ABSTRACT
Current hypertension guidelines fail to provide a recommendation on when-to-treat, thus disregarding relevant circadian rhythms that regulate blood pressure (BP) level and 24 h patterning and medication pharmacokinetics and pharmacodynamics. The ideal purpose of ingestion-time (chronopharmacology, i.e. biological rhythm-dependent effects on the kinetics and dynamics of medications, and chronotherapy, i.e. the timing of pharmaceutical and other treatments to optimize efficacy and safety) trials should be to explore the potential impact of endogenous circadian rhythms on the effects of medications. Such investigations and outcome trials mandate adherence to the basic standards of human chronobiology research. In-depth review of the more than 150 human hypertension pharmacology and therapeutic trials published since 1974 that address the differential impact of upon-waking/morning versus at-bedtime/evening schedule of treatment reveals diverse protocols of sometimes suboptimal or defective design and conduct. Many have been “time-of-day,” i.e. morning versus evening, rather than circadian-time-based, and some relied on wake-time office BP rather than around-the-clock ambulatory BP measurements (ABPM). Additionally, most past studies have been of too small sample size and thus statistically underpowered. As of yet, there has been no consensual agreement on the proper design, methods and conduct of such trials. This Position Statement recommends ingestion-time hypertension trials to follow minimum guidelines: (i) Recruitment of participants should be restricted to hypertensive individuals diagnosed according to ABPM diagnostic thresholds and of a comparable activity/sleep routine. (ii) Tested treatment-times should be selected according to internal biological time, expressed by the awakening and bed times of the sleep/wake cycle. (iii) ABPM should be the primary or sole method of BP assessment. (iv) The minimum-required features for analysis of the ABPM-determined 24 h BP pattern ought to be the asleep (not “nighttime”) BP mean and sleep-time relative BP decline, calculated in reference to the activity/rest cycle per individual. (v) ABPM-obtained BP means should be derived by the so-called adjusted calculation procedure, not by inaccurate arithmetic averages. (vi) ABPM should be performed with validated and calibrated devices at least hourly throughout two or more consecutive 24 h periods (48 h in total) to achieve the highest reproducibility of mean wake-time, sleep-time and 48 h BP values plus the reliable classification of dipping status. (vii) Calculation of minimum required sample size in adherence with proper statistical methods must be provided. (viii) Hypertension chronopharmacology and chronotherapy trials should preferably be randomized double-blind, randomized open-label with blinded-endpoint, or crossover in design, the latter with sufficient washout period between tested treatment-time regimens.
Introduction
Position statement: rationale and purpose
More than 150 published human trials describe the differential effects on blood pressure (BP) control, biomarkers of elevated BP-induced injury to blood vessels, heart, and kidney tissue, and cardiovascular disease (CVD) morbidity and mortality exerted by prescription single and combination hypertension medications when ingested in the morning or upon-waking versus evening or at bedtime (Bowles et al. Citation2018; De Giorgi et al. Citation2013; Hermida et al. Citation2016, Citation2020b, Citation2020c; Liu et al. Citation2014; Schillaci et al. Citation2015; Stranges et al. Citation2015; Sun et al. Citation2016; Zhao et al. Citation2011). In-depth review of these – some conceptualized as “time-of-day” rather than appropriate circadian-time – published trials reveal diverse and often suboptimal protocols. This is exemplified by discrepancies in the selection of times of treatment, manner of BP measurement – office versus ambulatory monitoring – and choice of BP endpoints sensitive to hypertension-associated end organ injury and cardiac and vascular morbidity and mortality outcomes. As a consequence of such differences and, sometimes, suboptimal methodology, the results and conclusions of various studies are inconsistent, even when involving the same specific medication. The ideal motivation for performing human ingestion-time hypertension therapy trials should be an exploration of the potential impact of endogenous circadian rhythms on the behavior and effects of BP-lowering medications to improve efficacy and limit side effectsFootnote1; however, protocols of these trials mandate proper adherence to the standards and guidelines established by the field of medical chronobiology (defined as the study of biological rhythms and medicine) for design and conduct of this type of research.
The field of medical chronobiology comprises clinical chronopharmacology (i.e. the influence of treatment-time relative to biological rhythm stage on the pharmacokinetics [PK] and pharmacodynamics [PD] of medications) and chronotherapeutics (i.e. the timing of medications [or other forms of treatment] to biological rhythms to optimize beneficial and/or minimize/avert adverse effects). Interest in clinical chronopharmacology and chronotherapeutics is growing rapidly, especially in regard to the management of hypertension to more effectively control BP to better diminish CVD risk (Bowles et al. Citation2018; De Giorgi et al. Citation2013; Gupta et al. Citation2020; Hermida et al. Citation2019a, Citation2016; Roush et al. Citation2014b; Schillaci et al. Citation2015). Most notably, hypertension chronotherapy that specifically targets the asleep systolic (SBP) mean and dipper pattern, as specific features of the BP 24 h profile, has been found to: (i) substantially improve control of elevated ambulatory BP (ABP), (ii) avert injury to blood vessels, tissues and organs at highest vulnerability – brain, heart, kidney and retina – and (iii) of upmost clinical importance, reduce the risk for nonfatal and fatal CVD events (Gupta et al. Citation2020; Hermida et al. Citation2019a; Roush et al. Citation2014b; Sobiczewski et al. Citation2014). These effects are verified by the: (i) meta-analyses concerning ingestion-time differences of hypertension medications on CVD risk conducted by Roush et al. (Citation2014b) and Gupta et al. (Citation2020); (ii) prospective hypertension chronotherapy study by Sobiczewski et al. (Citation2014) on high-risk patients with coronary heart disease followed for an average 6.6 years; plus (iii) MAPEC Study (Hermida et al. Citation2010) and much larger primary-care-based Hygia Chronotherapy Trial (Hermida et al. Citation2019a) that both entailed ABPM-diagnosed hypertensive patients. The conclusion of all such published evidence is consistent: ingestion of the complete daily dose of ≥1 conventional BP-lowering medication at bedtime, as opposed to the most common upon-awakening time, significantly improves asleep BP control and, most importantly, significantly reduces CVD morbidity and mortality, not only of adult patients of the general hypertension population (Hermida et al. Citation2010, Citation2019a), but those of greater vulnerability and enhanced CVD risk, including those having type 2 diabetes (Hermida et al. Citation2011b, Citation2019a), chronic kidney disease (CKD) (Hermida et al. Citation2011c, Citation2019a) and resistant hypertension (Ayala et al. Citation2013a; Hermida et al. Citation2019a).
Current hypertension guidelines fail to make a recommendation on the time of when-to-treat (Chiang et al. Citation2015; National Institute for Health and Clinical Excellence, Citation2019; Piper et al. Citation2015; Rabi et al. Citation2020; Umemura et al. Citation2019; Unger et al. Citation2020; Wang Citation2015; Whelton et al. Citation2018; Williams et al. Citation2018), despite the extensive information derived from past prospective, randomized, chronopharmacology and chronotherapy trials on the advantages and safety of the bedtime hypertension treatment strategy (Bowles et al. Citation2018; De Giorgi et al. Citation2013; Hermida et al. Citation2016, Citation2020b, Citation2020c; Liu et al. Citation2014; Schillaci et al. Citation2015; Stranges et al. Citation2015; Sun et al. Citation2016; Zhao et al. Citation2011). Thus, those hypertension guidelines disregard the relevance of circadian rhythms that: (i) regulate BP level and 24 h patterning as the basis for making the differential diagnosis of normotension versus hypertension and (ii) affect the PK (absorption, distribution, metabolism, and elimination) and PD (efficacy and safety) of hypertension medications as the basis for deciding the optimum time of when-to-treat.
The aims and goals of this Position Statement are to define the basic guidelines for the proper design, methods and conduct of hypertension chronopharmacology and chronotherapy trials. The circadian mechanisms that govern the 24 h BP patterning and administration-time differences in the efficacy and safety of prescription BP-lowering therapy are first addressed and, thereafter, recommendations, with justification, of the requirements for sound hypertension chronopharmacology and chronotherapeutic medication trials are detailed.
Endogenous circadian rhythms and exogenous 24 h cyclic factors affecting BP regulation
Current hypertension guidelines advocate primary reliance on wake-time office BP measurement (OBPM) as the ‘gold-standard’ to diagnose and manage arterial hypertension. In so doing, they assume the time during the 24 h when BP measurement is performed is clinically irrelevant. Most of these guidelines, nonetheless, now promote ABP monitoring (ABPM) of adult patients (≥18 years of age), if “economically and logistically feasible” (Williams et al. Citation2018) to confirm the traditional wake-time OBPM-based diagnosis of hypertension (Chiang et al. Citation2015; National Institute for Health and Clinical Excellence Citation2019; Piper et al. Citation2015; Rabi et al. Citation2020; Umemura et al. Citation2019; Unger et al. Citation2020; Wang Citation2015; Whelton et al. Citation2018; Williams et al. Citation2018), a recommendation founded on the well-documented significantly better value of ABPM than wake-time OBPM in prognosticating CVD risk (Clement et al. Citation2003; Dolan et al. Citation2005; Eguchi et al. Citation2008; Hermida et al. Citation2011a, Citation2020a, Citation2018, Citation2020d, Citation2015; Minutolo et al. Citation2011; Roush et al. Citation2014a; Salles et al. Citation2008; Verdecchia et al. Citation1994).
The majority of these same guidelines, however, propose ABPM be done either solely during the usual activity span or around-the-clock for only a single day/night span to derive, respectively, just the “daytime” (National Institute for Health and Clinical Excellence Citation2019; Whelton et al. Citation2018) or 24 h SBP and diastolic BP (DBP) means (Chiang et al. Citation2015; Rabi et al. Citation2020; Umemura et al. Citation2019; Wang Citation2015) to make the diagnosis of hypertension. This simplistic approach is unsatisfactory and leads to improper diagnoses, because it disregards the much more clinically relevant features of the mostly predictable BP 24 h pattern (Hermida et al. Citation2015, Citation2013e). The activity/rest synchronized BP temporal variation results from the interrelationship of (i) multiple external environmental 24 h cyclic factors and (ii) endogenous circadian rhythm determinants, as summarized in (Fabbian et al. Citation2013; Hermida et al. Citation2007; Portaluppi et al. Citation2012; Smolensky et al. Citation2007, Citation2017, Citation2016, Citation2015). The former comprise activity/rest cycle-associated temporal patterns of behavior-related factors, such as posture; physical activity level; emotional and mental stress; meal/diet and fluid (including stimulant, e.g. caffeine) consumption; and exposure to ambient thermal, noise and light (intensity and spectrum) conditions. The latter comprise endogenous circadian rhythms of the neuroendocrine, endothelial, vasoactive peptide and hemodynamic parameters, such as plasma noradrenaline and adrenaline, autonomic nervous system (ANS) sympathetic/parasympathetic balance, nitric oxide, atrial natriuretic and calcitonin gene-related peptides and components – prorenin, renin, angiotensin-converting enzyme (ACE), angiotensin I and II, and aldosterone concentration – of the renin-angiotensin-aldosterone system (RAAS) (Angeli et al. Citation1992; Fabbian et al. Citation2013; Hermida et al. Citation2007; Portaluppi et al. Citation2012; Smolensky et al. Citation2007, Citation2017, Citation2016, Citation2015; Sothern et al. Citation1995).
Table 1. Contribution of exogenous environmental 24 h cycles and innate circadian rhythms to the typical 24 h BP pattern of most normotensive and uncomplicated hypertensive persons
Endogenous circadian rhythms and exogenous 24 h cyclic factors influencing the PK of hypertension medications
The PK of ingested BP-lowering medications is significantly affected not only by 24 h cycle of feeding behavior but circadian rhythms of: (i) gastric pH and emptying rate, gastro-intestinal motility and tissue blood perfusion, passive and active transport phenomena, and biliary and pancreatic processes that influence their absorption; (ii) red and white blood cell count, plasma and tissue protein, passive and active cell membrane transport, and tissue blood perfusion that influence their distribution; and (iii) hepatic and renal blood perfusion, the activity of multiple hepatic enzymes and biochemical pathways, plus glomerular filtration and other renal tubular phenomena that influence their metabolism and elimination (Baraldo Citation2008; Bélanger et al. Citation1997; Bruguerolle Citation1998; Labrecque and Beauchamp Citation2003; Lemmer Citation1996; Reinberg and Smolensky Citation1982). Dosing-time-dependent difference in the PK of medications can also be affected by their chemical nature, e.g. the absorption rate of lipophilic, but not hydrophilic, medications have been reported to differ according to ingestion-time (Shiga et al. Citation1993).
