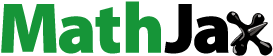
Abstract
Biotechnological and pharmaceutical processes based on bacteria require an inoculum with high long-term viability. In order to provide a stabilized bacterial culture for biodegradation of ammonia and ammonium, the model species Kocuria rhizophila DSM 348 was spray-dried using different protective agents. Providing a medium with 200 g/L skimmed milk powder (SMP) and 12.5 g/L monosodium L-glutamate (MSG) resulted in a survival rate of 99.1% ± 3.6% immediately after spray drying, whereas 200 g/L trehalose and 12.5 g/L MSG led to a lower viability (34.7% ± 5.9%). A reference medium with phosphates (7 g/L KH2PO4 + 7 g/L K2HPO4) accomplished a survival rate of 9.8% ± 2.9%. Long-term experiments confirmed high viability of spray-dried bacteria after 52 weeks at 4 °C with less than 1 log-reduction compared to the first day of storage, resulting in powders with at least 1010 CFU/g.
1. Introduction
Bacteria belonging to the genus Kocuria are mostly commensal microorganisms characterized by metabolic versatility. In consequence they have potential for numerous applications in biotechnological and pharmaceutical processes.[Citation1] Typical for Kocuria species is the production of yellowish, orange, or reddish pigments resulting in a distinct color attributed to different kinds of carotenoids.[Citation2] Following biosynthesis and down-stream processing, carotenoids from Kocuria are applied as functional ingredients for nutritional supplements, or pharmaceutical products.[Citation2] In addition to pigment production, K. rhizophila is also used in bioremediation to remove heavy metals from contaminated water,[Citation3] and to biodegrade volatile organic compounds from exhaust gases.[Citation4] Concerning cultivation conditions, growth of K. rhizophila is observed between 10 °C and 40 °C, when pH value of the medium is between 5.7 and 7.5. The gram-positive bacterium tolerates salt concentrations up to 15% (w/v) NaCl.[Citation5]
In order to generate an appropriate inoculum for microbial processes, such as biodegradation, cells have to be reproducibly provided in high viability, preferably in preserved form. One promising preservation method is spray drying, which is characterized by lower fixed and manufacturing costs compared to freeze drying.[Citation6] Spray drying is an established technology for microencapsulation of food bioactive components[Citation7] and was successfully applied by several authors for both probiotic bacteria[Citation6,Citation8–10] and yeasts.[Citation11–14] However, it has been demonstrated that spray drying leads to decreased cell viability due to exposition to high temperatures and removal of water. As for spray drying microorganisms are dispersed into a flow of hot air with up to 200 °C, thermal inactivation is an inherent consequence. Despite of evaporative cooling, temperatures are high enough to result in protein denaturation and disintegration of cell membrane structures. Another main reason for the reduction of viability during spray drying is dehydration. Biomolecules including proteins, nucleic acids, and lipids depend on the presence of some water to fulfill their cellular function. Low-water content as a consequence of drying causes damages to the cell membrane, the primary site of inactivation by dehydration.[Citation15,Citation16]
In order to reduce the negative impacts resulting from thermal stress and dehydration, protective agents can be added during the cultivation of the cells, or immediately before spray drying. Though a large variety of protectants has been proposed, most of them belong to the substance classes of oligosaccharides, polysaccharides, amino acids, and proteins. One of the main protective functions responsible for improving the survival rate is the replacement of the water bound to hydrophilic cellular structures by the protective agents. As a consequence, the native conformation of the proteins is preserved in the absence of water, leading to improved viability of the microorganisms after drying.[Citation17,Citation18] Accumulation of protective agents before desiccation paves the way for entering anhydrobiosis, a state which is characterized by extremely low metabolic activity. Certain microbial species remain viable in this state for years and are able to recover completely after rehydration.[Citation18] Besides addition of protective agents, the survival rate is also affected by several parameters of the spray drying process, including the feed rate, the processing time, the spray gas flow rate, and the composition of the drying medium with regard to solid and water content.[Citation6]
As the vast majority of previous studies is focused on preservation of lactic acid bacteria for manufacturing of starter cultures,[Citation6,Citation8–10,Citation19] it remains unclear if similar methods are also suitable for stabilizing species from other phylogenetic groups. For this reason, a spray drying process was developed to preserve cultures of the model organism K. rhizophila, which was selected due to its high relevance to our research group in addition to several established biotechnological applications.[Citation1–4] A current research objective is the selection of microorganisms capable of degrading ammonia (NH3) and ammonium (NH4+) derived from poultry houses. In first experiments, K. rhizophila showed a high degradation rate and was therefore chosen as a model species for the preservation experiments. In order to deliver a long-term stable powder for inoculation of the bioprocess, spray drying was evaluated under various experimental conditions. First, cultivation time was investigated to analyze the influence of the growth phase of the bacterial population on the survival rate. Subsequently, the inlet temperature during spray drying was varied to determine the influence of the incoming heat-flow on bacterial viability. In order to provide an extended shelf life, several protective agents were selected as components for adaptation media during cultivation and as carrier materials for microencapsulation by spray drying. Finally, storage experiments were conducted with powders containing either protective agents, or phosphate salts (negative control) to evaluate the bacterial viability in the dried state for a total period of 52 weeks.
2. Materials and methods
2.1. Chemicals
Agar (Kobe I), yeast extract, peptone (ex casein, tryptically digested), D-glucose, potassium dihydrogenphosphate (KH2PO4), dipotassium hydrogenphosphate (K2HPO4), and skimmed milk powder (SMP) were obtained from Carl Roth GmbH + Co. KG (Karlsruhe, Germany). Monosodium L-glutamate (MSG) was provided as a monohydrate by Merck KGaA (Darmstadt, Germany). Trehalose was purchased from GM GesundheitsManufaktur GmbH (Frankfurt a.d. Oder, Germany). Agar, yeast extract, peptone, and SMP were of microbiological grade, D-glucose, KH2PO4, K2HPO4, and MSG of analytical grade (p.a.), and trehalose of food grade.
2.2. Bacterial strain
Kocuria rhizophila DSM 348 was obtained as freeze-dried culture from the German Collection of Microorganisms and Cell Cultures GmbH (Braunschweig, Germany). The strain was selected for its high degradation rate of NH3 and NH4+. Furthermore, K. rhizophila was successfully applied previously to degrade volatile organic compounds (VOCs) in lab-scale experiments and in an industrial biotrickling filter.[Citation4]
2.3. Media composition and sterilization
2.3.1. YPD medium and YPD agar
Cultivation of K. rhizophila in flasks was performed in YPD medium, which was prepared by dissolving 10 g yeast extract, 20 g peptone, and 20 g D-glucose in 1 L of deionized water. The medium was sterilized by autoclaving at 121 °C for 20 min. In order to prevent loss of bioavailable nutrients due to Maillard reaction, D-glucose was sterilized separately from yeast extract and peptone in an autoclave (Fedegari FVA/A1, ibs | tecnomara GmbH, Fernwald, Germany). YPD agar was used to cultivate bacterial colonies. Addition of agar to YPD medium in a final concentration of 15 g/L resulted in YPD agar which was sterilized in the autoclave, as described above.
2.3.2. Phosphate buffer
Phosphate buffer (pH 6.8) was applied for serial dilutions and partially for resuspension of cells before spray drying. It was produced by mixing 7 g KH2PO4 and 7 g K2HPO4 with 1 L of deionized water before autoclaving under the same conditions as YPD medium and YPD agar.
