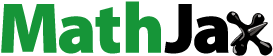
ABSTRACT
The Chalmers grouped actinide extraction (CHALMEX) process is focused on the co-separation of actinides from all other elements in spent nuclear fuel solution, with the ultimate purpose of transmuting the actinides into shorter-lived and less radiotoxic elements. Based on solvent extraction equilibrium distribution data of actinides and fission products, a preliminary flowsheet was developed and tested in batch mode. The flowsheet consists of one extraction step with the CHALMEX FS-13 solvent (25 mM CyMe4-BTBP in 30% v/v TBP and 70% v/v FS-13), using hydrophilic masking agents (20 mM bimet and 0.2 M mannitol) in the aqueous phase for the complexation of troublesome fission products. Two nitric acid scrub steps (0.5 M HNO3) were efficient in removing co-extracted acid, all molybdenum and the majority of silver. Two stripping stages (0.5 M glycolic acid at pH 4) were efficient in recovery of the actinides from the organic phase. The need for a solvent clean-up stage for the removal of nickel, cadmium, iron and the remaining silver from the organic phase was demonstrated. Based on the distribution data, it was calculated that a 99.9% recovery of americium is possible using only 3 ideal extraction stages, 3 ideal scrubbing stages and 2 ideal stripping stages.
Introduction
The recycling of plutonium and the minor actinides (Np, Am, Cm) for integration into advanced nuclear fuels is of interest due to their suitability for transmutation in fast nuclear reactors. Another option is the transmutation of the transuranium elements (TRU: Np, Pu, Am, Cm) in accelerator-driven systems.[Citation1,Citation2] Transmuting the TRU significantly reduces the long-term radiotoxicity and long-term heat generation of the final, highly radioactive waste.[Citation3] The trivalent minor actinides (Am and Cm) and pentavalent Np have however proven challenging to separate from the trivalent lanthanides due to the similar chemical properties of the two groups of elements. One approach has been to develop a solvent extraction process for Grouped ActiNide EXtraction (GANEX), while another approach focuses on the selective actinide extraction producing (near) pure elemental product streams.[Citation4,Citation5]
The overall aim of GANEX processes is to separate all actinides as a group from the fission and corrosion products present in used nuclear fuel, preferably without the need for redox control. The GANEX concept is based on a two-cycle process: the bulk uranium is extracted in the first cycle and TRU in the second cycle. The first GANEX cycle has been demonstrated on genuine spent nuclear fuel, using mixer settlers, resulting in a 99.99% recovery of uranium and with satisfying decontamination factors from the other actinides and fission products.[Citation6] In the second GANEX cycle, ligands are used which are specifically designed to either co-extract TRU and lanthanides, or to selectively extract only the TRU from the spent nuclear fuel solution. By dispensing with the separation of a pure plutonium product stream, the proliferation resistance of such a GANEX process is improved.[Citation4] Several GANEX versions are studied internationally. In two versions of the GANEX process, the CEA – and EURO-GANEX processes, the TRU and lanthanides are co-extracted in the second cycle. The separation of the TRU from the lanthanides occurs in the stripping stage, where the TRU are selectively stripped from the organic phase.[Citation7–14] In more recently proposed systems, the second cycle GANEX actinide recovery is achieved through the use of a single diamide of heterocyclic dicarboxylic acid or a single heterocyclic dicarboxamide.[Citation15,Citation16]
The Chalmers GANEX (CHALMEX) process is another version of the second cycle GANEX process. It combines the extractants 6,6ʹ-bis-(5,5,8,8-tetramethyl-5,6,7,8-tetrahydro-benzene-[1,2,4]-triazin-3-yl)-[2,2ʹ]-bipyridine (CyMe4-BTBP, ) and tri-n-butyl phosphate (TBP, ) in the fluorinated diluent phenyl trifluoromethyl sulfone (FS-13, ). In contrast to the CEA-GANEX and EURO-GANEX processes, the CHALMEX process aims to achieve direct TRU/fission product separation by extracting only the TRU elements from the spent nuclear fuel solution without any redox control or modification.[Citation17–21] The affinity of the extracting ligand for the trivalent actinides (An(III)) over trivalent lanthanides (Ln(III)) is explained by a higher degree of covalency in bonds between the soft N-donors in CyMe4-BTBP and An(III), compared to that of Ln(III) and the ligand.[Citation22–24] The CHALMEX process has shown promising results in its actinide-lanthanide separation, even during plutonium loading conditions. Extraction equilibrium is reached for all target radionuclides within 20 minutes when these are present in trace levels in isolated systems.[Citation17,Citation18,Citation21,Citation25–27]
Figure 1. (a) 6,6′‐bis-(5,5,8,8‐tetramethyl-5,6,7,8‐tetrahydro-benzene-[Citation1,Citation2,Citation4]-triazin‐3-yl)-[2,2′]-bipyridine (CyMe4-BTBP) (b) Tri-n-butyl phosphate (TBP) (c) Phenyl trifluoromethyl sulfone (FS-13).