Endogenous circadian rhythms and exogenous 24 h cyclic factors influencing the PD of hypertension medications
Statistically and clinically significant ingestion-time (with reference to the circadian staging of biological rhythms) differences in the PD, both in regards to the efficacy and safety of hypertension medications, are clearly evident by observed disparities in the therapeutic modulation of the BP 24 h pattern – especially those features identified with highest associated CVD risk, namely the asleep BP mean and sleep-time relative BP decline – and occurrence of adverse effects (Bowles et al. Citation2018; De Giorgi et al. Citation2013; Hermida et al. Citation2016, Citation2020b, Citation2020c; Liu et al. Citation2014; Schillaci et al. Citation2015; Stranges et al. Citation2015; Sun et al. Citation2016; Zhao et al. Citation2011). Such treatment-time-dependent (properly conceptualized as circadian rhythm-time-dependent) differences in the PD of hypertension medications result not only from those circadian rhythms that influence PK but also from various other circadian rhythms that: (i) affect circulating free-fraction concentration, cell/tissue receptor number/conformation and second messengers/signaling pathways of drug-targeted sites, such as blood vessels, heart and renal tissue plus (ii) comprise mechanisms of the 24 h BP pattern, especially the ANS and RAAS (Angeli et al. Citation1992; Fabbian et al. Citation2013; Hermida et al. Citation2007; Portaluppi et al. Citation2012; Smolensky et al. Citation2007, Citation2017; Sothern et al. Citation1995). Thus, it is important to note that due to the circadian organization of biological processes at all hierarchal levels, ingestion-time differences in the therapeutic and adverse effects of hypertension medications need not, as commonly assumed, be solely dependent upon ingestion-time disparities in PK, i.e. absorption, distribution and metabolism/elimination. Although the PK of medication may be the same no matter the time of day when ingested, the PD can differ significantly. This is because the timing of peak and trough blood concentrations of single or combination hypertension medications relative to the staging of the targeted circadian rhythms involved in the regulation of the 24 h BP pattern can be more strongly deterministic of the beneficial and adverse effects than the PK, alone (Bowles et al. Citation2018; De Giorgi et al. Citation2013; Hermida et al. Citation2016, Citation2020b, Citation2020c; Reinberg and Smolensky Citation1982; Stranges et al. Citation2015).
Many recent clinical trials and meta-analyses (Bowles et al. Citation2018; De Giorgi et al. Citation2013; Hermida et al. Citation2016, Citation2020b, Citation2020c; Liu et al. Citation2014; Schillaci et al. Citation2015; Stranges et al. Citation2015; Sun et al. Citation2016; Zhao et al. Citation2011) have found conventional single and combination hypertension medications when ingested at bedtime rather than upon awakening from sleep results in an enhanced reduction of the asleep BP mean and increased sleep-time relative BP decline – the two most relevant BP-derived prognostic markers of CVD risk – and without the added hazard of adverse effects (De Giorgi et al. Citation2013; Hermida et al. Citation2016, Citation2020b, Citation2020c; Zhao et al. Citation2011). These ingestion-time differences in therapeutic benefits, as discussed above, derive from the circadian rhythm-dependent disparity in effects upon the PK and PD of BP-lowering medications that occur in association with the differential staging during the 24 h of neuroendocrine and other circadian rhythm-organized mechanisms of BP regulation (Fabbian et al. Citation2013; Hermida et al. Citation2007; Portaluppi et al. Citation2012; Smolensky et al. Citation2007, Citation2017). For example, the activity of the RAAS increases or peaks between the middle to late sleep span and decreases to the lowest level between the late wake/early sleep period (Angeli et al. Citation1992). Accordingly, ingestion at bedtime of angiotensin-II receptor blocker (ARB) and angiotensin-converting enzyme inhibitor (ACEI) medications – plus their tested combinations with calcium-channel blockers (CCB) and diuretics – results in drug concentration being highest during sleep, which coincides in time with the peak activity during the 24 h of the RAAS (Angeli et al. Citation1992; Portaluppi et al. Citation2012; Sothern et al. Citation1995). Consequently, this treatment strategy enhances reduction of the asleep BP mean – without compromised beneficial effect upon the awake BP because of the favorable PK of modern therapeutic systems – and it increases the sleep-time BP decline, thus converting the high CVD risk nondipper BP patterning toward normal without inducing sleep-time hypotension. The improved beneficial effects of the ARB, ACEI and their combination medications – regardless of their unique plasma terminal half-life – are documented by multiple properly designed and conducted high-quality chronotherapy trials (De Giorgi et al. Citation2013; Hermida et al. Citation2016, Citation2020b; Zhao et al. Citation2011).
Guidelines for the design and conduct of hypertension chronopharmacology and chronotherapy trials
A very large number (N > 150) of clinical studies published since 1976 have investigated potential ingestion-time differences in the effects of hypertension medications (Hermida et al. Citation2020b, Citation2020c). However, sometimes the results and conclusions of these past trials, even when evaluating the same specific medications, are conflicting, in most instances the consequence of dissimilar methods. Over the years, new knowledge and technology have helped identify the circadian rhythm in BP and its dipping pattern phenotypes, plus circadian mechanisms of BP regulation and ingestion-time hypertension medication chronopharmacology; collectively, this new information has provided fresh insight as to how to improve the design and conduct of future such trials. Nonetheless, there has yet to be sufficient discussion or consensual agreement as to the proper design, methods and conduct of chronopharmacology and chronotherapy trials that takes into account this new knowledge. A critical review of the differing methods of previous published studies identifies in a subset of them a number of common deficiencies and inconsistencies, which potentially affected outcomes in a negative manner, including:
Absence as a key inclusion criterion that participants have a comparable activity/sleep routine, confirmed by diary entries or other methods, such as wrist actigraphy.
Selection of treatment times according to either nonspecific “morning” and “evening” periods or arbitrary clock times rather than distinctive biological markers of the circadian stage, e.g. upon waking and at bedtime, that takes into account differences between individuals in the 24 h activity/sleep rhythm (Hermida et al. Citation2017), including those emanating from an advanced and delayed phase sleep disorder and circadian chronotypes of extreme morningness and eveningness (Curtis et al. Citation2019; Roenneberg et al. Citation2007; Zhang et al. Citation2019).
Reliance solely on wake-time OBPM or home BP measurement (HBPM) to certify subjects as arterial hypertensive to qualify them for participation in medication trials. Qualification of subjects in this manner is likely to be erroneous due to the high likelihood of including those with masked normotension (also termed white-coat hypertension or isolated-office hypertension), i.e. elevated wake-time OBPM but normal out-of-office BP by ABPM-based criteria, and exclusion of otherwise high-risk patients with masked hypertension, i.e. normal wake-time OBPM but elevated out-of-office BP by ABPM-based criteria (Hermida et al. Citation2012, Citation2020d, Citation2013e).
Dependence, as the outcome variable, upon wake-time OBPM or wake-time HBPM that does not enable evaluation of the effects of timed hypertension treatment on the most relevant characteristics of the BP 24 h pattern and level, i.e. the asleep SBP mean and sleep-time relative SBP decline, now identified as the strongest prognostic markers of CVD risk (Astrup et al. Citation2007; Ben-Dov et al. Citation2007; Boggia et al. Citation2007; Brotman et al. Citation2008; Dolan et al. Citation2005; Fagard et al. Citation2008; Fan et al. Citation2010; Hermida et al. Citation2011a, Citation2013c, Citation2020a, Citation2018, Citation2020d; Ingelsson et al. Citation2006; Minutolo et al. Citation2011; Nakano et al. Citation1998; Ohkubo et al. Citation2002; Roush et al. Citation2014a; Salles et al. Citation2016; Sturrock et al. Citation2000), and thus the highest priority therapeutic targets for CVD prevention (Hermida et al. Citation2011a, Citation2018, Citation2020d).
When ABPM is utilized, reliance solely on the 24 h or “daytime” or awake BP mean as the unique or major response variable, which masks the much more valuable clinical information conveyed by the specific features of the circadian BP pattern that are known to be most strongly associated with BP-targeted organ pathology and elevated CVD risk.
When ABPM is utilized, the use of arbitrarily selected clock hours to define the commencement and termination of “diurnal activity” and “nocturnal rest” that gives rise to misleading and biologically and clinically irrelevant externally referenced “daytime” and “nighttime” BP means, as opposed to the biological-time referenced, and thus most clinically meaningful, awake and asleep BP means, calculated using diary recordings or other methods, such as wrist actigraphy, of actual bed and wake times (Booth 3rd et al. Citation2016; Jones and Sinha Citation2011; Peixoto Filho et al. Citation1995).
When ABPM is utilized, inappropriate/suboptimal ABP measuring scheme, i.e. the interval between individual around-the-clock BP measurements being either too short or too long, and additionally the total duration of sampling being too short, ≤24 h.
Inaccurate calculation of the awake, asleep and 24 h BP means – and, therefore, also the sleep-time relative BP decline derived from the first two of these means – just as the simple arithmetic average of all ABPM-determined BP values.
Lack of required sample size calculation that leads to underpowered studies because of an insufficient number of recruited participants and as a consequence results in an inability to draw valid conclusions.
Based on the above-mentioned deficiencies, here we propose the specific criteria for the proper design and conduct of future hypertension chronopharmacology and chronotherapy trials, as outlined in , each subsequently discussed in detail.
Table 2. Recommended guidelines for the design and conduct of prospective hypertension chronopharmacology and chronotherapy trials
Inclusion criteria for patient recruitment
Diagnosis of hypertension
Reliance on wake-time OBPM and/or wake-time HBPM versus around-the-clock ABPM for the diagnosis of arterial hypertension might lead to discrepancies due to marked differences between BP values obtained by each method. Current guidelines (Chiang et al. Citation2015; National Institute for Health and Clinical Excellence Citation2019; Piper et al. Citation2015; Rabi et al. Citation2020; Umemura et al. Citation2019; Unger et al. Citation2020; Wang Citation2015; Whelton et al. Citation2018; Williams et al. Citation2018) define “normotension” and “sustained hypertension,” respectively, as in-clinic and out-of-clinic measured BP both being normal or elevated. Discrepancies between diagnostic conclusions of the two measures have been defined as either isolated-office hypertension (also termed white-coat hypertension) – although the more accurate and preferred term is masked normotension (Hermida et al. Citation2013e) – when the wake-time OBPM is elevated but the out-of-office BP is normal by ABPM-based criteria, or masked hypertension when the wake-time OBPM is normal but the out-of-office BP is elevated by ABPM-based criteria (Hermida et al. Citation2012, Citation2020d, Citation2013e). These two highly prevalent disparate diagnostic categories are associated with markedly different CVD risk (Coccina et al. Citation2020; Fagard and Cornelissen Citation2007; Hermida et al. Citation2012; Pierdomenico and Cuccurullo Citation2011). Reliance upon wake-time OBPM or wake-time HBPM, rather than around-the-clock ABPM, to certify subjects as hypertensive as inclusion criterion might be erroneous and leads to misleading findings due to the high likelihood of including >20% low CVD risk participants with masked normotension and excluding >27% otherwise high CVD risk participants with masked hypertension, mainly those with normal OBPM but elevated asleep BP mean and/or nondipper BP pattern (Hermida et al. Citation2018, Citation2020d). Furthermore, focusing recruitment on treated hypertensive individuals whose BP is already properly controlled according to ABPM-based diagnostic and therapeutic thresholds is also inappropriate and leads to misleading findings when evaluating ingestion-time-dependent effects of BP-lowering therapies. Beyond BP-lowering efficacy being markedly associated with pre-treatment BP level, diminishing with lower baseline BP, it is judged unethical to change the treatment regimen of any patient whose BP is already properly controlled according to guideline-recommended threshold values. Accordingly, certification of subjects as being arterial hypertensive or having treatment-controlled BP according to diagnostic thresholds of wake-time OBPM or wake-time HBPM as the main means of recruitment is unsound and should be avoided. Furthermore, several studies have reported gender differences in hypertension and CVD risks (Hermida et al. Citation2013d; Howard et al. Citation2019; Ji et al. Citation2020; Madsen et al. Citation2019), effects of BP-lowering medications (Stolarz et al. Citation2015) and adverse medication reactions (De Vries et al. Citation2019). Therefore, balance distribution by sex among recruited hypertensive persons and assessment of potential sex-dependent differences in effects are also recommended.
In summary, one fundamental criterion for inclusion of participants in hypertension chronopharmacology and chronotherapy trials should be that they have elevated BP and that it is uncontrolled according to ABPM-based diagnostic thresholds, whether or not under treatment with prescription medications. Reliance solely on wake-time OBPM or wake-time HBPM to certify subjects as arterial hypertensive and main or sole recruitment of properly controlled treatment patients according to diagnostic thresholds for hypertension is unsound and should be avoided.
Sleep/wake routine
The body’s circadian structure is primarily synchronized by one’s 24 h routine of activity in light and sleep in darkness. Disparities between individuals in their rest/activity cycle, which can be considerable because of inherited or lifestyle-determined chronotype of morningness and eveningness (Horne and Östberg Citation1976; Lemoine et al. Citation2013; Roenneberg et al. Citation2007; Zhang et al. Citation2019), are thus associated with sometimes appreciable differences in the staging of the circadian rhythms that are mechanistic of both 24 h BP variability and response to therapy (Fabbian et al. Citation2013; Hermida et al. Citation2007; Portaluppi et al. Citation2012; Smolensky et al. Citation2007, Citation2017). Moreover, rotating shift workers and night workers, who have atypical and variable sleep/wake routines, and persons subjected to recent and continuing transmeridian air travel and whose circadian system is likely to be differently staged or disrupted in comparison with those of a stable sleep/wake routine should be investigated in specifically designed trials and excluded from joint participation with persons adhering to a stable routine of daytime activity and nighttime sleep. Therefore, an additional indispensable criterion for qualifying subjects to participate in hypertension chronopharmacology and chronotherapy trials is adherence to a stable and mutually common activity/sleep routine as confirmed by diary entries or other methods, such as wrist actigraphy.
In summary, taking into account the multiple innate circadian rhythms that contribute to the 24 h BP pattern as well as PK and PD of BP-lowering medications, a key inclusion criterion for all recruited participants of hypertension chronopharmacology and chronotherapy trials is adherence to a stable and similar activity in light/sleep in darkness conventional routine that is confirmed by diary entries or other methods.
