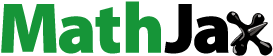
Abstract
Pancreatic adenocarcinoma is a disease with no effective treatment. Chemo-resistance contributes to the dismal prognosis for patients diagnosed with the disease. This study aims to evaluate the toxicity and the effect of Caralluma europaea (C.E) extracts on cancer cell survival, apoptosis, chemo-resistance, and pro-cancer pathways, in pancreatic cancer. The acute and subacute toxicities of C.E extracts were evaluated. The cytotoxic effect on pancreatic cancer cell survival and apoptosis was determined by MTT assay and DNA fragmentation. The expression of cancer stemness markers was measured using Western blot. A molecular docking was used to test the possible effects of C.E compounds in inhibiting the Hedgehog and activating caspase-3. The hydroethanolic extract’s DL50 was over 5000 mg/kg. During the subacute toxicity, only saponins extract showed some hepatic toxicity signs. Cells treated with C.E extracts combined with gemcitabine revealed an additive anti-survival activity. C.E extracts sensitized resistant MIA-PaCa-2 to gemcitabine treatment. Most of the C.E extracts downregulated the expression of cancer stemness-associated genes. Luteolin-7-O-glucoside presented the highest docking Gscore on human Smoothened. Isorhamnetin-3-O-rutinoside induced apoptosis via activation of caspase-3. C.E extracts can be considered safe in inhibiting pancreatic cancer cell survival, inducing apoptosis, and sensitizing cells to chemotherapy via Hedgehog inhibition and caspase-3 activation.
Communicated by Ramaswamy H. Sarma
1. Introduction
Pancreatic adenocarcinoma (PDAC) is a disease with no efficient treatment. The 5-year survival rate for pancreatic cancer patients is estimated at a mere 9%, and complete cures are rarely, if ever, achieved (Rahib et al., Citation2014; Siegel et al., Citation2014, Citation2019).
Plants’ ability to cure diseases, such as cancer, motivated scientific researchers to study their pharmacological activities. In some cases, compounds isolated from plants, such as aristolochic acids, possess serious side effects like kidney failure (Hossain et al., Citation2021; Moni et al., Citation2021). Therefore, toxicological studies are needed to evaluate the safety, and to ensure a suitable anticancer profiling of plants with minimal side effects.
PDAC’s resistance to current treatment regimens (both surgery and chemotherapy) contributes to the dismal prognosis for patients diagnosed with the disease. In addition, PDAC is often diagnosed after metastasis to other organs has already occurred. During the past decade, over 20 phase II/III clinical trials have targeted PDAC, but ‘advances’ involving chemotherapeutic drug combinations have been modest, at best (Philip, Citation2008; Bayraktar et al., Citation2010).
The most effective regimens available require intravenous administration and are associated with significant toxicity (Conroy et al., 2011; Sohal et al., Citation2016). Clearly, there is a critically unmet need for substantial improvement in the therapeutic options for pancreatic cancer.
One of the main clinical challenges of pancreatic cancer treatment is the ability of the tumour to self-renew and develop resistance. Recent research has hypothesized that cancer cells could be the source of these complications, and targeting them will lead to tumor regression. Nanog, CD-133, Sox-2 and Oct-4 have been suggested for labeling cancer stem cells in various tumor types, including pancreatic cancer (Villodre et al., Citation2016; Glumac & LeBeau, Citation2018).
The chemotherapy drug gemcitabine exhibits a limited benefit because of the rapid development of chemoresistance. Hence, it has been speculated that combinatory treatments using gemcitabine and natural extracts could improve pancreatic cancer treatment. Among these natural remedies: Caralluma europaea (C. europaea).
C. europaea (Family: Apocynaceae) is a leafless succulent plant used in folk medicine to treat different diseases including inflammatory diseases, diabetes, gastrointestinal disorders, atherosclerosis, hyperglycemia and cancer. It is one of the most commonly used plant species by the indigenous population of the Fez-Meknes region for cancer treatment (Amrati et al., 2020; Adnan et al., Citation2014; Amrati, Bourhia, Slighoua, Boukhira, et al., Citation2021). Previously published studies have demonstrated the antimicrobial, anti-inflammatory, antidiabetic, hepatoprotective and antioxidant properties of C. europaea (Issiki et al., Citation2017; Dra, Aghraz, et al., Citation2019; Dra, Sellami, et al., Citation2019; Amrati, Bourhia, Slighoua, Boukhira, et al., Citation2021; Amrati et al., 2020). However, very little is known about the activity of C.E on cancer in general and pancreatic cancer, specifically.
In PDAC, targeting signaling pathways mediating chemo-resistance is critical for developing a treatment strategy. Recent research studies show the Hedgehog (Hh) signaling pathway as an early target in cancer treatment (Edderkaoui et al., 2018 ; Kumar et al., Citation2017). Hh signaling pathway regulates cell growth and differentiation of various organs by affecting stem cells. Activation of the Hh pathway is associated with various human tumors such as prostate, lung, and pancreatic cancers. Most Hh pathway antagonists target a seven-transmembrane (7-TM) domain of Smoothened (Smo) (Hao et al., Citation2013; Jiang & Hui, Citation2008).
For many years, anti-cancer drug design has focused on the caspase family. Among the 14 human caspases discovered, Caspases -2, -3, -6, -7, -8, -9 and -10, have been described as apoptotic caspases. Generally, Caspase-3 is considered the main executioner of apoptosis (Aly et al., Citation2020; Slee et al., Citation2001; Walsh et al., Citation2008). Since many anticancer drugs are known to induce apoptosis in cancer cells, targeting apoptosis regulators such as Caspase-3 has been suggested as an important strategy for anticancer drug discovery (Kashaw et al., Citation2019).
The safety of C. europaea extracts and its use as an anticancer agent in Moroccan popular phytomedicine inspired us to evaluate its cytotoxic effect on pancreatic carcinoma cell survival, apoptosis, as well as sensitization of cancer cells to the chemotherapy drug gemcitabine. The main natural compounds of CE have inhibitory effect against five structures of human Smo, and their potential induction of apoptosis by activation of human Caspase-3 was evaluated by molecular docking.
2. Materials and methods
2.1. Plant material
The aerial part of Caralluma europaea (Guss.) N.E.Br. was collected in spring 2021 from the Imouzzer mountains of the Middle Atlas of Morocco, this region is situated in the northeastern part of western Moroccan (30° 40′ 48″ N 9° 28′ 58″ W). Authentication of the plant was done by a botanist Pr. Bari Amina, and a voucher specimen #18I4C001 has been deposited in the herbarium of the Department of Biology, Faculty of Sciences, Dhar El Mahraz, University Sidi Mohamed Ben Abdellah, Fez. This was confirmed on The Plant List online database (http://www.theplantlist.org/). The aerial part of C. europaea was dried in a shaded place in a well-ventilated room before being ground into powder (). The choice of this plant was based on the results of our previous ethnopharmacological survey in Fez Meknes region (Amrati, Bourhia, Slighoua, Mohammad Salamatullah, et al., 2021).
2.2. Animal material
Wistar rats weighing approximately 122–199 g, and adult Swiss albino mice weighing approximately 25–30 g were obtained from the animal house of the Department of Biology, University Sidi Mohamed ben Abdellah, Faculty of Sciences, Fez. The animals were housed under controlled laboratory conditions of temperature (23 ± 2 °C) and light (12 h light/dark cycles), with uninhibited access to food and water. The use of laboratory animals in all experimental procedures was conducted according to the ethical guidelines for the care and the use of laboratory animals (National Research Council (US) Committee for the Update of the Guide for the Care & Use of Laboratory Animals, 2011).
2.3. Extraction of hydroethanolic, polyphenol, flavonoid and saponin fractions from C. europaea aerial parts
2.3.1. Preparation of the hydroethanolic extract
An amount of 10 g of C. europaea powder was extracted with 100 ml of ethanol 70% (70:30 v/v ethanol: distilled water) using the ultrasonic apparatus (SB-100DT) at a temperature of 25 °C for 45 min. The mixture was then filtered through Whatman paper and finally concentrated at 40 °C using a rotary evaporator (BUCHI461) (Amrati et al., 2020). The hydroethanolic extract yield was 13.25%.
2.3.2. Preparation of the polyphenolic fraction
An amount of 100 g of C. europaea powder was extracted three times with 300 ml of methanol. The solvent was then evaporated, and the obtained extract was dissolved in distilled water (500 ml). The aqueous extract was successively extracted with (200 ml*3) of hexane, chloroform and ethyl acetate solvents. The ethyl acetate phase was concentrated using a rotary evaporator (BUCHI461). Finally, the obtained residue was redissolved in distilled water (300 ml) and freeze-dried (laboao, LFD-18C) to get the polyphenolic fraction (Ho et al., Citation1992). The polyphenolic extract yield was 7%.