2.3.3. Protective media
A mixture of protective agents was selected for experiments with K. rhizophila generating two different compositions of encapsulation matrix to increase the viability of the cells:
A: Trehalose (200 g/L) + MSG (12.5 g/L)
B: SMP (200 g/L) + MSG (12.5 g/L)
The values in brackets refer to the final concentration of each substance in the protective medium, which was used to resuspend bacterial cells before spray drying. Final concentration values are based on a previous study.[Citation20] Deionized water was used as a solvent for all protective agents.
In order to reduce the risk of contamination of the spray-dried samples, initial microbial load of the protective agents was reduced by heating. Trehalose + MSG were sterilized by autoclaving at 121 °C for 20 min. Undesirable heat-mediated reactions between the two components were avoided by preparing stock solutions in separate flasks for each substance. SMP was not autoclaved to prevent thermal denaturation of proteins as well as any Maillard reaction. Instead, pasteurization at a temperature of 70 °C for 30 min in a drying cabinet was selected for decontamination. SMP was dissolved in deionized water in separate flasks before incubation in the drying cabinet.
2.3.4. Adaptation media
Media for adaptation of bacteria to protective agents were prepared by adding the following substances to YPD medium (final concentration in brackets):
A: Trehalose (12.5 g/L)
B: SMP (12.5 g/L)
2.4. Culture conditions
The freeze-dried culture of K. rhizophila was mixed with liquid YPD medium, transferred onto a YPD agar plate and incubated at 28 °C for 48 h. Grown colonies served as a stem culture and were used to inoculate new YPD agar plates as subcultures after 4 weeks of storage at 4 °C. One single colony was collected with a sterilized loop and resuspended in liquid YPD medium to generate pre-cultures for each experiment. Inoculated flasks were incubated in a rotary shaker (Certomat BS-1, Sartorius AG, Göttingen, Germany) at 150 rpm and 28 °C. The incubation time depended on the type of experiment and is given in the corresponding paragraph.
2.5. Growth curve
In order to determine the optimal cell harvest time, resulting in a preferably high biomass yield for spray drying experiments, the growth of K. rhizophila was analyzed in liquid YPD medium for a total incubation time of 72 h in triplicate. Additionally, the effect of adaptation media (composition given in Section 2.3.4) on bacterial growth was investigated by exchanging the culture medium (YPD) after 24 h. Therefore, cells were separated by centrifugation at 2,400 g for 10 min at 20 °C and resuspended with the corresponding adaptation medium after removing the supernatant. Each flask had a total volume of 500 mL, but was filled with only 150 mL of medium to ensure sufficient mixing of air and liquid, providing a high concentration of dissolved oxygen for ideal growth of aerobic bacteria. During incubation at 150 rpm and 28 °C, samples were taken at an interval of 24 h to analyze the cell concentration by the plate count method with YPD agar. A tenfold serial dilution was performed using 900 µL of phosphate buffer and an initial sample volume of 100 µL. Subsequently, a volume of 50 µL of the diluted samples was plated on YPD agar in duplicate before incubation at 28 °C. After 48 h, the number of colony-forming units (CFU) was determined for each agar plate.
2.6. Spray drying workflow
Liquid media with K. rhizophila were dried using a laboratory spray dryer (B-290, Büchi Labortechnik AG, Flawil, Switzerland). The device was operated in the co-current mode for all experiments. A two-fluid nozzle with a tip diameter of 0.7 mm was used to mix compressed air as spray gas with liquid medium delivered by a peristaltic pump integrated in the laboratory spray dryer with a constant flow rate of 11 mL/min. The atomization pressure was set to 0.7 MPa. Drying air flow rate was adjusted by the aspirator to 100%, which is equal to 35 m3/h. Additionally, the spray gas flow was defined using a rotameter (Q-Flow V-100, Vögtlin Instruments GmbH, Muttenz, Switzerland) set to a height of 45 mm concerning the levitating sphere. This height indicates a spray gas flow of 0.536 m3/h.
After adjusting the specific parameters for each experiment, the bacteria suspension was transferred by a peristaltic pump to the nozzle, serving as a feed for the spray dryer. A magnetic stirrer (MR 3000, Heidolph Instruments GmbH & Co. KG, Schwabach, Germany) at 150 rpm was installed to prevent sedimentation of the bacteria within the medium. The inlet temperature was set individually dependent on the purpose of each experiment and refers to the inflowing air after passing an electric heater before being dispersed by the nozzle. The outlet temperature was significantly influenced by the inlet temperature and was measured in the air space between the spray tower and the cyclone. The maximum value of the outlet temperature was recorded and used to evaluate the heat-induced cellular stress.
The dried powder was collected at the bottom of the cyclone and transferred into sterile, transparent polypropylene centrifuge tubes with a total volume of 50 mL. The head space within the centrifuge tubes was not filled with inert gas. Experiments were conducted in triplicate resulting in three different powder samples for each approach to evaluate the reproducibility of the entire process.
2.7. Storage of samples
After spray drying, centrifuge tubes with powder samples were stored at 4 °C. Cellular damages by light, e.g. photoinduced DNA mutations, were avoided by storage in a refrigerator (KS-PROFI1400, GastroHero GmbH, Dortmund, Germany) with a nontransparent housing, except during short sampling periods of 2 min to analyze the survival rate.
2.8. Characterization of samples
2.8.1. Determination of water content and solid content
Water content was analyzed thermogravimetrically using an infrared drying scale (IR-35, Denver Instrument GmbH, Göttingen, Germany) with a temperature of 150 °C and an initial sample mass of 0.5 g. Based on the mass difference during drying, the water content was indicated automatically by the device as a percentage value. Determination of water content of the powders was conducted 5 min after spray drying. Bacterial biomass was analyzed 5 min after centrifugation (GR4-22, Gemini BV, Apeldoorn, Netherlands) using the pellet from the centrifuge tube as sample. Solid content (S, in %) was calculated based on the experimentally determined water content (W, in %) applying the following equation:
(1)
(1)
2.8.2. Enumeration of viable cells
Bacterial viability before and after spray drying was evaluated by the plate count method with YPD agar, as described in Section 2.5. In order to guarantee the comparability of results necessary for the determination of survival rate, the bacterial count was referred to the solid content of the sample before and after spray drying. By this approach, the influence of added substances, as protective agents and phosphates, which significantly increase the solid content apart from bacteria, was taken into account. A volume of 25 mL of phosphate buffer was added to rehydrate the spray-dried powder (mass dependent on solid content) in a centrifuge tube using a vortex mixer to provide a thoroughly homogenized suspension. After rehydration, a partial volume of 100 µL was used to determine the viability by the plate count method, as described before. The survival rate relative to liquid medium before spray drying (R1, in %) was calculated based on the bacterial counts before spray drying (N0, in CFU/mL) and immediately after spray drying (N1, in CFU/mL) using the following equation:
(2)
(2)
Concerning storage experiments, the time-dependent survival rate (R(t), in %) was determined from bacterial counts immediately after spray drying (N1, in CFU/mL) and after storage (N(t), in CFU/mL) by the following equation:
(3)
(3)
2.8.3. Microscopic analysis
The morphology of spray-dried powder was analyzed by a confocal laser scanning microscope (CLSM, VK-X3000, Keyence Corporation, Neu-Isenburg, Germany). The sample was transferred on a microscope slide and placed on the object plate. In contrast, to electron microscopy, e.g. SEM, neither coating nor vacuum was applied for CLSM analysis.