Selection of tested treatment times
As discussed in the previous sections of this Position Statement paper, the circadian structure is primarily synchronized by one’s 24 h routine of activity in light and sleep in darkness. Therefore, clock-time is not at all equivalent to biological-time. This fact is of fundamental relevance, not only to investigations entailing the chronopharmacology and chronotherapy of hypertension medications, but the application of their findings in the clinical setting to improve patient care (Hermida et al. Citation2015, Citation2013e). Accordingly, selection of treatment-times must be done according to internal biological time as expressed by features of the sleep/wake cycle of individuals, i.e. awakening and bed times, rather than according to biologically meaningless and nonspecific “morning” and “evening” spans or arbitrary clock hours. This recommendation is of essential importance.
In summary, hypertension chronopharmacology and chronotherapy protocols must not designate treatment times in terms of nonspecific “morning” and “evening” spans or arbitrary clock hours. Trials founded on external time-of-day criteria, rather than biomarkers of internal circadian time, are unacceptable, because of disrespect for one of the basic standards of quality biological rhythm research, thereby rendering findings potentially erroneous.
Primary BP measurement method
The diagnosis and management of arterial hypertension today are based almost exclusively on wake-time OBPM, sometimes complemented by wake-time HBPM as the preferred out-of-office BP assessment (Chiang et al. Citation2015; National Institute for Health and Clinical Excellence Citation2019; Piper et al. Citation2015; Rabi et al. Citation2020; Umemura et al. Citation2019; Unger et al. Citation2020; Wang Citation2015; Whelton et al. Citation2018; Williams et al. Citation2018). However, neither OBPM nor HBPM can reveal the clinically important features of the mostly predictable BP 24 h pattern that can be disclosed solely by comprehensive around-the-clock ABPM. In most diurnally active normotensive and uncomplicated hypertensive persons, BP is characterized by: (i) striking rise commencing ~1 h before the usual time of awakening from sleep; (ii) two wake-time time peaks – the major one ~2-3 h after awakening and the usually less prominent one ~10-12 h later; (iii) small mid-afternoon (post-prandial) wake-time nadir; and (iv) 10–20% decline of the sleep-time BP mean relative to the wake-time BP mean (Hermida et al. Citation2007; Smolensky et al. Citation2017). The usually greater awake than asleep BP, to large extent, derives from the high-amplitude circadian variation of sympathetic tone that peaks during the waking span, evidenced by highest activation of the ANS and concentrations then of plasma norepinephrine and epinephrine (Lakatua et al. Citation1986), plus elements of the RAAS – prorenin, renin, ACE, angiotensin I and II, and aldosterone – most of which increase in concentration between the middle to late-sleep span (Angeli et al. Citation1992; Kool et al. Citation1994; Sothern et al. Citation1995). The normally lower BP during sleep, relative to wakefulness, predominantly results from attenuation of behavioral and environmental factors in combination mainly with the decline of sympathetic and rise of vagal tone, the elevation of atrial natriuretic and calcitonin gene-related vasoactive peptides, and depression of the RAAS (Fabbian et al. Citation2013; Hermida et al. Citation2007; Portaluppi et al. Citation2012; Smolensky et al. Citation2007, Citation2017). The above described 24 h BP pattern is thought by many to be the most common one. However, with aging and decline in health, its features, particularly the asleep SBP mean and the sleep-time relative SBP decline (see Section 2.4.4 for a detailed discussion), may undergo significant alteration (Hermida et al. Citation2013a). Thus, >64% of individuals ≥60 years of age (Hermida et al. Citation2013a) and of those having a positive diagnosis of type 2 diabetes (Ayala et al. Citation2013b) or CKD (Mojón et al. Citation2013) are likely to exhibit an abnormally elevated asleep SBP mean and consequently nondipping 24 h profile, i.e. <10% sleep-time relative SBP decline, both indicative of elevated CVD risk and as such both worthy targets of hypertension therapy, as discussed in Section 2.4.2.
Only around-the-clock ABPM is capable of assessing the prognostic features of the 24 h BP variation that represents the totality of the exogenous cyclic and endogenous rhythmic influences. In this regard, multiple outcome trials consistently substantiate the correlation between BP parameters derived from around-the-clock ABPM and risk of CVD events and target organ injury and pathology – such as of the blood vessels, heart and kidneys – is much more robust than for wake-time OBPM (Clement et al. Citation2003; Dolan et al. Citation2005; Eguchi et al. Citation2008; Hermida et al. Citation2011a, Citation2018, Citation2020d, Citation2015; Minutolo et al. Citation2011; Roush et al. Citation2014a; Salles et al. Citation2008; Verdecchia et al. Citation1994). Therefore, ABPM is mandated to assess the prognostic features of the 24 h BP variation, particularly those of the asleep and awake SBP means and dipping pattern, which must be correctly calculated by specifically incorporating measurements made, respectively, during the actual sleep and wake spans of each involved person. This requires accurate knowledge of the bed and awakening times of each individual, as opposed to relying on presumed ones that generate “daytime” and “nighttime” BP means, which are time-of-day (not biological time) values that are likely to be poorly representative of the true awake and asleep BP means (Booth 3rd et al. Citation2016; Hermida et al. Citation2015, Citation2013e; Jones and Sinha Citation2011; Peixoto Filho et al. Citation1995). ABPM must be performed utilizing only previously validated (an updated list of validated ABPM devices can be found at http://dableducational.org/sphygmomanometers/devices_3_abpm.html#AbpmTable) and periodically calibrated devices (Hermida et al. Citation2013e; Williams et al. Citation2018). Moreover, contrary to popular opinion, ABPM, compared to traditional wake-time OBPM, is highly economical, as verified by a recent cost-effectiveness analysis of the database of the recruited population (N = 21,963) of the Hygia Project (Hermida et al. Citation2019a). The cost per 48 h ABPM study, preferred over the most common 24 h monitoring duration (see Section 2.5) to improve proper assessment of ABPM-based arterial hypertension and associated CVD risk (Hermida et al. Citation2013b), was found to be highly affordable, 4.38€ (~4.82US$). Moreover, the diagnosis and management of arterial hypertension by annual or more frequent periodic 48 h ABPM, versus traditional wake-time OBPM, reduced in a strikingly manner the financial outlay for elevated BP-associated pathology through both greatly improved detection and management of true arterial hypertension that resulted in markedly reduced CVD morbidity and mortality (Hermida et al. Citation2019a).
In summary, hypertension chronopharmacology and chronotherapy trials should rely on cost-effective around-the-clock ABPM as the primary method of BP measurement. ABPM must be performed utilizing previously validated and periodically calibrated devices (Hermida et al. Citation2013e; Williams et al. Citation2018). Trials based solely on wake-time OBPM and/or wake-time HBPM methods are incapable of assessing key features of the entire 24 h BP and, therefore, they are not recommended.
Primary ABP endpoints and ABPM requirements
Limitations of the 24 h ABP mean
The ordinarily used 24 h BP means to decide the diagnosis of arterial hypertension ignores the much more valuable and clinically relevant information conveyed by specific features of the circadian BP pattern. Individuals with the same 24 h BP mean – either above or below the diagnostic thresholds for the 24 h SBP/DBP mean, usually 130/80 mmHg (Chiang et al. Citation2015; Piper et al. Citation2015; Rabi et al. Citation2020; Umemura et al. Citation2019; Unger et al. Citation2020; Wang Citation2015; Williams et al. Citation2018) – are likely to display radically different 24 h BP patterns, ranging from extreme-dipper to riser phenotypes, thus constituting strikingly different CVD risk states (Astrup et al. Citation2007; Ben-Dov et al. Citation2007; Boggia et al. Citation2007; Brotman et al. Citation2008; Dolan et al. Citation2005; Fagard et al. Citation2008; Fan et al. Citation2010; Hermida et al. Citation2011a, Citation2013c, Citation2020a, Citation2018, Citation2020d; Ingelsson et al. Citation2006; Minutolo et al. Citation2011; Nakano et al. Citation1998; Ohkubo et al. Citation2002; Roush et al. Citation2014a; Salles et al. Citation2016; Sturrock et al. Citation2000).
The graphs of illustrate the major limitation of reliance upon the 24 h BP mean for diagnostic purpose and as a major endpoint of clinical hypertension chronopharmacology and chronotherapy trials. The graph in the upper-left-hand portion of presents for a normotensive man the 24 h SBP pattern, shown as the dashed thick line, with respect to the circadian time-specified tolerance limits of normal SBP, shown by the upper and lower continuous thin lines. The latter were calculated as a function of both sex and time during the rest-activity cycle (time expressed relative to hours after awakening from nighttime sleep) from the data of a reference population of normotensive individuals (Hermida et al. Citation2004). The shaded dark portion of the bar depicted on the lower horizontal axis designates the sleep span determined by wrist actigraphy for this individual. The graph shows SBP is within the upper and lower tolerance limits – normotensive range – throughout the entire 24 h, thereby corroborating the diagnosis of normotension, and which is inferred as well by the 24 h SBP mean being 124.5 mmHg – below the 130 mmHg threshold recommended for the diagnosis of arterial hypertension by current guidelines (Hermida et al. Citation2013e; Williams et al. Citation2018). The sleep-time relative SBP decline of 17% indicates he has a normal dipper BP pattern. The graph depicted in the upper-right-hand portion of presents in the same manner the SBP of a different man with an extreme-dipper BP pattern characterized by a sleep-time relative SBP decline of 24.3% caused by too-low sleep-time SBP, as depicted by the shadowed area below the lower limit of the tolerance intervals, but having the exact same 24 h SBP mean of 124.5 mmHg as the normal dipper individual whose BP profile is shown in the upper-left-hand graph of this figure. The graphs of the lower left- and right-hand portions of display the 24 h SBP profile of two additional men, both having the same 24 h SBP mean, once again of 124.5 mmHg. The first man has a nondipper profile with sleep-time relative BP decline of 5.4% and asleep SBP mean of 122.1 mmHg, thereby corroborating the diagnosis of arterial hypertension. The second man has a riser BP pattern, associated with the highest CVD risk among all the possible BP patterns, with sleep-time relative BP decline of −2.4% and asleep SBP mean of 126.8 mmHg, thereby once again corroborating the diagnosis of arterial hypertension. These illustrative four examples of indicate the identical 24 h “normal” BP mean, i.e. below the diagnostic threshold of arterial hypertension according to current guidelines, might indeed be associated with totally different circadian BP patterns and, thus, markedly different CVD risk and, moreover, actually different diagnostic status with the use of proper ABPM criteria.
Figure 1. 24 h SBP profile (dashed thick lines) of four male subjects, respectively, dipper (upper left), extreme-dipper (upper right), nondipper (lower left) and riser pattern (lower right), having the same 24 h SBP mean of 124.5 mmHg. The SBP profiles are plotted with respect to circadian time-specified tolerance limits for normal SBP (continuous thin lines), calculated from a reference population of normotensive individuals of comparable rest-activity cycle and male sex
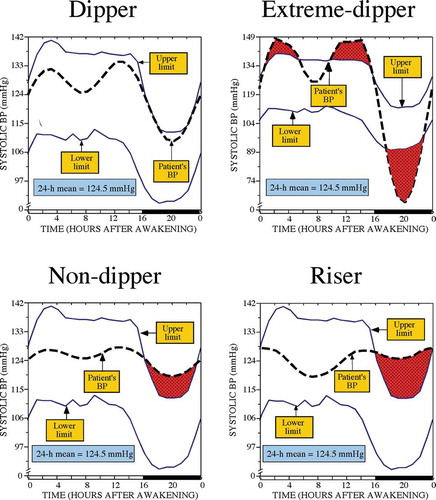
In summary, reliance on the 24 h BP mean – whether to diagnose arterial hypertension, evaluate response to therapeutic intervention, or assess CVD risk – is insufficient and suboptimal, both as a clinical and research procedure (Hermida et al. Citation2015, Citation2013e).
ABP sensitive prognostic markers of CVD risk
It is noteworthy that many independent prospective studies demonstrate CVD events are much better predicted by the asleep than the 24 h or awake ABP means (Ben-Dov et al. Citation2007; Boggia et al. Citation2007; Dolan et al. Citation2005; Fagard et al. Citation2008; Fan et al. Citation2010; Hermida et al. Citation2011a, Citation2013c, Citation2020a, Citation2018, Citation2020d; Minutolo et al. Citation2011). This is additionally substantiated by the published meta-analysis of original databases, rather than summary statistics, of 13,844 hypertensive patients (Roush et al. Citation2014a). Although the wake-time office SBP and ABPM-derived awake and asleep SBP means, when each was analyzed as a separate variable, were individually found to be significantly associated with elevated CVD risk, only the asleep SBP mean remained as an independent predictor of CVD events when all three SBP measures were simultaneously entered into the survival model (Roush et al. Citation2014a).
The differential importance of the multiple ABPM-derived parameters, in comparison with wake-time OBPM, as risk markers of CVD morbidity and mortality were verified by the large primary care-based multicenter Hygia Project (Hermida et al. Citation2018), a research network established in 2007 to incorporate ABPM as a routine procedure in primary-care medicine to diagnose and manage arterial hypertension, assess response to BP-lowering therapy and appraise patient CVD and other medical risks. Between 2008 and 2018, the participating primary-care physicians referred 21,963 persons for 48 h (rather than just 24 h for reasons explained subsequently, Section 2.5) ABPM annually, or more often in treated hypertensive participants above ABP therapeutic targets (respectively, 135/85 and 120/70 mmHg for awake and asleep SBP/DBP means [Hermida et al. Citation2013e; Williams et al. Citation2018]) and individuals having compelling clinical conditions of elevated CVD risk, such as diabetes, CKD and past CVD events. Corroborating and extending previously reported findings based on the data derived from the Hygia Project cohort of 18,078 individuals recruited up to 2015 (Hermida et al. Citation2018), more recent Cox proportional-hazard analyses on the enlarged sample of 21,963 persons that includes 3,885 additional participants recruited by primary-care physicians of the Hygia Project between 2015 and 2018, again substantiated the asleep SBP mean to be the most significant BP marker of CVD risk, independent of the absence or presence of hypertension therapy at baseline or treatment-time strategy (upon awakening versus at bedtime) during the several years of follow-up (Hermida et al. Citation2020d).