2.3.3. Preparation of the flavonoids extract
An amount of 15 g of C. europaea aerial parts powder was extracted with methanol (50 ml) for 72 h at ambient temperature. The obtained solution was filtered through Whatman paper and concentrated at 40 °C. The residue was dissolved in distilled water and the aqueous solution successively fractionated with chloroform (3*15 ml), diethyl ether (3*15 ml), n-hexane (3*15 ml), ethyl acetate (3*15 ml) and n-butanol (3*15 ml). The butanolic phase was recorded and then evaporated using a rotary evaporator (BUCHI461) at 40 °C to obtain the flavonoids fraction (Amrati et al., 2020). The flavonoids extract yield was 1.97%.
2.3.4. Preparation of the saponins extract
An amount of 10 g of C. europaea powder was extracted with hexane (100 ml) for 2 h. The defatted powder was macerated with ethanol (300 ml) for 1 d, and then evaporated using a rotary evaporator (BUCHI461) at 40 °C. The obtained extract was extracted with hexane and distilled water (50:50, v/v), with n-butanol solvent (150 ml) was added to the aqueous portion. The n-butanol fraction was evaporated at 40 °C to obtain the saponins fraction (Amrati et al., 2020). The saponins extract yield was 2.44%.
2.4. Phytochemical composition of C. europaea hydroethanolic extract
2.4.1. Solvents and reagents
Syringic acid SIM, gallic acid SIM, quercetin SIM, p coumaric acid SIM, hydroxytyrosol SIM, trans ferulic acid SIM, oleuropein SIM, hesperetin, trimethoxyflavone, arbutin, rosmarinic acid, ursolic acid, apigenin, amentoflavone, luteolin, naringin, rutin, kaempeferol, myricetin, procyanidin, catechin gallate, chlorogenic acid, trans-cinnamic acid, ferulic acid, sinapic acid, caffeic acid, gentisic acid, syringic acid, vanillic acid, protocatechuic acid, tyrosol, kaempferol-3-O-hexose deoxyhexose, kaempferol-3-O-pentose, kaempferol-3-O-glucuronic acid, luteolin-7-O-glucoside, isorhamnetin-3-O-rutinoside, Quercetin-3-O-hexose deoxyhexose, kaempferol-3-O-glucose, quercetin-3-O- glucuronic acid, quercetin-3-O-glucoside, isorhamnetin-7-O- pentose, acetonitrile and formic acid were purchased from Sigma Aldrich (Hamburg, Germany).
2.4.2. Analytical equipment
Qualitative analysis of the phenolic compounds in C. europaea hydroethanolic extract was performed using ultra high performance liquid chromatography (UHPLC) equipped with triple quadrupole mass spectrometry (LC–MS/MS). A developed database of phenolic compounds was used for the Caralluma europaea hydroethanolic extract screening based on m/z ion detection.
The chemical analysis was carried out by using Ultra-High-Performance Liquid Chromatography (Nexera, XR LC 40), coupled to a MS/MS detector (LCMS 8060, Shimadzu, Milan). The flow of nebulizer gas, heater gas, and dryer gas was 2.9 l/min, 10 l/min and 10 l/min respectively. The interface temperature, the DL temperature, as well as the heat block temperature were 300 °C, 250 °C and 400 °C, respectively. The MS/MS was done with electrospray ionization. Chemical profiling was performed on the mobile phase containing water: acetonitrile (95:5, v/v) + formic acid (0.01%) in ESI+ and ESI–. Chromatographic separation was achieved on a C18 Accucore Polar Premium (2.6 µm; 46 ∗150 mm) column with a total running time of 5 min, and flow rate of 0.7 ml/min in isocratic conditions.
The dried hydroethanolic extract of C. europaea was dissolved in 1 ml of saline phosphate Buffer-Acetonitrile 1:1 LCMS grade solution. After centrifugation, the supernatant was transferred into glass vial for UHPLC analysis.
2.5. Toxicity tests of C. europaea extracts
2.5.1. Acute toxicity
The acute toxicity assay was conducted following the Organization for Economic Cooperation and Development (OECD) guidelines no.423. Acute toxicity of C. europaea hydroethanolic extract at different doses was performed using 12 h fasted rats. Five groups, with five animals, each were used. Extracts were administered orally by gastric gavage as a single dose to animal groups as follows:
Group 1: Negative control (0.9% NaCl);
Group 2: Hydroethanolic extract at 100 mg/kg;
Group 3: Hydroethanolic extract at 300 mg/kg;
Group 4: Hydroethanolic extract at 2000 mg/kg;
Group 5: Hydroethanolic extract at 5000 mg/kg
Symptoms of toxicity such as the general appearance of the animal, body weight, relative weight of organs, behavior, somnolence, diarrhea, restlessness as well as mortality (if any) were observed and recorded. The acute toxicity test was used to determine the median lethal dose (LD50) of C. europaea hydroethanolic extract (Goni et al., Citation2021).
2.5.2. Sub-acute toxicity
The sub-acute toxicity of hydroethanolic, polyphenols, flavonoids and saponins extracts of C. europaea was performed according to the OECD guideline no.407. Twenty-five rats were randomly divided into five groups (n = 5/group) and treated for 28 consecutive days with C. europaea extracts as follows:
Group 1: Negative control (0.9% NaCl);
Group 2: Hydroethanolic extract (100 mg/kg);
Group 3: Polyphenol extract (50 mg/kg);
Group 4: Flavonoid extract (15 mg/kg);
Group 5: Saponin extract (10 mg/kg)
The mortality, behavioral changes, and weight of the animals were observed and recorded daily (Rhallab et al., Citation2014).
2.5.3. Serum biochemical analysis
At the end of the experimental period of acute and subacute toxicity tests, the animals were anesthetized with sodium pentobarbital at a dose of 30 mg/Kg before being sacrificed for blood and organ collection. Blood samples were collected from the heart, in heparinized tubes (0.2 ml with 10 U/ml heparin) and then centrifuged at 1500 rpm for 10 min. Next, the recovered plasma was analyzed for aspartate aminotransferase (AST), alanine aminotransferase (ALT), creatinine and urea parameters.
2.5.4. Relative organ weight
At the end of the acute and subacute toxicity test periods, the kidneys, livers, and spleens of treated animals were removed and weighed. The relative organ body weight (ROW) of each rat was calculated as:
(Ramadan et al., Citation2012). where ROW: Relative organ weight; Wt of organ: Weight of the organ (g); and Body Wt of animal: Body weight of the animal (g).
2.5.5. Histopathological examination
Livers and kidneys of rat groups from the subacute toxicity test were collected for histopathological examination. The tissues were fixed in a 10% formalin solution, dehydrated with alcohol, and then enclosed in paraplast. Afterward, tissues were embedded in paraffin and sectioned with a microtome. Sections of 5 µm thickness were cut and mounted on slides. All sections were stained with hematoxylin-eosin before being examined under a light microscope (Amrati, Bourhia, Slighoua, Boukhira, et al., Citation2021).
2.6. Anti-cancer activity of C. europaea
2.6.1. Cell culture
Human pancreatic cancer cell lines: MIA PaCa-2 (Poorly differentiated) and Bx-PC3 (Moderately differentiated) were received from the American Type Culture Collection (Manassas, VA). Bx-PC3 was grown in RPMI 1640 supplemented with antibiotic/antimycotics solution (1%) and fetal bovine serum (10%). MIA PaCa-2 cells were cultured in 1:1 DMEM/F-12 medium (GIBCO Invitrogen Corporation, Grand Island, NY) complemented with antibiotic/antimycotics solution (1%), fetal bovine serum (15%) and glutamine (4 mM l). Cells were kept at 37 °C in a humidified incubator containing CO2 (5%). For the experiments, MIA PaCa-2 and Bx-PC3 cells were incubated for 72 h. MIA PaCa-2 cells resistant to gemcitabine were obtained by treating MIA PaCa-2 cells for 2 or 3 weeks with increasing doses of gemcitabine drug as follow: 1, 5, 30, 60 and 120 ng/ml, respectively.
2.6.2. Cell survival
Cell survival was assessed by MTT assay and Cell Death Detection ELISA Plus kit (Roche Applied Science, Los Angeles, CA) according to the manufacturer’s instructions (Edderkaoui et al., 2018). Gemcitabine was used as a positive control. The percentage of cell survival was calculated as follows:
OD: Optical density
2.6.3. Western blot analysis
Cells were suspended in RIPA lysis buffer Tris/HCl (50 mM), NaCl (50 mM), Triton X-100 (1%), SDS (0.1%), deoxycholic acid (1%), Na3VO4 (2 mM), PMSF (1 mM), Na2HPO4 (10 mM) + NaH2PO4, glycerophosphate (80 μM), NaF (100 mM), glycerol (20%), 5 μg/ml each of leupeptin, antipain, pepstatin, aprotinin and chymostatin. Lysates were then centrifuged (4 °C, 15 min). The supernatant proteins were separated using SDS–PAGE and transferred to PVDF or nitrocellulose membranes. Bovine serum albumin (5%) or nonfat dry milk in Tris-buffered saline (TBS) containing Tween 20 (0.05%) was used to block nonspecific binding. Membranes were incubated one night at 4 °C with primary antibody, and after that with peroxidase-conjugated secondary antibody for about 1 h. Blots were developed using Super Signal Chemiluminescent Substrate (Pierce, Rockford, IL) (Edderkaoui et al., 2018).