2.9. Spray drying experiments
2.9.1. Effect of cultivation time
Initially, two different cultivation times of K. rhizophila were selected according to the growth curve to maximize the biomass yield. The objective was to investigate whether the cellular age of the bacterial culture, determined by the growth phase at the time of harvesting, influences the survival rate. Besides, the effect of cultivation time on water content of the powders and on the outlet temperature during spray drying was studied. After cultivation for 24 h or 48 h, cells were separated by centrifugation at 2,400 g for 10 min at 20 °C and resuspended with phosphate buffer after removing the supernatant. Samples were spray-dried using an inlet temperature of 140 °C.
2.9.2. Effect of inlet temperature
Three different inlet temperatures were chosen to investigate the influence of the incoming heat-flow on bacterial survival, water content of the powder, and the outlet temperature in the spray dryer. According to the pertinent literature, the temperature of the inflowing air has a significant impact on the survival rate of microorganisms after spray drying.[Citation6,Citation16] Based on publications in the field of microbial preservation,[Citation6,Citation8,Citation9,Citation18] 120 °C and 160 °C were selected as inlet temperatures, additionally to already tested value of 140 °C (see Section 2.9.1).
2.9.3. Effect of protective agents
After selecting a suitable cultivation time and inlet temperature, different protective agents (given in Section 2.3.3) were investigated for their ability to increase the survival rate of bacteria after spray drying and during refrigerated storage at 4 °C.
In order to adapt the cells to the protective agents promoting increased tolerance against desiccation, the medium used in the bacterial cultures was exchanged after an incubation time of 24 h. For this purpose, cells were separated by centrifugation at 2,400 g for 10 min at 20 °C. After discarding the supernatant, the bacteria were resuspended with an adaptation medium containing the protective agents specified in Section 2.3.4.
After 48 h of incubation in the rotary shaker, culture broth with K. rhizophila was centrifuged at 2,400 g for 10 min at 20 °C to separate the cells from liquid components. The supernatant was discarded and the bacteria were resuspended with the same volume of protective medium, as described in Section 2.3.3. The pH value of resuspended samples was determined via pH meter (Giorgio Bormac S.r.l., Carpi, Italy).
Additionally, a negative control was implemented by using phosphate buffer with a solid concentration of 14 g/L (7 g/L KH2PO4 + 7 g/L K2HPO4) to resuspend the cells. Bacterial cultures treated with phosphate buffer did not receive any adaptation medium after an incubation time of 24 h. Samples were spray-dried using an inlet temperature of 140 °C. Storage experiments were performed with three powders for each protective agent as well as for the negative control, resulting from three spray drying experiments. Samples were stored, as described in Section 2.7, for a period of 52 weeks. Viability of bacteria was measured regularly according to Section 2.8.2.
2.10. Statistical analysis
All experiments were performed in triplicate. The results were expressed as arithmetic mean ± standard deviation. Data analysis was conducted using Microsoft Excel 2016. The results were compared by the Student’s t-test statistical method with a significant difference at P < 0.05.
3. Results and discussion
3.1. Growth curve
Initially, the time-dependent growth behavior of K. rhizophila in liquid YPD medium and adaptation media was analyzed to determine the best time for cell harvest at high biomass yield for subsequent spray drying experiments. illustrates the development of the cell concentration in the culture over an incubation time of 72 h. The maximum cell concentration is achieved 48 h after inoculation. Presumably, the population at this time enters the stationary phase or is in the late exponential phase. After 72 h, the cell count decreases compared to the previous value, indicating transition to the death phase. Based on the growth curve, harvesting biomass at 48 h provides the maximum yield of viable bacteria. Both adaptation media are characterized by a similar cell concentration which is significantly higher than in YPD medium after 48 h and 72 h. The positive effect on microbial growth can be explained by the exchange of medium after 24 h, providing additional nutrients for the bacteria. indicates that growth in adaptation media is independent from the type of protectant, as no statistically significant difference was observed between trehalose and SMP regarding cell concentrations after 24 h and 72 h. According to literature,[Citation5] K. rhizophila is not able to utilize the disaccharides trehalose and lactose as substrates for acid production. As SMP consists of 51% − 57% lactose, residual ingredients (predominantly milk proteins) might also not be bioavailable for the bacteria under the cultivation conditions in this study.[Citation21] Therefore, the increase of cell concentration in adaptation media results from yeast extract, peptone, and D-glucose, which were a priori included in YPD medium and suitable substrates for K. rhizophila. Timkina et al.[Citation22] also observed a maximum growth after 48 h of cultivation regarding two species of the genus Kocuria obtained from radon spring water, when incubated in LB medium in a rotary shaker with 150 rpm at 30 °C.
3.2. Effect of cultivation time
After evaluation of microbial growth, spray drying experiments were conducted focusing on the cultivation time of bacterial cells. As described in the previous section, the cell concentration reached a maximum after 48 h of cultivation indicating high biomass yield. In addition, another point of time during cultivation was selected to harvest the cells. indicates that bacteria enter the death phase after 72 h of cultivation. Therefore, using the respective cultures could have negative effects on the viability of the cells during spray drying. It is assumed that microorganisms with cellular damages a priori, e.g. due to depletion of nutrients or accumulation of toxic metabolites, are more sensitive toward heat-induced stress and desiccation leading to decreased survival rates.[Citation6] For this reason, bacterial cultures after 72 h of incubation were not selected for spray drying experiments. Instead, cells from the exponential growth phase harvested after 24 h were used, despite of the lower biomass yield indicated by cell concentration.
illustrates three characteristic spray drying parameters to compare the two selected cultivation times. Taking into account the standard deviation based on experiments in triplicate, there is no significant difference in the survival rate of the bacteria which is 11.1% ± 1.6% after 24 h, and 9.8% ± 2.9% after 48 h. Regarding the given percentages of viability, it has to be considered that the absolute number of bacteria resuspended in phosphate buffer before spray drying was higher after 48 h than after 24 h of cultivation, as indicated by the growth curve in .
Figure 2. Effect of cultivation time on survival rate relative to liquid medium before spray drying, water content and outlet temperature during spray drying of Kocuria rhizophila in phosphate buffer using an inlet temperature of 140 °C. n = 3.