To further illustrate the relative clinical relevance of the asleep versus awake BP means on CVD risk, the enlarged Hygia Project study population (N = 21,963) was categorized into four mutually exclusive nonoverlapping cohorts according to patient ABP level, i.e. normal or elevated awake and normal or elevated asleep BP mean, according to the guidelines-recommended ABPM thresholds of 135/85 mmHg for the awake SBP/DBP means and 120/70 mmHg for the asleep SBP/DBP means (Hermida et al. Citation2013e; Williams et al. Citation2018). indicates: (i) similar adjusted hazard ratio (HR) for CVD outcome – CVD death, myocardial infarction, coronary revascularization, heart failure, ischemic stroke, hemorrhagic stroke, transient ischemic attack, angina pectoris, or peripheral artery disease – of participants with normal asleep BP mean, whether the awake BP mean is normal or elevated (P = .825); (ii) equivalent HR for hypertensive patients with elevated asleep BP mean, whether the awake BP mean is normal or elevated (P = .821) and (iii) significantly higher adjusted HR of CVD outcome for participants with elevated than normal asleep BP mean, independent of the awake BP mean being below or above 135/85 mmHg (always P < .001) (Hermida et al. Citation2020d).
Figure 2. Adjusted HR of CVD events in the Hygia Project cohort entailing 21,963 individuals. Participants were categorized into four nonoverlapping groups according to the level (normal or elevated) of the ABPM-derived awake and asleep SBP/DBP means. The ABPM-derived awake SBP/DBP means were considered normal if <135/85 mmHg and elevated otherwise. The asleep SBP/DBP means were considered normal if <120/70 mmHg and elevated otherwise. Adjustments were applied, if significant, for sex, age, diabetes, CKD and history of previous major CVD event
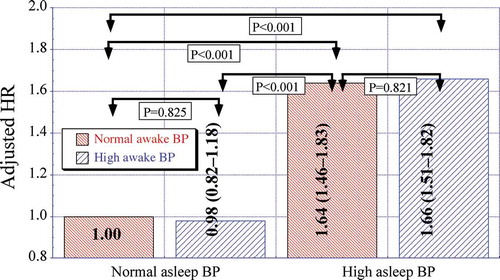
The relationship between attenuated sleep-time relative BP decline, i.e. nondipper/riser BP 24 h patterning, and risk for CVD events is additionally well documented, both for hypertensive (Dolan et al. Citation2005; Hermida et al. Citation2011a, Citation2018; Nakano et al. Citation1998; Ohkubo et al. Citation2002; Salles et al. Citation2016; Sturrock et al. Citation2000) and normotensive (Hermida et al. Citation2013c) persons. To illustrate the importance of the sleep-time relative SBP decline to CVD risk, the 21,963 participants of the Hygia Project, whose awake and asleep BP means make up , were further categorized into four mutually exclusive nonoverlapping groups according to the diagnostic 120/70 mmHg thresholds for the asleep SBP/DBP means (Hermida et al. Citation2013e; Williams et al. Citation2018) and also the arbitrary ≥10% (dipper BP pattern) or <10% (nondipper BP pattern) threshold for the sleep-time relative SBP decline. indicates: (i) significantly higher HR of CVD events for nondipper than dipper participants, independent of the asleep BP mean being normal or elevated and (ii) equivalent adjusted HR of CVD events for nondipper patients with normal asleep BP mean and dipper patients with elevated asleep BP mean (1.41 95%CI [1.19–1.67] and 1.50 [1.29–1.74], respectively; P = .210) (Hermida et al. Citation2020d). Collectively, these findings denote increased CVD risk is jointly associated with an elevated asleep BP mean – regardless of the wake-time OBPM and awake BP mean – and nondipper BP patterning, regardless of the asleep BP level, leading to the perspective provided by around-the-clock ABPM of a novel definition of true arterial hypertension based specifically on the asleep SBP mean and sleep-time relative SBP decline variables (Hermida et al. Citation2018, Citation2020d). Consistent with this perspective, prospective trials entailing at least annual patient ABPM evaluations – as per protocol in both the MAPEC Study (Monitorización Ambulatoria para Predicción de Eventos Cardiovasculares, i.e. Ambulatory Monitoring for Prediction of Cardiovascular Events) (Hermida et al. Citation2011a) and Hygia Project (Hermida et al. Citation2018) – document treatment-induced progressive attenuation of the asleep SBP mean and increase of the sleep-time relative SBP decline during the many years of follow-up are the most significant joint markers of lowered CVD risk, independent of changes in the wake-time OBPM or ABPM-obtained awake and 24 h SBP means (Hermida et al. Citation2011a, Citation2018).
Figure 3. Adjusted HR of CVD events in the Hygia Project cohort entailing 21,963 individuals. Participants were categorized into four nonoverlapping groups according to the level (normal or elevated) of the ABPM-derived asleep SBP/DBP means and extent of the sleep-time relative SBP decline. The ABPM-derived asleep SBP/DBP means were considered normal if <120/70 mmHg and elevated otherwise. Participants were designated as dipper when the sleep-time-relative SBP decline was ≥10% and as nondippers when <10%, using data sampled at 20–30 min intervals by ABPM for 48 consecutive hours. Adjustments were applied, if significant, for sex, age, diabetes, CKD and history of previous major CVD event
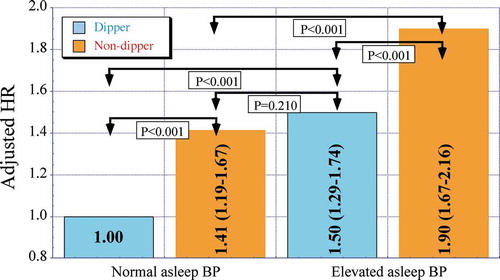
In summary, the asleep SBP mean, but not the wake-time OBPM or awake SBP mean, and the sleep-time relative SBP decline constitute sensitive prognostic markers of CVD risk and thus novel therapeutic targets for meaningful advances in CVD prevention and prolongation of patient CVD event-free survival (Hermida et al. Citation2011a, Citation2018), thereby warranting them as primary ABP endpoints in hypertension chronopharmacology and chronotherapy trials. Beyond the asleep (not “nighttime”) BP mean and sleep-time relative SBP decline – both calculated utilizing data obtained by around-the-clock ABPM and specifically in terms of the individualized activity/rest cycle of each person – hypertension chronopharmacology and chronotherapy trials might also incorporate additional biomarkers indicative of improvement in target organ pathology, such as the kidney – reduced albuminuria and increased estimated glomerular filtration rate – and heart – decreased left ventricular posterior diameter and left ventricular mass.
Requirements for proper assessment of ABP prognostic parameters
The collective results presented in previous sections of the Position Statement paper document the asleep SBP mean and sleep-time relative SBP decline (dipping) constitute the most significant and independent prognostic markers of CVD risk and, therefore, crucial critical clinical therapeutic targets for CVD prevention. Calculation of these ABPM-based parameters should be based only on the known bed and rise times of individual participants, rather than on the unrealistic assumption of a common activity/rest routine among all individuals that, from a clinical perspective, results in unrepresentative “daytime” and “nighttime” BP means (Hermida et al. Citation2017, Citation2020d, Citation2013e).
To illustrate the importance of this requirement, presents for a normotensive man the 24 h SBP pattern shown as the dashed thick line with respect to the circadian time-specified tolerance limits of normal SBP shown by the upper and lower continuous thin lines. The latter were calculated as a function of both sex and time during the rest-activity cycle (time expressed relative to hours after awakening from nighttime sleep) from the data of a reference population of normotensive individuals who on average slept 8.9 h and who on average retired to sleep at 23:58 h (Hermida et al. Citation2004). The individual assessed by ABPM slept for 9 h, from 21:00 to 06:00 h, as corroborated by wrist actigraphy. In keeping with the most common current approach for ABPM analysis, the graph depicted on the left-hand portion of displays the SBP data of this test subject expressed according to the clock time of each measurement. Results from this improper analysis of the BP data erroneously indicate (bottom table of ) the test subject is apparently “hypertensive,” with “nighttime” SBP mean >120 mmHg, and “nondipper,” i.e. sleep-time relative SBP decline of 3.5%. The graph on the right-hand portion of displays the exact same BP data of this test subject after being re-expressed using his wrist actigraphy data, in terms of hours from his actual bed time (21:00 h); the reference tolerance limits did not change, since the zero time (bedtime) was coincident with midnight. Analysis of the re-expressed/synchronized data in reference to the man’s specific sleep/wake routine correctly indicates he is normotensive and a normal dipper, with the correctly calculated asleep SBP mean and sleep-time relative SBP decline both being normal, respectively, 110.8 mmHg and 13.4%.
Figure 4. 24 h SBP profile (dashed thick lines) of a normotensive dipper man plotted with respect to circadian time-specified tolerance limits for normal SBP (continuous thin lines) calculated from a reference population of normotensive individuals of comparable rest-activity cycle and male sex. The same ABP data are represented as a function of clock time (left) and biological time, i.e. hours from bedtime (right)
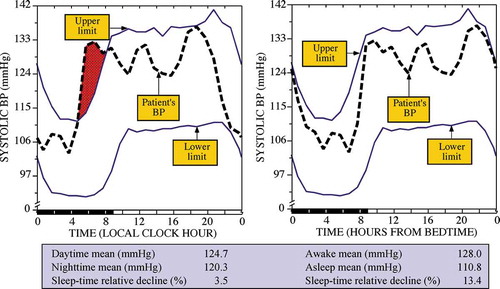
The critical importance of expressing ABPM data according to the individual sleep-wake 24 h routine, rather than assumed or common ones across all participants, is further substantiated by the results of a large epidemiology study of human sleep entailing 35,475 adults, 18–101 years of age, of 10 different countries of Europe, Asia and North America (Ohayon Citation2011). The findings reveal great disparity among people in the clock times of awakening from and retiring to nighttime sleep. Although the median time of awakening was 07:00 h, the clock times of such among the individuals ranged from 02:30 h to 12:30 h. Similarly, although the median time of bedtime was 23:00 h, the clock times of such among the individuals ranged from 18:00 h to 05:30 h, thereby also substantiating marked individual differences in sleep duration (Ohayon Citation2011; Ohayon et al. Citation2004).
Practical demonstration of the requirement of calculating ABPM-derived parameters on the basis of known actual bed and wake times of individual patients is further provided by analyses of the sleep patterns of the 3,344 normotensive and hypertensive participants of the earlier conducted MAPEC Study (Hermida et al. Citation2011a). An actigraphy was worn on the dominant wrist while undergoing 48 h ABPM to record the level of physical activity at 1 min intervals from which the commencement and termination of the activity and sleep span of each participant was determined using dedicated software (Crespo et al. Citation2012, Citation2013) that enabled the accurate derivation of the SBP and DBP means of interest and additionally to confirm adherence to the investigative protocol requiring sleep duration being between 6 and 12 h (Hermida et al. Citation2011a). The right and left-hand graphs of , respectively, depict the distribution of the actigraphy-determined wake-up and bed times at the baseline ABPM evaluation of the 3,344 MAPEC Study participants. The median wake-up and bed times were, respectively, 08:00 h and 23:30 h. However, the between-individual variation of wake-up and bed times were great. For wake-up and bed times, the total range was, respectively, 03:00–13:00 h and 19:30–06:30 h. The left-hand portion of depicts the distribution of the sleep duration, restricted by protocol to be ≥6 h and ≤12 h, of the same MAPEC Study participants. Sleep duration varied appreciably between participants, with a mean of 8.89 h and a standard deviation of 1.16 h. Moreover, as shown in the right-hand graph of , sleep duration was significantly correlated with age (r = 0.251, P < .001), with sleep duration of this sample of clinical patients – including, among others, participants with previous CVD events, type 2 diabetes and CKD – being significantly longer for older (≥55 years of age) than younger individuals (respectively, 9.19 ± 1.14 h vs. 8.66 ± 1.11 h, P < .001).
Figure 5. Clock hour distribution of wake-up (left) and bed times (right) at the baseline ABPM evaluation of the MAPEC Study cohort entailing 3,344 individuals
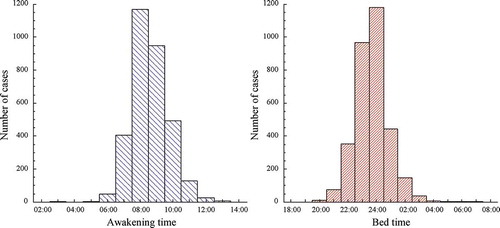
Figure 6. Left: Distribution of duration of sleep determined by wrist actigraphy at the baseline ABPM evaluation of the MAPEC Study cohort entailing 3,344 individuals. Right: Correlation between duration of sleep and age for the same MAPEC Study clinical cohort

In summary, the analysis and interpretation of ABPM data as most often done, i.e. according to the actual clock time of BP sampling, is inadequate and should be avoided, both in population studies, individual patient evaluations, and hypertension chronopharmacology and chronotherapy trials (Hermida et al. Citation2013e). Accordingly, as a minimum requirement, all individuals undergoing ABPM should maintain a diary to record the times of retiring to bed at night, awakening in the morning, consumption of meals, participation in exercise, and episodes of unusual physical activity, mood/emotional states and other atypical events that might affect BP (Hermida et al. Citation2013e). This individualized information serves many purposes. It is useful for deciding the quality of the ABPM assessment and editing of suspected unrepresentative measured BP values (as subsequently discussed in Section 2.4.5) when undergoing around-the-clock ABPM. Moreover, the individualized information pertaining to the commencement and termination of the activity and sleep spans is indispensable in order to accurately derive the awake and asleep BP means on a per person basis. Alternatively, objective determination of the bed and wake-up times during ABPM can be achieved by simultaneously wearing of an actigraph on the dominant wrist to record the level of physical activity during ABP measurement (Crespo et al. Citation2012, Citation2013).