2.6.4. Measurements of apoptosis
Inter-nucleosomal DNA fragmentation was determined by Cell Death Detection ELISA Plus kit (Roche Molecular Biochemicals, Mannheim, Germany) in concordance with the manufacturer’s instructions.
2.7. Molecular docking
2.7.1. Ligand preparation
The identified molecules (luteolin 7-O-glucoside, quercetin, luteolin, hesperetin, kaempferol, trimethoxyflavone, caffeic acid, gallic acid, ferulic acid, syringic acid, tyrosol, sinapic acid and isorhamnetin- 3-O-rutinoside) are downloaded from PubChem in SDF format. Ligands were then prepared for molecular docking using the OPLS3 force field LigPrep tool in the Maestro 11.5 version Schrödinger Software program. A maximum of 32 stereoisomers were produced for each ligand after the ionization states at pH 7.0 ± 2.0.
2.7.2. Protein preparation
For human Smo receptors, the three-dimensional crystal structure was downloaded in PDB format from the protein data bank using the following PDB IDs: 5L7I, 4QIM, 4QIN, 4O9R and 4N4W. For the Caspase-3 receptor, the three-dimensional crystal structure was downloaded from PDB ID:3GJQ. Then all the structures are prepared and refined using the Protein Preparation Wizard of Schrödinger-Maestro version 11.5 (New York, NY, USA: Schrödinger, LLC, 2018). Charges and bond orders were assigned, hydrogens were added to the heavy atoms, selenomethionines were converted to methionines, and all waters were deleted. Using force field OPLS3, minimization was carried out setting maximum heavy atom root-mean-square-deviation (RMSD) to 0.30 Å.
2.7.3. Receptor grid generation
The receptor grid is created automatically by clicking on any atom of the ligand. For the Caspase-3 receptor, the volumetric spacing performed was 20 × 20 × 20 and the coordinates were taken as x: 28.642, y: 34.169 and z: 12.047. For the five human Smo receptors, the receptor grid was prepared by the same method described for Caspase-3, regarding the volumetric spacing defined as 20 × 20 × 20 for each receptor. The defined XYZ coordinates are according to .
Table 1. Receptor grid coordinates of human Smo receptors (PDB ID).
2.7.4. Glide standard precision (SP) ligand docking
The docking of all phenolic compounds identified in the hydroethanolic extract of C.E was carried out using the SP flexible ligand docking from Glide of Schrödinger-Maestro version 11.5. Van der Waals scaling factor and partial charge cutoff for the ligand atoms were selected at 0.80 and 0.15, respectively. Final scoring was executed on energy-minimized and displayed as Glide score. The best-docked pose with the lowest Glide score value was recorded for each ligand (Banerjee et al., Citation2011).
2.8. Statistical analysis
Statistical analysis was done by one-way analysis of variance (ANOVA) with GraphPad Prism (GraphPad software, La Jolla, CA). The data were expressed as means ± standard error of the mean (SEM) from at least three different experiments. p value < 0.05 was considered statistically significant.
3. Results
3.1. Qualitative profiling of C. europaea hydroethanolic extract revealed the presence of several phenolic compounds
Through the LC–MS/MS screening of C.E hydroethanolic extract, several compounds were identified. As shown in , kaempferol, luteolin and kaempferol-3-O-hexose deoxyhexose are the major phenolic compounds contained in C.E hydroethanolic extract, according to the intensity of the given signal. The AUC of the identified phenolic compounds is presented in .
Figure 2. LC–MS/MS results based on AUC (Area Under Curve) of Caralluma europaea hydroethanolic extract phenolic compounds through direct infusion.
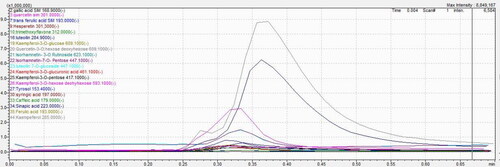
Table 2. Principal phenolic compounds revealed in C. europaea hydroethanolic extract through direct infusion.
3.2. Evaluation of the toxicological effect of C. europaea extracts
The toxicity effect of hydroethanolic, polyphenols, flavonoids and saponins extracts of C.E were evaluated to determine the toxicity of C.E used traditionally in the treatment of cancer in Morocco, as well as the fraction of the plant which may be responsible for its toxic effect.
3.2.1. General signs and body weight
During the acute toxicity test of C.E hydroethanolic extract at the doses of 100, 300, 2000 and 5000 mg/kg and the subacute toxicity of the total extract, polyphenols, flavonoids and saponins at the doses of 100, 50, 15 and 10 mg/kg, respectively, no animal death recorded by the end of the two tests, and all animal groups showed no behavioral or body weight changes ( and ).
Table 3. Effect of C. europaea hydroethanolic extract on weekly body weight gain of rat groups (n = 5). Values expressed as mean ± SEM.
Table 4. Effect of C. europaea extracts on weekly body weight gain of rat groups (n = 5). Values expressed as mean ± SEM.
The relative organ weight (ROW) results of kidneys, livers, and spleens of acute and subacute toxicities are presented in and , respectively. For the acute toxicity test at doses of 100, 300, 2000 and 5000 mg/kg, the relative organ weight of the kidneys, livers and spleens were all within the normal range of the control group. Similar results were obtained for subacute toxicity of hydroethanolic, polyphenols, flavonoids and saponins extracts at 100, 50, 15 and 10 mg/kg, respectively.
Table 5. The relative organ weight (ROW) results of rats treated orally with C. europaea hydroethanolic extract. Values are expressed as mean ± SEM (n = 5).
Table 6. The relative organ weight (ROW) results of rats treated orally with C. europaea extracts. Values are expressed as mean ± SEM (n = 5).
3.2.2. Biochemical parameters
Quantification of AST, ALT, creatinine (CREA) and urea of rats fed with C.E extracts in the acute and subacute toxicity tests are presented in and .
Table 7. Effects of C. europaea hydroethanolic extract on the liver (aspartate transaminase (AST), alanine aminotransferase (ALT)), and the kidney (creatinine (CREA) and urea) quantification during the acute toxicity test. (*) indicates a significant difference at p < 0.05 from the control group using one-way ANOVA followed by Dunnett test. Values are expressed as mean ± SEM (n = 5).
Table 8. Effects of hydroethanolic, polyphenols, flavonoids and saponins extracts on the liver (aspartate transaminase (AST), alanine aminotransferase (ALT)), and the kidney (creatinine (CREA) and urea) quantification during the subacute toxicity test.
The acute toxicity test of hydroethanolic extract at the doses of 100, 300, 2000 and the subacute toxicity test of total extract, polyphenols, flavonoids at 100, 50 and 15 mg/kg, respectively, did not present any significant differences when compared with those of control group (NaCl) (p < 0.05). The acute toxicity of the hydroethanolic extract at 5000 mg/kg displayed a significant increase in AST, and the subacute toxicity of the saponin extract presented an increase in AST and ALT.
3.2.3. Histopathological examination of subacute toxicity test rat tissues
Compared with the control group, the histopathological examination of rat kidneys showed no lesions or pathological changes attributable to treatment with hydroethanolic, polyphenols, flavonoids or saponins extracts of C. europaea at the doses of 100, 50, 15, 10 mg/kg, respectively. Microscopic observation of liver sections of rats treated with C. europaea hydroethanolic, polyphenols and flavonoids extracts showed no remarkable pathological differences when compared to the control group. However, the liver histology of rats treated with saponins extract at 10 mg/kg showed a number of pathological changes: sinus dilatation, inflammatory cell infiltration of neutrophils, and lymphocytes ().
Figure 3. Histopathological photomicrographs of kidney and liver slices stained with hematoxylin-eosin (×40) after oral administration of C. europaea hydroethanolic, polyphenols, flavonoids and saponins extracts in rats on day 28 of the subacute toxicity. (A–E) Kidney section of groups treated with NaCl, hydroethanolic, polyphenol, flavonoid and saponin extracts, respectively. (F–J) Liver section of groups treated with NaCl, hydroethanolic, polyphenol, flavonoid and saponin extracts, respectively. A, Arteriole; G, Glomerulus; CPT, Peritubular capillaries; VC, Central vein; VP, Portal vein; CB, Bile duct; HA, Hepatic artery; H, Hepatocyte; N, Neutrophil; L, Leukocyte.