![Figure 1. (a) 6,6′‐bis-(5,5,8,8‐tetramethyl-5,6,7,8‐tetrahydro-benzene-[Citation1,Citation2,Citation4]-triazin‐3-yl)-[2,2′]-bipyridine (CyMe4-BTBP) (b) Tri-n-butyl phosphate (TBP) (c) Phenyl trifluoromethyl sulfone (FS-13).](/cms/asset/7502449c-71c1-4d00-984b-846fe2600e93/lsei_a_1890372_f0001_b.gif)
Previous studies on the CHALMEX FS-13 process have focused on the scrubbing of co-extracted acid from the organic phase and the stripping of transuranic elements in isolated systems only.[Citation26] For the current solvent (CyMe4-BTBP and TBP in FS-13), no published studies focus on more process-like conditions, such as extraction, scrubbing and stripping under simulated processconditions. In our previous study, we showed that an optimised CHALMEX solvent (10 mM CyMe4-BTBP, 30% v/v TBP and 70% v/v FS-13) co-extracts palladium, silver and cadmium to a significant extent.[Citation28] Furthermore, the solvent was shown to be ineffective in extracting the An(III) from a simulated raffinate solution, with distribution ratios < 1, most likely as a result of solvent loading. The concentration of CyMe4-BTBP has therefore been increased to 25 mM in the experiments presented here, in order to ensure minor actinide extraction. The TBP/FS-13 ratio has been kept at 30% v/v for TBP, as was shown satisfactory for both uranium and plutonium extraction.[Citation28]
Another study has reported on the complexation of fission products, specifically molybdenum, zirconium and palladium, in the aqueous phase using mannitol and bimet ().[Citation19] Although efficient in masking zirconium, palladium and silver, the extraction of cadmium, molybdenum and nickel is still problematic, and so back-extraction of these elements in the scrubbing stages is desirable. Preliminary screening of different scrubbing solutions has been performed and 0.5 M HNO3 has been determined to be the most suitable scrubbing solution for the back-extraction of silver, molybdenum, palladium and zirconium.[Citation29]
Figure 2. (a) 2 R,3 R,4 R,5 R)-hexane-1,2,3,4,5,6-hexol (D-mannitol) b) (2S,2’S)-4,4ʹ-(ethane- 1,2 -diylbis(sulfanediyl))bis(2-aminobutanoic acid) (bimet).
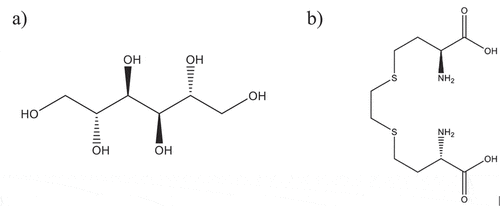
Since the CHALMEX process aims to separate plutonium, trivalent and pentavalent actinides from fission products, it is of importance to investigate the route of all fission products in the process. Such process data will demonstrate whether sufficient actinide/fission product separation is achieved, or if the fission products are back extracted with the actinides causing an impure product stream. The actinides can be back extracted by using 0.5 M glycolic acid at pH 4 as a stripping solution.[Citation26] Earlier assessments have estimated the CHALMEX process to Technology Readiness Level 2–3 (TRL 2–3), based on the absence of both computer simulations and cold tests defining fundamental data for the experimentation.[Citation30] Although the work here is considered a cold test of the process which supports the progress of the process’ TRL, computer simulations that could produce an official TRL increase are still lacking. This is of importance not only for comparative purposes with the other GANEX processes, but also an important official stage in the process development as it allows for calculation of the number of ideal stages for all process steps.
In this paper, the testing of a batch flowsheet using the aforementioned scrubbing and stripping solutions is presented. The ultimate purpose is to determine whether the fission product management strategy of the CHALMEX process is sufficient to ensure acceptable partitioning of plutonium and the MA from the fission products.
Materials and methods
Batch flowsheet testing
A full schematic representation of the process steps tested is shown in , where each stage represents a single batch experiment. In the test, 5.00 mL of pre-equilibrated (4 M HNO3) solvent (25 mM CyMe4-BTBP in 30% v/v TBP and 70% v/v FS-13) has been contacted with a simulated raffinate solution (see for composition) with a nitric acid concentration of 3.2 M HNO3 (4.70 mL). The sample was spiked with a mixed 241Am (281 kBq mL−1)/152Eu (278 kBq mL−1)/244Cm (151 kBq mL−1) tracer, 100 μL 239Pu tracer (323 kBq mL−1), and 100 μL 237Np tracer (30 kBq mL−1), in a test tube with volumetric markings. The radionuclides were obtained from the following sources:
244Cm: Oak Ridge National Laboratory, Oak Ridge, Tn, USA
241Am: Isotopendienst M. Blaseg GmbH, Waldburg, Germany
239Pu: Forschungszentrum Jülich laboratory stock solution
237Np: Forschungszentrum Jülich laboratory stock solution
152Eu: Eckert & Ziegler Nuclitec GmbH, Braunschweig, Germany
Table 1. The composition (mg L−1) of the simulated raffinate solution in 3.2 M HNO3. The simulated raffinate solution composition was based on 5000 L t−1 dissolved UOx fuel with initial 3.5% 235U enrichment, thermal burnup of 33,000 MWd tHM−1 and 3 years cooling
Figure 3. Suggested flowsheet for the CHALMEX FS-13 process. Each box represents one batch-contacting unit, with 90-minute contact time.