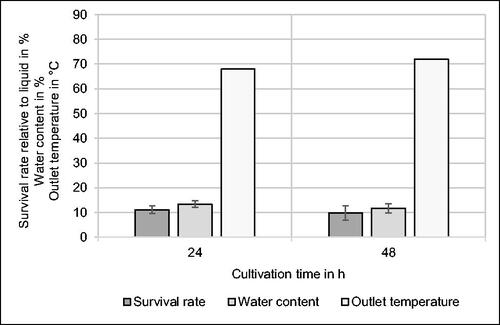
There is evidence from literature that a difference in the number of cells used in spray drying feed influences the survival rate after spray drying.[Citation9] Increasing the cell concentration of Lactobacillus rhamnosus GG from 109 CFU/mL to 1010 CFU/mL in phosphate-buffered saline (PBS) resulted in a significantly lower survival rate.[Citation9] However, the effect of cell concentration on the viability after spray drying depends on several parameters, e.g. bacterial species, or composition of the drying and rehydration media.[Citation9] Experiments with Pediococcus acidilactici CFR2193 demonstrated that an increase in cell concentration from 1% (w/v) to 5% (w/v) led to a decrease in the survival rate when maltodextrin was used as a carrier material.[Citation23] Substitution of maltodextrin by nonfat skimmed milk did not result in a lower viability of bacteria when the cell concentration was increased.[Citation23] Regarding the growth phase, harvesting cells at the stationary phase had a beneficial effect on the viability of lactic acid bacteria after spray drying according to the literature.[Citation6] It is proposed that microorganisms entering the starvation mode become more resistant toward osmotic and heat stress.[Citation6] In this study, the number of bacteria in the spray drying feed as well as the growth phase at cell harvest time did not significantly influence the survival rate using phosphate buffer as a resuspension medium. As experimental conditions, e.g. bacterial species, cell concentration, feed rate, and inlet temperature, are not identical with previous studies,[Citation6,Citation9,Citation23] the observed differences seem plausible.
The water content of spray-dried powders with K. rhizophila shows no significant, time-dependent differences, as values of 13.4% ± 1.3% after 24 h, and 11.7% ± 1.9% after 48 h are given. As bacteria were cultivated in liquid YPD medium and resuspended in phosphate buffer, the water content before spray drying was at least 95%. Therefore, significant dehydration was induced causing inactivation of microorganisms which was quantified by a survival rate of approximately 10%. As a consequence, 90% of viable cells were inactivated by dehydration or thermal denaturation representing the main causes for microbial inactivation regarding spray drying.[Citation15,Citation16] Broeckx et al.[Citation9] report a lower water content (3.95% − 6.75%) compared to our study for powders containing L. rhamnosus GG using a different feed rate, inlet temperature, and drying air flow rate. Shokri et al.[Citation24] obtained similar results for the water content (3.30% − 7.19%) after spray drying of Bifidobacterium bifidum. Experiments of Slavutsky et al.[Citation25] with L. acidophilus led to spray-dried powders with a moisture content of 5.41% − 10.35%. Generally, water content is regarded as an important parameter for the stability of dried microbial cultures, whereas optimal values depend on storage atmosphere and the species of bacteria. For preservation of microbial cells in dried dairy products, a residual moisture content of 4% is recommended.[Citation6] The water content referring to spray-dried powders in this study was approximately 10%, which is relatively high compared to literature.[Citation6,Citation9,Citation13,Citation24] As the moisture content is affected by inlet temperature and predominantly relevant for storage experiments, the corresponding discussion is given in Section 3.3 and Section 3.4, respectively.
For both cell suspensions, the same inlet temperature of 140 °C was used, which results in slightly different outlet temperatures with values of 68 °C after 24 h, and 72 °C after 48 h. The standard deviation was 0 in both cases. The resulting outlet temperatures are in accordance with the range of 60 °C − 80 °C, which led to good survival of lactobacilli.[Citation6] As the same resuspension medium was used for each sample after centrifugation, the 4 °C higher outlet temperature is likely to be caused by an increased solid content due to the improved biomass yield after 48 h.
This hypothesis is supported by the mass of powder collected from the cyclone, corresponding to 0.8874 g ± 0.0745 g after 24 h, and 2.1158 g ± 0.1785 g after 48 h. Regarding the stated values, it can be concluded that spray drying cells after 48 h increases the dried biomass yield by a factor of 2.4 compared to 24 h. Broeckx et al. [Citation9] also observed an increase of dry biomass yield by spray drying media with higher cell concentrations. Elevating the number of bacteria by a factor of 8 resulted in a 2.6 times higher yield.[Citation9]
3.3. Effect of inlet temperature
After the investigation of the cultivation time, the effect of different inlet temperatures on the spray drying outcome was evaluated using bacteria after 48 h of incubation to accomplish higher powder yields compared to 24 h. The results for three tested inlet temperatures are visualized in .
Figure 3. Effect of inlet temperature on survival rate relative to liquid medium before spray drying, water content and outlet temperature during spray drying of Kocuria rhizophila in phosphate buffer. n = 3.
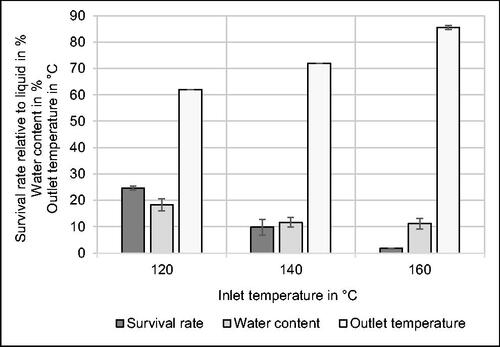
According to the data, the survival rate significantly declines with increasing inlet temperature. The highest viability is observed at 120 °C with 24.6% ± 0.8%. An increase of the inlet temperature by 20 °C leads to 9.8% ± 2.9% viable cells. The lowest survival rate corresponds to the highest thermal stress caused by 160 °C hot air resulting in a residual viable population of 1.8% ± 0.1%. The decline of viability by increasing inlet temperature was also confirmed by other studies.[Citation6,Citation9] The increase in outlet temperature with rising inlet temperature is plausible as well and in accordance with literature.[Citation6] Air temperature resulting after the evaporation of water in the spray dryer is regarded as a crucial parameter determining the viability of bacteria. An increase in outlet temperature is linked to reduced microbial survival due to cellular damage by an elevated level of heat stress. According to literature,[Citation6] a reduction of inlet temperature results in an increase of water content leading to a negative impact on the viability, when long-term storage of spray-dried powders is considered. Conversely, the microbial inactivation rate regarding stored samples is reduced as the moisture content decreases.[Citation6] A low water activity (aw) decelerates chemical reaction rates referring to molecular processes which cause cell death, e.g. lipid oxidation, and has therefore a positive effect on long-term viability of spray-dried bacteria.[Citation25]
Taking into account the development of the water content, illustrated in , no significant difference is observed between an inlet temperature of 140 °C and 160 °C. Due to the considerably higher survival rate, it was decided to perform the spray drying process for subsequent storage experiments at 140 °C. Although an inlet temperature of 120 °C would have led to an even higher viability according to the data shown in , the water content would have increased to 18.3% ± 2.4% under these conditions. As this value is regarded as too high to guarantee prolonged viability of bacteria in powders during storage according to literature,[Citation6,Citation9,Citation13,Citation24] an inlet temperature of 120 °C was not used for further spray drying experiments. As an alternative, a post-treatment step could be added to decrease the water content of the spray-dried powder. For example, a spray dryer can be combined with a fluidized bed dryer to produce agglomerated powder with very low water content and beneficial properties concerning rehydration.[Citation6]
3.4. Effect of protective agents
In order to increase the survival rate of K. rhizophila, two different formulations of protective agents were added during cultivation and used to resuspend the cells before spray drying providing additional carrier materials for microencapsulation. visualizes results from experiments with protective substances and phosphates as a negative control. Regarding the survival rate, each of the protectant mixtures significantly increases the yield of viable cells, compared to bacteria spray-dried with phosphates. The highest survival rate is achieved by SMP + MSG with 99.1% ± 3.6%, followed by trehalose + MSG (34.7% ± 5.9%). Addition of phosphates results in a survival rate of 9.8% ± 2.9%. The cell morphology was not affected by the additives. Bacteria were visible as non-motile cocci with a diameter of approximately 1 µm in accordance to the first description of the species.[Citation5] As K. rhizophila is a non-spore-forming bacterium,[Citation5,Citation26–28] a falsification of the survival rate by spores can be excluded. However, the pH of the medium after cell resuspension was influenced by the additives. Medium exchange by phosphates, SMP + MSG, and trehalose + MSG resulted in pH values of 6.8, 6.4, and 5.9, respectively. As culture medium after 48 h of incubation was characterized by pH 4.5, medium exchange with protectants and phosphates might have a beneficial effect because pH 5.7 − 7.5 is ideal for growth of K. rhizophila.[Citation5]
Figure 4. Effect of protective agents on survival rate relative to liquid medium before spray drying, water content and outlet temperature during spray drying of Kocuria rhizophila using an inlet temperature of 140 °C. Phosphates were used as a negative control. MSG Monosodium L-glutamate. SMP Skimmed milk powder. n = 3.
