Abstract
Over 30–35% of patients down with AML are caused by mutations of FLT3‐ITD and FLT3‐TKD which keeps the protein activated while it activates other signaling proteins downstream that are involved in cell proliferation, differentiation, and survival. As drug targets, many inhibitors are already in clinical practice. Unfortunately, the average overall survival rate for patients on medication suffering from AML is 5 years despite the huge efforts in this field. To perform docking simulation and ADMET studies on selected phytochemicals against FLT3 protein receptor for drug discovery against FLT3 induced AML, molecular docking simulation was performed using human FLT3 protein target (PDB ID: 6JQR) and 313 phytochemicals with standard anticancer drugs (Sorafenib and Gilteritinib in addition to other anticancer drugs). The crystal structure of the protein was downloaded from the protein data bank and prepared using Biovia Discovery Studio. The chemical structures of the phytochemicals were downloaded from the NCBI PubChem database and prepared using Open Babel and VConf softwares. Molecular docking was performed using PyRx on Autodock Vina. The ADMET properties of the best performing compounds were calculated using SwissADME and pkCMS web servers. The results obtained showed that glabridin, ellipticine and derivatives (elliptinium and 9-methoxyellipticine), mezerein, ursolic acid, formononetin, cycloartocarpesin, hypericin, silymarin, and indirubin are the best performing compounds better than sorafenib and gilteritinib based on their binding affinities. The top-performing compounds which had better binding and ADMET properties than sorafenib and gilteritinib could serve as scaffolds or leads for new drug discovery against FLT3 induced AML.
Communicated by Ramaswamy H. Sarma
1. Introduction
The processes involved in blood cell formation in the hematopoietic stem cell (HSC) are called hematopoiesis. Specifically, blood cell formation takes place in the extravascular cavities between the sinuses of the marrow and occurs during embryonic development and throughout adulthood to produce and replenish the blood system (Jagannathan-Bogdan & Zon, Citation2013). Different types of blood cells are produced by the HSC. In different lineages, blood cells could emerge from myeloid or lymphoid lineage. Examples of blood cells from myeloid lineage are macrophages, monocytes, basophils, eosinophils, neutrophils, erythrocytes, megakaryocytes, and platelets, whereas examples of blood cells from lymphoid lineage are T cells, B cells, natural killer cells, and innate lymphoid cells. For smooth functioning of the body, the body must meet certain daily blood cell production requirements. On average, at least 500 billion new blood cells have to be produced daily (Birbrair & Frenette, Citation2016; Nombela-Arrieta & Manz, Citation2017). The hematopoietic stem and progenitor cells (HSPCs) and its self-renewing ability together with its multi-lineage differentiation (multi-potent ability) potentials have to be properly controlled or regulated (Basilico & Göttgens, Citation2017). Dysregulation of some genes in HSPCs can lead to different types of hematological malignancies. Notably, some forms of leukemogenic mutations can disrupt regulatory networks which blocks cell differentiation and then promotes cell proliferation and survival (Basilico & Göttgens, Citation2017). The continuous activation of signaling pathways that promotes cell proliferation while blocking differentiation and apoptosis is a hallmark of cancer. In leukemogenesis, mutation in certain genes for example the Fms-like tyrosine kinase 3 (FLT3) receptor genes in chromosome 13q12 favor cell proliferation which has been identified in 30–35% of patients suffering from acute myeloid leukemia (AML) (Gilliland & Griffin, Citation2002). FLT3 (containing 993 amino acids with a molecular weight of 158–160 kDa) plays a vital role in hematopoiesis (American Physiological Society, 2019) where it is found expressed in early hematopoietic progenitor cells and myeloid leukemia blasts (Kawase et al., Citation2019; Turner et al., Citation1996). Mutation in FLT3 genes is arguably the most frequently mutated genes in AML (Gilliland & Griffin, Citation2002).
FLT3 is a cytokine protein receptor in humans, a member of the class III receptor tyrosine kinases (RTKs) that also includes c-KIT, colony-stimulating factor 1 receptor (formerly known as FMS), and platelet-derived growth factor receptors α and β (PDGFRA and PDGFRB) (Elyamany et al., Citation2014). Within the extracellular domain of class III RTKs are 5 immunoglobulin G-like motifs with 3 outer ligand-binding domains and 2 tyrosine kinase domains (TKDs) which are situated in the C-terminal, intracytoplasmic side of the protein (Fernandes et al., Citation2020). In an unbound state, the domain is usually in an auto-inhibited state (Berenstein, Citation2015; Sutamtewagul & Vigil, Citation2018). However, in a bound state (ligand-binding), there is oligomerization (homodimerization in FLT3) with subsequent transphosphorylation of tyrosine residues within the TKDs, leading to the activation of cascades of proteins such as PI3K, AKT, mTOR, MAPK, RAS, and STAT5 which are key proteins in different signaling pathways involved in cell proliferation, anti-apoptosis, differentiation, and survival (Berenstein, Citation2015; Grafone et al., Citation2012; Sutamtewagul & Vigil, Citation2018). Mutation in the FLT3 receptor often results in constitutive activation even in the absence of FLT3 ligand (FL). There are basically two types of FLT3 mutations. They are the internal tandem duplication (ITD), found in approximately 25% of AML cases and point mutations in the TKDs found in 7–10% of AML cases (Kottaridis et al., Citation2003; Levis, Citation2013; Papaemmanuil et al., Citation2016; Sutamtewagul & Vigil, Citation2018).