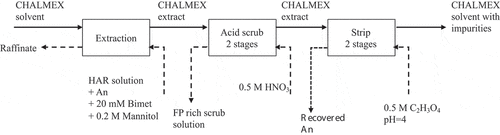
The simulated raffinate solution was prepared by introducing 0.2 M D-mannitol (Difco laboratories, Michigan, USA) and 20 mM bimet (produced in-house according to Kanesaka et al.[Citation31]) to the aqueous phase prior to contacting with the extractant phase for masking of selected fission products (Mo, Zr, Pd). CyMe4BTBP was supplied by Karlsruhe Institute of Technology, Karlsruhe, Germany and FS-13 by HaiHang Industry Co., Ltd., Jinan City, China. TBP (97% purity) was supplied by Aldrich (Steinheim, Germany). After the initial contacting and centrifugation, 500 μL samples of organic and aqueous phase, respectively, were each collected for analysis. The remaining aqueous phase was removed, and the remaining volume of organic phase was recorded. An equal volume to the remaining organic solution of acid/fission product scrub solution was added. The scrub solution was 0.5 M HNO3 (diluted from 65% HNO3 SupraPur, Merck). The contacting, centrifugation and sampling procedure was repeated for the scrub and for both stripping stages. A 0.5 M glycolic acid (99.5%, Merck) solution (adjusted to pH 4 by adding NaOH) was used as the strip solution.[Citation26] Each stage was contacted for 90 minutes at ambient temperatures using Heidolph reax top test tube shakers (Heidolph Instruments GmbH Co. KG, Schwabach) to reach extraction equilibrium at each stage. To limit heating effects from the shakers, the sample vial was swapped between two identical shakers every 15 minutes. The sample vial was centrifuged (Hettich EBA 8S) for 5 minutes to ensure full phase separation after each stage.
Analysis
A 200 μL sample of each phase was analysed by gamma spectrometry (Eurisys EGC35-195-R germanium coaxial N-type detector) to determine americium and europium radioactivities. Similarly, a 10 μL was collected from each phase for alpha spectrometry (Ortec Octête-pc eight chamber alpha measurement system equipped with PIPS detectors) to determine 237Np, 239Pu, 241Am and 244Cm radioactivities. The 10 μL sample was added to 100 μL of a mixture of 1% ZAP lacquer in acetone, and the liquid was then distributed on a metal planchet. The planchet was first allowed to dry under an IR lamp, and the planchet was next heated with a gas torch to burn off any residual organic matter. The planchet was then inserted into the alpha spectrometer and the measurement was allowed to proceed until a minimum of 10,000 counts per peak was recorded. A 20 μL sample of each phase was also collected and diluted by a factor of 104 for ICP-MS analysis. ICP-MS measurements of all elements were conducted using a Perkin Elmer NexION 2000, Perkin Elmer LAS GmbH, Rodgau, Germany. The aqueous phase was diluted in 1% v/v suprapur HNO3, while the organic phase was diluted in 1% v/v SupraPur HNO3 containing 2% v/v of the surfactant EcoSurf (Carl Roth, Karlsruhe, Germany), to allow dissolution. All pH measurements were made on the aqueous phases during the experiment using a Metrohm pH Meter 691. To confirm the pH measurements later, the aqueous solutions were titrated against NaOH using a Metrohm 905 Titrando automatic titrator.
Calculations
Number of ideal stages
Calculations for the ideal number of stages in a cascade counter-current operation for solvent extraction purposes can be made according to the same principles as for distillation and gas absorption.[Citation32] The extraction, P, of a solute can be calculated from EquationEquation 1(1)
(1) , where
is the phase ratio (O:A).
The fraction of a solute remaining in the aqueous phase compared to in the organic phase, given by the symbol , can be calculated using EquationEquation 2
(2)
(2) .
Here, x is the concentration of solute in the aqueous phase, subscript F refers to feed solution, while R refers to the raffinate. By rearranging EquationEquation 2(2)
(2) , the number of ideal stages, n, can be calculated according to EquationEquation 3
(3)
(3) .
Results and discussion
Batch flowsheet tests were conducted according to the flowsheet shown in . The distribution ratios of the radionuclides in each process step are shown in . The combination of increased CyMe4-BTBP concentration and masking agents allows for significant extraction of the An(III), compared to what has been reported earlier, due to the increased free CyMe4-BTBP concentration. The distribution ratio of Am (D = 32) is significantly higher than was reported in previous systems where 10 mM CyMe4-BTBP was used in the absence of masking agents.[Citation28] As mentioned above, the CyMe4-BTBP concentration was increased for the system tested here, as 10 mM CyMe4-BTBP was not concentrated enough to ensure sufficient An(III) extraction due to the preferential extraction of fission products over americium and curium.[Citation28] The D-values of neptunium and plutonium are both > 1.