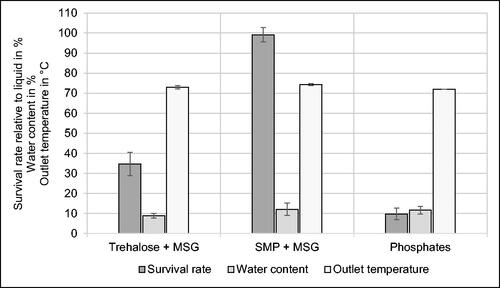
SMP is regarded as one of the most effective protective agents to increase the viability of bacteria during and after spray drying, as it combines the beneficial properties of proteins and carbohydrates.[Citation6] SMP consists to a large extent of proteins (33% − 37%) and lactose (51% − 57%).[Citation21] At molecular level, proteins and disaccharides interact with cell membrane components resulting in a stabilizing effect.[Citation19,Citation29] Several studies report successful application of SMP to increase the viability of lactic acid bacteria.[Citation30–35] Survival rate of L. acidophilus NCIMB 701748 could be improved by SMP from 0.44% ± 0.03% (with 100% maltodextrin) to 19.2% ± 3.7% using a mixture of 70% maltodextrin and 30% SMP.[Citation30] Experiments with L. acidophilus FTDC 3081 showed a viability of 70% after addition of SMP (200 g/L) compared to 25% without addition of protective agents.[Citation31] The survival rate of K. rhizophila preserved with SMP in this study is even higher than the one of lactic acid bacteria in the literature.[Citation30,Citation31] However, it must be taken into account that SMP was already added during cultivation and combined with another protective agent (MSG). Presumably, a synergistic beneficial effect resulted from this treatment increasing the survival rate. Addition of MSG also led to an enhanced viability of L. rhamnosus GG and L. rhamnosus E-97800.[Citation36] Experiments with L. casei LK-1 showed an improved protection of cells by skimmed milk compared to trehalose which is in accordance with the results of this study.[Citation37] Besides SMP, whey or whey proteins serve as milk-derived protectants for several microorganisms.[Citation34,Citation38–40]
Trehalose was also used by several other authors[Citation20,Citation41] as a protectant for spray drying of bacteria. The survival rate of L. plantarum TISTR 2075 was improved from 0.1% to 25% by adding trehalose (50 g/L) to the resuspended microorganisms.[Citation41] The viability of L. rhamnosus GG after spray drying was also positively influenced by trehalose in a concentration of 20% (w/w) leading to survival rate of 69%.[Citation20] Addition of 12.5 g/L of MSG combined with 20% (w/w) trehalose resulted in a further improvement keeping 81% of the cells alive.[Citation20]
PBS (0.23 g/L NaH2PO4 + 1.23 g/L Na2HPO4 + 8.2 g/L NaCl) applied by Broeckx et al.[Citation8] as a spray drying medium also had a positive impact on the survival rate of L. rhamnosus GG compared to saline (9 g/L NaCl). PBS resulted only in a 0.54 log-reduction of the viability after spray drying, in contrast to a 1.89 log-reduction when saline was used. Therefore, a stabilizing effect of inorganic phosphates on bacteria is proposed. On the cellular level, the positive influence on the viability might be related to the accumulation of polyphosphates by bacteria as well as to the osmotic and pH buffering capacity of phosphates.[Citation8]
Besides bacteria, also eukaryotic microorganisms have been spray-dried by several authors, e.g. yeasts as biocontrol agents to prevent proliferation of phytopathogens. In the following, three examples of yeast-related studies are described to compare the results produced with prokaryotic species K. rhizophila in this study. Spray drying of Rhodotorula mucilaginosa with 18% (w/v) maltodextrin and an inlet temperature of 140 °C resulted in a survival rate of 31.8% ± 4.5%.[Citation13] The yeast species Candida sake CPA-1 had a lower survival rate of 17.0%, when 10% (w/v) skimmed milk and an inlet temperature of 150 °C were used during spray drying.[Citation14] Romano et al. investigated 11 Saccharomyces cerevisiae strains suitable for wine production and described large varieties in the survival rate ranging from 0.02% ± 0.00% to 62.39% ± 5.05%, when 3% (v/v) skimmed milk and an inlet temperature of 120 °C were applied for spray drying.[Citation42]
illustrates the results of storage experiments. Starting values of 100% correspond to the bacterial count immediately after spray drying referring to 1010 CFU/g – 1011 CFU/g. Regarding the entire storage period, both protectants show slightly higher mean survival rates compared to phosphates as a negative control. However, there is no significant difference in most cases, taking into account the standard deviations. As discussed before, phosphates could have a positive effect on bacterial viability by different cellular mechanisms leading to an extended shelf life of spray-dried microorganisms. Broeckx et al. [Citation8] also report a beneficial effect of PBS on L. rhamnosus GG resulting in a survival rate of 15% after 28 weeks of storage at 4 °C. Using demineralized water as a spray drying medium generated more than 5 log-reductions in the same time.
Figure 5. Effect of protective agents on survival rate of Kocuria rhizophila relative to the spray-dried powder during 52 weeks of storage at 4 °C. Phosphates were used as a negative control. MSG Monosodium L-glutamate. SMP Skimmed milk powder. n = 3.