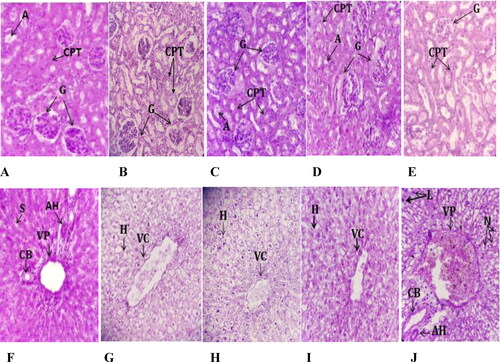
3.3. Effects of C. europaea extracts on pancreatic cancer
3.3.1. C. Europaea extracts dose dependently decrease pancreatic cancer cell survival
Treatment of Bx-PC3 (pancreatic cancer cells with an epithelial phenotype) and MIA PaCa-2 (pancreatic cancer cells with a mesenchymal phenotype) cells with increasing doses (1 mg/ml, 100 µg/ml and 10 µg/ml) of hydroethanolic, polyphenol, flavonoid and saponin extracts of C. europaea, dose-dependently reduced cell survival of both cell cultures, except for hydroethanolic extract on MIA-PaCa-2 ().
The saponins fraction was the most potent of all the C. europaea extracts, with significance achieved at the low dose of 10 µg/ml in both cell lines. Saponins decreased cancer cell survival by over 50% at 10 µg/ml and completely prevented all cancer cell survival at the doses of 100 µg/ml and 1 mg/ml (). The flavonoids fraction completely inhibited MIA PaCa-2 cells survival at the dose of 1 mg/ml, and decreased survival to 40% at the dose of 100 µg/ml, with an IC50 value of 32.34 µg/ml (). The polyphenols (IC 50 = 1.196 mg/ml) and total extract (IC 50 = 3.404 mg/ml) induced a significant decrease in cancer cell survival in Bx-PC3 cells at the doses of 1 mg/ml and 100 µg/ml, respectively (); whereas MIA PaCa-2 cells were slightly more resistant ().
Figure 4. Bx-PC3 (A) and MIA PaCa-2 (B) cells were cultured for 72 h in the presence of C. europaea extracts with different doses of total extract, polyphenols, flavonoids and saponins. Cell survival was measured by MTT assay. Values are expressed as mean ± SEM (n = 3). *p < 0.05 compared to the control.
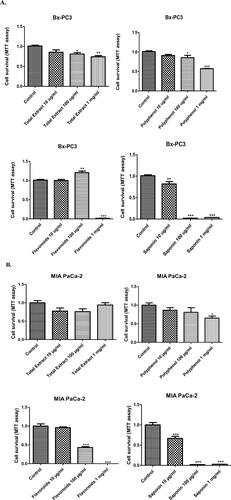
3.3.2. Combination of C. europaea extracts with gemcitabine treatment has an additive effect on cancer cell survival in MIA-PaCa-2 and Bx-PC3 cells
To test the possibility of an additive or synergistic anti-cancer effect when combining C. europaea extracts with gemcitabine, the standard chemotherapy drug used for treating pancreatic cancer patients, we treated MIA PaCa-2 and Bx-PC3 cells with each of the C. europaea extracts and gemcitabine, alone and in combination. We applied low doses of gemcitabine and the C. europaea extracts.
Treatment with gemcitabine alone induced a decrease in cancer cell survival in Bx-PC3 and MIA PaCa-2 (41% and 14%, respectively). shows that the combination of gemcitabine at 15 ng/ml with hydroethanolic, polyphenol, flavonoid extracts at the indicated doses had a significant additive effect inhibiting cancer cell survival. The strongest additive effect was achieved by flavonoids, whereas, saponins did not further sensitize cancer cells to gemcitabine.
3.3.3. C. Europaea extracts regulate expression of cancer stemness markers, the mediators of chemo-resistance
Cancer stemness is considered the driving force behind chemo-resistance. Based on the effects observed in , some of the C. europaea extracts had additive effects when combined with gemcitabine, especially in Bx-PC3 cells. Therefore we assessed the effect of the C. europaea extracts on cancer stemness markers. We found a significant decrease in multiple markers of cancer stemness in Bx-PC3, but less in MIA PaCa-2. In Bx-PC3 cells, total extract, polyphenols and flavonoids decreased the levels of Nanog and CD133; while only flavonoids and saponins decreased the levels of Sox-2 (). In MIA PaCa-2 cells, all extracts decreased the level of CD133, while only flavonoids decreased the level of Sox-2 (). Nanog was not detected in MIA PaCa-2 cells. This data indicates that most of the C. europaea extracts down-regulate some of the cancer stemness markers, and that flavonoid extract is the only extract that decreased all cancer stemness markers in both cell lines. This explains why flavonoids were the only extract that sensitized both cancer cell lines to gemcitabine.
3.3.4. C. Europaea extracts (except flavonoids) combined with gemcitabine induced internucleosomal DNA fragmentation in gemcitabine-resistant MIA PaCa-2
Resistance to apoptosis is a key factor preventing response to therapies in pancreatic cancer. We found that C.C. europaea extracts induced apoptosis in MIA PaCa-2 cancer cells.
Polyphenols and flavonoids extracts of C. europaea stimulated apoptosis in the wild type MIA PaCa-2 cells. However, C. europaea extracts combined with gemcitabine didn’t induce any additional effect on apoptosis ().
Figure 8. Internucleosomal DNA fragmentation. (A) MIA PaCa-2 cells; (B) MIA PaCa-2 gemcitabine resistant cells. Values are standardized to the control. Values are expressed as mean ± SEM (n = 3). *p< 0.05 vs. control.
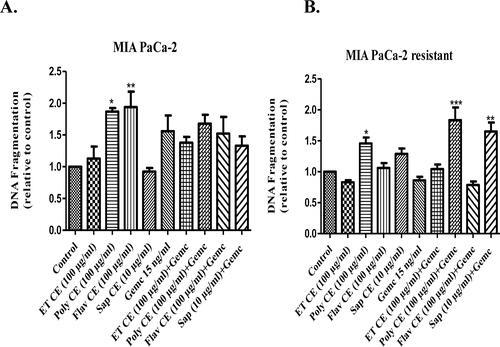
Furthermore, the polyphenol extract induced a significant apoptotic effect in MIA PaCa-2 gemcitabine-resistant cells. In the presence of gemcitabine, polyphenols and saponins increased internucleosomal DNA fragmentation in gemcitabine-resistant MIA-PaCa-2 cells ().
3.3.5. Caralluma europaea natural compounds enhanced Hh pathway inhibition, and induced apoptosis via caspase-3 activation using a molecular docking study
The majority of Caralluma europaea natural molecules exhibited a remarkable inhibitory effect on human Smo receptor active sites and activating Caspase-3 receptor. presents the glide score (Gscore) of molecules (ligands) identified by UHPLC in the C. europaea hydroethanolic extract. In the active site of 5L7I, luteolin 7-O-glucoside was the most active molecule followed by quercetin with a glide score of −8.387 and −7.678 kcal/mol, respectively. Luteolin was the most active molecule in the active sites of 4QIM and 4QIN receptors with a docking score of −8.746 and −8.041 kcal/mol, respectively. In the active site of 4N4W, trimethoxyflavone and luteolin 7-O-glucoside showed a docking score of −9.091 kcal/mol and −8.514, respectively.
Table 9. Docking results with ligands in human Smo and Caspase-3 receptor.
The five active sites of human Smo, luteolin 7-O-glucoside, luteolin, quercetin, hesperetin and kaempferol showed better docking scores, compared with gemcitabine (−7.491 kcal/mol).
Concerning the human Caspase-3 receptor, isorhamnetin-3-O-rutinoside is the most active molecule in the active site followed by gallic acid, with a glide score of −9.038 and −7.219 kcal/mol, respectively. Luteolin 7-O-glucoside, quercetin, luteolin, hesperetin, syringic acid and isorhamnetin-3-O-rutinoside showed a docking score higher than gemcitabine (−6.221 kcal/mol).
and present the number and types of possible bonds between the ligands and the active sites of the human Smo and Caspase-3 receptor. In the 5L7I receptor, luteolin 7-O-glucoside formed 5 hydrogen bonds with residues: Asn 219, Tyr 394, Gln 477, Gly 383 and Asp 384. In 4QIM and 4QIN, luteolin established 4 hydrogen bonds with the first receptor, and 2 hydrogen bonds with the second. The residues formed in the active site of 4QIM are Gly 383, Gln 477, Asp 473 and Glu 518. The active site of 4QIN forms a Glu 518 residue.
Figure 9. 2D diagrams of ligands interactions with the active sites. (A) Luteolin 7-O-glucoside interactions with the active site of 5L7I. (B and C) Luteolin interactions with the active site of 4QIM and 4QIN, respectively. (D) Isorhamnetin-3-O-rutinoside interactions with the active site of 4O9R. (E) Trimethoxyflavone interactions with the active site of 4N4W. (F) Isorhamnetin-3-O-rutinoside interactions with the active site of the Caspase-3 receptor (3GJQ).
