Abstract
Collingsworth, PD and Kohler, CC. 2010. Abundance and habitat use of juvenile sunfish among different macrophyte stands. Lake Reserv. Manage. 26:35-42.
Juvenile Lepomis sunfish (< 75 mm total length, TL) density, plant stem density and invertebrate density were compared in the 3 most common habitats in the littoral zone of Cedar Lake, a Midwestern North American reservoir: nonvegetated areas, stands of the exotic macrophyte Eurasian watermilfoil (Myriophyllum spicatum) and stands of American pondweed (Potomogeton nodosus). Eurasian watermilfoil stands consistently had significantly higher stem density and invertebrate density than American pondweed stands. Juvenile sunfish densities were significantly higher in vegetated habitats than nonvegetated areas, but no significant differences were observed between the 2 vegetated habitats. Juvenile sunfish densities were initially higher in American pondweed stands than Eurasian watermilfoil stands, but fish density in American pondweed stands declined dramatically as water levels fell in autumn. Juvenile sunfish size structure was related to habitat type, with the smallest fish associated with Eurasian watermilfoil (38.2 mm TL), intermediate fish associated with American pondweed (43.1 mm TL), and the largest fish associated with nonvegetated habitats (51.2 mm TL). We suggest resource agencies should focus vegetation management efforts on eradicating Eurasian watermilfoil stands to provide foraging access for largemouth bass to improve their growth rate and reduce stunting of sunfish. However, management agencies must recognize that a healthy plant community below the depth of water level fluctuation may be the only source of cover for juvenile centrarchids during late summer in reservoirs prone to fluctuating water levels.
Macrophytes enhance habitat quality for a variety of fish species by providing shade (CitationJohnson 1993), increasing surface area for attachment of epiphyton and invertebrate food resources (CitationCrowder and Cooper 1982, CitationLalonde and Downing 1992) and providing refuge from predators (CitationSavino and Stein 1982, CitationWerner et al. 1983). Abundant and diverse macrophytes tend to support larger populations and more diverse fish communities. For example, CitationKillgore et al. (1989) found in the Potomac River relative fish densities 2–7 times higher in areas with submersed vegetation compared to areas lacking such vegetation. Numerous other studies have also shown a link between the presence of macrophytes and increased fish abundance in a variety of systems (CitationDewey et al. 1997, CitationWeaver et al. 1997, CitationHatzenbeler et al. 2000).
In Midwestern lakes and reservoirs, juvenile sunfish (< 75 mm total length, TL) of the genus Lepomis use macrophyte beds to minimize threats from their primary predator, largemouth bass (Micropterus salmoides; CitationWerner et al. 1983). Largemouth bass foraging success has been shown to decline as both a function of increasing macrophyte stem density (CitationSavino and Stein 1982) and macrophyte growth form (e.g., canopy, simple complex leaf type; CitationValley and Bremigan 2002a). Serving as partial refuge areas from predators, stands of aquatic vegetation may hold high densities of juvenile sunfish. The presence of predators can also influence juvenile sunfish through nonlethal effects such as modification of behavior. Specifically, occupying dense stands of vegetation may represent a tradeoff between protection from predators and foraging efficiency and profitability in juvenile sunfish (CitationWerner et al. 1983).
We examined seasonal changes in macrophyte stem density, invertebrate density and juvenile sunfish density within the littoral zone of a small Midwestern reservoir. Macrophyte, invertebrate and fish densities were compared between stands of 2 macrophytes, American pondweed (Potamogeton nodosus) and Eurasian watermilfoil (Myriophyllum spicatum). Additionally we quantified the diets of juveniles from the 4 co-occurring sunfish species in the reservoir: bluegill (Lepomis macrochirus); green sunfish (Lepomis cyanellus); longear sunfish (Lepomis megalotis); and redear sunfish (Lepomis microlophus). The objectives of this study were to (1) evaluate the foraging and physical habitat provided by the two plant types; (2) determine if juvenile sunfish abundance differs according to habitat type and, in the case of vegetated habitats, plant stem density; and (3) evaluate the potential of resource overlap by juvenile sunfish species based on diets. The over-arching goal of this research was to provide insight into possible vegetation management techniques to enhance foraging opportunities for piscivores in vegetated reservoirs.