Besides FLT3 gene mutation as a possible cause of AML, mutation in other genes can as well result in AML. For instance, a mutation in AKT1 protein can as well lead to the uncontrolled activation of other proteins in key signaling pathways similar to what FLT3-ITD does. For this reason, the root cause of AML is heterogeneous and best defined by its cytogenetic or mutational characteristics (Arber et al., Citation2016). The heterogeneous nature of AML makes it difficult to treat and without sensitive diagnostic methodologies, medical doctors will be unable to help even with the most sophisticated treatment options. Again, genetic deletions, amplifications, point mutations, and rearrangements are issues in AML which has caused an increased risk of relapse, and poor overall survival (Daver et al., Citation2019; Döhner et al., Citation2015; Nakao et al., Citation1996; Small, Citation2006). This makes it necessary at different points across a patient's disease course for tests to be carried out (Daver et al., Citation2019). As at 2015, AML affected 1 million people and caused 147,000 deaths (Vos et al., 2016; Wang et al., 2016). Despite the huge progress in the discovery of new drugs, 5 years survival rate for patients with AML under treatment is known (Cancer.Net, Citation2020). Without treatment, the survival rate is counted in weeks or months (Meillon-Garcia & Demichelis-Gómez, Citation2020). The present 5 years average overall survival (OS) for people 20 years and older is 25% while people younger than 20 years is 67% (Cancer.Net, Citation2020). Although the present 5 years OS may not give the exact statistics because it is calculated every 5 years (Cancer.Net, Citation2020), it is useful in determining the progress made in the past years.
As potential druggable targets (FLT3‐ITD and FLT3‐TKD), many FLT3 inhibitors have been developed. The activities of these inhibitors can be classified based on their binding affinity (Kiyoi et al., Citation2020). According to Kiyoi et al. (Citation2020), FLT3 has an active and inactive conformation based on the arrangements of 3 amino acid residues (Asp‐Phe‐Gly) in the activation loop, AL. The inhibitors can be grouped as either type I or II. Type I inhibitors (e.g. Midostaurin, gilteritinib, and crenolanib) can bind to both the active and inactive forms of FLT3 specifically at the ATP-binding site, whereas the type II inhibitors (e.g. Quizartinib and sorafenib) can bind to only inactive conformation specifically at the back pocket of the ATP‐binding region of the inactive conformation, making it to be selective in action (Daver et al., 2015; Kiyoi et al., Citation2020). The ability of type I inhibitors to compete with the ATP-binding site confers on them the potential to inhibit multiple kinases.
To consolidate on the progress made so far in search of drugs capable of increasing the OS of AML patients and to overcome drug resistance of current inhibitors (Kawase et al., Citation2019), the search for novel inhibitors with fewer side effects from natural products is laudable and necessary for life-saving. Virtual high-throughput molecular docking techniques now increase the chances of drug discovery success yet reducing cost and time spent in the laboratory. This study is aimed at identifying natural compounds with better binding affinities to FLT3 yet with fewer side effects when compared to approve drugs though molecular docking studies and ADMET properties evaluation.
2. Materials and methods
2.1. Ligand curation and preparation
Literature searches were carried out to identify phytochemicals previously reported to possess anti-cancer properties. Some of the sources consulted are Phytochemistry: An in silico and in vitro Update edited by Kumar and Egbuna (2018), Phytochemicals as Lead Compounds for New Drug Discovery edited by Egbuna et al. (2019), and Drug Development for Cancer and Diabetes: A Path to 2030 edited by Saravanan et al. (2020). The chemical structures (in 3D SDF) of three hundred and thirteen (313) compounds (), standard FLT3 inhibitors (Sorafenib and Gilteritinib), and other approved anticancer drugs with their respective CIDs () were downloaded from the NCBI PubChem database (pubchem.ncbi.nlm.nih.gov/). The choice of Sorafenib and Gilteritinib as standards are because they have been found to be effective against FLT3 inspired AML (Perl, Citation2019). Compounds existing in only 2D SDF formats were converted to 3D SDF format using VConf software (VeraChem LLC), whereas compounds not found on the PubChem database were drawn using ChemDraw Ultra 12.0 (CambridgeSoft). All the respective ligands generated were compressed into a single SDF file using Open Babel software (openbabel.org) to aid easy insert into PyRx software.
Table 1. Acceptable range of selected ADMET properties.
Table 2. Molecular docking scores of FLT3 inhibitors.
2.2. Protein preparation
The crystal structure of FLT3 in complex with gilteritinib (PDB ID: 6JQR, 2.20 Å resolution) was downloaded in .pdb format from protein data bank (https://www.rcsb.org/) () and processed using BIOVIA Discovery Studio Visualizer 2021 v21.1.0.20298 (Dassault Systèmes: https://www.3ds.com) as described by Qasaymeh et al. (Citation2019). Gilteritinib in complexation with FLT3 served as a model for easy prediction of the active site of FLT3. During processing, water molecules and hetero atoms were deleted while polar hydrogens were added.
2.3. Active site prediction
The active site of FLT3 was predicted through PDB, PrankWeb (P2Rank), Discovery Studio, and from literature. PrankWeb gave an insightful prediction together with its predicted center coordinates (Centre X: −27.89, Y: −2.73, Z: −28.41). PrankWeb (https://prankweb.cz/) is a novel machine learning-based server for online prediction of ligand binding sites of the protein. The predictions from the above-mentioned sources were harmonized. The amino acid residues that appear to be common among them were selected and used for this study. They are VAL624, ALA642, TYR694, ASP829, and PHE830. The selection of the rightly predicted amino acid residue is necessary to ensure that the setting of the grid box in the PyRx software covers the protein binding site of the target. Thereafter, the resolved center coordinate for this study was found to be X: −28.1226, Y: −1.2759, Z: −28.2055 with dimensions (Angstrom) as X: 25.0000, Y: 25.3101, Z: −257970.
2.4. Molecular docking studies
Molecular docking simulations were performed using PyRx software version 0.8 (https://pyrx.sourceforge.io) as described by Dallakyan and Olson (Citation2015). PyRx is a molecular docking simulation software for high-throughput virtual screening of molecules against protein targets. It is useful in predicting compounds with the best potentials to bind firmly to a protein through the analysis of the binding energy of compounds in kcal/mol. In this study, the prepared and compressed ligands in 3D SDF format were imported into PyRx through the inbuilt OpenBabel graphic user interface. The various compounds were subjected to energy minimization via the Universal Force Field (UFF) by conjugate gradient algorithm with a total number of steps set at 200. The number of steps for update was set as 1 and then 0.1 kcal/mol for stop if energy difference is less. After energy minimization, all ligands were converted into AutoDock ligand (pdbqt). For the prepared protein, it was loaded into PyRx and then converted to .pdbqt in preparation for docking. Docking simulation was performed under 8 exhaustiveness. The highest binding energy (most negative) was recognized as the ligand with maximum binding affinity (Prasanth et al., Citation2020). Specific interactions of the best docking poses were visualized using BIOVIA Discovery Studio.