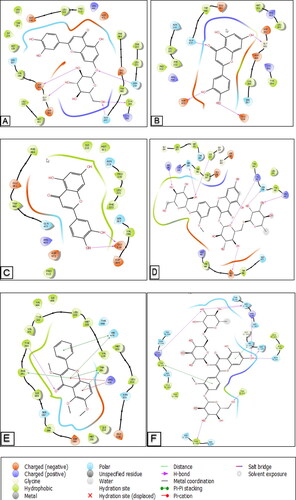
Figure 10. 3D diagrams of ligands interactions with the active sites. (A) Luteolin 7-O-glucoside interactions with the active site of 5L7I. (B and C) Luteolin interactions with the active site of 4QIM and 4QIN, respectively. (D) Isorhamnetin-3-O-rutinoside interactions with the active site of 4O9R. (E) Trimethoxyflavone interactions with the active site of 4N4W. (F) Isorhamnetin-3-O-rutinoside interactions with the active site of the Caspase-3 receptor (3GJQ).
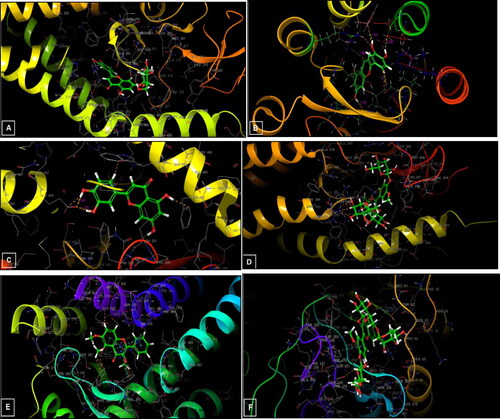
In the active site of 4N4M, trimethoxyflavone established a single hydrogen bond with the residue Tyr 394, five bonds Pi-Pi stacking with the residues His 470, Trp 281 and Phe 391, and two Pi-cation bonds with Arg 400. Furthermore, isorhamnetin-3-O rutinoside established 8 hydrogen bonds and 2 Pi Bond-Pi stacking in the caspase-3 active site.
4. Discussion
Plant species produce an unlimited number of bioactive compounds. Among these bioactive compounds are polyphenols, flavonoids and saponins, which have been profusely studied for their antitumor effect (Kregiel et al., Citation2017; Pérez-Soto et al., Citation2019). In Moroccan traditional medicine, C. europaea has been used to treat various diseases including cancer (Amrati et al., 2020). The anticancer effects of C. europaea extracts on pancreatic cancer have not been previously studied. In this study, C. europaea extracts were investigated for their acute and subacute toxicities, as well as their antitumor effect on two pancreatic cancer cell lines.
Acute toxicity results showed neither incidence of mortality, nor signs of toxicity at all tested doses. Regarding the biochemical parameters, groups treated at doses of 100, 300 and 2000 mg/kg did not show any significant modification compared to the negative group. However, the dose of 5000 mg/kg leads to an increase in the AST parameter. Therefore, the LD50 of C. europaea hydroethanolic extract was higher than 5000 mg/kg and it may be considered non-toxic at concentrations lower than 5000 mg/kg. These results are in agreement with another study reporting that the LD50 of ethanolic and ethyl acetate extracts of C. europaea is greater than 5000 mg/kg (Kebbou et al., Citation2019).
The subacute toxicity of C. europaea hydroethanolic, polyphenol, and flavonoid extracts at 100, 50 and 15 mg/kg, respectively, did not cause any change in body weight, relative organ weight, biochemical parameters or histopathological differences in the liver and kidney, compared to the negative control. Our results highlighted the biosafety of C. europaea hydroethanolic, polyphenols and flavonoids extracts at the tested doses.
However, saponins fraction at the dose of 10 mg/kg slightly decreased the animals’ body weight. This decrease in body weight may be related to (i) the decrease in food and water consumption (Yang et al., Citation2019); (ii) the exposure time to saponins extract, (iii) or probably caused by the effect of saponins. In previous research, saponins have been considered responsible for decreasing food intake, reducing weight gain, and inhibiting the active uptake of nutrients (Chen et al., Citation2011).
Saponin extract also caused an increase in ALT and AST parameters, in addition to histological signs of toxicity in the livers of treated animals. A similar result was reported in previous research which demonstrated that Pulsatilla chinensis saponins cause liver injury, and that the damage to the rats’ liver was time-dependent manner (Su et al., Citation2020).
The analysis of the phytochemicals in the C. europaea hydroethanolic extract by LC–MS/MS revealed the presence of gallic acid, quercetin, luteolin 7-O-glucoside, quercetin-3-O-hexose deoxyhexose, kaempferol-3-O-hexose deoxyhexose, ferulic acid, trans-ferulic acid, kaempferol-3-O-pentose, hesperetin, trimethoxyflavone, syringic acid, isorhamnetin-7-O- pentose, luteolin, kaempferol-3-O-glucose, isorhamnetin- 3-O rutinoside, kaempferol-3-O-glucuronic acid, tyrosol, caffeic acid, kaempferol and sinapic acid (; ).
In this research work, C. europaea extracts induced a significant decrease in cancer cell survival in two pancreatic cancer cell lines: Bx-PC3 and MIA PaCa-2 (). More importantly, some C. europaea extracts may cause (i) sensitization of pancreatic cancer cells to chemotherapy, (ii) decrease in the expression of cancer stemness markers, (iii) induction of apoptosis, (iv) inhibition of the Hh pathway and (v) activation of Caspase-3.
In vitro survival data in Bx-PC3 and MIA PaCa-2 cell lines showed a strong cytotoxic effect of polyphenols and flavonoids. Most importantly, saponins clearly exhibited an important effect at the three doses of 1, 100 and 10 µg/ml on both cell lines. However, the total extract had little anti-cancer effect (). The cytotoxic effect of C. europaea polyphenols and flavonoids in Bx-PC3 and MIA-PaCa-2 cell lines is most likely due to the presence of phenolic compounds in the tested extracts ().
The apoptosis induced by C. europaea extracts may be related to their LC–MS/MS identified phenolic compounds. Ferulic acid (Wang et al., Citation2016), quercetin (Reyes-Farias & Carrasco-Pozo, Citation2019), hesperetin (Zhang et al., Citation2015), luteolin (Li et al., Citation2018), kaempferol (Huang et al., Citation2010), isorhamnetin (Antunes-Ricardo et al., Citation2014), syringic acid (Abijeth & Ezhilarasan, Citation2020), caffeic acid (Chang et al., Citation2010) and sinapic acid (Janakiraman et al., Citation2014) have been found to inhibit cancer development by promoting apoptosis ().
Saponins exhibit several biological activities and have been proposed for the treatment of cancer. The efficacy of C. europaea against Bx-PC3 and MIA PaCa-2 cell lines may be attributed to its ability to stimulate apoptosis and inhibit cell proliferation ( and ). However, the action of saponins on hemolysis may limit their therapeutic uses (Kregiel et al., Citation2017).
The antitumoral activities of many species of Caralluma genus have been studied in early research works. The crude extracts of Caralluma lasiantha, Caralluma diffusa, Caralluma umbellata and Caralluma attenuate were performed against multiple human cancer cells (breast, lung, colon, cervical and prostate cancer cell lines) and have shown a moderate cytotoxic effect (Bellamakondi et al., Citation2014).
As shown in , we found an additive effect on inhibiting cancer cell survival when combining total extract, polyphenols and flavonoids of C. europaea at the dose of 100 µg/ml, with a low dose of gemcitabine. The effect was observed in both cell lines but was stronger in Bx-PC3 compared to MIA PaCa-2. C. europaea extracts can sensitize pancreatic cancer cells to gemcitabine.
The resistance of cancer cells to chemotherapeutics represents the main clinical challenge in the development of cancer treatments. It has been suggested that CSCs are responsible for this resistance. So, targeted therapy of CSCs could inhibit cancer proliferation (Glumac & LeBeau, Citation2018).
Nanog, CD-133 and Sox-2 have been proposed to mark CSCs in a diverse list of cancers, including PDAC. Current clinical research has demonstrated that aberrant expression of Nanog, Sox-2 and CD-133 are considered to be bad prognoses for cancer progression (Gawlik-Rzemieniewska & Bednarek, Citation2016; Glumac & LeBeau, Citation2018; Weina & Utikal, Citation2014).
Total extract, polyphenol, flavonoid and saponin extracts of C. europaea decreased the levels of Nanog, CD-133 and Sox-2 cancer stemness proteins in both cell lines Bx-PC3 and MIA PaCa-2, with a stronger effect in Bx-PC3 cells (). The importance of these results is consequence of cytotoxic therapies, used for treating pancreatic cancer, promoting drug resistance and cancer stemness (Volk-Draper et al., Citation2014). CC. europaea extracts can reverse cancer cells resistance developed from treatment with gemcitabine ( and ).