Study site
The study was conducted in Cedar Lake, Illinois, a 708-ha reservoir with a shoreline length of 48.3 km, a maximum depth of 12.2 m and an average depth of 4.4 m. Normal pool elevation is 131.7 m a.s.l. Largemouth bass is the primary predator of sunfish in Cedar Lake and is abundant in the reservoir. Water clarity is relatively high, with Secchi depths ranging from 15 cm to 6 m, allowing for a healthy macrophyte community. The littoral zone macrophyte community consists of native species such as American pondweed, sago pondweed (Potomogeton pectinatus L.) and coontail (Ceratophyllum demersum), as well as the exotic submergent Eurasian watermilfoil that occurs from the shoreline to a depth of 3 m. American pondweed and Eurasian watermilfoil predominate in the littoral zone and, accordingly, were the focus of our sampling effort.
Materials and methods
All field collections were conducted on a monthly basis from June through October 2002. Prior to monthly sampling dates, 6 areas, 3 in the northern coves and 3 in the southern coves of the reservoir, were randomly selected for sampling from coordinates on a grid. Within each area 3 different habitat types were randomly selected: one nonvegetated shoreline, an American pondweed stand, and a Eurasian watermilfoil stand. Vegetation stands were selected such that targeted species comprised at least 80% of the total vegetation. Samples were collected within each of the 3 habitat types in each area before moving to another area. Sampling was repeated in 6 randomly selected areas of the reservoir on each sampling date, yielding 6 samples per habitat type per month.
Macrophyte density was sampled using a 25-cm PVC frame with an attached 210-μ m Nytex mesh bag. Macrophyte samples were obtained by randomly placing the 25-cm frame within each site, manually clipping the plants near the substrate surface and then inverting the frame and lifting the bag out of the water. Three vegetation samples were taken at each site, yielding 18 vegetation samples for each macrophyte type per month. Macrophyte samples were bagged and placed on ice in the field for transport back to the lab where they were thoroughly washed to remove epiphyton and invertebrates. Stems were counted to provide mean monthly stem densities.
Macrophyte associated invertebrates, including attached and free-swimming zooplankton, were sampled in the 2 vegetated habitats using Nytex mesh bags using the same methods previously described for macrophyte sampling. Invertebrate samples were stored in a buffered 10% formalin-Rose Bengal solution to aid in sorting. Sorting began with samples being gently washed through a graded series of sieves (2, 1, 0.250, 0.125 and 0.063 mm mesh sizes). All organisms collected in the 1 and 2 mm sieves were sorted, identified to order or family level, counted and measured to the nearest millimeter. Organisms collected in the smaller 3 sieves were subsampled using a 1.0-L capacity Folsom plankton splitter prior to separation, identification, counting and measurement.
Juvenile sunfish were sampled from all 3 habitat types using buoyant pop nets (CitationSerafy et al. 1988). Fish samples were collected during morning hours to ensure that fish were actively feeding. The nets were set at each site for a minimum of 1 h to allow fish adequate time to recolonize the site, with most nets being set considerably longer. After the pop net was sprung, fish were collected by closing the bottom of the net, lifting the net out of the water and removing fish by hand. Preliminary experiments conducted in aquaculture ponds with varying densities of vegetation indicated that > 90% of juvenile bluegill in the pop net could be successfully collected in this manner.
All juvenile sunfish between 15 and 75 mm TL were counted and identified to species in the field. Due to the difficulty of field identification of very small fish, all fish < 15 mm TL were classified as unidentified Lepomis, which typically comprised over 90% of juvenile sunfish sampled. Prior to statistical analysis, unidentified Lepomis, were then classified to species according to the proportion of fish that could be identified (i.e., fish > 15 mm TL) at each site. In cases where a large number of fish were sampled, a subsample of 25 individuals of each species present were randomly taken and immediately placed on ice. Upon return to the lab, fish were measured for total length to the nearest millimeter, fixed in a 10% buffered formalin solution and then preserved in 70% ethanol.