Figure 4. The distribution ratio, D, of the radionuclides investigated in the batch flowsheet test. The extraction was performed by contacting the feed aqueous phase (simulated raffinate solution with addition of radiotracers) with the CHALMEX FS-13 solvent comprising 25 mM CyMe4-BTBP in 30% v/v TBP and 70% v/v FS-13. The acid scrub was 0.5 M HNO3. 0.5 M glycolic acid adjusted to pH 4 using NaOH was used as the stripping solution. Distribution ratios below 0.01 are not shown.
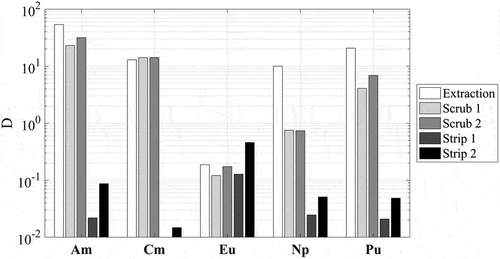
An increase in D-value is also seen for the curium extraction, for which a distribution ratio of 13 is obtained. The distribution ratio of plutonium (D = 21) is consistent with the previously reported values for the CHALMEX FS-13 system. TBP is a well-established extracting agent for plutonium and two TBP molecules form an extractable complex with plutonium. In contrast to uranium, plutonium is also extracted by the BTBP-ligand which explains the higher D-value of plutonium compared to uranium in PUREX systems.[Citation18,Citation28,Citation33–35] The neptunium distribution ratio (D = 10) is consistent with work recently published,[Citation28] although this is in contrast to work published by Halleröd et al., who reported D(Np) = 1.2.[Citation18] The difference is most probably due to differences in speciation of the neptunium stock solutions. In the present work, the neptunium speciation was monitored as Np(V) using UV-VIS spectrometer; the earlier publications assumed the presence of mixed oxidation states (without verification).[Citation18] Neptunium’s valence chemistry is highly variable, and is known to be affected by a range of conditions, including acid concentration ([H+]) and the presence of nitrous acid (HNO2).[Citation36–38] In concentrations of both 3.2 M HNO3 and 4 M HNO3, neptunium is known to undergo a spontaneous disproportionation reaction to form a mixture of Np(V) and Np(VI), with a mole fraction of more than 0.75 of Np favouring the Np(VI) state.[Citation39] The neptunium used in Halleröd et al.[Citation18] was milked from a 243Am/239Np column using HCl, and HCl is known to be a reducing acid. It is possible that the presence of HCl either reduced any Np(VI) that might have been formed back into Np(V), or that HCl hinders the disproportionation reaction by interacting with any formed nitrous acid, which is a potent catalyst for the reaction.[Citation39]
From , it can be seen that americium, curium, and plutonium largely remain in the organic phase during the acid/fission product scrubbing stages. Neptunium, in contrast, is readily back extracted by 0.5 M HNO3. This is unexpected as literature shows that in 0.5 M HNO3, Np will still exist with mole fractions of above 0.65 for Np(VI) and less than 0.35 for Np(V)[Citation39] . Both Np(IV) and Np(VI) have been shown to remain in the organic phase during such scrubbing conditions in similar systems, while Np(V) is readily back-extracted and has low distribution ratios at low acid concentrations.[Citation13,Citation40] It also is known that Np(V) in nitric acid media readily (but slowly) oxidizes due to the presence of dissociated nitrates, forming the more TBP-extractable Np(VI) species. It has also been reported that extracted Np(VI) in TBP-dodecane media, is reduced to the less extractable Np(V) by the presence of either U(IV) or nitrous acid in the organic phase. In addition, the presence of both H2O and acids in the organic phase has been shown have an impact on neptunium speciation.[Citation41,Citation42] Further studies are suggested to understand both the forward- and the back-extraction mechanism and speciation of neptunium in the CHALMEX FS-13 system.
Most of the remaining actinides are stripped in the first stripping stage. Approximately 2.3% of the americium activity was recorded in the organic phase compared to the aqueous phase after the first stripping stage, giving a distribution ratio of 0.02. The curium activity in the organic phase was below the detection limit after the first stripping stage, implying that practically all curium was removed from the organic phase. Plutonium had a distribution ratio of 0.02 after the first stripping stage, confirming the suitability of glycolic acid as a stripping agent. Despite the loss of neptunium in the scrubbing stages, the remaining fractions in the organic phase are stripped along with the other actinides.
The number of ideal stages required to extract 99.99% americium from the feed in a counter-current cascade operation was calculated according to EquationEquations 1(1)
(1) –Equation3
(3)
(3) . It was calculated that under identical conditions, 3 ideal stages of batch extraction will be required to extract 99.99% of the americium initially present in the spent fuel solution. For 99.99% extraction of both curium and neptunium, 4 extraction stages are required. For plutonium, 3 stages will be required for a 99.00% extraction.