Definition and assessment of ABP dipping phenotype
The sleep-time relative BP decline, index of BP dipping, is defined as the percent decrease in mean BP during the sleep span relative to mean BP during the activity span; it is calculated as: ([awake BP mean – asleep BP mean]/awake BP mean) × 100 (Hermida et al. Citation2013e). Accordingly, individuals have been arbitrarily classified as dippers or nondippers (sleep-time relative SBP decline ≥ or <10%, respectively). The classification has been extended by categorizing individuals into four groups: extreme-dippers (sleep-time relative SBP decline ≥20%), dippers (sleep-time relative SBP decline ≥10% but <20%), nondippers (sleep-time relative SBP decline <10%) and inverse-dippers or risers (sleep-time relative SBP decline <0%, indicating asleep BP > awake BP mean) (Hermida et al. Citation2013e). Correct calculation of the amount of BP dipping must be based on BP measurements made during the actual activity and sleep spans – not those made during arbitrarily assumed daytime and nighttime spans. Additionally, selection of the BP variable – SBP, DBP or mean arterial pressure (MAP) – utilized to calculate the sleep-time relative BP decline and thus categorize dipping is an extremely relevant issue. This is because dipping is an arbitrary classification defined in terms of a given percentage of the relative difference between the wake-time and sleep-time BP means, but the range of BP variability is markedly different for SBP, DBP and MAP. Thus, employing the same 10% arbitrary threshold to define dipping/nondipping for the SBP, DBP, or MAP is incorrect and leads to marked misclassification and, thus, misleading perspectives and erroneous findings (Hermida et al. Citation2013e).
To illustrate this point, presents the markedly different dipping categorization that results when the calculated sleep-time relative decline is based upon the ABPM-assessed SBP in comparison to DBP 24 h profiles of the same hypertensive individual. The upper continuous line with squares represents the 24 h SBP profile of a nondipper individual with an awake SBP mean of 140 mmHg and asleep SBP mean of 127 mmHg. Both of these mean values are above the corresponding 135 and 120 mmHg thresholds for the diagnosis of arterial hypertension (Hermida et al. Citation2013e; Williams et al. Citation2018). The difference between the awake and asleep SBP means is 13 mmHg. Finally, the sleep-time relative SBP decline is 9.3% (i.e. 100×[140–127]/140) indicating the individual has an elevated CVD risk nondipper SBP profile. The lower dashed line with circles represents the 24 h DBP profile of an apparently dipper individual with an awake DBP mean of 87 mmHg and asleep DBP mean of 74 mmHg. Both of these mean values are also above the corresponding 85 and 70 mmHg thresholds for the diagnosis of arterial hypertension (Hermida et al. Citation2013e; Williams et al. Citation2018). Again there is a 13 mmHg difference between the awake and asleep DBP means. However, the calculated sleep-time relative DBP decline is 14.9% (i.e. 100×[87–74]/87), thereby indicating that this same individual has a nonelevated CVD risk dipper DBP profile. The rather homogeneous pulse pressure (the difference between SBP and DBP) of 53 mmHg throughout the 24 h further indicates the SBP and DBP patterns of variation are almost identical, i.e. having exactly the same circadian amplitude and staging of the peak and trough BP values. The example illustrated in is the norm and not at all the exception; evaluation of data from the baseline ABPM assessment of the 21,963 participants of the Hygia Project indicates the average (±SD) sleep-time relative decline for SBP and DBP are, respectively, 9.8 ± 7.5% and 14.3 ± 8.3% (P < .001 between SBP and DBP), causing the corresponding prevalence of nondipping based on SBP versus DBP to differ greatly, respectively, 46.6% and 27.9% (P < .001 between SBP and DBP). This example demonstrates reliance on DBP, rather than SBP, results in the erroneous categorization of dipping status and in turn inaccurate determination of CVD risk status.
Figure 7. Difference of ~4.4% in the calculated sleep-time relative decline for SBP versus DBP of the same arterial hypertensive individual assessed by around-the-clock ABPM, demonstrating the differential and misleading sleep-time relative decline dipping classification when improperly based on DBP measurements (resulting in conclusion of dipper categorization) rather than when properly based on SBP measurements (resulting in conclusion in nondipper categorization)
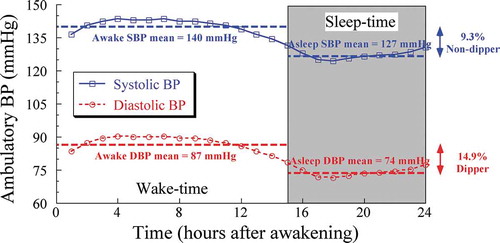
In summary, for any given individual, the sleep-time relative decline is, on average, ~4.5% larger for DBP than SBP. Accordingly, the sleep-time relative BP decline and corresponding categorization of individuals as dipper or nondipper – or, when using the extended classification, as extreme-dipper, dipper, nondipper or riser – should be based on ambulatory SBP alone. Dipping classification based on MAP, DBP or a combination of SBP and DBP is misleading and should be avoided.
Additional ABPM requirements
BP values assessed by ABPM require validation and editing before clinical use, as earlier recommended (Hermida et al. Citation2013e). As a general rule, SBP readings >250 or <70 mmHg, DBP >150 or <40 mmHg, and pulse pressure (the difference between SBP and DBP) >150 or <20 mmHg are beyond the range of usual physiologic values and, therefore, should be eliminated. Furthermore, ABPM measurements, according to diary entries, obtained during physical exercise, excessive movement, driving, unusual mood/emotional states and other confounding circumstances must be checked and edited for nonrepresentative values. Finally, ABPM data series must be considered invalid for analysis if diary entries reveal the rest-activity schedule was inconsistent during the two consecutive days of assessment (e.g. a difference of >2 h between times of waking or going to bed for the two days), the duration of the sleep span was <6 h or >12 h, if ≥30% of the scheduled BP measurements are missing from the time series, or BP data are lacking for a continuous interval of >2 h (Hermida et al. Citation2013e).
Furthermore, in keeping with current ABPM recommendations (Hermida et al. Citation2013e), participants in hypertension chronopharmacology and chronotherapy trials at the time of every clinic visit for ABPM evaluation must be specifically instructed to: (i) fit and properly adjust the BP cuff to the nondominant arm; (ii) preferably wear a thin layer of cotton clothing under the cuff to minimize risk for discomfort, skin reactions and bruising so as to enhance compliance (Thien et al. Citation2015); (iii) adhere to usual activities with minimal restrictions, i.e. maintain a similar activity-rest schedule and avoid daytime napping during the two consecutive days of evaluation; (iv) avoid activities that might interfere with operation of the ABPM device and representative measurement of BP values; (v) position the BP cuff at heart level, cease moving or talking, maintain the arm motionless and relaxed, and breathe normally when the cuff starts to inflate and until it fully deflates; (vi) switch the device off every time it is removed, even for brief periods of time, e.g. to shower/bathe, change clothes, etc., and switch it on again thereafter; (vii) keep the device on during sleep, and not to switch it off; and (viii) fill in all required entries in the provided daily diary (see Section 2.4.3) (Hermida et al. Citation2013e).
ABPM sampling rate and duration
The vast majority of hypertension guidelines (Chiang et al. Citation2015; Piper et al. Citation2015; Rabi et al. Citation2020; Umemura et al. Citation2019; Unger et al. Citation2020; Wang Citation2015; Whelton et al. Citation2018; Williams et al. Citation2018) recommend ABPM be performed just for a single 24 h or, according to one of them, an even shorter duration – wakefulness (National Institute for Health and Clinical Excellence Citation2019). These same guidelines additionally advocate ABPM measurements be performed at 20–30 min intervals based on the misleading statement – only applicable if the duration of sampling is not taken into consideration – that the greater the number of acquired BP readings, the more precise is the derivation of the average BP (Rabi et al. Citation2020). Perhaps, this is the rationale, albeit inappropriate, of many medical practitioners attempting to acquire BP measurements as frequently as feasible, at 10–15 min intervals, which is not only burdensome to patients and threatens compliance but results in discomfort and anxiety with risk for nonrepresentative elevated BP values (Hermida et al. Citation2013e). This recommendation of such a high sampling frequency is based on ABPM studies that were limited to <24 h (Agarwal and Tu Citation2018; Mancia et al. Citation1994). Thus, past evaluation of the impact of the interval of BP sampling on the reproducibility of ABPM-derived parameters was done without consideration of the known relevance of the duration of BP sampling. The critically important consideration of proper sampling interval in conjunction with proper duration of monitoring to achieve accuracy and reproducibility of the derived BP means and features of its 24 h patterning, and thus the validity of clinical decisions, have only been occasionally addressed (Bovha Hus and Kersnik Levart Citation2019; Hermida et al. Citation2013b; Hernández-del Rey et al. Citation2007; Mochizuki et al. Citation1998).
Studies summarized elsewhere (Hermida et al. Citation2013e) consistently substantiate the indisputable mathematical principle of signal processing that establishes reproducibility of BP characteristics, including mean BP values and sleep-time relative BP decline, as well as individualized CVD risk assessment, depend to a much greater extent on the duration than the frequency of BP measurements (Bovha Hus and Kersnik Levart Citation2019; Fernández et al. Citation2020; Hermida et al. Citation2013b). The practical demonstration of the requirements of sampling rate and duration of ABPM was first provided by analyses of the ABPM data of the 3,344 normotensive and hypertensive participants of the MAPEC Study (Hermida et al. Citation2011a). Their baseline ABPM profiles were modified to generate reconstructed time series either of: (i) identical 48 h duration but composed of data sampled at a larger sampling interval of every 1 h; or (ii) shorter duration of just the first 24 h span and of data sampled at the original rate of 20–30 min intervals (Hermida et al. Citation2013b). shows the limits of agreement for the asleep SBP mean – the most significant prognostic marker of CVD events (Ben-Dov et al. Citation2007; Boggia et al. Citation2007; Dolan et al. Citation2005; Fagard et al. Citation2008; Fan et al. Citation2010; Hermida et al. Citation2011a, Citation2013c, Citation2020a, Citation2018, Citation2020d; Minutolo et al. Citation2011; Roush et al. Citation2014a) – calculated for the original complete 48 h ABPM profiles of each participant evaluated upon recruitment versus the modified ABPM series, i.e. less-dense sampling of either one BP measurement per hour for 48 h (top) or the original sampling rate of one BP measurement per 20 to 30 min for the first 24 h span only (bottom). Each depicted Bland-Altman plot represents the difference between the asleep SBP mean calculated from the 48 h original series and that calculated from the modified series (vertical axis), plotted against the average of those two respective mean values (horizontal axis). The average of the individual differences in the asleep SBP mean was slightly, but, nonetheless, significantly greater for data sampled every 20–30 min for the restricted duration of 24 h than for the reconstructed time series that simulated data sampled every 1 h for 48 h (0.12 ± 4.4 vs. −0.16 ± 1.6 mmHg in asleep SBP mean; P < .001). Of more direct clinical relevance, further illustrates the asleep SBP mean values obtained from the original complete 48 h ABPM profiles were much better reproduced with data sampled every 1 h for 48 h (time series composed of up to 48 of the maximum original 128 BP measurements/participant; , top) than with the data obtained at the original sampling rate of 20–30 min intervals during the first 24 h of assessment only (time series composed of up to 64 BP measurements/participant; , bottom). Specifically, reduction of ABPM duration to just 24 h resulted in a very significant error in determining the asleep SBP mean, in the range of −21.4 to +24.0 mmHg, i.e. 45.4 mmHg in total across the participants of the MAPEC Study. Such a great amount of error in determining the asleep SBP mean when ABPM duration is limited to just 24 h, which is typically the case, is neither acceptable for diagnostic and prognostic purposes nor for evaluating response to hypertension therapy (Hermida et al. Citation2013b).
Figure 8. Bland-Altman plots assessing agreement of the asleep SBP mean calculated utilizing the original data sampled by ABPM every 20–30 min for 48 consecutive hours versus that estimated from the (i) modified time series composed of data sampled every 1 h for 48 consecutive hours (top) and (ii) original sampling rate of every 20–30 min for the first 24 h, only (bottom). Dotted horizontal line of each graph represents the average of the differences across the entire studied population. Dashed lines represent the values of the average difference ±1.96SD (assumingly containing 95% of the individual values)
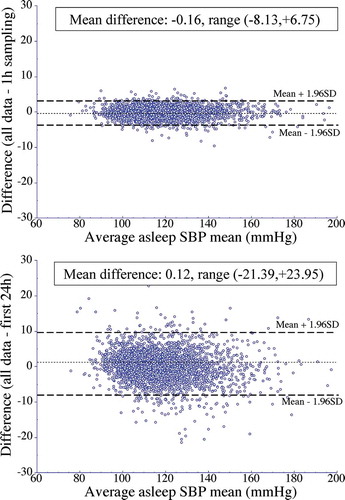
The finding is of great relevance, since the design of most reported administration-time (more appropriate, circadian rhythm-dependent ingestion-time) trials (Bowles et al. Citation2018; De Giorgi et al. Citation2013; Hermida et al. Citation2016, Citation2020b, Citation2020c; Liu et al. Citation2014; Schillaci et al. Citation2015; Stranges et al. Citation2015; Sun et al. Citation2016; Zhao et al. Citation2011) aimed to demonstrate as minimally clinically acceptable a significant difference between treatment-time groups of 3–4 mmHg in either the 24 h, awake and/or asleep BP means. This amount is 11- to 15-fold lower than the documented range of error in these mean BP values when derived from ABPM conducted for ≤24 h duration. Even more noteworthy, however, is the finding that the HR of CVD events in the MAPEC Study was markedly overestimated by SBP and underestimated by DBP when the duration of ABPM was reduced to only 24 h, rather than the recommended 48 h (Hermida et al. Citation2013b).