For diet analysis fish were eviscerated and stomach linings were irrigated to remove all diet items. Stomach contents were then counted and identified to the lowest possible taxonomic level. Fish from all sampling periods were grouped according to species and dietary proportions averaged for each species. Results of the diet analysis were calculated as percent composition by number of total stomach contents for major prey groups.
Statistical analyses
All statistical tests were performed using JMP software (CitationSAS Institute, Inc. 2005) at a significance level of 0.05, unless otherwise noted. When necessary, data were square-root or log-transformed to normalize variances. A two-way analysis of variance (ANOVA) was used to assess the independent variables habitat type, sampling month and the habitat-sampling month interaction on the dependent variables vegetation density, invertebrate density, and juvenile sunfish density. When significant differences were found, Tukey's HSD test was used to indicate where differences occurred. To test the relative importance of plant type versus plant stem density, macrophyte stem density was also analyzed as a covariate to fish density. To test for differences in size structure among habitat types, 3 sequential two-sample Kolmogorov-Smirnov tests were employed (sequential Bonnferoni-adjusted α″ = 0.0167; CitationAbdi 2007). Due to low sample sizes for other Lepomis species, analysis of size structure was limited to juvenile bluegills only. Diet selectivity of juvenile sunfish was assessed using Strauss' linear selectivity index-L, calculated as:
Results
Juvenile sunfish density differed significantly among habitat types and across sampling dates, with vegetated habitats having higher juvenile fish densities than nonvegetated habitats (P < 0.0001, F = 58.72, df = 2); however, no differences between fish densities in American pondweed and Eurasian watermilfoil stands were found. Sampling date also had a significant effect on juvenile sunfish density, with higher densities occurring in June and July than later in the study (P = 0.004, F = 4.28, df = 4). An interaction between habitat type and sampling date on fish density was also detected (P < 0.0001, F = 14.30, df = 8). Fish density was initially higher in American pondweed stands in June and July than in Eurasian watermilfoil stands. Between July and August, however, fish density in American pondweed declined dramatically while fish density in Eurasian watermilfoil remained relatively unchanged ().
Figure 1 Monthly mean Lepomis densities (± 1 SE) among 3 habitat types (open water, American pondweed stands, and Eurasian watermilfoil stands) in Cedar Lake.
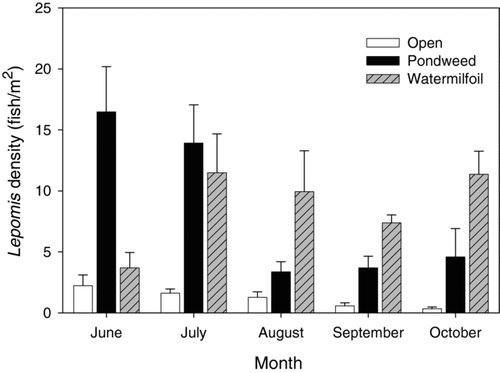
Juvenile bluegill size structure differed within the 3 habitat types (). The total catch of small < 15 mm TL), unidentified juvenile Lepomis were classified as 88, 97 and 96% bluegill in nonvegetated, pondweed and watermilfoil habitats, respectively, based on the catches of larger individuals in each habitat. Open water habitats had proportionally the largest juvenile bluegill compared to American pondweed habitats (P < 0.001, KS = 0.264) and Eurasian watermilfoil stands (P < 0.001, KS = 0.416). Bluegill sampled in open water habitats had an average size (± 1 SE) of 49.0 mm TL (21.9). American pondweed habitats supported intermediate size (42.7 mm TL, ± 16.1) juveniles, while the smallest bluegill (37.5 mm TL, ±15.7) were associated with Eurasian watermilfoil stands (P < 0.001, KS = 0.172).