By allowing less than 0.01% loss of americium in the scrubbing stages, a maximum of 3 scrubbing stages were calculated. For 3 scrubbing stages, a 0.03% loss of curium, 37% loss of neptunium and 3% loss of plutonium was found. For the overall recovery of 99.9% of americium, 2 stripping stages are required. Applying the number of stages for each process stage to obtain 99.9% americium recovery, the overall recovered percentages of the remaining actinides were calculated using EquationEquation 2(2)
(2) . The possible recovery, assuming ideal stages, is 61% for neptunium, 99% for curium and 94% for plutonium.
For comparison, both the CEA-GANEX and the EURO-GANEX processes have been hot-tested with genuine spent nuclear fuel, the former using mixer-settlers and the latter using centrifugal contactors.[Citation10,Citation11] The CEA-GANEX was demonstrated using 57 process stages in total and the EURO-GANEX using 32 process stages. Both processes were able to recover >99% of the Pu, Np and Am. The CEA-GANEX study also reported a high degree of Pd (44.0%), Fe (32.5%) and Sm (18.0%) contamination of the actinide stream. In a heterogeneous recycling option such as the TRU-SANEX process, a 32 stage flowsheet was tested using centrifugal contactors and a surrogate feed.[Citation43]
Even though the results for the CHALMEX process presented here are for ideal conditions and a simulated spent fuel raffinate, the total number of 8 process stages offer a significant simplification of the process flowsheet compared to each of the aforementioned processes. This is advantageous not only for capital costs of a plant, but also operational costs related to solvents and waste management. Furthermore, an actinide stream free from fission product contaminants is beneficial for fuel manufacture.
The distribution ratios for all fission products with D > 0.1 in the extraction stage are shown in , along with the titrated acidity for each process step. Nickel, cadmium and molybdenum are significantly extracted, with distribution ratios of > 100, 41.8 and 2.2 respectively in the extraction stage. Nickel is present in the raffinate at 38.4 mg L−1, which corresponds to 0.65 mM. Cadmium is present at 14.1 mg L−1 (0.12 mM), while molybdenum is present at 548 mg L−1 (5.7 mM). Both nickel and cadmium are largely seen to remain in the organic phase during both the scrubbing steps and the stripping steps , causing only low contamination of the actinide product. On the other hand, this causes concern for build-up of these elements in the organic phase, demonstrating the need for a solvent clean up stage. Meanwhile, molybdenum is efficiently removed from the organic phase during the two scrubbing stages. Due to the high distribution ratios of the actinides, molybdenum does not require any further handling.
Table 2. Distribution ratios, D, for all inactive (fission products) elements with D > 0.1 in the batch flowsheet test. The extracting solvent was 25 mM CyMe4-BTBP in 30% v/v TBP and 70% v/v FS-13. The scrub solution was 0.5 M HNO3 and the strip solution 0.5 M glycolic acid at pH 4. The table also shows the pH calculated from titrations of the aqueous phase after each contacting stage
Iron is the element present in the highest concentration in the raffinate solution used here, at 1545 mg L−1 (27.7 mM). Typically, the iron content of PUREX raffinates are considerably lower, as either U(IV) or hydrazine are used as reducing agents instead of ferrous iron.[Citation44] Nevertheless, an earlier CHALMEX solvent showed issues with solvent loading by iron, and so a high iron content raffinate was chosen to determine if the CHALMEX FS-13 solvent could suffer similar difficulties. It is seen that iron loading issues are avoided with the current solvent.[Citation45] However, even with D = 0.1, a significant amount of iron (~ 2.5 mM) is extracted by the CHALMEX FS-13 solvent. Neither the scrubbing solution, nor the stripping solution back-extracts the extracted iron. Reports on the UNEX process, which also uses FS-13 as a diluent, show success in reducing both the iron and zirconium extraction by complexing them with fluoride ions.[Citation46,Citation47]
The remaining elements with extraction distribution ratios above 0.1 are copper, silver and tin. Despite the low abundance in the simulated raffinate, silver is an efficient neutron poison and its presence in nuclear fuel is unwanted.[Citation48] Although 75% of the extracted silver is scrubbed in the two scrubbing stages (D = 0.5), the 25% remaining in the organic phase upon stripping largely remain in the solvent. As with cadmium and nickel, this further demonstrates the need for a solvent clean-up stage.
It is also worth noting that the pH of the aqueous phases low at each stage in the process. The pH of the acid scrubbing solutions after contacting with the organic phase show a pH as expected from pristine 0.5 M HNO3, suggesting little to no acid has been back extracted. After the second stripping stage the pH was titrated to 0.8. This suggests that there are significant amounts of residual acid in the organic phase and that this is scrubbed by the stripping solution along with the actinides.