2.5. Theoretical prediction of ADME/T parameters
After the docking simulation was complete, the best-ranked compounds (first 19 compounds + standard inhibitors) in SMILES format were exported to SwissADME and pkCMS web server for toxicity and bioavailability prediction protocols such as those of Lipinski’s rule of 5. SwissADME (http://www.swissadme.ch/) and pkCMS (http://biosig.unimelb.edu.au/pkcsm/prediction) are free web tools for predicting pharmacokinetics, drug-likeness and medicinal chemistry friendliness of small molecules (Daina et al., Citation2017; Pires et al., Citation2015). The significance and standard range of selected ADMET parameters used in this study were presented in .
3. Results and discussion
3.1. Molecular docking simulation
Molecular docking studies to identify new FLT3 inhibitors from natural products were carried out. The result indicates that some natural compounds have better binding energy than the standard drugs (Sorafenib and Gilteritinib) used in this study. The binding energies of the best performing nineteen (19) compounds with standard anticancer drugs were presented in . The plant sources of the compounds were obtained from Wikipedia, FoodDB, and other literary sources. The result shows that glabridin which is present in licorice (root extract), Glycyrrhiza glabra (Fabaceae) has the best binding energy of −10.2 kcal/mol better than Sorafenib and Gilteritinib which have −9 and −8.5 kcal/mol binding energies, respectively. Glabridin is a prenylated isoflavonoid that has been reported to possess anti-inflammatory, anti-atherogenic, estrogenic-like effects, energy metabolism regulation, anticancer, antimicrobial, neuroprotective, anti-osteoporotic, and skin-whitening properties (Simmler et al., Citation2013). Glabridin is reported to form 0.08% to 0.35% of licorice roots’ dry weight (Hayashi et al., Citation2003). Interestingly, our study is the first molecular docking study directly linking glabridin as an inhibitor of FLT3 in AML. This discovery is worthy of being investigated in vitro and in vivo. Google search (as of 02 December 2020, 9 pm Lagos West Africa time) on studies about the anti-AML properties of glabridin returned one relevant result with some unrelated articles. The relevant article reported that glabridin induced apoptosis through JNK1/2 and p38 MAPK signaling pathways through caspases −3, −8, and −9 activation in human promyelocytic leukemia cell lines (Huang et al., Citation2014). This revelation is a justification of the call to further evaluate the potential role that glabridin could play in the treatment of AML. The 3D and 2D structures of glabridin in complex with FLT3 protein (PDB ID: 6JQR) were presented in . The interaction between glabridin at the ATP binding site of 6JQR shows some similarities when compared to the 3D and 2D sorafenib ligand-protein complex (). The interaction between glabridin at the ATP binding site of 6JQR shows some similarities compared to the 3D and 2D sorafenib ligand-protein complex (). The interacting amino acid residue found for the glabridin-protein complex are LEU616, VAL624, ALA642, VAL675, PHE691, LEU818, CYS828, and ASP829 whereas the amino acid residue found for the sorafenib-protein complex are VAL624, ALA642, LYS644, PHE691, ASP698, ASN701, ARG815, LEU818, ASP829, and ASP839. Both protein complexes have at least 5 amino acid residues in common (VAL624, ALA642, PHE691, LEU818, and ASP829). Comparing these amino acid residues to those of the gilteritinib complex before docking (), we confirm the docking protocol of this study as the grid box was properly centered on the protein target site.
Figure 2. The 3D and 2D structures of glabridin, cycloartocarpesin, sorafenib and gilteritinib complexes.
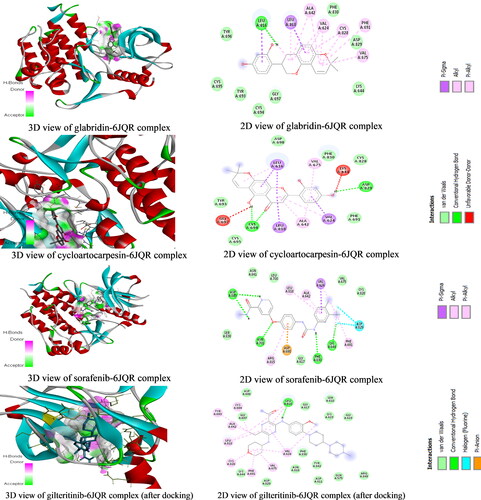
Other compounds such as ellipticine, elliptinium, mezerein, ursolic acid, formononetin, cycloartocarpesin, hypericin, silymarin, indirubin, pomiferin among others also performed better than the two standard FLT3 inhibitors used in this study. The binding energies of these compounds ranged from −10.2 to −9.2 kcal/mol. Compounds with lower binding energies (more negative values) tends to have a better binding affinity to a protein (Choudhary et al., Citation2020). As found in this study, ellipticine and its derivatives elliptinium and 9-methoxyellipticine with similar chemical structures ( and ) all came top out of a pool of over 300 compounds. This attribute can best be interpreted from a structure-activity relationship (SAR) point of view as compounds with similar chemical structures are expected to exhibit similar biological activities. The concept of SAR is useful but not always reliable (Guha, Citation2013), except if additional study is carried out to confirm the claim. Ellipticine is present in plants of the following genera Ochrosia, Rauvolfia, Aspidosperma, and Apocynaceae have been reported to possess anticancer properties (Miller et al., Citation2019; Stiborová et al., Citation2001). Amino acid residue ASN816 in 6JQR was used to form a conventional hydrogen bond with ellipticine. Other compounds with good binding affinities such as ursolic acid, cycloartocarpesin, and naringin that are found in many edible plants () can be useful in making anti-cancer supplements. Of course, they can as well serve as lead or chemical scaffolds for the discovery of potent anticancer drugs. Previous studies have linked ursolic acid, cycloartocarpesin, naringin as having anti-leukemic properties in vitro (Gao et al., Citation2012; Kuete et al., 2015; Li et al., Citation2015; Lin et al., Citation2016). Silymarin and hypericin which are among the top-performing compounds that are present in milk thistle and St John's Wort, respectively, are surprised candidates. Studies supporting their anti-leukemic properties include those by Zhong et al. (Citation2006) and Lee et al. (Citation1999).