Flavonoids extracted from C. europaea appear to have a very important cytotoxic effect when used alone and is the only extract that induced a significant sensitization of both cancer cell lines to gemcitabine. Therefore, C. europaea flavonoids are the most promising therapeutic extract.
Our findings are in agreement with previous works where luteolin was identified as an inhibitor of the Hh signaling pathway (Arai et al., Citation2013). Whereas, luteolin 7-O-glucoside was mentioned as a powerful molecule against several types of cancer such as breast cancer and oral squamous carcinoma (Goodarzi et al., Citation2020; Velmurugan et al., Citation2020). Regarding Caspase-3 activation, isorhamnetin-3-O- rutinoside exhibited strong activity against the active site of Caspase-3, with a glide score of −9.038 kcal/mol. This finding is in accordance with previous research that demonstrated the role of isorhamnetin-3-O-rutinoside as an important executioner of apoptosis (Boubaker et al., Citation2011; Antunes-Ricardo et al., 2021).
In the five active sites of human Smo, luteolin 7-O-glucoside, luteolin, quercetin, hesperetin and kaempferol demonstrated higher docking scores compared to gemcitabine (−7.491 kcal/mol). Many studies demonstrated that quercetin inhibited the Hh signaling pathway, survival, and proliferation of cancer cells via induction of apoptosis (Salama et al., Citation2019; Mousavi et al., Citation2021).
Conclusion
The hydroethanolic, polyphenols and flavonoids extracts of C. europaea at 100, 50, and 15 mg/kg may be considered safe for further therapeutic uses. However, the saponins extract at the dose of 10 mg/kg shows little toxicity during daily oral administration and should, therefore, be used with caution. C. europaea extracts could (i) inhibit pancreatic cancer cell proliferation in combination with gemcitabine via inhibition of the Hh pathway, (ii) induce apoptosis via activation of human caspase-3, (iii) and sensitize the pancreatic cancer cells to chemotherapy. The obtained results could be the key to the introduction of C. europaea as a co-therapeutic agent in pancreatic cancer treatment. The findings of our study support the ethnomedicinal value of C. europaea extracts as a natural treatment for cancer, and offered innovative in vitro results. Other advanced pharmacological studies on C. europaea effect, individually and in nano-formulations, will be a very interesting course to promote general health care.
Institutional review board statement
The institutional ethical committee of care and use of laboratory animals at the Faculty of Sciences Dhar El Mehraz, Sidi Mohamed Ben Abdallah Fez University, Morocco, reviewed and approved the present study NO-04/2021/LBEAS.
Authors’ contributions
F.Z.A: Investigation, Conceptualization, Data curation, Software, writing original draft; OHM.E: Investigation, Data analysis, review & editing; M.C: Data analysis; Validation; B.S: Data curation, Investigation; R.C: Formal analysis; M.S: Investigation; A.S: Validation, Data analysis, Resources; O.A: Data curation, visualization; T.Z, A.D: Investigation; M.E: Resources, Formal analysis, Methodology; review and editing; D.B: Investigation, Visualization; Supervision.
Abbreviations | ||
A | = | arteriole |
ALT | = | alaninine aminotransferase |
AST | = | aspartate aminotransferase |
CB | = | bile duct |
AUC | = | area under curve |
ACN | = | acetonitrile |
Arg | = | arginine |
Asn | = | asparagine |
Asp | = | aspartic acid |
C.E | = | Caralluma europaea (Guss.)/C. europaea |
CPT | = | peritubular capillaries |
CRE | = | creatinine |
CSCs | = | cancer stem cells |
ET | = | hydroethanolic extract |
Flav | = | flavonoids |
G | = | glomerulus |
Gln | = | glutamine |
Glu | = | glutamic acid |
Gly | = | glycine |
H | = | hepatocyte |
HA | = | hepatic artery |
Hh | = | hedgehog pathway |
His | = | histidine |
LC-MS/MS | = | liquid chromatography tandem mass spectrometry |
LD50 | = | median lethal dose |
N | = | neutrophil |
OECD | = | Organization for Economic Cooperation and Development |
MeOH | = | methanol |
MTT | = | 3-(4,5-dimethylthiazol-2-yl)-25-diphenyltetrazolium bromide |
PDAC | = | pancreatic ductal adenocarcinoma |
Phe | = | phenylalanine |
Poly | = | polyphenols |
ROW | = | relative organ body weight |
Sap | = | saponins |
TBS | = | tris-buffered saline |
Trp | = | tryptophan |
Tyr | = | tyrosine |
UHPLC | = | ultra high performance liquid chromatography |
VC | = | central vein |
VP | = | portal vein |
Acknowledgments
The authors extend their appreciation to Princess Nourah bint Abdulrahman University Researchers Supporting Project number (PNURSP2022R141), Princess Nourah bint Abdulrahman University, Riyadh, Saudi Arabia.
Disclosure statement
Authors declare that there are no conflicts of interest.
Additional information
Funding
References
- Abijeth, B., & Ezhilarasan, D. (2020). Syringic acid induces apoptosis in human oral squamous carcinoma cells through mitochondrial pathway. Journal of Oral and Maxillofacial Pathology, 24(1), 40–45. https://doi.org/10.4103/jomfp.JOMFP_178_19
- Adnan, M., Jan, S., Mussarat, S., Tariq, A., Begum, S., Afroz, A., & Shinwari, Z. K. (2014). A review on ethnobotany, phytochemistry and pharmacology of plant genus Caralluma R. Br. The Journal of Pharmacy and Pharmacology, 66(10), 1351–1368. https://doi.org/10.1111/jphp.12265
- Aly, A. A., Sayed, S. M., Abdelhafez, E. S., Abdelhafez, S. M. N., Abdelzaher, W. Y., Raslan, M. A., Ahmed, A. E., Thabet, K., El-Reedy, A. A. M., Brown, A. B., & Bräse, S. (2020). New quinoline-2-one/pyrazole derivatives; design, synthesis, molecular docking, anti-apoptotic evaluation, and caspase-3 inhibition assay. Bioorganic Chemistry, 94, 103348. https://doi.org/10.1016/j.bioorg.2019.103348
- Amrati, F. E, Z., Bourhia, M., Slighoua, M., Ibnemoussa, S., Bari, A., Ullah, R., Amaghnouje, A., Di Cristo, F., El Mzibri, M., Calarco, A., Benbacer, L., & Bousta, D. (2020). Phytochemical study on antioxidant and antiproliferative activities of moroccan Caralluma europaea extract and its bioactive compound classes [WWW Document]. Evidence-Based Complementary and Alternative Medicine, 2020, 8409718. https://doi.org/10.1155/2020/8409718
- Amrati, F. E.-Z., Bourhia, M., Slighoua, M., Boukhira, S., Ullah, R., Ezzeldin, E., Mostafa, G., Grafov, A. E., & Bousta, D. (2021). Protective effect of chemically characterized polyphenol-rich fraction from Apteranthes europaea (Guss.) Murb. subsp. maroccana (Hook.f.) plowes on carbon tetrachloride-induced liver injury in mice. Applied Sciences, 11(2), 554. https://doi.org/10.3390/app11020554
- Amrati, F. E.-Z., Bourhia, M., Slighoua, M., Mohammad Salamatullah, A., Alzahrani, A., Ullah, R., Bari, A., & Bousta, D. (2021). Traditional medicinal knowledge of plants used for cancer treatment by communities of mountainous areas of Fez-Meknes-Morocco. Saudi Pharmaceutical Journal, 29(10), 1185–1204. https://doi.org/10.1016/j.jsps.2021.09.005
- Antunes-Ricardo, M., Guardado-Félix, D., Rocha-Pizaña, M. R., Garza-Martínez, J., Acevedo-Pacheco, L., Gutiérrez-Uribe, J. A., Villela-Castrejón, J., López-Pacheco, F., & Serna-Saldívar, S. O. (2021). Opuntia ficus-indica extract and Isorhamnetin-3-O-glucosyl-rhamnoside diminish tumor growth of colon cancer cells xenografted in immune-suppressed mice through the activation of apoptosis intrinsic pathway. Plant Foods for Human Nutrition, 76(4), 434–441. https://doi.org/10.1007/s11130-021-00934-3
- Antunes-Ricardo, M., Moreno-García, B. E., Gutiérrez-Uribe, J. A., Aráiz-Hernández, D., Alvarez, M. M., & Serna-Saldivar, S. O. (2014). Induction of apoptosis in colon cancer cells treated with isorhamnetin glycosides from opuntia ficus-indica pads. Plant Foods for Human Nutrition (Dordrecht, Netherlands), 69(4), 331–336. https://doi.org/10.1007/s11130-014-0438-5
- Arai, M. A., Fujimatsu, T., Uchida, K., Sadhu, S. K., Ahmed, F., & Ishibashi, M. (2013). Hh signaling inhibitors from Vitex negundo; naturally occurring inhibitors of the GLI1–DNA complex. Molecular bioSystems, 9(5), 1012–1018. https://doi.org/10.1039/C3MB25567K
- Banerjee, K., Gupta, U., Gupta, S., Wadhwa, G., Gabrani, R., Sharma, S. K., & Jain, C. K. (2011). Molecular docking of glucosamine-6-phosphate synthase in Rhizopus oryzae. Bioinformation, 7(6), 285–290. https://doi.org/10.6026/007/97320630007285
