. Curr Pharm Des. 2014;20(31):5004–5009. doi:10.2174/1381612819666131206110317
- Dilliard SA, Cheng Q, Siegwart DJ. On the mechanism of tissue-specific mRNA delivery by selective organ targeting nanoparticles. Proc Natl Acad Sci USA. 2021;118(52):e2109256118. doi:10.1073/pnas.2109256118
- Huerta M, Rosello S, Sabater L, et al. Circulating tumor DNA detection by digital-droplet PCR in pancreatic ductal adenocarcinoma: a systematic review. Cancers (Basel). 2021;13(5):994. doi:10.3390/cancers13050994
- Guo T, Li W, Qian L, et al. Highly-selective detection of EGFR mutation gene in lung cancer based on surface enhanced Raman spectroscopy and asymmetric PCR. J Pharm Biomed Anal. 2020;190:113522. doi:10.1016/j.jpba.2020.113522
- Shlyapnikov YM, Malakhova EA, Shlyapnikova EA. Rapid amplification-free microarray-based ultrasensitive detection of DNA. Anal Chem. 2019;91(17):11209–11214. doi:10.1021/acs.analchem.9b02149
- Tzanikou E, Haselmann V, Markou A, et al. Direct comparison study between droplet digital PCR and a combination of allele-specific PCR, asymmetric rapid PCR and melting curve analysis for the detection of BRAF V600E mutation in plasma from melanoma patients. Clin Chem Lab Med. 2020;58(11):1799–1807. doi:10.1515/cclm-2019-0783
- Zhao S, Yu L, Yang S, et al. Boolean logic gate based on DNA strand displacement for biosensing: current and emerging strategies. Nanoscale Horiz. 2021;6(4):298–310. doi:10.1039/D0NH00587H
- Wang R, Zhao X, Chen X, et al. Rolling circular amplification (RCA)-assisted CRISPR/Cas9 cleavage (RACE) for highly specific detection of multiple extracellular vesicle MicroRNAs. Anal Chem. 2020;92(2):2176–2185. doi:10.1021/acs.analchem.9b04814
- Zhang G, Zhang L, Tong J, Zhao X, Ren J. CRISPR-Cas12a enhanced rolling circle amplification method for ultrasensitive miRNA detection. Microchem J. 2020;158(2020):105239. doi:10.1016/j.microc.2020.105239
- Dekaliuk M, Qiu X, Troalen F, et al. Discrimination of the V600E mutation in BRAF by rolling circle amplification and forster resonance energy transfer. ACS Sens. 2019;4(10):2786–2793. doi:10.1021/acssensors.9b01420
- Fang J, Yuan C, Luo X, et al. A Thermus thermophilus argonaute-coupling exponential amplification assay for ultrarapid analysis of circulating tumor DNA. Talanta. 2024;266(Pt 2):125034. doi:10.1016/j.talanta.2023.125034
- Wang X, Zhou S, Chu C, et al. Dual methylation-sensitive restriction endonucleases coupling with an RPA-assisted CRISPR/Cas13a System (DESCS) for highly sensitive analysis of DNA methylation and its application for point-of-care detection. ACS Sens. 2021;6(6):2419–2428. doi:10.1021/acssensors.1c00674
- Mu Q, Liu G, Yang D, et al. Ultrasensitive detection of DNA based on exonuclease III-assisted recycling amplification and DNAzyme motor. Bioconjug Chem. 2018;29(11):3527–3531. doi:10.1021/acs.bioconjchem.8b00774
- Chai H, Tang Y, Guo Z, Miao P. Ratiometric electrochemical switch for circulating tumor DNA through recycling activation of blocked DNAzymes. Anal Chem. 2022;94(6):2779–2784. doi:10.1021/acs.analchem.1c04037