Figure 2 Length frequency distribution of juvenile bluegills sampled among 3 habitats (open water, American pondweed stands, and Eurasian watermilfoil stands) in Cedar Lake. Distributions were significantly different among all habitat types (Kolmogorov-Smirnov 2-Sample Test; α″ = 0.016).
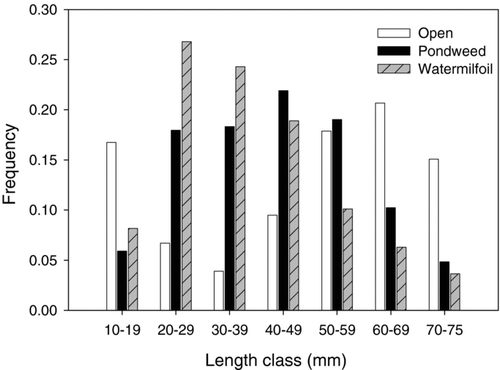
To address the relative importance of plant stem density versus plant type, nonvegetated habitats were removed from the analysis, and vegetation density was added as a covariate to fish density; variation in macrophyte stem density was not significantly related to fish density (P = 0.494, F = 0.476, df = 1). The effect of both macrophyte type (P = 0.573) and sampling date (P = 0.036) on fish density were relatively unchanged with the addition of the covariate. However, plant density had a dramatic effect on the macrophyte type by sampling date interaction (P = 0.021).
At least 5% of the total diet for all sunfish species was represented by 8 prey taxa: amphipods, cladocera, copepods, dipteran larvae, ephemeropteran larvae, gastropods, ostracods, and trichopteran larvae. All other invertebrate taxa, which collectively accounted for < 20% of sunfish diets, were excluded from the analysis. Bluegill fed extensively on zooplankton and dipteran larvae in Cedar Lake while other insect larvae and snails contributed less to their diet. Cladocera and dipteran larvae were the dominant prey items in juvenile bluegill diets, but ostracods and copepods were also frequently eaten. Positive selectivity values for dipteran larvae (L = 0.227) and ostracods (L = 0.114) were found for juvenile bluegills (), whereas a negative index value was found for cladocera (L = −0.214).
Table 1 Strauss' linear selectivity indices (L) of juvenile sunfish for vegetation-associated invertebrates in Cedar Lake. Positive L values indicate a preference for a taxon, whereas negative L values indicate a lack of preference for a taxon; N refers to the number of individuals in which the taxon was present in the sunfish diets. For each species, the number of individuals processed for diet is indicated in parentheses next to the species name.
Green sunfish ate larger insect larvae as well as a large number of copepods. Amphipods, mayfly and caddisfly larvae were present in ∼ 50% of the green sunfish sampled. No single prey taxa dominated green sunfish diets, but dipteran larvae and zooplankton were frequently eaten by a high percentage of fish. Juvenile green sunfish had positive selectivity values for ostracods (L = 0.163) and ephemeroptera larvae (L = 0.134), whereas negative index values were found for cladocera (L = −0.325; ).
Longear sunfish diets also contained a large contribution of epiphytic insect larvae. Mayfly and caddisfly larvae were present in ∼ 50% of longear sunfish diets in the study but were not eaten as frequently as by green sunfish. Cladocera, ostracods and dipteran larvae provided the largest contribution to juvenile longear sunfish diets, although copepods were also eaten by a large percentage of fish. Longear sunfish positively selected dipteran larvae (L = 0.209) and ostracods (L = 0.192), whereas a negative linear selectivity index existed for cladocera (L = −0.359; ).
Redear sunfish frequently consumed dipteran larvae but also had a large contribution of hard-shelled prey types. Snails and ostracods were important prey items in stomachs of redear, representing ∼ 80% of those sampled. Another unique characteristic of redear diets was a less frequent use of cladocera and copepods. Redear sunfish positively selected ostracods (L = 0.291) and gastropods (L = 0.294), whereas a negative linear selectivity index value (L = −0.436) was found for cladocera ().