- Bayraktar, S., Bayraktar, U. D., & Rocha-Lima, C. M. (2010). Recent developments in palliative chemotherapy for locally advanced and metastatic pancreas cancer. World Journal of Gastroenterology, 16(6), 673–682. https://doi.org/10.3748/wjg.v16.i6.673
- Bellamakondi, P. K., Godavarthi, A., Ibrahim, M., Kulkarni, S., Naik, R. M., & Maradam, S. (2014). In vitro cytotoxicity of caralluma species by mtt and trypan blue dye exclusion. Asian Journal of Pharmaceutical and Clinical Research, 7, 17–19. https://innovareacademics.in/journals/index.php/ajpcr/article/view/836
- Boubaker, J., Bhouri, W., Ben Sghaier, M., Ghedira, K., Dijoux Franca, M. G., & Chekir-Ghedira, L. (2011). Ethyl acetate extract and its major constituent, isorhamnetin 3-O-rutinoside, from Nitraria retusa leaves, promote apoptosis of human myelogenous erythroleukaemia cells. Cell Proliferation, 44(5), 453–461. https://doi.org/10.1111/j.1365-2184.2011.00772.x
- Chang, W. C., Hsieh, C. H., Hsiao, M. W., Lin, W. C., Hung, Y. C., & Ye, J. C. (2010). Caffeic acid induces apoptosis in human cervical cancer cells through the mitochondrial pathway. Taiwanese Journal of Obstetrics & Gynecology, 49(4), 419–424. https://doi.org/10.1016/S1028-4559(10)60092-7
- Chen, W., Ai, Q., Mai, K., Xu, W., Liufu, Z., Zhang, W., & Cai, Y. (2011). Effects of dietary soybean saponins on feed intake, growth performance, digestibility and intestinal structure in juvenile Japanese flounder (Paralichthys olivaceus). Aquaculture, 318(1–2), 95–100. https://doi.org/10.1016/j.aquaculture.2011.04.050
- Conroy, T., Desseigne, F., Ychou, M., Bouché, O., Guimbaud, R., Bécouarn, Y., Adenis, A., Raoul, J.-L., Gourgou-Bourgade, S., de la Fouchardière, C., Bennouna, J., Bachet, J.-B., Khemissa-Akouz, F., Péré-Vergé, D., Delbaldo, C., Assenat, E., Chauffert, B., Michel, P., Montoto-Grillot, C., & Ducreux, M. (2011). FOLFIRINOX versus gemcitabine for metastatic pancreatic cancer. New England Journal of Medicine, 364(19), 1817–1825. https://doi.org/10.1056/NEJMoa1011923
- Dra, L. A., Aghraz, A., Boualy, B., Oubaassine, S., Barakate, M., Markouk, M., & Larhsini, M. (2019). Chemical characterization and in vitro antimicrobial activity of Caralluma europaea essential oil and its synergistic potential with conventional antibiotics. Journal of Advances in Medical and Pharmaceutical Sciences, 19(4), 1–11. https://doi.org/10.9734/jamps/2018/v19i430094
- Dra, L. A., Sellami, S., Rais, H., Aziz, F., Aghraz, A., Bekkouche, K., Markouk, M., & Larhsini, M. (2019). Antidiabetic potential of Caralluma europaea against alloxan-induced diabetes in mice. Saudi Journal of Biological Sciences, 26(6), 1171–1178. https://doi.org/10.1016/j.sjbs.2018.05.028
- Edderkaoui, M., Chheda, C., Soufi, B., Zayou, F., Hu, R. W., Ramanujan, V. K., Pan, X., Boros, L. G., Tajbakhsh, J., Madhav, A., Bhowmick, N. A., Wang, Q., Lewis, M., Tuli, R., Habtezion, A., Murali, R., & Pandol, S. J. (2018). An inhibitor of GSK3B and HDACs kills pancreatic cancer cells and slows pancreatic tumor growth and metastasis in mice. Gastroenterology, 155(6), 1985–1998.e5. https://doi.org/10.1053/j.gastro.2018.08.028
- Gawlik-Rzemieniewska, N., & Bednarek, I. (2016). The role of NANOG transcriptional factor in the development of malignant phenotype of cancer cells. Cancer Biology & Therapy, 17(1), 1–10. https://doi.org/10.1080/15384047.2015.1121348
- Glumac, P. M., & LeBeau, A. M. (2018). The role of CD133 in cancer: A concise review. Clinical and Translational Medicine, 7(1), 18. https://doi.org/10.1186/s40169-018-0198-1
- Goni, O., Khan, M. F., Rahman, M., Hasan, M., Kader, F. B., Sazzad, N., Sakib, M. A., Romano, B., Haque, M., & Capasso, R. (2021). Pharmacological insights on the antidepressant, anxiolytic and aphrodisiac potentials of Aglaonema hookerianum Schott. Journal of Ethnopharmacology, 268, 113664. https://doi.org/10.1016/j.jep.2020.113664
- Goodarzi, S., Tabatabaei, M. J., Mohammad Jafari, R., Shemirani, F., Tavakoli, S., Mofasseri, M., & Tofighi, Z. (2020). Cuminum cyminum fruits as source of luteolin- 7-O-glucoside, potent cytotoxic flavonoid against breast cancer cell lines. Natural Product Research, 34(11), 1602–1606. https://doi.org/10.1080/14786419.2018.1519824
- Hao, K., Tian, X. D., Qin, C. F., Xie, X. H., & Yang, Y. M. (2013). Hedgehog signaling pathway regulates human pancreatic cancer cell proliferation and metastasis. Oncology Reports, 29(3), 1124–1132. https://doi.org/10.3892/or.2012.2210
- Ho, C. T., Chen, Q., Shi, H., Zhang, K. Q., & Rosen, R. T. (1992). Antioxidative effect of polyphenol extract prepared from various Chinese teas. Preventive Medicine, 21(4), 520–525. https://doi.org/10.1016/0091-7435(92)90059-Q
- Hossain, S., Urbi, Z., Karuniawati, H., Mohiuddin, R. B., Moh Qrimida, A., Allzrag, A. M. M., Ming, L. C., Pagano, E., & Capasso, R. (2021). Andrographis paniculata (Burm. f.) Wall. ex nees: An updated review of phytochemistry, antimicrobial pharmacology, and clinical safety and efficacy. Life (Basel), 11(4), 348. https://doi.org/10.3390/life11040348
- Huang, W. W., Chiu, Y. J., Fan, M. J., Lu, H. F., Yeh, H. F., Li, K. H., Chen, P. Y., Chung, J. G., & Yang, J. S. (2010). Kaempferol induced apoptosis via endoplasmic reticulum stress and mitochondria-dependent pathway in human osteosarcoma U-2 OS cells. Molecular Nutrition & Food Research, 54(11), 1585–1595. https://doi.org/10.1002/mnfr.201000005
- Issiki, Z., Moundir, C., Marnissi, F., Seddik, N., Benjelloun, N., Zaid, Y., & Oudghiri, M. (2017). Toxicological evaluation of the aqueous extract of Caralluma europaea and its immunomodulatory and inflammatory activities. Pharmacognosy Research, 9(4), 390–395. https://doi.org/10.4103/pr.pr_24_17
- Janakiraman, K., Kathiresan, S., & Mariadoss, A. (2014). Influence of sinapic acid on induction of apoptosis in human laryngeal carcinoma cell line. International Journal of Modern Research and Reviews, 2, 165–170. https://www.semanticscholar.org/paper/INFLUENCE-OF-SINAPIC-ACID-ON-INDUCTION-OF-APOPTOSIS-Janakiraman-Kathiresan/06d808dc9211dc2592a97e4a79b938712ec1f44b
- Jiang, J., & Hui, C. (2008). Hedgehog signaling in development and cancer. Developmental Cell, 15(6), 801–812. https://doi.org/10.1016/j.devcel.2008.11.010
- Kashaw, S. K., Agarwal, S., Mishra, M., Sau, S., & Iyer, A. K. (2019). Molecular docking analysis of caspase-3 activators as potential anticancer agents. Current Computer-Aided Drug Design, 15(1), 55–66. https://doi.org/10.2174/1573409914666181015150731
- Kebbou, A., Laaradia, M. A., Oufquir, S., Aarab, A., Gabbas, Z. E., Rais, H., Zyad, A., & Chait, A. (2019). Antioxidant activity, anti-inflammatory and analgesic effects of Caralluma europaea (Eddaghmouss) in mice. OnLine Journal of Biological Sciences, 19(4), 272–285. https://doi.org/10.3844/ojbsci.2019.272.285
- Kregiel, D., Berlowska, J., Witonska, I., Antolak, H., Proestos, C., Babic, M., Babic, L., & Zhang, B. (2017). Saponin-Based, Biological-Active Surfactants from Plants. International Journal of Pharma and Bio Sciences, 4, B42–B48. https://doi.org/10.5772/68062
- Kumar, V., Chaudhary, A. K., Dong, Y., Zhong, H. A., Mondal, G., Lin, F., Kumar, V., & Mahato, R. I. (2017). Design, synthesis and biological evaluation of novel hedgehog inhibitors for treating pancreatic cancer. Scientific Reports, 7(1), 15. https://doi.org/10.1038/s41598-017-01942-7
- Li, Z., Zhang, Y., Chen, L., & Li, H. (2018). The dietary compound luteolin inhibits pancreatic cancer growth by targeting BCL-2. Food & Function, 9(5), 3018–3027. https://doi.org/10.1039/C8FO00033F
- Moni, J. N. R., Adnan, M., Tareq, A. M., Kabir, M. I., Reza, A., Nasrin, M. S., Chowdhury, K. H., Sayem, S. A. J., Rahman, M. A., Alam, A. K., Alam, S. B., Sakib, M. A., Oh, K. K., Cho, D. H., & Capasso, R. (2021). Therapeutic potentials of Syzygium fruticosum fruit (seed) reflected into an array of pharmacological assays and prospective receptors-mediated pathways. Life, 11(2), 155. https://doi.org/10.3390/life11020155
- Mousavi, N., Rahimi, S., Emami, H., Kazemi, A. H., Mohammad Taghi Kashi, R., & Heidarian, R. (2021). The effect of quercetin nanosuspension on prostate cancer cell line LNCaP via hedgehog signaling pathway. Reports of Biochemistry & Molecular Biology, 10(1), 69–75. https://doi.org/10.52547/rbmb.10.1.69
- National Research Council (US) Committee for the Update of the Guide for the Care and Use of Laboratory Animals. (2011). Guide for the care and use of laboratory animals, 8th ed, the national academies collection: Reports funded by national institutes of health. National Academies Press (US).