Also, we discovered that some standard anticancer drugs meant for different purposes also exhibited good binding affinities to 6JQR which demonstrates that they can target multiple proteins in vivo. For instance, smoothened protein (SMO) standard inhibitors, Sonidegib and Daurismo/Glasdegib with binding energies of −10.2 and −9.4 kcal/mol, respectively, were found to have better binding energies when compared to Sorafenib and Gilteritinib. SMO is a component of the hedgehog signaling pathway. Similarly, Venetoclax, a Bcl-2 standard inhibitor has a better binding energy of −10 kcal/mol. This revelation calls for an overall assessment of drugs in clinical use to ascertain key proteins in different signaling pathways that they can as well inhibit or promote their activities. Successful studies in this area will pave way for better knowledge about the best treatment options for personalized medicine.
From the above discussion, it can be stated that molecular docking simulations have been a vital tool in drug discovery research especially as it concerns deadly diseases (Gokhale et al., Citation2019; Guimarães et al., 2011; Prasad et al., 2020; Zhang et al., Citation2021). However, the accuracy or reliability of results from MD simulation studies remain a subject of controversy which has attracted support and criticisms in the academia and industry. Within the last 10 years, many MD tools have evolved with great improvement and dependence while success stories are recorded for drugs that progress to clinical trials. The assessment of the theoretical output of MD tools in conformational profiling of best-performing ligands is a crucial step in validating results from computational studies, while ensuring the best conformation is selected (Fernandes et al., Citation2020; Willian et al., Citation2016). In this study, after the preparation of ligand and proteins, PyRx software was used for molecular docking under 8 exhaustiveness. The highest binding energy (most negative) was recognized as the ligand with maximum binding affinity of 8 or 9 best poses (or orientations) which are programmatically arranged showing best orientation with factor 0.
3.2. ADMET prediction
In pharmacokinetics, pharmacology, and toxicology, ADMET is an acronym that is used to denote the words absorption, distribution, metabolism, excretion, and toxicity. It provides insight into the physicochemical, druglikeness, and physiochemical properties of a compound. The concept of ADMET hinges on the fact that for a compound to be orally effective, it should be bioavailable, distributed to selective target areas, metabolized accordingly, excreted after it does its job while it does not cause adverse effects to the body cells, tissues and organs it came in contact with. Predicting the ADMET properties of a natural compound from the onset of a drug discovery process is an important step in increasing the overall chances of success during clinical trials (Guan et al., Citation2019; Jia et al., Citation2020). In this study, we utilized SwissADME and pkCMS web servers to predict the ADMET properties of the best performing compounds and standard inhibitors. The result obtained from the ADMET test were presented in and . From the result, we found that some compounds violated Lipinski's rule of 5 (RO5). For instance, hypericin, naringin, baicalin together with the standard drug, gilteritinib all violated more than 1 Lipinski’s RO5 whereas compounds such as glabridin, ellipticine and its derivatives, cycloartocarpesin, silymarin, etc. did not violate the Lipinski’s RO5 (). Besides the physicochemical and druglikeness properties considered in Lipinski's RO5, we looked at physiochemical properties as obtained from the pkCMS web server. Under the absorption parameter (), we found that naringin and baicalin would have poor oral absorption in the intestine. According to Pires et al. (Citation2015), a compound with Caco-2 and intestinal absorbance values of <0.90 and <30%, respectively, will have poor absorption in the intestine (). With the progress made so far, bioavailability issues can be resolved through the application of micro and nano-encapsulation technologies (Aqil et al., Citation2013; Grgić et al., Citation2020; Wang et al., Citation2014) or other techniques. In the distribution section, we found that ellipticine and its derivatives have a high blood-brain barrier (BBB) and central nervous system (CNS) values which suggest that they can get to the nervous system easily. This calls for caution in the adoption of ellipticine and its derivatives as FLT3 inhibitors for the treatment of AML as they may likely cause some undesirable side effects. For metabolism, we found that only elliptinium will likely be metabolized by CYP2D6 while over 80% of the compounds are likely to be metabolized by CYP3A4. The total clearance (TC) value represents the capacity of a drug to be eliminated by different organs such as the hepatic and renal organs. In this study, the TC for each compound was presented. We found that elliptinum had the highest clearance value of 1.02 (log(ml/min/kg)). This is expected because it is the only compound that served as both substrates of CYP2D6 and CYP3A4 (). Again, from the toxicity test (Ames test), we found that ellipticine and its derivatives may be mutagenic. Also, compounds such as ursolic acid and the standard drugs may cause hepatoxicity. However, simple rules of druglikeness and physiochemical property predictions are useful but have some limitations (Neidle, Citation2012; Schneider, Citation2013). For instance, druglikeness does not investigate the actual biological response that a drug will cause, and of course some helpful drugs in clinical practice today violated one rule or the other.
Table 3. Physicochemical and druglikeness properties of FLT3 inhibitors by SwissADME.
Table 4. ADMET properties of FLT3 inhibitors by pkCMS.