Vegetation density varied considerably with macrophyte type and across sampling date. Eurasian watermilfoil habitats had higher stem density than American pondweed habitats (P < 0.0001, F = 24.1, df = 1). Sampling date was also significantly related to vegetation density (P < 0.0001, F = 23.4, df = 4), with higher plant densities found later in the study. A macrophyte type by date interaction effect for vegetation density existed (P < 0.0001, F = 38.0, df = 4). Stem density in June was higher in the shallow water American pondweed stands than the deeper water Eurasian watermilfoil stands due to the earlier emergence of the former species; however, pondweed densities remained largely unchanged throughout the study. By July, Eurasian watermilfoil densities equaled or exceeded American pondweed values ().
Figure 3 Monthly mean stem density (± 1 SE) of American pondweed and Eurasian watermilfoil stands in Cedar Lake.
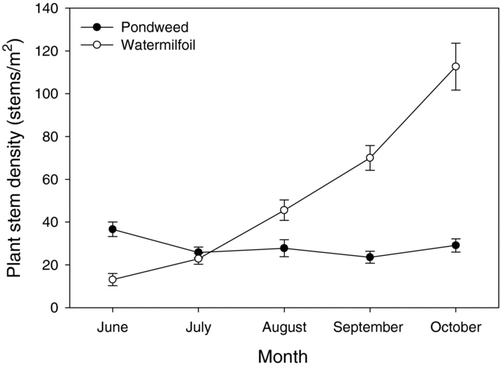
Invertebrate community composition differed between vegetated habitats, but invertebrate densities varied between habitats and across sampling periods. Eurasian watermilfoil stands supported higher invertebrate density per stem than American pondweed stands (P = 0.014, F = 6.5, df = 1). Sampling date also had a significant effect on invertebrate density (P < 0.0001, F = 8.9, df = 4), with higher invertebrate densities in June than any other sampling date. No significant interaction between macrophyte type and sampling date was found for invertebrate density (). A total of 39 different invertebrate taxa were identified, with 37 taxa found in American pondweed stands and 28 taxa found in Eurasian watermilfoil stands. Invertebrate densities ranged from 149 to 2758 individuals/m2 in American pondweed stands and 92 to 129,775 individuals/m2 in Eurasian watermilfoil stands. The most common groups of invertebrates living in the epiphytic community in both habitats were nematodes, cladocera, chironomids, copepods and ostracods. Amphipods and planaria were also common in stands of American pondweed.
Discussion
Juvenile sunfish preferentially utilized vegetated habitats over nonvegetated areas in Cedar Lake. Differences in plant stem density and invertebrate density between 2 macrophyte types were measured, but these differences did not translate into differences in juvenile sunfish densities within these habitats. Juvenile sunfish used vegetated habitats extensively, but no differences in habitat use by plant species were observed. These results are consistent with previous studies, which reported juvenile sunfish are typically associated with densely vegetated habitat to minimize threats from size-specific predators (CitationHall and Werner 1977, CitationWerner et al. 1977, CitationMittelbach 1981, Citation1984).
CitationValley and Bremigan (2002a) found largemouth bass consumed fewer bluegills while foraging in simulated Eurasian watermilfoil stands than in native macrophyte communities. They determined that the canopy structure of Eurasian watermilfoil was difficult for foraging bass to penetrate, and watermilfoil stands may serve as a refuge for small bluegill. Though juvenile sunfish densities did not differ by macrophyte type in the present study, differences in bluegill size structure among vegetated habitats were observed. The high proportion of small bluegill in Eurasian watermilfoil stands suggests this habitat could offer superior protection from predators. Stands of Eurasian watermilfoil in Cedar Lake also had higher plant and zooplankton densities than American pondweed stands. These factors should make Eurasian watermilfoil an ideal habitat for very small fish avoiding predators.