- Pérez-Soto, E., Estanislao-Gómez, C. C., Pérez-Ishiwara, D. G., Ramirez-Celis, C., & Gómez-García, M. d C. (2019). Cytotoxic effect and mechanisms from some plant-derived compounds in breast cancer. Cytotoxicity - definition, identification, and cytotoxic compounds. Erman Salih Istifli.
- Philip, P. A. (2008). Targeted therapies for pancreatic cancer. Gastrointestinal Cancer Research, 2(4), S16–S19. https://www.ncbi.nlm.nih.gov/pmc/articles/PMC2661543/
- Rahib, L., Smith, B. D., Aizenberg, R., Rosenzweig, A. B., Fleshman, J. M., & Matrisian, L. M. (2014). Projecting cancer incidence and deaths to 2030: The unexpected burden of thyroid, liver, and pancreas cancers in the United States. Cancer Research, 74(11), 2913–2921. https://doi.org/10.1158/0008-5472.CAN-14-0155
- Ramadan, A., Soliman, G., Mahmoud, S. S., Nofal, S. M., & Abdel-Rahman, R. F. (2012). Evaluation of the safety and antioxidant activities of Crocus sativus and Propolis ethanolic extracts. Journal of Saudi Chemical Society, 16(1), 13–21. https://doi.org/10.1016/j.jscs.2010.10.012
- Reyes-Farias, M., & Carrasco-Pozo, C. (2019). The anti-cancer effect of quercetin: Molecular implications in cancer metabolism. International Journal of Molecular Sciences, 20(13), 3177. https://doi.org/10.3390/ijms20133177
- Rhallab, A., Chakir, S., Elbadaoui, K., Alaoui, & T., lmolek. (2014). Evaluation of acute and subacute toxicity of aqueous extracts from Marrubium vulgare L in rodents. Journal of Pharmacy Research, 8, 684–688. http://eprints.umi.ac.ma/id/eprint/1031.
- Salama, Y. A., El-karef, A., El Gayyar, A. M., & Abdel-Rahman, N. (2019). Beyond its antioxidant properties: Quercetin targets multiple signalling pathways in hepatocellular carcinoma in rats. Life Sciences, 236, 116933. https://doi.org/10.1016/j.lfs.2019.116933
- Siegel, R., Ma, J., Zou, Z., & Jemal, A. (2014). Cancer statistics, 2014. CA: A Cancer Journal for Clinicians, 64(1), 9–29. https://doi.org/10.3322/caac.21208
- Siegel, R. L., Miller, K. D., & Jemal, A. (2019). Cancer statistics, 2019. CA: A Cancer Journal for Clinicians, 69(1), 7–34. https://doi.org/10.3322/caac.21551
- Slee, E. A., Adrain, C., & Martin, S. J. (2001). Executioner caspase-3, -6, and -7 perform distinct, non-redundant roles during the demolition phase of apoptosis*. The Journal of Biological Chemistry, 276(10), 7320–7326. https://doi.org/10.1074/jbc.M008363200
- Sohal, D. P. S., Mangu, P. B., Khorana, A. A., Shah, M. A., Philip, P. A., O'Reilly, E. M., Uronis, H. E., Ramanathan, R. K., Crane, C. H., Engebretson, A., Ruggiero, J. T., Copur, M. S., Lau, M., Urba, S., & Laheru, D. (2016). Metastatic pancreatic cancer: American society of clinical oncology clinical practice guideline. Journal of Clinical Oncology, 34(23), 2784–2796. https://doi.org/10.1200/JCO.2016.67.1412
- Su, D., Liao, Z., Feng, B., Wang, T., shan, B., Zeng, Q., Song, J., & Song, Y. (2020). Pulsatilla Chinensis saponins cause liver injury through interfering ceramide/sphingomyelin balance that promotes lipid metabolism dysregulation and apoptosis. Phytomedicine: International Journal of Phytotherapy and Phytopharmacology, 76, 153265. https://doi.org/10.1016/j.phymed.2020.153265
- Velmurugan, B. K., Lin, J. T., Mahalakshmi, B., Chuang, Y. C., Lin, C. C., Lo, Y. S., Hsieh, M. J., & Chen, M. K. (2020). Luteolin-7-O-glucoside inhibits oral cancer cell migration and invasion by regulating matrix metalloproteinase-2 expression and extracellular signal-regulated kinase pathway. Biomolecules, 10(4), 502. https://doi.org/10.3390/biom10040502
- Villodre, E. S., Kipper, F. C., Pereira, M. B., & Lenz, G. (2016). Roles of OCT4 in tumorigenesis, cancer therapy resistance and prognosis. Cancer Treatment Reviews, 51, 1–9. https://doi.org/10.1016/j.ctrv.2016.10.003
- Volk-Draper, L., Hall, K., Griggs, C., Rajput, S., Kohio, P., DeNardo, D., & Ran, S. (2014). Paclitaxel therapy promotes breast cancer metastasis in a TLR4-dependent manner. Cancer Research, 74(19), 5421–5434. https://doi.org/10.1158/0008-5472.CAN-14-0067
- Walsh, J. G., Cullen, S. P., Sheridan, C., Lüthi, A. U., Gerner, C., & Martin, S. J. (2008). Executioner caspase-3 and caspase-7 are functionally distinct proteases. Proceedings of the National Academy of Sciences, 105(35), 12815–12819. https://doi.org/10.1073/pnas.0707715105
- Wang, T., Gong, X., Jiang, R., Li, H., Du, W., & Kuang, G. (2016). Ferulic acid inhibits proliferation and promotes apoptosis via blockage of PI3K/Akt pathway in osteosarcoma cell. American Journal of Translational Research, 8(2), 968–980. https://www.ncbi.nlm.nih.gov/pmc/articles/PMC4846940/
- Weina, K., & Utikal, J. (2014). SOX2 and cancer: Current research and its implications in the clinic. Clinical and Translational Medicine, 3, 19. https://doi.org/10.1186/2001-1326-3-19
- Yang, M., Wu, Z., Wang, Y., Kai, G., Singor Njateng, G. S., Cai, S., Cao, J., & Cheng, G. (2019). Acute and subacute toxicity evaluation of ethanol extract from aerial parts of Epigynum auritum in mice. Food and Chemical Toxicology, 131, 110534. https://doi.org/10.1016/j.fct.2019.05.042
- Zhang, J., Wu, D., Song, J., Wang, J., Yi, J., & Dong, W. (2015). Hesperetin induces the apoptosis of gastric cancer cells via activating mitochondrial pathway by increasing reactive oxygen species. Digestive Diseases and Sciences, 60(10), 2985–2995. https://doi.org/10.1007/s10620-015-3696-7