Conclusions
The proof of concept of the CHALMEX FS-13 process was successfully demonstrated in a batch flowsheet test. It was shown that the TRU element’s distribution ratios are sufficiently high in the extraction process step to allow 99.99% extraction of americium from a simulated raffinate solution in 3 extraction stages. Americium, curium, and plutonium remain extracted in the scrubbing stages, while neptunium is partially back extracted, demanding further studies to optimise the scrubbing conditions. Stripping of the actinides was successfully achieved using glycolic acid at pH 4. For an overall recovery of 99.9% americium in the CHALMEX FS-13 process a theoretical number of 3 extraction stages, 3 scrubbing stages and 2 stripping stages were calculated, assuming ideal conditions. Such experimental conditions yield an overall 99% recovery of curium, 61% recovery of neptunium and 94% recovery of plutonium. Nickel, cadmium and molybdenum have the highest distribution ratios of the fission products. Iron is also a cause for concern, due to the very high abundance in spent nuclear fuel solution. While molybdenum is sufficiently scrubbed by 0.5 M HNO3, a solvent clean-up stage is required to remove iron, nickel and cadmium from the organic solvent, and any residual acid in the organic phase.
Additional information
Funding
References
- World Nuclear Association. (2019) Fast Neutron Reactors. https://www.world-nuclear.org/information-library/current-and-future-generation/fast-neutron-reactors.aspx.
- Abram, T.; Ion, S. Generation-IV Nuclear Power: A Review of the State of the Science. Energy Policy. 2008, 36(12), 4323–4330.
- Serp, J.; Poinssot, C.; Bourg, S. Assessment of the Anticipated Environmental Footprint of Future Nuclear Energy Systems. Evidence of the Beneficial Effect of Extensive Recycling. Energies. 2017, 10(9), 1445.
- Adnet, J.-M.; Miguirditditchian, M.; Hill, C.; Heres, X.; Lecomte, M.; Massin, M.; Brossard, P.; Baron, P. Development of New Hydrometallurgical Processes for Actinide Recovery: GANEX Concept. In GLOBAL 2005. In Proceedings of the International Conference on Nuclear Energy Systems for Future Generation and Global Sustainability. Atomic Energy Society of Japan: Japan, 2005.
- Geist, A.; Adnet, J.-M.; Bourg, S.; Ekberg, C.; Galán, H.; Guilbaud, P.; Miguirditchian, M.; Modolo, G.; Rhodes, C.; Taylor, R. An Overview of Solvent Extraction Processes Developed in Europe for Advanced Nuclear Fuel Recycling, Part 1 — Heterogeneous Recycling. Sep. Sci. Technol. 2020. doi:https://doi.org/10.1080/01496395.2020.1795680
- Miguirditchian, M.; Sorel, C.; Camès, B.; Bisel, I.; Baron, P.; Espinoux, D.; Calor, J.-N.; Viallesoubranne, C.; Lorrain, B.; Masson, M. (2009) HA Demonstration in the Atalante Facility of the GANEX 1st Cycle for the Selective Extraction of Uranium from HLW. In GLOBAL 2009: Proceedings of the GLOBAL 2009 congress - The Nuclear Fuel Cycle: Sustainable Options and Industrial Perspectives. Paris, France.
- ACSEPT. ACSEPT- Final Project Report; Bourg, S.; Eds. European Commission, 2012.
- Geniors Project. About Geniors. 2018. http://www.geniors.eu/about-geniors/.
- Bell, K.; Carpentier, C.; Carrott, M.; Geist, A.; Gregson, C.; Hérès, X.; Magnusson, D.; Malmbeck, R.; McLachlan, F.; Modolo, G.; et al. Progress Towards the Development of a New GANEX Process. Procedia Chem. 2012, 7, 392–397.
- Malmbeck, R.; Magnusson, D.; Bourg, S.; Carrott, M.; Geist, A.; Heres, X.; Miguirditchian, M.; Modolo, G.; Müllich, U.; Sorel, C.; et al. Homogenous Recycling of Transuranium Elements from Irradiated Fast Reactor Fuel by the EURO-GANEX Solvent Extraction Process. Radiochim. Acta. 2019, 107, 9–11.
- Miguirditchian, M.; Roussel, H.; Chareyre, L.; Baron, P.; Espinoux, D.; Calor, J. N.; Viallesoubranne, C.; Lorrain, B.; Masson, M. (2009) HA Demonstration in the Atalante Facility of the Ganex 2 Cycle for the Grouped TRU Extraction. In Proceedings of the GLOBAL 2009 congress - The Nuclear Fuel Cycle: Sustainable Options and Industrial Perspectives. France.
- Taylor, R.; Carrott, M.; Galan, H.; Geist, A.; Hères, X.; Maher, C.; Mason, C.; Malmbeck, R.; Miguirditchian, M.; Modolo, G.; et al. The EURO-GANEX Process: Current Status of Flowsheet Development and Process Safety Studies. Procedia Chem. 2016, 21, 524–529.
- Taylor, R.;. Reprocessing and Recycling of Spent Nuclear Fuel; Woodhead Publishing: Cambridge, U.K, 2015; Vol. 79.
- Joly, P.; Boo, E. ROADMAP- Actinide Separation Processes. Euroatom Research and Traning Programme on Nuclear Energy within the Seventh Framework Programme, 2015.