It is generally believed that one of two factors is important to small fish seeking refuge from predators: stem density (CitationGotceitas and Colgan 1987) or macrophyte growth form (CitationLillie and Budd 1992). When introduced as a covariate to fish density, plant density did not explain additional variation in sunfish densities; therefore, these results were not consistent with either plant density or plant type influencing juvenile sunfish density, which suggests that some other factor is involved. Reservoir hydrology during the summer was an important environmental variable affecting fish densities within the littoral zone of Cedar Lake, as illustrated by the interaction among reservoir hydrology, plant type and sampling month (). As water levels remained near normal pool level, juvenile sunfish densities were higher in shallow American pondweed stands. Later, as water levels fell below pool level, vegetated shoreline areas became less accessible to the fish, and densities rapidly fell in these habitats. Conversely, fish density in Eurasian watermilfoil stands, which are more generally located in deeper water away from the shoreline, remained relatively unchanged with the changes in water level. The use of shallow vegetated habitats by bluegills has been documented previously (CitationPaukert and Willis 2002); however, the suitability of shallow littoral zone habitats in the face of fluctuating water levels has received little attention. One exception is a study by CitationChick and McIvor (1994), where in the littoral zone fish community in Lake Okeechobee, Florida, some monthly variation in fish density was related to concurrent changes in depth and vegetation standing crop. This demonstrates the importance of vegetation management efforts that promote both shallow and deep-water vegetation in systems with fluctuating water levels. In these systems, recruitment of juvenile sunfish may benefit by fish selecting deeper vegetated habitats as water levels fall.
Figure 5 Interaction between Lepomis density (± 1 SE) within vegetated habitats and water level data in Cedar Lake. Normal pool level for Cedar Lake is indicated with a dashed line.
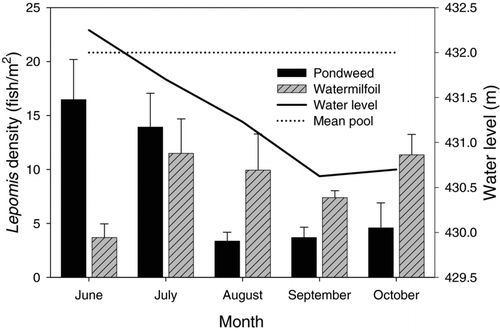
Plant type affected invertebrate density in Cedar Lake. Individual stems of Eurasian watermilfoil supported higher densities of invertebrates than stems of American pondweed, a plant having considerably simpler architecture. These results are in agreement with CitationKrecker (1939) who suggested that plants with finely divided leaves provide better habitat than plants with broad, simple leaves. A large portion of the variation in invertebrate densities was found in differences in zooplankton abundance between the 2 plant types. Cladocera were especially abundant in Eurasian watermilfoil stands, accounting for 44% of the total invertebrate catch. Similarly, CitationRobinson (1981) found cladocerans in lakes in British Columbia to be 5 times more abundant in Eurasian watermilfoil than other vegetated habitats.
Results from diet analyses indicate that all juvenile sunfish species preyed on similar resources. Microcrustaceans and dipteran larvae were dominant food items of all sunfish species in Cedar Lake. Similar diet habits have been reported for small sunfish in a variety of studies (e.g., CitationWerner and Hall 1977, CitationLaughlin and Werner 1980, CitationVanderKooy et al. 2000). Although direct competition was not measured in this study, such a high degree of overlap in these areas suggests that competition among juvenile sunfish species could result in a density-dependent slowing of growth in systems with limited resources. Such a situation would result in a longer time period when sunfish are exposed to size-specific predation and would likely adversely impact adult populations. As fish develop, morphological characteristics of adult sunfish allow a high degree of trophic specialization (CitationLauder 1983). Also, as body size increases and threats from size-specific predators decrease, habitat shifts among adult sunfish allow segregation of habitats among species (CitationCrowder and Cooper 1982). Size-specific predation threats limit juveniles of co-occurring sunfish species to densely vegetated habitats and increases the likelihood of inter- and intraspecific competition (CitationMittlebach 1984). Accordingly, the juvenile period in sunfish represents a potential bottleneck of recruitment and final adult size.