In summary, ABPM conducted for ≤24 h is highly unlikely to be sufficient to correctly calculate representative BP means or other ABPM-derived parameters of clinical relevance, confidently make the diagnosis of arterial hypertension, reliably identify dipping status, accurately evaluate treatment efficacy and, most important, properly stratify CVD risk (Hermida et al. Citation2013e).
Calculation of ABPM-derived mean values
Recommendation of the proper method to calculate mean BP values from data acquired by ABPM is a critical issue that has been ignored by all hypertension guidelines, except those jointly endorsed by the International Society for Chronobiology and the American Association of Medical Chronobiology and Chronotherapeutics (Hermida et al. Citation2013e). This is a crucial matter, since the awake, asleep and 24 h BP means – and, consequently, the sleep-time relative BP decline as a measure of BP dipping derived from the first two of these means – is typically calculated just as the simple arithmetic average of all ABPM-determined BP values per each of the respective spans. However, the arithmetic mean is highly dependent on the BP sampling rate – the interval of time between consecutive BP measurements. The usual, but nonetheless unsound, so-called unadjusted calculation approach that derives arithmetic means might result in overestimation of the true awake and 24 h BP means and, accordingly, mistaken computation of dipper phenotype. This is because BP measurements are typically obtained at a higher rate and for a longer duration of time during the usual ~16 h wake period than ~8 h sleep span. Moreover, even if the ABPM device is programmed to assess BP at equidistant intervals around-the-clock, commonly occurring measurement errors or failures render virtually all ABPM profiles being composed of nonequidistant interval data.
The so-called adjusted calculation approach is recommended to avoid the confounding effect of nonequidistant-in-time obtained ABPM measurements producing nonrepresentative values when deriving BP means based on the simple arithmetic average of BP measurements. This approach, as a first step, necessitates that the 24 h, awake and asleep spans each be divided into an integer number of time classes of identical length, e.g. as close as possible to 1 h intervals. In so doing, all BP measurements can be assigned, regardless of the total duration of ABPM, to a single idealized 24 h period according to the circadian time of sampling, e.g. expressed in terms of hours after awakening from sleep. As a second step, the approach necessitates the calculation of the mean BP for each of these time-class intervals. Thereafter, the respective 24 h, awake and asleep BP means are determined as the average value of all the corresponding individual time-class means (Frank et al. Citation2010; Hermida et al. Citation2013e). Comparison between individuals or populations of these more accurate and representative mean values would additionally require an estimator of BP variance that can be easily obtained following the approach described by Dixon and Massey (Citation1983). Differences between the erroneous arithmetic unadjusted calculation versus correct adjusted calculation procedure are appreciable. Using the ABPM data of the 21,963 participants of the Hygia Project to derive the 48 h, awake and asleep SBP means plus sleep-time relative SBP decline as arithmetic averages by the unadjusted calculation procedure, instead of recommended adjusted calculation procedure, would have resulted in a clinically unacceptable high range of error, respectively, [−6.8 to 11.2] mmHg for the 48 h SBP mean, [−8.1 to 7.3] mmHg for the awake SBP mean, [−8.9 to 9.3] mmHg for the asleep SBP mean, and [−9.1 to 8.2]% for the sleep-time relative SBP decline.
In summary, the so-called adjusted calculation approach is recommended and required to avoid the confounding effect of nonequidistant-in-time obtained ABPM measurements producing nonrepresentative BP means when incorrectly calculated as the simple arithmetic average of BP values.
Sample size calculation
The a priori calculation of the minimum required sample size is an essential requirement of prospective clinical investigations devised to assess treatment-time differences in the effects of BP-lowering medications (Julious Citation2004). Flight and Julious (Citation2016a) previously summarized specific considerations regarding the calculation of sample size. They include: (i) aim of the study, i.e. determination of superiority, noninferiority, or equivalence of BP features or outcomes; (ii) primary outcome endpoint, i.e. continuous, binary, ordinal, or time-to-event (survival); (iii) type I (probability of rejecting the null hypothesis when it is actually true) and type II errors (probability of not rejecting the null hypothesis when it is actually false); in sample size calculations, it is more common to work in terms of the power of a clinical trial, i.e. one minus the probability of a type II error, thus giving the probability of correctly rejecting the null hypothesis; (iv) measurement of variance, i.e. estimated or known population variability (usually expressed as standard deviation) for the primary endpoint; and (v) minimum clinically acceptable difference between comparisons for the selected primary endpoint. For example, for an estimated between-individuals standard deviation of 10 mmHg for the asleep SBP mean, at the two-sided level of 5% (type I error) and power of 80% (one minus the probability of a type II error), detection as statistically significant of a 5 mmHg difference in the reduction of the asleep SBP mean between those randomized to two parallel arms according to the ingestion time of hypertension treatment – e.g. either upon awakening or at bedtime – requires a minimum sample size of 64 individuals per treatment-time group, i.e. a total of 128 participants, raising to 172 persons if the pursued power is 90% (Flight and Julious Citation2016b). In actuality, this required number of subjects for the sample size is much larger than that recruited by investigators of several reported hypertension chronopharmacology and chronotherapy trials (Bowles et al. Citation2018; De Giorgi et al. Citation2013; Hermida et al. Citation2016, Citation2020b, Citation2020c; Liu et al. Citation2014; Schillaci et al. Citation2015; Stranges et al. Citation2015; Sun et al. Citation2016; Zhao et al. Citation2011).
In summary, properly designed hypertension chronopharmacology and chronotherapy trials must provide the calculation of the minimum required sample size in keeping with the aim of the study, type I and type II errors, the reliable variance for the primary endpoint and the minimum clinically acceptable difference between treatment-time groups for the primary endpoint.
Clinical trial design
A variety of study designs – cross-sectional; crossover (randomized or nonrandomized open-label, single-blind, or double-blind); nonrandomized open-label; prospective, randomized, open-label, blinded-endpoint (PROBE); randomized double-blind; and randomized open-label (absence of blinded endpoint) – have been utilized in the large number (N > 150) of previously reported hypertension chronopharmacology and chronotherapy trials. Cross-sectional designed studies, which are observational in nature and usually conducted on much larger cohorts than are randomized clinical trials, might provide insights on hypertension treatment-time differences in BP-lowering efficacy, the prevalence of BP control, safety and/or compliance. However, the findings of such designed trials are likely to be subject to bias and enable only inference of an association, but not causation (Sedgwick Citation2014). The crossover design, which is not an entirely ethically acceptable means of conducting prospective randomized trials, requires that all participants be transferred at the middle of the investigational span from one tested treatment-time regimen to a second different one. This approach is increasingly disapproved by medical ethics committees and institutional review boards, because it is judged unethical to change the treatment regimen of a therapeutic scheme that properly controls, according to guideline-recommended threshold values, the BP of any participant (Fernández et al. Citation2020). Moreover, as discussed earlier (Section of 2.1.1), inclusion in hypertension chronopharmacology and chronotherapy trials of hypertensive persons already properly controlled by treatment, according to ABPM criteria, is inadequate and with a high risk of producing potentially misleading results. The major assumptions of the crossover design of hypertension trials are: (i) prior to commencing the second treatment, BP and all of the other assessed variables return to the before-first treatment-time baseline condition and (ii) the order of the tested treatment times does not impact BP and other biomarkers of assessed therapeutic effects (Julious Citation2004). These two assumptions are rarely met, especially when the protocol fails to incorporate a minimum, usually 2-week, a washout period between treatment schemes.
In summary, although crossover randomized trials are scientifically valid when properly conducted, hypertension chronopharmacology and chronotherapy studies should preferably be of a randomized double-blind or PROBE design that entail the evaluation of parallel groups of individuals randomized to the different treatment-time arms.
Summary of recommendations
Consistent with the detailed discussion of the preceding sections of this Guidelines Position Statement paper, the Hypertension Subcommittee of the International Society for Chronobiology (ISC) and the American Association of Medical Chronobiology and Chronotherapeutics (AAMCC) recommend the design and conduct of clinical chronopharmacology and chronotherapy trials of hypertension medications be performed in adherence to the following minimum standards:
Criteria for recruitment of subjects should include they all have uncontrolled arterial hypertension according to ABPM-based diagnostic thresholds, and they have a comparable activity/sleep routine confirmed by diary entries or other methods, such as wrist actigraphy. Reliance solely on wake-time OBPM or wake-time HBPM to certify subjects as hypertensive, and, also, primary recruitment of properly controlled treated persons according to diagnostic thresholds for arterial hypertension is unsound and should be avoided.
Tested treatment-times must be selected only according to internal biological time, as expressed by specific features, i.e. awakening and bed times, of the sleep/wake cycle of individuals. Designation of treatment schemes as “morning” and “evening” or arbitrary chosen clock hour units are nonspecific for biological time and, therefore, meaningless by chronobiology standards, and must be avoided.
Hypertension chronopharmacology and chronotherapy trials should rely on around-the-clock ABPM as the sole primary method of BP measurement. Wake-time OBPM and/or wake-time HBPM is unable to determine the features of the 24 h BP pattern and, therefore, are not recommended to assess ingestion-time differences of treatment effects.
ABPM-based studies of treatment-time differences in the effects of BP-lowering medications should avoid the use of common clock hours or time spans to designate sleep and wake periods across all participants as the method to derive features of the 24 h BP pattern. Moreover, it is not recommended that ingestion-time trials of hypertension medications rely on the 24 h or daytime or awake BP means, alone. The minimum required primary ABP endpoints are the asleep (not “nighttime”) BP mean – properly calculated per features of the individualized activity/rest cycle of each patient – and BP dipping in terms of the sleep-time relative SBP decline – properly calculated from the actual awake and asleep SBP means. Additionally, hypertension chronopharmacology and chronotherapy trials might also incorporate as additional endpoints, for example, improvement in biomarkers of target organ pathology of the kidney – reduced albuminuria and increased estimated glomerular filtration rate – and heart – decreased left ventricular posterior diameter and left ventricular mass.
ABPM should be performed at least hourly for two consecutive 24 h periods (48 h in total) to achieve high reproducibility of the BP means, most reliable classification of persons according to dipping status, and greatest accuracy of prognosticating CVD risk.
Calculation of BP means as simple arithmetic averages, irrespectively of the ABPM sampling rate, as commonly done in most reported trials, is suboptimal because it leads to inaccurate and biased findings. ABPM-derived BP means should be obtained by the so-called adjusted calculation procedure.
Hypertension chronopharmacology and chronotherapy trials should provide a proper calculation of the minimum required sample size according to the specified design and aim of the study, type I and type II errors, reliably estimated or known variance for the primary endpoint, and the minimum clinically acceptable difference between treatment-time groups for the primary study endpoint.
The design of hypertension chronopharmacology and chronotherapy studies should preferably be in the form of a randomized double-blind, PROBE, or crossover double-blind approach, with a washout period of at least 2-week duration between tested treatment regimens. Crossover trials without an incorporated washout period and nonrandomized studies are not recommended and must be avoided.
These recommendations are summarized in as a guide to the proper design and conduct of prospective hypertension chronopharmacology and chronotherapy trials (Hermida et al. Citation2020b); however, they may be additionally utilized to assess the quality of already reported ones.
Disclosure statement
The authors report no conflicts of interest.
Notes
1. The focus of the article is to recommend guidelines for the design and conduct of human ingestion-time (chronopharmacology and chronotherapy) prescription hypertension medications trials. Guidelines for the design and conduct of such trials involving animal, cell culture and other models are endorsed, but are beyond the scope of this position paper.
References
- Agarwal R, Tu W. 2018. Minimally sufficient numbers of measurements for validation of 24-hour blood pressure monitoring in chronic kidney disease. Kidney Int. 94:1199–1204. doi:10.1016/j.kint.2018.08.021
- Angeli A, Gatti G, Masera R. 1992. Chronobiology of the hypothalamic-pituitary-adrenal and renin-angiotensin-aldosterone systems. In: Touitou Y, Haus E, editors. Biologic rhythms in clinical and laboratory medicine. Berlin: Springer-Verlag; p. 292–314.
- Astrup AS, Nielsen FS, Rossing P, Ali S, Kastrup J, Smidt UM, Parving HH. 2007. Predictors of mortality in patients with type 2 diabetes with or without diabetic nephropathy: a follow-up study. J Hypertens. 25:2479–2485. doi:10.1097/HJH.0b013e3282f06428
- Ayala DE, Hermida RC, Mojón A, Fernández JR. 2013. Cardiovascular risk of resistant hypertension: dependence on treatment-time regimen of blood pressure-lowering medications. Chronobiol Int. 30:340–352. doi:10.3109/07420528.2012.701455
- Ayala DE, Moyá A, Crespo JJ, Castiñeira C, Domínguez-Sardiña M, Gomara S, Sineiro E, Mojón A, Fontao MJ, Hermida RC. 2013b. Circadian pattern of ambulatory blood presure in hypertensive patients with and without type 2 diabetes. Chronobiol Int. 30:99–115. doi:10.3109/07420528.2012.701489
- Baraldo M. 2008. The influence of circadian rhythyms on the kinetics of drugs in humans. Expert Opin Drug Metab Toxicol. 4:175–192. doi:10.1517/17425255.4.2.175
- Bélanger PM, Bruguerolle B, Labrecque G. 1997. Rhythms in pharmacokinetics: absorption, distribution, metabolism, and excretion. In: Redfern PH, Lemmer B, editors. Physiology and pharmacology of biological rhythms. handbook of experimental pharmacology series (Vol. 125). Berlin-New York: Springer-Verlag; p. 177–204.