- Alyapyshev, M.; Babain, V.; Tkachenko, L.; Kenf, E.; Voronaev, I.; Dar’in, D.; Matveev, P.; Petrov, V.; Kalmykov, S.; Ustynyuk, Y. Extraction of Actinides with Heterocyclic Dicarboxamides. J. Radioanal. Nucl. Ch. 2018, 316(2), 419–428.
- Alyapyshev, M.; Babain, V.; Tkachenko, L. Various Flowsheets of Actinides Recovery with Diamides of Heterocyclic Dicarboxylic Acids. J. Radioanal. Nucl. Ch. 2017, 312(1), 47–58.
- Halleröd, J.; Ekberg, C.; Authen, T. L.; Bertolo, L.; Lin, M.; Grüner, B.; Švehla, J.; Wagner, C.; Geist, A.; Panak, P.; et al. On the Basic Extraction Properties of a Phenyl Trifluoromethyl Sulfone-based GANEX System Containing CyMe4-BTBP and TBP. Solvent Extr. Ion Exch. 2018, 36(4), 360–372.
- Halleröd, J.; Ekberg, C.; Aneheim, E. Phenyl Trifluoromethyl Sulfone as Diluent in a Grouped Actinide Extraction Process: Extraction Properties of the Solvent Components TBP and CyMe4-BTBP. J. Radioanal. Nucl. Ch. 2016, 307(3), 1711–1715.
- Aneheim, E.; Ekberg, C.; Foreman, M. R. S. J. A TBP/BTBP-based GANEX Separation Process – Part 3: Fission Product Handling. Solvent Extr. Ion Exch. 2013, 31(3), 237–252.
- Aneheim, E.; Ekberg, C.; Fermvik, A.; Foreman, M. R. S.; Grűner, B.; Hájková, Z.; Kvičalová, M. A TBP/BTBP-based GANEX Separation process—Part 2: Ageing, Hydrolytic, and Radiolytic Stability. Solvent Extr. Ion Exch. 2011, 29(2), 157–175.
- Aneheim, E.; Ekberg, C.; Fermvik, A.; Foreman, M. R. S.; Retegan, T.; Skarnemark, G. A TBP/BTBP-based GANEX Separation Process. Part 1: Feasibility. Solvent Extr. Ion Exch. 2010, 28(4), 437–458.
- Miguirditchian, M.; Guillaneux, D.; Guillaumont, D.; Moisy, P.; Madic, C.; Jensen, M. P.; Nash, K. L. Thermodynamic Study of the Complexation of Trivalent Actinide and Lanthanide Cations by ADPTZ, a Tridentate N-donor Ligand. Inorg. Chem. 2005, 44(5), 1404–1412.
- Ekberg, C.; Löfström-Engdahl, E.; Aneheim, E.; Foreman, M.; Geist, A.; Lundberg, D.; Denecke, M.; Persson, I. The Structures of CyMe4-BTBP Complexes of americium(III) and europium(III) in Solvents Used in Solvent Extraction, Explaining Their Separation Properties. Dalton Trans. 2015, 44, 18395–18402.
- Adam, C.; Kaden, P.; Beele, B. B.; Müllich, U.; Trumm, S.; Geist, A.; Panak, P. J.; Denecke, M. A. Evidence for Covalence in a N-donor Complex of americium(III). Dalton Trans. 2013, 42(39), 14068–14074.
- Halleröd, J.; Ekberg, C.; Löfström-Engdahl, E.; Aneheim, E. Development of the Chalmers Grouped Actinide Extraction Process. Nukleonika. 2015, 60(4), 829–835.
- Halleröd, J. On a Solvent Extraction System for Recycling Used Nuclear Fuel with CyMe4-BTBP and TBP as Extracting Agents. PhD thesis in Department of Chemistry and Chemical Engineering, Chalmers University of Technology Gothenburg, Sweden, 2018.
- Halleröd, J.; Ekberg, C.; Foreman, M.; Engdahl, E. L.; Aneheim, E. Stability of Phenyl Trifluoromethyl Sulfone as Diluent in a Grouped Actinide Extraction Process. J. Radioanal. Nucl. Ch. 2015, 304(1), 287–291.
- Authen, T. L.; Wilden, A.; Halleröd, J.; Schneider, D.; Kreft, F.; Modolo, G.; Ekberg, C. Batch Tests for Optimisation of Solvent Composition and Process Flexibility of the CHALMEX FS-13 Process. Solvent Extr. Ion Exch. 2020, 39(1), 1–17.
- Authen, T. L. CHALMEX FS-13 Investigations for Process Implementation, in Department of Chemistry and Chemical Engineering. Lic. of Eng. thesis in Department of Chemistry and Chemical Engineering, Chalmers University of Technology: Gothenburg, Sweden, 2020.