In conclusion, we found differences in invertebrate density and sunfish size structure among different vegetated habitats in Cedar Lake, but sunfish densities were similar among vegetated habitats. Instead, sunfish density within macrophyte stands was linked to changing water levels in the reservoir. These findings should aid in managing sunfish and vegetation in 2 ways. First, dense populations of slow growing sunfish are characteristics of many small vegetated systems. This and other studies have found smaller bluegill associated with Eurasian watermilfoil. Moreover, it is also known that largemouth bass are inefficient consumers of bluegill in complex environments of canopy-forming macrophytes such as Eurasian watermilfoil. In systems with high densities of slow growing bluegill, any vegetation management efforts should concentrate on replacing Eurasian watermilfoil stands with structurally diverse native plant communities (e.g., with low-dose applications of fluridone herbicide; CitationValley and Bremigan 2002b). Through the establishment of diverse submerged vegetation communities, predators will gain access to small bluegill, increasing the growth rates of both predator and prey fish. Second, we found juvenile sunfish moved out of the shallower American pondweed habitats as water levels fell, whereas fish density in deeper Eurasian watermilfoil habitats remained unchanged. This phenomenon likely occurs frequently because many artificial reservoirs are prone to dramatic water level fluctuations. In these systems, vegetation management efforts should be aimed at replacing Eurasian watermilfoil stands below the depth of water level fluctuation as well as in shallow habitats. Maintaining structurally diverse native plant communities in deep water habitats should allow switches in habitat use between predators and prey as water levels fluctuate.
Acknowledgement
The authors would like to thank Emily Wonnacott, Cliff O'Neal, Brian O'Neil, and Rob Colombo for their assistance in data collection and analysis. This research was sponsored by the Fisheries Division of the Illinois Department of Natural Resources, funded through the Federal Aid in Sportfish Restoration Act (F-140-R).
References
- Abdi , H. 2007 . “ Bonferroni and Šidák corrections for multiple comparisons ” . In Encyclopedia of Measurement and Statistics , Edited by: Salkind , N J . 103 – 106 . Thousand Oaks (CA) : Sage .
- Chick , J H and McIvor , C C . 1994 . Patterns in the abundance and composition of fishes among beds of different macrophytes: viewing the littoral zone as a landscape . Can J Fish Aquat Sci , 51 : 2873 – 2882 .
- Crowder , L B and Cooper , W E . 1982 . Habitat structural complexity and the interaction between bluegills and their prey . Ecology , 63 : 1802 – 1813 .
- Dewey , M R , Richardson , W B and Zigler , S J . 1997 . Patterns of foraging and distribution of bluegill sunfish in a Mississippi River backwater: influence of macrophytes and predation . Ecol Freshw Fish , 6 : 8 – 15 .
- Gotceitas , V and Colgan , P. 1987 . Selection between densities of artificial vegetation by young bluegills avoiding predation . Trans Am Fish Soc , 116 : 40 – 49 .
- Hall , D J and Werner , E E . 1977 . Seasonal distribution and abundance of fishes in the littoral zone of a Michigan lake . Trans Am Fish Soc , 106 : 545 – 555 .
- Hatzenbeler , G R , Jennings , M J , Emmons , E E and Bozek , M A . 2000 . Seasonal variation in fish assemblage structure and habitat structure in the nearshore littoral zone of Wisconsin lakes . N Am J Fish Manage , 20 : 360 – 368 .
- Johnson , SL . 1993 . Cover choice by bluegills: orientation of underwater structure and light intensity . Trans Am Fish Soc , 122 : 148 – 154 .
- Killgore , K J , Morgan , R P and Rybicki , N B . 1989 . Distribution and abundance of fishes associated with submersed aquatic plants in the Potomac River . N Am J Fish Manage , 9 : 101 – 111 .
- Krecker , FH . 1939 . Animal population of submerged aquatic plants . Ecology , 20 : 553 – 562 .