4. Conclusion
Acute myeloid leukemia is characterized by excessive proliferation of abnormal cells in the blood and bone marrow which subsequently interfere with normal blood cell production. It is named 'acute' because it progresses very fast and if not treated on time leaves it to get worst. So far, there are many AML drugs designed to target one defect protein or the other. But despite the availability of these drugs, the overall survival rate of patients still stood at 5 years. In this study, molecular docking studies conducted revealed that glabridin, ellipticine and its derivatives (elliptinium and 9-methoxyellipticine), mezerein, ursolic acid, formononetin, cycloartocarpesin, hypericin, silymarin, and indirubin were the best performing compound in the molecular docking studies, as such could serve as drug leads or scaffolds for the treatment of AML caused by FLT3‐ITD and FLT3‐TKD. However, the ADMET properties of some of these compounds such as ellipticine and its derivatives makes them undesirable for further consideration. Because they have a high BBB and CNS values which suggest that they can get to the nervous system easily which calls for caution.
Author contributions
Conceptualization, C.E., K.C.P. and E.N.O.; methodology, C.E., K.C.P. and J.K.; software, C.E. and J.K.; validation, J.K. and B.A.; formal analysis, C.E., K.C.P. and B.A.; resources, J.K. and B.A.; data curation, C.E.; writing—original draft preparation, C.E., J.K. and B.A.; supervision, K.C.P and E.N.O; funding acquisition, J.K. and B.A. All authors have read and agreed to the published version of the manuscript.
Supplementary_Table_2.pdf
Download PDF (143.7 KB)Supplementary_Table_1.pdf
Download PDF (277.9 KB)Acknowledgements
Authors are thankful to World Bank, University of Port Harcourt, Majmaah University, National Universities Commission, and the Association of African Universities for their support. Authors are also thankful to the Deanship of Scientific Research at Majmaah University for supporting this study.
Disclosure statements
Authors declare no conflict of interest.
Additional information
Funding
References
- American Physiological Society. (2019). FMS-like tyrosine kinase 3/FLT3: From basic science to clinical implications. https://journals.physiology.org/doi/full/10.1152/physrev.00029.2018. https://doi.org/10.1152/physrev.00029.2018
- Aqil, F., Munagala, R., Jeyabalan, J., & Vadhanam, M. V. (2013). Bioavailability of phytochemicals and its enhancement by drug delivery systems. Cancer Letters, 334(1), 133–141. https://doi.org/10.1016/j.canlet.2013.02.032
- Arber, D. A., Orazi, A., Hasserjian, R., Thiele, J., Borowitz, M. J., Le Beau, M. M., Bloomfield, C. D., Cazzola, M., & Vardiman, J. W. (2016). The 2016 revision to the World Health Organization classification of myeloid neoplasms and acute leukemia. Blood, 127(20), 2391–2405. https://doi.org/10.1182/blood-2016-03-643544
- Basilico, S., & Göttgens, B. (2017). Dysregulation of haematopoietic stem cell regulatory programs in acute myeloid leukaemia. Journal of Molecular Medicine (Berlin, Germany), 95(7), 719–727. https://doi.org/10.1007/s00109-017-1535-3
- Berenstein, R. (2015). Class III receptor tyrosine kinases in acute leukemia - Biological functions and modern laboratory analysis. Biomarker Insights, 10(Suppl 3), 1–14. https://doi.org/10.4137/BMI.S22433
- Birbrair, A., Frenette, P. S. (2016, 1 March). Niche heterogeneity in the bone marrow. Annals of the New York Academy of Sciences, 1370 (1), 82–96. https://doi.org/10.1111/nyas.13016
- Cancer.Net. (2020). Leukemia - Acute myeloid - AML: Statistics. https://www.cancer.net/cancer-types/leukemia-acute-myeloid-aml/statistics.
- Choudhary, M. I., Shaikh, M., Tul-Wahab, A., & Ur-Rahman, A. (2020). In silico identification of potential inhibitors of key SARS-CoV-2 3CL hydrolase (Mpro) via molecular docking, MMGBSA predictive binding energy calculations, and molecular dynamics simulation. PloS One, 15(7), e0235030. https://doi.org/10.1371/journal.pone.0235030
- Daina, A., Michielin, O., & Zoete, V. (2017). SwissADME: a free web tool to evaluate pharmacokinetics, drug-likeness and medicinal chemistry friendliness of small molecules. Scientific reports, 7(1), 1–13. https://doi.org/10.1038/srep42717
- Daina, A., Michielin, O., & Zoete, V. (2017). SwissADME: A free web tool to evaluate pharmacokinetics, drug-likeness and medicinal chemistry friendliness of small molecules. Scientific Reports, 7, 42717. https://doi.org/10.1038/srep42717 PMID: 28256516; PMCID: PMC5335600.
- Dallakyan, S., & Olson, A. J. (2015). Small-molecule library screening by docking with PyRx. Methods in Molecular Biology (Clifton, N.J.), 1263, 243–250. https://doi.org/10.1007/978-1-4939-2269-7_19 PMID: 25618350.