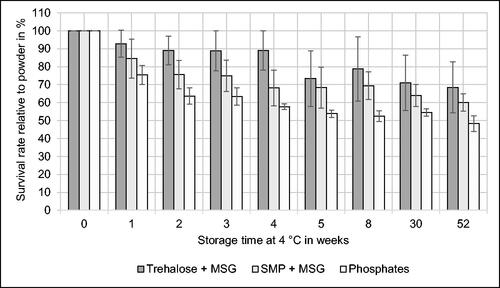
In previous studies,[Citation41,Citation43] an increase of bacterial viability by protein- and carbohydrate-based protectants was also observed. L. murinus PS85 spray-dried with 20% nonfat skimmed milk showed a survival rate of nearly 100% ± 20% after 60 days of storage at 5 °C. Experiments with Enterococcus raffinosus PS7 under the same conditions led to a viability of 95% ± 5%.[Citation43] However, the number of viable cells seems to decrease significantly when spray-dried powders are stored for several months. Regarding a storage temperature of 4 °C, L. plantarum TISTR 2075 showed a survival rate of 63% after 120 days and 32% after 360 days when trehalose (50 g/L) and maltodextrin DE 10 (200 g/L) were used as protectants. Spray drying of Streptococcus thermophilus applying a whey protein concentrate (40 g/L) resulted in a survival rate of 46% after 6 months and 14% after 12 months of storage at 6 °C.[Citation38] The survival rate of baker’s yeast (S. cerevisiae) spray-dried with 10% (w/v) maltodextrin reached a value of 78% ± 2% after a storage period of 180 days at 27 °C ± 2 °C.[Citation11] Another fungus, Beauveria bassiana, preserved by low-temperature spray drying with a mixture of protectants (10% dextrin, 10% skimmed milk, and 5% polyvinylpyrrolidone) exhibited 80% viability after storage for 6 months at 4 °C.[Citation44] Compared to the reference values stated above, the survival rates of K. rhizophila given in seem to be in the same order of magnitude and are therefore in accordance with previous studies dedicated to spray drying of gram-positive bacteria[Citation8,Citation38,Citation41,Citation43] and fungi.[Citation11,Citation44]
Relatively high standard deviations regarding the viability of bacteria in spray-dried powders were also observed by several other authors.[Citation36,Citation45,Citation46] In this study, standard deviation is based on three independent spray drying experiments indicating the reproducibility. Considering the inherent variability of the single procedures during experiments, e.g. initial growth of bacteria during the cultivation or operation of the spray dryer, it seems plausible that the results imply a certain variation.
Interpreting survival rates as percentages derived from the bacterial count, it is important to consider that corresponding numerical values in this study refer to at least 1010 CFU/g. This means that even viability percentages which seem to be low at first glance represent absolute values with a comparably high level. As given in , the water content of powders with protective agents and phosphates was approximately 10%. Despite the relatively high moisture content compared to literature[Citation6,Citation9,Citation13,Citation24] we observed a high viability in spray-dried powders during the storage experiments. Therefore, it was decided to keep the water content in the range of 10%, without performing subsequent drying. As K. rhizophila differs from lactic acid bacteria regarding phylogenesis, morphology, and metabolism, it is plausible that also the tolerance concerning the moisture content deviates from starter cultures for yogurt production.[Citation5,Citation19] Additionally, species of the genus Kocuria are found in challenging environments characterized by high levels of ionizing radiation, toxic heavy metals, and critical salt concentrations.[Citation3,Citation5,Citation22] Therefore, specific cellular adaptations are required which might be involved in maintaining viability during and after spray drying.
Regarding the intended application of the spray-dried product as an inoculum, the results summarized in confirm a sufficient preservation of viability with high absolute bacterial count in the powders if refrigerated storage is provided. A significant decrease of the survival rate was not observed during a test period of 52 weeks (1 year), corroborating a high long-term stability of spray-dried K. rhizophila. Concerning the survival rate of bacteria relative to liquid medium before spray drying and relative to spray-dried powder during storage, SMP + MSG provided the highest total viability. In order to visualize the morphology of microcapsules containing bacteria and SMP + MSG as protectans, CLSM micrographs of the corresponding powder are illustrated in . The spherical structure of the particles is characterized by surface concavities which have been described by several authors[Citation30,Citation47,Citation48] regarding SEM micrographs of spray-dried powders. The partially collapsed, wrinkled shape is caused by high spray drying temperatures and is characteristic for microcapsules containing proteins derived from milk.[Citation30,Citation48] However, a disintegration of microcapsules by fissures or cracks on the surface is not visible in . Therefore, the structure seems to provide robust protection of encapsulated bacteria maintaining a high viability of cells during and after spray drying which was quantitatively confirmed by survival rates in and .
4. Conclusion
In this study, biotechnologically relevant bacteria belonging to the species K. rhizophila were spray-dried using different cultivation times, inlet temperatures, and protective agents. 48 h of cultivation led to an increase of dried biomass yield by a factor of 2.4 compared to 24 h of incubation. A medium exchange after 24 h generated significantly higher cell concentrations, when adaptation media with protective agents were used. However, the type of protectant did not affect the cell concentration. Investigation of three different inlet temperatures resulted in the conclusion that 140 °C (corresponding to an outlet temperature of 72 °C) represent a suitable compromise referring to a preferably low water content and high viability of bacteria. Protective agents added during cultivation and before spray drying significantly increased the survival rate after the drying process compared to phosphates as a negative control. Addition of SMP + MSG allowed preservation of 99.1% ± 3.6% of cells compared to the bacterial count before spray drying, whereas trehalose + MSG led to a lower percentage of viable cells (34.7% ± 5.9%). In subsequent long-term experiments at 4 °C, trehalose + MSG provided the highest mean survival rate (68.5% ± 14.2%) after a period of 52 weeks compared to the first day of storage, whereas SMP + MSG resulted in 60.1% ± 4.8%. Phosphates accomplished a slightly lower percentage of viable cells (48.3% ± 4.3%) after 52 weeks. The moisture content of powders with protective agents and phosphates was relatively high, achieving values of approximately 10%. In the future, a subsequent drying step could be added after spray drying to reduce the water content as an alternative approach to increase the survival rate during long-term storage. In order to determine if phosphates factually exert a positive effect on the viability of K. rhizophila, the experimental approach could be expanded. For example, cells could be resuspended in saline (without phosphates) before spray drying to prevent lethal damages from osmosis which might result if deionized water is used as a drying medium.
As SMP + MSG provided a significantly higher survival rate referring to the bacterial count before spray drying compared to trehalose + MSG and phosphates, it is regarded as the most effective formulation of protective agents for preservation of K. rhizophila.
Regarding a future scale-up, the presented results from laboratory experiments can lay the basis for an industrial application of the developed spray drying process to supply specific microbial cultures with high long-term viability for various economic sectors.
Disclosure statement
No potential conflict of interest was reported by the authors.
Additional information
Funding
References
- Ramos, G. L.; de, P. A.; Vigoder, H. C.; Nascimento, J.; dos, S. Kocuria spp. in Foods: Biotechnological Uses and Risks for Food Safety. Appl. Food Biotechnol. 2021, 8, 79–88. DOI: 10.22037/afb.v8i2.30748.
- Rezaeeyan, Z.; Safarpour, A.; Amoozegar, M. A.; Babavalian, H.; Tebyanian, H.; Shakeri, F. High Carotenoid Production by a Halotolerant Bacterium, Kocuria sp. Strain QWT-12 and Anticancer Activity of Its Carotenoid. Excli J 2017, 16, 1206. DOI: 10.17179/EXCLI2017-218.
- Haq, F.; Butt, M.; Ali, H.; Chaudhary, H. J. Biosorption of Cadmium and Chromium from Water by Endophytic Kocuria rhizophila: Equilibrium and Kinetic Studies. Desalin. Water Treat. 2016, 57, 19946–19958. DOI: 10.1080/19443994.2015.1109561.
- Grüner-Lempart, S.; Eckert, J.; Gredmaier, L. Reinigung Von Lösemittelhaltiger Prozessabluft in Biorieselbettreaktoren. Chem. Ing. Tech. 2021, 93, 1555–1564. DOI: 10.1002/cite.202100058.