- Ben-Dov IZ, Kark JD, Ben-Ishay D, Mekler J, Ben-Arie L, Bursztyn M. 2007. Predictors of all-cause mortality in clinical ambulatory monitoring. Unique aspects of blood pressure during sleep. Hypertension. 49:1235–1241. doi:10.1161/HYPERTENSIONAHA.107.087262
- Boggia J, Li Y, Thijs L, Hansen TW, Kikuya M, Björklund-Bodegård K, Richart T, Ohkubo T, Kuznetsova T, Torp-Pedersen CH, et al. 2007. Prognostic accuracy of day versus night ambulatory blood pressure: a cohort study. Lancet. 370:1219–1229. doi:10.1016/S0140-6736(07)61538-4
- Booth 3rd JN, Muntner P, Abdalla M, Diaz KM, Viera AJ, Reynolds K, Schwartz JE, Shimbo D. 2016. Differences in night-time and daytime ambulatory blood pressure when diurnal periods are defined by self-report, fixed times, and actigraphy: improving the Detection of Hypertension study. J Hypertens. 34:235–243. doi:10.1097/HJH.0000000000000791
- Bovha Hus K, Kersnik Levart T. 2019. Does the duration of ambulatory blood pressure measurement matter in diagnosing arterial hypertension in children? Blood Press Monit. 24:199–202. doi:10.1097/MBP.0000000000000387
- Bowles NP, Thosar SS, Herzig MX, Shea SA. 2018. Chronotherapy for hypertension. Curr Hypertens Rep. 20:97. doi:10.1007/s11906-018-0897-4
- Brotman DJ, Davidson MB, Boumitri M, Vidt DG. 2008. Impaired diurnal blood pressure variation and all-cause mortality. Am J Hypertens. 21:92–97. doi:10.1038/ajh.2007.7
- Bruguerolle B. 1998. Chronopharmacokinetics. Current status. Clin Pharmacokinet. 35:83–94. doi:10.2165/00003088-199835020-00001
- Chiang CE, Wang TD, Ueng KC, Lin TH, Yeh HI, Chen CY, Wu YJ, Tsai WC, Chao TH, Chen CH, et al. 2015. 2015 guidelines of the taiwan society of cardiology and the taiwan hypertension society for the management of hypertension. J Chin Med Assoc. 78:1–47. doi:10.1016/j.jcma.2014.11.005
- Clement DL, De Buyzere ML, De Bacquer DA, de Leeuw PW, Duprez DA, Fagard RH, Gheeraert PJ, Missault LH, Braun JJ, Six RO, et al. 2003. Prognostic value of ambulatory blood-pressure recordings in patients with treated hypertension. N Engl J Med. 348:2407–2415. doi:10.1056/NEJMoa022273
- Coccina F, Pierdomenico AM, Cuccurullo C, Pizzicannella J, Madonna R, Trubiani O, Cipollone F, Pierdomenico SD. 2020. Prognostic value of masked uncontrolled hypertension defined by different ambulatory blood pressure criteria. Am J Hypertens. 33:726–733. doi:10.1093/ajh/hpaa078
- Crespo C, Aboy M, Fernández JR, Mojón A. 2012. Automatic identification of activity-rest periods based on actigraphy. Med Biol Eng Comput. 50:329–340. doi:10.1007/s11517-012-0875-y
- Crespo C, Fernández JR, Aboy M, Mojón A. 2013. Clinical application of a novel automatic algorithm for actigraphy-based activity and rest period identification to accurately determine awake and asleep ambulatory blood pressure parameters and cardiovascular risk. Chronobiol Int. 30:43–54. doi:10.3109/07420528.2012.701147
- Curtis BJ, Ashbrook LH, Young T, Finn LA, Fu YH, Plácek LJ, Jones CR. 2019. Extreme morning chronotypes are often familial and not exceedingly rare: the estimated prevalence of advanced sleep phase, familial advanced sleep phase, and advanced sleep-wake phase disorder in a sleep clinic population. Sleep. 42:zsz148. doi:10.1093/sleep/zsz148
- De Giorgi A, Menegatti AM, Fabbian F, Portaluppi F, Manfredini R. 2013. Circadian rhythms and medical diseases: does it matter when drugs are taken? Eur J Intern Med. 24:698–706. doi:10.1016/j.ejim.2013.03.019
- De Vries ST, Denig P, Ekhart C, Burgers JS, Kleefstra N, Mol PGM, van Puijenbroek EP. 2019. Sex differences in adverse drug reactions reported to the National Pharmacovigilance Centre in the Netherlands: an explorative observational study. Br J Clin Pharmacol. 85:1507–1515. doi:10.1111/bcp.13923
- Dixon WJ, Massey FJ. 1983. Introduction to statistical analysis. 4th ed. New York: McGraw-Hill.
- Dolan E, Stanton A, Thijs L, Hinedi K, Atkins N, McClory S, Den Hond E, McCormack P, Staessen JA, O’Brien E. 2005. Superiority of ambulatory over clinic blood pressure measurement in predicting mortality: the Dublin outcome study. Hypertension. 46:156–161. doi:10.1161/01.HYP.0000170138.56903.7a
- Eguchi K, Pickering TG, Hoshide S, Ishikawa J, Ishikawa S, Schwartz J, Shimada K, Kario K. 2008. Ambulatory blood pressure is a better marker than clinic blood pressure in predicting cardiovascular events in patients with/without type 2 diabetes. Am J Hypertens. 21:443–450. doi:10.1038/ajh.2008.4
- Fabbian F, Smolensky MH, Tiseo R, Pala M, Manfredini R, Portaluppi F. 2013. Dipper and non-dipper blood pressure 24-hour patterns: circadian rhythm-dependent physiologic and pathophysiologic mechanisms. Chronobiol Int. 30:17–30. doi:10.3109/07420528.2012.715872
- Fagard RH, Celis H, Thijs L, Staessen JA, Clement DL, De Buyzere ML, De Bacquer DA. 2008. Daytime and nighttime blood pressure as predictors of death and cause-specific cardiovascular events in hypertension. Hypertension. 51:55–61. doi:10.1161/HYPERTENSIONAHA.107.100727
- Fagard RH, Cornelissen VA. 2007. Incidence of cardiovascular events in white-coat, masked and sustained hypertension versus true normotension: a meta-analysis. J Hypertens. 25:2193–2198. doi:10.1097/HJH.0b013e3282ef6185
- Fan HQ, Li Y, Thijs L, Hansen TW, Boggia J, Kikuya M, Björklund-Bodegard K, Richart T, Ohkubo T, Jeppesen J, et al. 2010. Prognostic value of isolated nocturnal hypertension on ambulatory measurement in 8711 individuals from 10 populations. J Hypertens. 28:2036–2045. doi:10.1161/HYPERTENSIONAHA.107.100727
- Fernández JR, Mojón A, Hermida RC; for the Hygia Project Investigators. 2020. Chronotherapy of hypertension: advantages of 48-h ambulatory blood pressure monitoring assessments in MAPEC and Hygia Chronotherapy Trial. Chronobiol Int. 37:739–750. doi:10.1080/07420528.2020.1771355
- Flight L, Julious SA. 2016a. Practical guide to sample size calculations: an introduction. Pharm Stat. 15:68–74. doi:10.1002/pst.1709
- Flight L, Julious SA. 2016b. Practical guide to sample size calculations: superiority trials. Pharm Stat. 15:75–79. doi:10.1002/pst.1718
- Frank M, Peyrard S, Bobrie G, Azizi M. 2010. Method of mean value calculation as an additional source of variability in ambulatory blood pressure measurement. Am J Hypertens. 23:725–731. doi:10.1038/ajh.2010.47
- Gupta R, Malik AH, Popli T, Ranchal P, Yandrapalli S, Aronow WS. 2020. Impact of bedtime dosing of antihypertensives compared to morning therapy: A meta-analysis of randomised controlled trials. Eur J Prev Cardiol. [Epub ahead of print]. doi:10.1177/2047487320903611.
- Hermida RC, Ayala DE, Crespo JJ, Mojón A, Chayán L, Fontao MJ, Fernández JR. 2013a. Influence of age and hypertension treatment-time on ambulatory blood pressure in hypertensive patients. Chronobiol Int. 30:176–191. doi:10.3109/07420528.2012.701131
- Hermida RC, Ayala DE, Fontao MJ, Mojón A, Fernández JR. 2013b. Ambulatory blood pressure monitoring: importance of sampling rate and duration – 48 versus 24 hours – on the accurate assessment of cardiovascular risk. Chronobiol Int. 30:55–67. doi:10.3109/07420528.2012.701457
- Hermida RC, Ayala DE, Mojón A, Fernández JR. 2010. Influence of circadian time of hypertension treatment on cardiovascular risk: results of the MAPEC study. Chronobiol Int. 27:1629–1651. doi:10.3109/07420528.2010.510230
- Hermida RC, Ayala DE, Mojón A, Fernández JR. 2011a. Decreasing sleep-time blood pressure determined by ambulatory monitoring reduces cardiovascular risk. J Am Coll Cardiol. 58:1165–1173. doi:10.1016/j.jacc.2011.04.043
- Hermida RC, Ayala DE, Mojón A, Fernández JR. 2011b. Influence of time of day of blood pressure-lowering treatment on cardiovascular risk in hypertensive patients with type 2 diabetes. Diabetes Care. 34:1270–1276. doi:10.2337/dc11-0297/-/DC1
- Hermida RC, Ayala DE, Mojón A, Fernández JR. 2011c. Bedtime dosing of antihypertensive medications reduces cardiovascular risk in CKD. J Am Soc Nephrol. 22:2313–2321. doi:10.1681/ASN.2011040361
- Hermida RC, Ayala DE, Mojón A, Fernández JR. 2012. Sleep-time blood pressure and the prognostic value of isolated-office and masked hypertension. Am J Hypertens. 25:297–305. doi:10.1038/ajh.2011.208
- Hermida RC, Ayala DE, Mojón A, Fernández JR. 2013c. Blunted sleep-time relative blood pressure decline increases cardiovascular risk independent of blood pressure level – the “normotensive non-dipper” paradox. Chronobiol Int. 30:87–98. doi:10.3109/07420528.2012.701127
- Hermida RC, Ayala DE, Mojón A, Fontao MJ, Chayán L, Fernández JR. 2013d. Differences between men and women in ambulatory blood pressure thresholds for diagnosis of hypertension based on cardiovascular outcomes. Chronobiol Int. 30:221–232. doi:10.3109/07420528.2012.701487
- Hermida RC, Ayala DE, Mojón A, Smolensky MH, Crespo JJ, Otero A, Domínguez-Sardiña M, Moyá A, Ríos MT, Castiñeira MC, et al. 2020a. Cardiovascular disease risk stratification by the Framingham Score is markedly improved by ambulatory compared to office blood pressure. Rev Esp Cardiol. [Epub ahead of print]. doi:10.1016/j.rec.2020.08.004.
- Hermida RC, Ayala DE, Mojón A, Smolensky MH, Fernández JR. 2019a. Diagnosis and management of hypertension: around-the-clock ambulatory blood pressure monitoring is substantially more effective and less costly than daytime office blood pressure measurements. Chronobiol Int. 36:1515–1527. doi:10.1080/07420528.2019.1658201
- Hermida RC, Ayala DE, Portaluppi F. 2007. Circadian variation of blood pressure: the basis for the chronotherapy of hypertension. Adv Drug Deliv Rev. 59:904–922. doi:10.1016/j.addr.2006.08.003
- Hermida RC, Ayala DE, Smolensky MH, Fernández JR, Mojón A, Portaluppi F. 2016. Chronotherapy with conventional blood pressure medications improves management of hypertension and reduces cardiovascular and stroke risks. Hypertens Res. 39:277–292. doi:10.1007/s00125-015-3748-8
- Hermida RC, Ayala DE, Smolensky MH, Fernández JR, Mojón A, Portaluppi F. 2017. Sleep-time blood pressure: unique sensitive prognostic marker of vascular risk and therapeutic target for prevention. Sleep Med Rev. 33:17–27. doi:10.1016/j.smrv.2016.04.001
- Hermida RC, Crespo JJ, Domínguez-Sardiña M, Otero A, Moyá A, Ríos MT, Sineiro E, Castiñeira MC, Callejas PA, Pousa L, et al. 2019b. Bedtime hypertension treatment improves cardiovascular risk reduction: the Hygia Chronotherapy Trial. Eur Heart J. [Epub ahead of print]. doi:10.1093/eurheartj/ehz754.