- Lalonde , S and Downing , J A . 1992 . Phytofauna of eleven macrophyte beds of differing trophic status, depth, and composition . Can J Fish Aquat Sci , 49 : 992 – 1000 .
- Lauder , GV . 1983 . Functional and morphological bases of trophic specialization in sunfishes (Teleostei, Centrarchidae) . J Morphol , 178 : 1 – 21 .
- Laughlin , D R and Werner , E E . 1980 . Resource partitioning in two coexisting sunfish: pumpkinseed (Lepomis gibbous) and northern longear sunfish (Lepomis megalotis peltastes) . Can J Fish Aquat Sci , 37 : 1411 – 1420 .
- Lillie , R A and Budd , J. 1992 . Habitat architecture of Myriophyllum spicatum as an index to habitat quality for fish and macroinvertebrates . J. Freshw. Ecol. , 7 : 113 – 125 .
- Mittelbach , GG . 1981 . Foraging efficiency and body size, a study of optimal diet and habitat use by bluegills . Ecology , 62 : 1370 – 1386 .
- Mittelbach , GG . 1984 . Predation and resource partitioning in two sunfishes (Centrarchidae) . Ecology , 65 : 499 – 513 .
- Paukert , C P and Willis , D W . 2002 . Seasonal and diel habitat selection by bluegills in a shallow natural lake . Trans. Am. Fish Soc. , 131 : 1131 – 1139 .
- Robinson , MC . 1981 . The effect of Eurasian watermilfoil (Myriophyllum spicatum L.) on fish and waterfowl in the Okanagan Valley, 1977 Assessment and Planning Division, British Columbia Ministry of Environment, Bulletin Number 15
- SAS . 2005 . JMP User guide. Version 6 , Cary (NC) : SAS Institute Inc .
- Savino , J F and Stein , R A . 1982 . Predator-prey interaction between largemouth bass and bluegills as influenced by simulated submersed vegetation . Trans. Am. Fish Soc. , 111 : 255 – 266 .
- Serafy , J E , Harrell , R M and Stevenson , J C . 1988 . Quantitative sampling of small fishes in dense vegetation: design and field testing of portable “pop-nets.” . J. Appl. Ichthyol. , 4 : 149 – 157 .
- Strauss , RE . 1979 . Reliability estimates for Ivlev's electivity index, the forage ratio, and a proposed linear index of food selection . Trans. Am. Fish Soc. , 108 : 344 – 352 .
- Valley , R D and Bremigan , M T . 2002a . Effects of macrophyte bed architecture on largemouth bass foraging: implications of exotic macrophyte invasions . Trans. Am. Fish Soc. , 131 : 234 – 244 .
- Valley , RD and Bremigan , MT . 2002b . Effects of selective removal of Eurasian watermilfoil on age-0 largemouth bass piscivory and growth in southern Michigan lakes . J. Aquat. Plant Manage. , 40 : 79 – 87 .
- VanderKooy , K E , Rakocinski , C F and Heard , R W . 2000 . Trophic relationships of three sunfishes (Lepomis spp.) in an estuarine bayou . Estuaries , 23 : 621 – 632 .
- Weaver , M J , Magnunson , J J and Clayton , M K . 1997 . Distribution of littoral fishes in structurally complex macrophytes . Can. J. Fish Aquat. Sci. , 54 : 2277 – 2289 .
- Werner , E E and Hall , D J . 1977 . Competition and habitat shift in two sunfishes (Centrarchidae) . Ecology , 58 : 869 – 876 .
- Werner , E E , Hall , D J , Laughlin , D R , Wagner , D J , Wilsmann , L A and Funk , F C . 1977 . Habitat partitioning in a freshwater fish community . J. Fish Res. Board. Can. , 34 : 360 – 370 .
- Werner , E E , Mittelbach , G G , Hall , D J and Gilliam , J F . 1983 . Experimental tests of optimal habitat use in fish: the role of relative habitat profitability . Ecology , 64 : 1525 – 1539 .