- Kovács, G.; Burghardt, J.; Pradella, S.; Schumann, P.; Stackebrandt, E.; Màrialigeti, K. Kocuria palustris sp. nov. and Kocuria rhizophila sp. nov., Isolated from the Rhizoplane of the Narrow-Leaved Cattail (Typha angustifolia). Int. J. Syst. Bacteriol. 1999, 49 Pt 1, 167–173. DOI: 10.1099/00207713-49-1-167.
- Peighambardoust, S. H.; Golshan Tafti, A.; Hesari, J. Application of Spray Drying for Preservation of Lactic Acid Starter Cultures: A Review. Trends in Food Sci. Technol. 2011, 22, 215–224. DOI: 10.1016/j.tifs.2011.01.009.
- Furuta, T.; Neoh, T. L. Microencapsulation of Food Bioactive Components by Spray Drying: A Review. Drying Technol. 2021, 39, 1800–1831. DOI: 10.1080/07373937.2020.1862181.
- Broeckx, G.; Vandenheuvel, D.; Henkens, T.; Kiekens, S.; van den Broek, M. F. L.; Lebeer, S.; Kiekens, F. Enhancing the Viability of Lactobacillus rhamnosus GG after Spray Drying and during Storage. Int J. Pharm 2017, 534, 35–41. DOI: 10.1016/j.ijpharm.2017.09.075.
- Broeckx, G.; Kiekens, S.; Jokicevic, K.; Byl, E.; Henkens, T.; Vandenheuvel, D.; Lebeer, S.; Kiekens, F. Effects of Initial Cell Concentration, Growth Phase, and Process Parameters on the Viability of Lactobacillus rhamnosus GG after Spray Drying. Drying Technol. 2020, 38, 1474–1492. DOI: 10.1080/07373937.2019.1648290.
- Pupa, P.; Apiwatsiri, P.; Sirichokchatchawan, W.; Pirarat, N.; Muangsin, N.; Shah, A. A.; Prapasarakul, N. The Efficacy of Three Double-Microencapsulation Methods for Preservation of Probiotic Bacteria. Sci. Rep. 2021, 11, 13753. DOI: 10.1038/s41598-021-93263-z.
- Chandralekha, A.; Tavanandi, A. H.; Amrutha, N.; Hebbar, H. U.; Raghavarao, K. S. M. S.; Gadre, R. Encapsulation of Yeast (Saccharomyces cerevisiae) by Spray Drying for Extension of Shelf Life. Drying Technol. 2016, 34, 1307–1318. DOI: 10.1080/07373937.2015.1112808.
- Chandralekha, A.; Rani, A.; Tavanandi, H. A.; Amrutha, N.; Hebbar, U.; Raghavarao, K. S. M. S. Role of Carrier Material in Encapsulation of Yeast (Saccharomyces cerevisiae) by Spray Drying. Drying Technol. 2017, 35, 1029–1042. DOI: 10.1080/07373937.2016.1230626.
- González-Esparza, A.; Gentina, J. C.; Ah-Hen, K. S.; Alvarado, R.; Stevenson, J.; Briceño, E.; Montenegro, O. Survival of Spray-Dried Rhodotorula mucilaginosa Isolated from Natural Microbiota of Murta Berries and Antagonistic on Botrytis cinerea. Food Technol. Biotechnol. 2019, 57, 222–229. DOI: 10.17113/ftb.57.02.19.6139.
- Cañamás, T. P.; Viñas, I.; Usall, J.; Magan, N.; Solsona, C.; Teixidó, N. Impact of Mild Heat Treatments on Induction of Thermotolerance in the Biocontrol Yeast Candida sake CPA‐1 and Viability after Spray‐Drying. J. Appl. Microbiol. 2008, 104, 767–775. DOI: 10.1111/j.1365-2672.2007.03590.x.
- Santivarangkna, C.; Wenning, M.; Foerst, P.; Kulozik, U. Damage of Cell Envelope of Lactobacillus helveticus during Vacuum Drying. J. Appl. Microbiol. 2007, 102, 748–756. DOI: 10.1111/j.1365-2672.2006.03123.x.
- Santivarangkna, C.; Kulozik, U.; Foerst, P. Inactivation Mechanisms of Lactic Acid Starter Cultures Preserved by Drying Processes. J. Appl. Microbiol. 2008, 105, 1–13. DOI: 10.1111/j.1365-2672.2008.03744.x.
- García, A. H. Anhydrobiosis in Bacteria: From Physiology to Applications. J. Biosci. 2011, 36, 939–950. DOI: 10.1007/s12038-011-9107-0.
- Morgan, C. A.; Herman, N.; White, P. A.; Vesey, G. Preservation of Micro-Organisms by Drying; a Review. J. Microbiol Methods 2006, 66, 183–193. DOI: 10.1016/j.mimet.2006.02.017.
- Li, C.; Wang, Y.; Li, Q.; Xu, N. Yogurt Starter Obtained from Lactobacillus plantarum by Spray Drying. Drying Technol. 2012, 30, 1698–1706. DOI: 10.1080/07373937.2012.714824.
- Oluwaseun Sunny‐Roberts, E.; Knorr, D. Cellular Injuries on Spray‐Dried Lactobacillus rhamnosus GG and Its Stability during Food Storage. Nutr. Food Sci. 2011, 41, 191–200. DOI: 10.1108/00346651111132466.
- Pugliese, A.; Cabassi, G.; Chiavaro, E.; Paciulli, M.; Carini, E.; Mucchetti, G. Physical Characterization of Whole and Skim Dried Milk Powders. J. Food Sci. Technol. 2017, 54, 3433–3442. DOI: 10.1007/s13197-017-2795-1.
- Timkina, E.; Drábová, L.; Palyzová, A.; Řezanka, T.; Maťátková, O.; Kolouchová, I. Kocuria Strains from Unique Radon Spring Water from Jachymov Spa. Fermentation 2022, 8, 35. DOI: 10.3390/fermentation8010035.
- Reddy, K. B. P. K.; Madhu, A. N.; Prapulla, S. G. Comparative Survival and Evaluation of Functional Probiotic Properties of Spray-Dried Lactic Acid Bacteria. Int. J. of Dairy Tech. 2009, 62, 240–248. DOI: 10.1111/j.1471-0307.2009.00480.x.
- Shokri, Z.; Fazeli, M. R.; Ardjmand, M.; Mousavi, S. M.; Gilani, K. Factors Affecting Viability of Bifidobacterium bifidum during Spray Drying. DARU J. Pharm. Sci. 2015, 23, 7. DOI: 10.1186/s40199-014-0088-z.
- Slavutsky, A. M.; Chávez, M. C.; Favaro-Trindade, C. S.; Bertuzzi, M. A. Encapsulation of Lactobacillus acidophilus in a Pilot-Plant Spray-Dryer. Effect of Process Parameters on Cell Viability. J. Food Process Engin. 2017, 40, e12394. DOI: 10.1111/jfpe.12394.
- Elhariry, H.; Gado, E.; El-Deeb, B.; Altalhi, A. Antibiofilm Potential of Biogenic Silver Nanoparticles against Kocuria rosea and Kocuria rhizophila. Microbiology 2018, 87, 9–20. DOI: 10.1134/S0026261718010071.