- Daver, N., Schlenk, R. F., Russell, N. H., & Levis, M. J. (2019). Targeting FLT3 mutations in AML: Review of current knowledge and evidence. Leukemia, 33(2), 299–312. ‐https://doi.org/10.1038/s41375-018-0357-9
- Diseases, N., Grewal, A. S., Bhardwaj, S., Pandita, D., Lather, V., & Singh Sekhon, B. (2015). Updates on aldose reductase inhibitors for management of diabetic complications and non-diabetic diseases. Mini Reviews in Medicinal Chemistry, 16(2), 120–162. https://doi.org/10.2174/1389557515666150909143737
- Döhner, H., Weisdorf, D. J., & Bloomfield, C. D. (2015). Acute myeloid leukemia. The New England Journal of Medicine, 373(12), 1136–1152. https://doi.org/10.1056/NEJMra1406184
- Elyamany, G., Awad, M., Fadalla, K., Albalawi, M., Al Shahrani, M., & Al Abdulaaly, A. (2014). Frequency and prognostic relevance of FLT3 mutations in Saudi acute myeloid leukemia patients. Advances in Hematology, 2014, 141360. https://doi.org/10.1155/2014/141360
- Erukainure, O. L., Mopuri, R., Chukwuma, C. I., Koorbanally, N. A., & Islam, M. S. (2018). Phaseolus lunatus (lima beans) abates Fe2+‐induced hepatic redox imbalance; inhibits intestinal glucose absorption and major carbohydrate catabolic enzymes; and modulates muscle glucose uptake. Journal of Food Biochemistry, 42(6), 1–14. https://doi.org/10.1111/jfbc.12655
- Fernandes, Í. A., Braga Resende, D., Ramalho, T. C., Kuca, K., & da Cunha, E. F. F. (2020). Theoretical studies aimed at finding FLT3 inhibitors and a promising compound and molecular pattern with dual aurora B/FLT3 activity. Molecules, 25(7), 1726. https://doi.org/10.3390/molecules25071726
- Gao, N., Cheng, S., Budhraja, A., Gao, Z., Chen, J., Liu, E. H., Huang, C., Chen, D., Yang, Z., Liu, Q., Li, P., Shi, X., & Zhang, Z. (2012). Ursolic acid induces apoptosis in human leukaemia cells and exhibits anti-leukaemic activity in nude mice through the PKB pathway. British Journal of Pharmacology, 165(6), 1813–1826. https://doi.org/10.1111/j.1476-5381.2011.01684.x
- Ghose, A. K., Viswanadhan, V. N., & Wendoloski, J. J. (January, 1999). A knowledge-based approach in designing combinatorial or medicinal chemistry libraries for drug discovery. 1. A qualitative and quantitative characterization of known drug databases. Journal of Combinatorial Chemistry, 1 (1), 55–68. https://doi.org/10.1021/cc9800071
- Gilliland, D. G., & Griffin, J. D. (2002). Role of FLT3 in leukemia. Current Opinion in Hematology, 9(4), 274–281. https://doi.org/10.1097/00062752-200207000-00003 PMID: 12042700.
- Gokhale, P., Chauhan, A., Arora, A., Khandekar, N., Nayarisseri, A., & Singh, S. K. (2019). FLT3 inhibitor design using molecular docking based virtual screening for acute myeloid leukemia. Bioinformation, 15(2), 104–115. https://doi.org/10.6026/97320630015104
- Grafone, T., Palmisano, M., Nicci, C., & Storti, S. (2012). An overview on the role of FLT3-tyrosine kinase receptor in acute myeloid leukemia: Biology and treatment. Oncology Reviews, 6(1), e8. https://doi.org/10.4081/oncol.2012.e8 PMID: 25992210; PMCID: PMC4419636.
- Grgić, J., Šelo, G., Planinić, M., Tišma, M., & Bucić-Kojić, A. (2020). Role of the encapsulation in bioavailability of phenolic compounds. Antioxidants (Basel), 9(10), 923. https://doi.org/10.3390/antiox9100923 PMID: 32993196; PMCID: PMC7601682.
- Guan, L., Yang, H., Cai, Y., Sun, L., Di, P., Li, W., Liu, G., & Tang, Y. (2019). ADMET-score - a comprehensive scoring function for evaluation of chemical drug-likeness. MedChemComm, 10(1), 148–157. https://doi.org/10.1039/c8md00472b
- Guha, R. (2013). On exploring structure-activity relationships. Methods in Molecular Biology (Clifton, N.J.), 993, 81–94. https://doi.org/10.1007/978-1-62703-342-8_6.
- Guimarães, A. P., Oliveira, A. A., da Cunha, E. F. F., Ramalho, T. C., & França, T. C. C. (2011). Design of new chemotherapeutics against the deadly anthrax disease. Docking and molecular dynamics studies of inhibitors containing pyrrolidine and riboamidrazone rings on nucleoside hydrolase from Bacillus anthracis. Journal of Biomolecular Structure & Dynamics, 28(4), 455–469. https://doi.org/10.1080/07391102.2011.10508588
- Hanh, H., Tran, T., Nguyen, M. C., Le, H. T., Nguyen, T. L., & Pham, T. B. (2013). Inhibitors of a -glucosidase and a -amylase from Cyperus rotundus. Pharmaceutical Biology, 52(1), 74–77. https://doi.org/10.3109/13880209.2013.814692
- Hayashi, H., Hattori, S., Inoue, K., Khodzhimatov, O., Ashurmetov, O., Ito, M., & Honda, G. (2003). Field survey of Glycyrrhiza plants in Central Asia (3). Chemical characterization of G. glabra collected in Uzbekistan. Chemical & Pharmaceutical Bulletin, 51(11), 1338–1340. https://doi.org/10.1248/cpb.51.1338
- Huang, H.-L., Hsieh, M.-J., Chien, M.-H., Chen, H.-Y., Yang, S.-F., & Hsiao, P.-C. (2014). Glabridin mediate caspases activation and induces apoptosis through JNK1/2 and p38 MAPK pathway in human promyelocytic leukemia cells. PLoS One, 9(6), e98943. https://doi.org/10.1371/journal.pone.0098943
- Jagannathan-Bogdan, M., & Zon, L. I. (2013). Hematopoiesis. Development (Cambridge, England), 140(12), 2463–2467. https://doi.org/10.1242/dev.083147
- Jia, C.-Y., Li, J.-Y., Hao, G.-F., & Yang, G.-F. (2020). A drug-likeness toolbox facilitates ADMET study in drug discovery. Drug Discovery Today, 25(1), 248–258. https://doi.org/10.1016/j.drudis.2019.10.014
- Kawase, T., Nakazawa, T., Eguchi, T., Tsuzuki, H., Ueno, Y., Amano, Y., Suzuki, T., Mori, M., & Yoshida, T. (2019). Effect of Fms-like tyrosine kinase 3 (FLT3) ligand (FL) on antitumor activity of gilteritinib, a FLT3 inhibitor, in mice xenografted with FL-overexpressing cells. Oncotarget, 10(58), 6111–6123. https://doi.org/10.18632/oncotarget.27222
- Kiyoi, H., Kawashima, N., & Ishikawa, Y. (2020). FLT3 mutations in acute myeloid leukemia: Therapeutic paradigm beyond inhibitor development. Cancer Science, 111(2), 312–322. https://doi.org/10.1111/cas.14274
- Kottaridis, P. D., Gale, R. E., & Linch, D. C. (2003). Flt3 mutations and leukaemia. British Journal of Haematology, 122(4), 523–538. https://doi.org/10.1046/j.1365-2141.2003.04500.x
- Kuete, V., Mbaveng, A. T., Zeino, M., Fozing, C. D., Ngameni, B., Kapche, G. D., Ngadjui, B. T., & Efferth, T. (2015). Cytotoxicity of three naturally occurring flavonoid derived compounds (artocarpesin, cycloartocarpesin and isobavachalcone) towards multi-factorial drug-resistant cancer cells. Phytomedicine: International Journal of Phytotherapy and Phytopharmacology, 22(12), 1096–1102. https://doi.org/10.1016/j.phymed.2015.07.006 PMID: 26547532.