- Joly, P.; Boo, E. ROADMAP- Actinide Separation Processes 2015. SACSESS. 2016. http://www.sacsess.eu/Docs/SACSESS.PDF
- Kanesaka, M.; Okamoto, K.-I.; Hidaka, J. Preparation and Spectral Properties of Cobalt (III) Complexes with S, S′-Ethylenebis (L-homocysteinate) and S, S′-Trimethylenebis (L-homocysteinate). Bull. Chem. Soc. Jpn. 1986, 59(4), 1145–1150.
- McCabe, W. L.; Smith, J. C.; Harriott, P. Unit Operations of Chemical Engineering, 7th ed.; McGraw Hill: Singapore, 2005.
- Petrich, G.; Kolarik, Z. The 1981 PUREX Distribution Data Index; Kernforschungszentrum Karlsruhe: Germany, 1982.
- Irish, E. R.; Reas, W. H. The PUREX Process— A Solvent Extraction Reprocessing Method for Irradiated Uranium; General Electric: Richland, WA, 1957.
- Gopakumar, G.; Sreenivasulu, B.; Suresh, A.; Brahmmananda Rao, C. V. S.; Sivaraman, N.; Joseph, M.; Anoop, A. Complexation Behavior of the Tri-n-butyl Phosphate Ligand with Pu(IV) and Zr(IV): A Computational Study. J. Phys. Chem. A. 2016, 120(24), 4201–4210.
- Mincher, B. J.; Precek, M.; Mezyk, S. P.; Martin, L. R.; Paulenova, A. The Role of Oxidizing Radicals in Neptunium Speciation in γ-irradiated Nitric Acid. J. Radioanal. Nucl. Ch. 2013, 296(1), 27–30.
- Siddall, T., III; Dukes, E. Kinetics of HNO2 Catalyzed Oxidation of Neptunium (V) by Aqueous Solutions of Nitric Acid. Am. J. Phys. Chem. 1959, 81(4), 790–794.
- Precek, M.; Paulenova, A.; Tkac, P.; Knapp, N. Effect of Gamma Irradiation on the Oxidation State of Neptunium in Nitric Acid in the Presence of Selected Scavengers. Sep. Sci. Technol. 2010, 45(12-13, 1699–1705.
- Kim, K.-W.; Song, K.-C.; Lee, E.-H.; Choi, I.-K.; Yoo, J.-H. Oxidation State and Extraction of Neptunium with TBP. J. Radioanal. Nucl. Ch. 2000, 246(1), 215.
- Carrot, M. J.; Gregson, C. R.; Taylor, R. J. Neptunium Extraction and Stability in the GANEX Solvent: 0.2 M TODGA/0.5 M DMDOHEMA/kerosene. Solvent Extr Ion Exch 2013, 31(5), 463–482.
- Guillaume, B.; Maurice, C.; Moulin, J. P. (1984) Chemical Properties of Neptunium Applied to Neptunium Management in Extraction Cycles of Purex Process. In: Symposium on liquid-liquid extraction science; Dounreay (UK); Nov 27–29.
- Shilov, V. P.; Gogolev, A. V.; Fedoseev, A. M. Behavior of Neptunium Ions in Organic Media. Radiochemistry. 2012, 54(4), 315–323.
- Carrott, M.; Maher, C.; Mason, C.; Sarsfield, M.; Taylor, R. “TRU-SANEX”: A Variation on the EURO-GANEX and i-SANEX Processes for Heterogeneous Recycling of Actinides Np-Cm. Sep. Sci. Technol. 2016, 51(13), 2198–2213.
- Paviet-Hartmann, P.; Riddle, C.; Campbell, K.; Mausolf, E. Overview of Reductants Utilized in Nuclear Fuel Reprocessing/Recycling. In Proceedings of GLOBAL. Idaho National Laboratory: Salt Lake City, UT, 2013.
- Aneheim, E.; Ekberg, C.; Modolo, G.; Wilden, A. Single Centrifugal Contactor Test of a Proposed Group Actinide Extraction Process for Partitioning and Transmutation Purposes. Sep. Sci. Technol. 2015, 50(10), 1554–1559.
- Romanovskiy, V. N.; Valeriy, N.; Smirnov, I. V.; Babain, V. A.; Todd, T. A.; Herbst, R. S.; Law, J. D.; Brewer, K. N. The Universal Solvent Extraction (UNEX) Process. I. Development of the UNEX Process Solvent for the Separation of Cesium, Strontium, and the Actinides from Acidic Radioactive Waste. Solvent Extr Ion Exch. 2001, 19(1), 1–21.
- Law, J. D.; Herbst, R. S.; Todd, T. A.; Romanovskiy, V. N.; Babain, V. A.; Esimantovskiy, V. M.; Smirnov, I. V.; Zaitsev, B. N. The Universal Solvent Extraction (UNEX) Process. II. Flowsheet Development and Demonstration of the UNEX Process for the Separation of Cesium, Strontium, and Actinides from Actual Acidic Radioactive Waste. Solvent Extr Ion Exc 2001, 19(1), 23–36.
- Macklin, R. L.;. Neutron Capture Cross Sections of the Silver Isotopes 107Ag and 109Ag from 2.6 To 2000 keV. Nucl. Sci. Eng. 1982, 82(4), 400–407.