- Guesmi, S.; Pujic, P.; Nouioui, I.; Dubost, A.; Najjari, A.; Ghedira, K.; Igual, J. M.; Miotello, G.; Cherif, A.; Armengaud, J.; et al. Ionizing-Radiation-Resistant Kocuria rhizophila PT10 Isolated from the Tunisian Sahara Xerophyte Panicum turgidum: Polyphasic Characterization and Proteogenomic Arsenal. Genomics 2021, 113, 317–330. DOI: 10.1016/j.ygeno.2020.11.029.
- García-López, M.-L.; Santos, J.-Á.; Otero, A. Micrococcus. In Encyclopedia of Food Microbiology; Robinson, R. K., Ed.; Elsevier: Oxford, 1999; pp 1344–1350. DOI: 10.1006/rwfm.1999.1025.
- Wang, N.; Fu, N.; Chen, X. D. The Extent and Mechanism of the Effect of Protectant Material in the Production of Active Lactic Acid Bacteria Powder Using Spray Drying: A Review. Curr. Opin. Food Sci. 2022, 44, 100807. DOI: 10.1016/j.cofs.2022.01.003.
- Soukoulis, C.; Behboudi-Jobbehdar, S.; Yonekura, L.; Parmenter, C.; Fisk, I. Impact of Milk Protein Type on the Viability and Storage Stability of Microencapsulated Lactobacillus acidophilus NCIMB 701748 Using Spray Drying. Food Bioprocess Technol 2014, 7, 1255–1268. DOI: 10.1007/s11947-013-1120-x.
- Tang, H. W.; Abbasiliasi, S.; Murugan, P.; Tam, Y. J.; Ng, H. S.; Tan, J. S. Influence of Freeze-Drying and Spray-Drying Preservation Methods on Survivability Rate of Different Types of Protectants Encapsulated Lactobacillus acidophilus FTDC 3081. Biosci. Biotechnol. Biochem. 2020, 84, 1913–1920. DOI: 10.1080/09168451.2020.1770572.
- Würth, R.; Foerst, P.; Kulozik, U. Effects of Skim Milk Concentrate Dry Matter and Spray Drying Air Temperature on Formation of Capsules with Varying Particle Size and the Survival Microbial Cultures in a Microcapsule Matrix. Drying Technol. 2018, 36, 93–99. DOI: 10.1080/07373937.2017.1301952.
- Jokicevic, K.; Lebeer, S.; Kiekens, F. Atomization Gas Type, Device Configuration and Storage Conditions Strongly Influence Survival of Lactobacillus casei after Spray Drying. Drying Technol. 2022, 40, 494–504. DOI: 10.1080/07373937.2020.1809447.
- Bustos, P.; Bórquez, R. Influence of Osmotic Stress and Encapsulating Materials on the Stability of Autochthonous Lactobacillus plantarum after Spray Drying. Drying Technol. 2013, 31, 57–66. DOI: 10.1080/07373937.2012.717325.
- Zhu, Z.; Luan, C.; Zhang, H.; Zhang, L.; Hao, Y. Effects of Spray Drying on Lactobacillus plantarum BM-1 Viability, Resistance to Simulated Gastrointestinal Digestion, and Storage Stability. Drying Technol. 2016, 34, 177–184. DOI: 10.1080/07373937.2015.1021009.
- Sunny-Roberts, E. O.; Knorr, D. The Protective Effect of Monosodium Glutamate on Survival of Lactobacillus rhamnosus GG and Lactobacillus rhamnosus E-97800 (E800) Strains during Spray-Drying and Storage in Trehalose-Containing Powders. Int. Dairy J. 2009, 19, 209–214. DOI: 10.1016/j.idairyj.2008.10.008.
- Liao, L.-K.; Wei, X.-Y.; Gong, X.; Li, J.-H.; Huang, T.; Xiong, T. Microencapsulation of Lactobacillus casei LK-1 by Spray Drying Related to Its Stability and in Vitro Digestion. LWT - Food Sci. Technol. 2017, 82, 82–89. DOI: 10.1016/j.lwt.2017.03.065.
- Minorova, A.; Romanchuk, I.; Verbytskyi, S.; Danylenko, S.; Krushelnytska, N.; Potemska, O.; Narizhnyi, S. Effect of Protein and Carbohydrate Components upon Quality Parameters and Viable Probiotic Bacteria Content in Milk Mixtures during Their Drying and Storage. J. Microb. Biotech Food Sci. 2022, 11, e3778. DOI: 10.55251/jmbfs.3778.
- Çabuk, B.; Harsa, Ş. Whey Protein-Pullulan (WP/Pullulan) Polymer Blend for Preservation of Viability of Lactobacillus acidophilus. Drying Technol. 2015, 33, 1223–1233. DOI: 10.1080/07373937.2015.1021008.
- Eratte, D.; Gengenbach, T. R.; Dowling, K.; Barrow, C. J.; Adhikari, B. Survival, Oxidative Stability, and Surface Characteristics of Spray Dried Co-Microcapsules Containing Omega-3 Fatty Acids and Probiotic Bacteria. Drying Technol. 2016, 34, 1926–1935. DOI: 10.1080/07373937.2016.1141782.
- Lapsiri, W.; Bhandari, B.; Wanchaitanawong, P. Viability of Lactobacillus plantarum TISTR 2075 in Different Protectants during Spray Drying and Storage. Drying Technol. 2012, 30, 1407–1412. DOI: 10.1080/07373937.2012.684226.
- Romano, P.; Pietrafesa, R.; Romaniello, R.; Zambuto, M.; Calabretti, A.; Capece, A. Impact of Yeast Starter Formulations on the Production of Volatile Compounds during Wine Fermentation. Yeast 2015, 32, 245–256. DOI: 10.1002/yea.3034.
- Zamora, L. M.; Carretero, C.; Parés, D. Comparative Survival Rates of Lactic Acid Bacteria Isolated from Blood, following Spray-Drying and Freeze-Drying. Food Sci. Technol. Int. 2006, 12, 77–84. DOI: 10.1177/1082013206062443.
- Liu, C.-P.; Liu, S.-D. Low-Temperature Spray Drying for the Microencapsulation of the Fungus Beauveria bassiana. Drying Technol. 2009, 27, 747–753. DOI: 10.1080/07373930902828005.
- Hugo, A. A.; Bruno, F.; Golowczyc, M. A. Whey Permeate Containing Galacto-Oligosaccharides as a Medium for Biomass Production and Spray Drying of Lactobacillus plantarum CIDCA 83114. LWT - Food Sci. Technol. 2016, 69, 185–190. DOI: 10.1016/j.lwt.2016.01.031.
- Ananta, E.; Volkert, M.; Knorr, D. Cellular Injuries and Storage Stability of Spray-Dried Lactobacillus rhamnosus GG. Int. Dairy J. 2005, 15, 399–409. DOI: 10.1016/j.idairyj.2004.08.004.
- Behboudi-Jobbehdar, S.; Soukoulis, C.; Yonekura, L.; Fisk, I. Optimization of Spray-Drying Process Conditions for the Production of Maximally Viable Microencapsulated L. acidophilus NCIMB 701748. Drying Technol. 2013, 31, 1274–1283. DOI: 10.1080/07373937.2013.788509.
- Fang, Y.; Rogers, S.; Selomulya, C.; Chen, X. D. Functionality of Milk Protein Concentrate: Effect of Spray Drying Temperature. Biochem. Eng. J. 2012, 62, 101–105. DOI: 10.1016/j.bej.2011.05.007.