- Lee, K. T., Kim, J. I., Rho, Y. S., Chang, S. G., Jung, J. C., Park, J. H., Park, H. J., & Miyamoto, K. (1999). Hypericin induces both differentiation and apoptosis in human promyelocytic leukemia HL-60 cells. Biological & Pharmaceutical Bulletin, 22(12), 1271–1274. Dechttps://doi.org/10.1248/bpb.22.1271 PMID: 10746153.
- Levis, M. (2013). FLT3 mutations in acute myeloid leukemia: What is the best approach in 2013? Hematology. American Society of Hematology. Education Program, 2013, 220–226.
- Li, R. F., Feng, Y. Q., Chen, J. H., Ge, L. T., Xiao, S. Y., & Zuo, X. L. (2015). Naringenin suppresses K562 human leukemia cell proliferation and ameliorates adriamycin-induced oxidative damage in polymorphonuclear leukocytes. Experimental and Therapeutic Medicine, 9(3), 697–706. https://doi.org/10.3892/etm.2015.2185
- Lin, Z., Jiang, J., & Liu, X. S. (2016). Ursolic acid-mediated apoptosis of K562 cells involves Stat5/Akt pathway inhibition through the induction of Gfi-1. Scientific Reports, 6, 33358. https://doi.org/10.1038/srep33358
- Lipinski, C. A., Lombardo, F., Dominy, B. W., & Feeney, P. J. (2001, March). Experimental and computational approaches to estimate solubility and permeability in drug discovery and development settings. Advanced Drug Delivery Reviews, 46 (1-3), 3–26. https://doi.org/10.1016/S0169-409X(00)00129-0
- Masood, A.M., Ali, H.H., Naeem, M., Latif, M., Bukhari, A.H. and Tanveer, A. (2015). Cyperus rotundus L.: Traditional uses, phytochemistry, and pharmacological activities. Journal of ethnopharmacology, 174, 540–560. https://doi.org/10.1016/j.jep.2015.08.012
- Meillon-Garcia, L. A., & Demichelis-Gómez, R. (2020). Access to therapy for acute myeloid leukemia in the developing world: Barriers and solutions. Current Oncology Reports, 22(12), 125. https://doi.org/10.1007/s11912-020-00987-8
- Miller, C. M., O'Sullivan, E. C., & McCarthy, F. O. (2019). Novel 11-substituted ellipticines as potent anticancer agents with divergent activity against cancer cells. Pharmaceuticals (Basel), 12(2), 90. https://doi.org/10.3390/ph12020090 PMID: 31207878; PMCID: PMC6631919.
- Nakao, M., Yokota, S., Iwai, T., Kaneko, H., Horiike, S., Kashima, K., Sonoda, Y., Fujimoto, T., & Misawa, S. (1996). Internal tandem duplication of the flt3 gene found in acute myeloid leukemia. Leukemia, 10(12), 1911–1918.
- Neidle, S. (2012). Design principles for quadruplex-binding small molecules. In Therapeutic applications of quadruplex nucleic acids (pp. 151–174). US: Academic Press:. https://doi.org/10.1016/b978-0-12-375138-6.00009-1
- Nombela-Arrieta, C. G., & Manz, M. (2017). Quantification and three-dimensional microanatomical organization of the bone marrow. Blood Adv, 1 (6), 407–416. https://doi.org/10.1182/bloodadvances.2016003194
- Papaemmanuil, E., Gerstung, M., Bullinger, L., Gaidzik, V. I., Paschka, P., Roberts, N. D., Potter, N. E., Heuser, M., Thol, F., Bolli, N., Gundem, G., Van Loo, P., Martincorena, I., Ganly, P., Mudie, L., McLaren, S., O'Meara, S., Raine, K., Jones, D. R., … Campbell, P. J. (2016). Genomic classification and prognosis in acute myeloid leukemia. The New England Journal of Medicine, 374(23), 2209–2221. https://doi.org/10.1056/NEJMoa1516192
- Perl, A. E. (2019). Availability of FLT3 inhibitors: How do we use them? Blood, 134 (9), 741–745. https://doi.org/10.1182/blood.2019876821
- Pires, D. E., Blundell, T. L., & Ascher, D. B. (2015, May 14). pkCSM: Predicting small-molecule pharmacokinetic and toxicity properties using graph-based signatures. Journal of Medicinal Chemistry, 58(9), 4066–4072. https://doi.org/10.1021/acs.jmedchem.5b00104 Epub 2015 Apr 22. PMID: 25860834; PMCID: PMC4434528.
- Prasad, S. K., Pradeep, S., Shimavallu, C., Kollur, S. P., Syed, A., Marraiki, N., Egbuna, C., Gaman, M.-A., Kosakowska, O., Cho, W. C., Patrick-Iwuanyanwu, K. C., Ortega, C. J., Frau, J., Flores-Holguín, N., & Glossman-Mitnik, D. (2020). Evaluation of Annona muricata acetogenins as potential anti-SARS-CoV-2 agents through computational approaches. Frontiers in Chemistry, 8, 624716. https://doi.org/10.3389/fchem.2020.624716
- Prasanth, D., Murahari, M., Chandramohan, V., Panda, S. P., Atmakuri, L. R., & Guntupalli, C. (2020). In silico identification of potential inhibitors from Cinnamon against main protease and spike glycoprotein of SARS CoV-2. Journal of Biomolecular Structure & Dynamics, 39(13), 4618-4632. . Advance online publication. https://doi.org/10.1080/07391102.2020.1779129
- Qasaymeh, R. M., Rotondo, D., Oosthuizen, C. B., Lall, N., & Seidel, V. (2019). Predictive binding affinity of plant-derived natural products towards the protein kinase G enzyme of Mycobacterium tuberculosis (MtPknG). Plants (Basel, Switzerland), 8(11), 477. https://doi.org/10.3390/plants8110477
- Schneider, G. (2013). Prediction of drug-like properties. In: Madame Curie Bioscience Database [Internet]. Landes Bioscience. 2000–2013. https://www.ncbi.nlm.nih.gov/books/NBK6404/.
- Simmler, C., Pauli, G. F., & Chen, S. N. (2013). Phytochemistry and biological properties of glabridin. Fitoterapia, 90, 160–184. https://doi.org/10.1016/j.fitote.2013.07.003
- Small, D. (2006). FLT3 mutations: Biology and treatment. Hematology. American Society of Hematology. Education Program, 2006(1), 178–184. https://doi.org/10.1182/asheducation-2006.1.178
- Stiborová, M., Bieler, C. A., Wiessler, M., & Frei, E. (2001). The anticancer agent ellipticine on activation by cytochrome P450 forms covalent DNA adducts⋆1. Biochemical Pharmacology, 62(12), 1675–1684. https://doi.org/10.1016/S0006-2952(01)00806-1
- Sutamtewagul, G., & Vigil, C. E. (2018). Clinical use of FLT3 inhibitors in acute myeloid leukemia. OncoTargets and Therapy, 11, 7041–7052. https://doi.org/10.2147/OTT.S171640
- Turner, A. M., Lin, N. L., Issarachai, S., Lyman, S. D., & Broudy, V. C. (1996). FLT3 receptor expression on the surface of normal and malignant human hematopoietic cells. Blood, 88(9), 3383–3390. Nov 1PMID: 8896403.
- Vos, T., Allen, C., Arora, M., Barber, R. M., Bhutta, Z. A., Brown, A., Carter, A., Casey, D. C., Charlson, F. J., Chen, A. Z., Coggeshall, M., Cornaby, L., Dandona, L., Dicker, D. J., Dilegge, T., Erskine, H. E., Ferrari, A. J., Fitzmaurice, C., Fleming, T., … Murray, C. J. L. (2016, October). Global, regional, and national incidence, prevalence, and years lived with disability for 310 diseases and injuries, 1990-2015: A systematic analysis for the Global Burden of Disease Study 2015. The Lancet, 388 (10053), 1545–1602. https://doi.org/10.1016/S0140-6736(16)31678-6
- Wang, B., Yang, L. P., Zhang, X. Z., Huang, S. Q., Bartlam, M., & Zhou, S. F. (2009). New insights into the structural characteristics and functional relevance of the human cytochrome P450 2D6 enzyme. Drug Metabolism Reviews, 41 (4), 573–643. https://doi.org/10.1080/03602530903118729
- Wang, H., Naghavi, M., Allen, C., Barber, R. M., Bhutta, Z. A., Carter, A., Casey, D. C., Charlson, F. J., Chen, A. Z., Coates, M. M., Coggeshall, M., Dandona, L., Dicker, D. J., Erskine, H. E., Ferrari, A. J., Fitzmaurice, C., Foreman, K., Forouzanfar, M. H., Fraser, M. S., … Murray, C. J. L. ((2016, October ). Global, regional, and national life expectancy, all-cause mortality, and cause-specific mortality for 249 causes of death, 1980-2015: A systematic analysis for the Global Burden of Disease Study 2015. The Lancet, 388 (10053), 1459–1544. https://doi.org/10.1016/S0140-6736(16)31012-1
- Wang, S., Su, R., Nie, S., Sun, M., Zhang, J., Wu, D., & Moustaid-Moussa, N. (2014). Application of nanotechnology in improving bioavailability and bioactivity of diet-derived phytochemicals. The Journal of Nutritional Biochemistry, 25(4), 363–376. https://doi.org/10.1016/j.jnutbio.2013.10.002
- Willian, E. A d L., Pereira, A. F., Castro, A. A. d., Cunha, E. F. F d., & Ramalho, T. C. (2016). Flexibility in the molecular design of acetylcholinesterase reactivators: probing representative conformations by chemometric techniques and docking/QM calculations. Letters in Drug Design & Discovery, 13(5), 360–371. https://doi.org/10.2174/1570180812666150918191550
- Zanger, U. M., & Schwab, M. (2013, April). Cytochrome P450 enzymes in drug metabolism: Regulation of gene expression, enzyme activities, and impact of genetic variation. Pharmacology & Therapeutics, 138 (1), 103–141. https://doi.org/10.1016/j.pharmthera.2012.12.007 PMID 23333322.
- Zhang, K., Liu, Z., Yao, Y., Qiu, Y., Li, F., Chen, D., Hamilton, D. J., Li, Z., & Jiang, S. (2021). Structure-based design of a selective class I histone deacetylase (HDAC) near-infrared (NIR) probe for epigenetic regulation detection in Triple-Negative Breast Cancer (TNBC). Journal of Medicinal Chemistry, 64 (7), 4020–4033. https://doi.org/10.1021/acs.jmedchem.0c02161
- Zhong, X., Zhu, Y., Lu, Q., Zhang, J., Ge, Z., & Zheng, S. (2006). Silymarin causes caspases activation and apoptosis in K562 leukemia cells through inactivation of Akt pathway. Toxicology, 227(3), 211–216. https://doi.org/10.1016/j.tox.2006.07.021