- Hermida RC, Crespo JJ, Otero A, Domínguez-Sardiña M, Moyá A, Ríos MT, Castiñeira MC, Callejas PA, Pousa L, Sineiro E, et al. 2018. Asleep blood pressure: significant prognostic marker of vascular risk and therapeutic target for prevention. Eur Heart J. 39:4159–4171. doi:10.1093/eurheartj/ehy475
- Hermida RC, Fernández JR, Ayala DE, Mojón A, Alonso I, Calvo C. 2004. Circadian time-qualified tolerance intervals for ambulatory blood pressure monitoring in the diagnosis of hypertension. Chronobiol Int. 21:149–160. doi:10.1081/cbi-120027988
- Hermida RC, Hermida-Ayala RG, Smolensky MH, Mojón A, Crespo JJ, Otero A, Ríos MT, Domínguez-Sardiña M, Fernández JR. 2020b. Does timing of antihypertensive medication dosing matter? Curr Cardiol Rep. 22:118. doi:10.1007/s11886-020-01353-7
- Hermida RC, Hermida-Ayala RG, Smolensky MH, Mojón A, Fernández JR. 2020c. Chronopharmacology of hypertension medications: impact of ingestion-time on pharmacokinetics and pharmacodynamics. Exp Opin Drug Metab Toxicol. 16:1159–1173. doi:10.1080/17425255.2020.1825681.
- Hermida RC, Mojón A, Fernández JR, Otero A, Crespo JJ, Domínguez-Sardiña M, Ríos MT, Smolensky MH. 2020d. Ambulatory blood pressure monitoring-based definition of true arterial hypertension. Minerva Med. [Epub ahead of print]. doi:10.23736/S0026-4806.20.06834-2.
- Hermida RC, Smolensky MH, Ayala DE, Portaluppi F. 2015. Ambulatory blood pressure monitoring (ABPM) as the reference standard for diagnosis of hypertension and assessment of vascular risk in adults. Chronobiol Int. 32:1329–1342. doi:10.3109/07420528.2015.1113804
- Hermida RC, Smolensky MH, Ayala DE, Portaluppi F, Crespo JJ, Fabbian F, Haus E, Manfredini R, Mojón A, Moyá A, et al. 2013e. 2013 ambulatory blood pressure monitoring recommendations for the diagnosis of adult hypertension, assessment of cardiovascular and other hypertension-associated risk, and attainment of therapeutic goals. Joint recommendations from the International Society for Chronobiology (ISC), American Association of Medical Chronobiology and Chronotherapeutics (AAMCC), Spanish Society of Applied Chronobiology, Chronotherapy, and Vascular Risk (SECAC), Spanish Society of Atherosclerosis (SEA), and Romanian Society of Internal Medicine (RSIM). Chronobiol Int. 30:355–410. doi:10.3109/07420528.2013.750490
- Hernández-del Rey R, Martin-Baranera M, Sobrino J, Gorostidi M, Vinyoles E, Sierra C, Segura J, Coca A, Ruilope LM; for the Spanish Society of Hypertension Ambulatory Blood Pressure Monitoring Registry Investigators. 2007. Reproducibility of the circadian blood pressure pattern in 24-h versus 48-h recordings: the Spanish Ambulatory Blood Pressure Monitoring Registry. J Hypertens. 45:2406–2412. doi:10.1097/HJH.0b013e3282effed1
- Horne JA, Östberg O. 1976. A self-assessment questionnaire to determine morningness-eveningness in human circadian rhythms. Int J Chronobiol. 4:97–110. PMID: 1027738.
- Howard VJ, Madsen TE, Kleindorfer DO, Judd SE, Rhodes JD, Soliman EZ, Kissela BM, Safford MM, Moy CS, McClure LA, et al. 2019. Sex and race differences in the association of incident ischemic stroke with risk factors. JAMA Neurol. 76:179–186. doi:10.1001/jamaneurol.2018.3862.
- Ingelsson E, Bjorklund-Bodegard K, Lind L, Arnlov J, Sundstrom J. 2006. Diurnal blood pressure pattern and risk of congestive heart failure. JAMA. 295:2859–2866. doi:10.1001/jama.295.24.2859
- Ji H, Kim A, Ebinger JE, Niiranen TJ, Claggett BL, Merz CNB, Cheng S. 2020. Sex differences in blood pressure trajectories over the life course. JAMA Cardiol. 5:19–26. doi:10.1001/jamacardiol.2019.5306
- Jones HE, Sinha MD. 2011. The definition of daytime and nighttime influences the interpretation of ABPM in children. Pediatr Nephrol. 26:775–781. doi:10.1007/s00467-011-1791-3
- Julious SA. 2004. Sample sizes for clinical trials with normal data. Stat Med. 23:1921–1986. doi:10.1002/sim.1783
- Kool MJ, Wijnen JA, Derkx FH, Struijker Boudier HA, Van Bortel LM. 1994. Diurnal variation in prorenin in relation to other humoral factors and hemodynamics. Am J Hypertens. 7:723–730. doi:10.1093/ajh/7.8.723
- Labrecque G, Beauchamp D. 2003. Rhythms and pharmacokinetics. In: Redfern P, editor. Chronotherapeutics. London: Pharmaceutical Press; p. 75–110.
- Lakatua DJ, Haus E, Halberg F, Halberg E, Wendt HW, Sackett-Lundeen LL, Berg HG, Kawasaki T, Ueno M, Uezono K, et al. 1986. Circadian characteristics of urinary epinephrine and norepinephrine from healthy young women in Japan and U.S.A. Chronobiol Int. 3:189–195. doi:10.3109/07420528609066366
- Lemmer B. 1996. The clinical relevance of chronopharmacology in therapeutics. Pharmacol Res. 33:107–115. doi:10.1006/phrs.1996.0016
- Lemoine P, Zawieja P, Ohayon MM. 2013. Associations between morningness/eveningness and psychopathology: an epidemiological survey in three in-patient psychiatric clinics. J Psychiatr Res. 47:1095–1098. doi:10.1016/j.jpsychires.2013.04.001
- Liu X, Liu X, Huang W, Leo S, Li Y, Liu M, Yuan H. 2014. Evening – versus morning – dosing drug therapy for chronic kidney disease patients with hypertension: a systematic review. Kidney Blood Press Res. 39:427–440. doi:10.1159/000368456
- Madsen TE, Howard G, Kleindorfer DO, Furie KL, Oparil S, Manson JE, Liu S, Howard VJ. 2019. Sex differences in hypertension and stroke risk in the REGARDS Study: A longitudinal cohort study. Hypertension. 74:749–755. doi:10.1161/HYPERTENSIONAHA.119.12729
- Mancia G, Ulian L, Parati G, Trazzi S. 1994. Increase in blood pressure reproducibility by repeated semi-automatic blood pressure measurements in the clinic environment. J Hypertens. 12:469–473. PMID: 8064172. doi:10.1097/00004872-199404000-00018.
- Minutolo R, Agarwal R, Borrelli S, Chiodini P, Bellizzi V, Nappi F, Cianciaruso B, Zamboni P, Conte G, Gabbai FB, et al. 2011. Prognostic role of ambulatory blood pressure measurement in patients with nondialysis chronic kidney disease. Arch Intern Med. 171:1090–1098. doi:10.1001/archinternmed.2011.230
- Mochizuki Y, Okutani M, Donfeng Y, Iwasaki H, Takusagawa M, Kohno I, Mochizuki S, Umetani K, Ishii H, Ijiri H, et al. 1998. Limited reproducibility of circadian variation in blood pressure dippers and nondippers. Am J Hypertens. 11:403–409. doi:10.1016/s0895-7061(97)00497-4
- Mojón A, Ayala DE, Piñeiro L, Otero A, Crespo JJ, Moyá A, Bóveda J, Pérez de Lis J, Fernández JR, Hermida RC. 2013. Comparison of ambulatory blood pressure parameters of hypertensive patients with and without chronic kidney disease. Chronobiol Int. 30:145–158. doi:10.3109/07420528.2012.703083
- Nakano S, Fukuda M, Hotta F, Ito T, Ishii T, Kitazawa M, Nishizawa M, Kigoshi T, Uchida K. 1998. Reversed circadian blood pressure rhythm is associated with occurrences of both fatal and nonfatal events in NIDDM subjects. Diabetes. 47:1501–1506. doi:10.2337/diabetes.47.9.1501
- National Institute for Health and Clinical Excellence. 2019. Hypertension in adults: diagnosis and management. NICE Guideline 136: methods, evidence and recommendations. National Clinical Guidelines Centre, London, UK. 2019. https://www.nice.org.uk/guidance/ng136 (accessed 14 August 2020).
- Ohayon MM 2011. Epidemiological data or normal sleep patterns. SleepEval. Com Website. https//www.sleepeval.com/norms/epidemiologicalData.html (accessed 27 August 2020).
- Ohayon MM, Carskadon MA, Guilleminault C, Vitiello MV. 2004. Meta-analysis of quantitative sleep parameters from childhood to old age in healthy individuals: developing normative sleep values across the human lifespan. Sleep. 27:1255–1273. doi:10.1093/sleep/27.7.1255
- Ohkubo T, Hozawa A, Yamaguchi J, Kikuya M, Ohmori K, Michimata M, Matsubara M, Hashimoto J, Hoshi H, Araki T, et al. 2002. Prognostic significance of the nocturnal decline in blood pressure in individuals with and without high 24-h blood pressure: the Ohasama study. J Hypertens. 20:2183–2189. doi:10.1097/00004872-200211000-00017
- Peixoto Filho AJ, Mansoor GA, White WB. 1995. Effects of actual versus arbitrary awake and sleep times on analyses of 24-h blood pressure. Am J Hypertens. 8:676–680. doi:10.1016/0895-7061(95)00211-7
- Pierdomenico SD, Cuccurullo F. 2011. Prognostic value of white-coat and masked hypertension diagnosed by ambulatory monitoring in initially untreated subjects: an updated meta-analysis. Am J Hypertens. 24:52–58. doi:10.1038/ajh.2010.203
- Piper MA, Evans CV, Burda BU, Margolis KL, O’Connor E, Whitlock EP. 2015. Diagnosis and predictive accuracy of blood pressure screening methods with consideration of rescreening intervals: A systematic review for the U.S. Preventive Services Task Force. Ann Intern Med. 162:192–204. doi:10.7326/M14-1539
- Portaluppi F, Tiseo R, Smolensky MH, Hermida RC, Ayala DE, Fabbian F. 2012. Circadian rhythms and cardiovascular health. Sleep Med Rev. 16:151–166. doi:10.1016/j.smrv.2011.04.003
- Rabi DM, McBrien KA, Sapir-Pichhadze R, Nakhla M, Ahmed SB, Dumanski SM, Butalia S, Leung AA, Harris KC, Cloutier L, et al. 2020. Hypertension Canada’s 2020 comprehensive guidelines for the prevention, diagnosis, risk assessment, and treatment of hypertension in adults and children. Can J Cardiol. 36:596–624. doi:10.1016/j.cjca.2020.02.086
- Reinberg A, Smolensky MH. 1982. Circadian changes of drug disposition in man. Clin Pharmacokin. 7:401–420. doi:10.2165/00003088-198207050-00002
- Roenneberg T, Kuehnle T, Juda M, Kantermann T, Allebrandt K, Gordijn M, Merrow M. 2007. Epidemiology of the human circadian clock. Sleep Med Rev. 11:429–438. doi:10.1016/j.smrv.2007.07.005
- Roush GC, Fagard RH, Salles GF, Pierdomenico SD, Reboldi G, Verdecchia P, Eguchi K, Kario K, Hoshide S, Polonia J, et al. 2014a. Prognostic impact from clinic, daytime, and nighttime systolic blood pressure in 9 cohorts on 13,844 patients with hypertension. J Hypertens. 32:2332–2340. doi:10.1097/HJH.0000000000000355
- Roush GC, Fapohunda J, Kostis JB. 2014b. Evening dosing of antihypertensive therapy to reduce cardiovascular events: a third type of evidence based on a systematic review and meta-analysis of randomized trials. J Clin Hypertens (Greenwich). 16:561–568. doi:10.1111/jch.12354
- Salles GF, Cardoso CR, Muxfeldt ES. 2008. Prognostic influence of office and ambulatory blood pressures in resistant hypertension. Arch Intern Med. 168:2340–2346. doi:10.1001/archinte.168.21.2340
- Salles GF, Reboldi G, Fagard RH, Cardoso C, Pierdomenico SD, Verdecchia P, Eguchi K, Kario K, Hoshide S, Polonia J, et al. 2016. Prognostic impact of the nocturnal blood pressure fall in hypertensive patients: the ambulatory blood pressure collaboration in patients with hypertension (ABC-H) meta-analysis. Hypertension. 67:693–700. doi:10.1161/HYPERTENSIONAHA.115.06981
- Schillaci G, Battista F, Settimi L, Schillaci L, Pucci G. 2015. Antihypertensive drug treatment and circadian blood pressure rhythm: a review of the role of chronotherapy in hypertension. Curr Pharm Des. 21:756–772. doi:10.2174/1381612820666141024130013
- Sedgwick P. 2014. Cross sectional studies: advantages and disadvantages. BMJ. 348:g2276. doi:10.1136/bmj.g2276
- Shiga T, Fujimura A, Tateishi T, Ohashi K, Eibara A. 1993. Differences of chronopharmacokinetic profiles between propranolol and atenolol in hypertensive subjects. J Clin Pharmacol. 33:756–761. doi:10.1002/j.1552-4604.1993.tb05620.x
- Smolensky MH, Hermida RC, Castriotta RJ, Portaluppi F. 2007. Role of sleep-wake cycle on blood pressure circadian rhythms and hypertension. Sleep Med. 8:668–680. doi:10.1016/j.sleep.2006.11.011
- Smolensky MH, Hermida RC, Portaluppi F. 2017. Circadian mechanisms of 24-hour blood pressure regulation and patterning. Sleep Med Rev. 33:4–16. doi:10.1016/j.smrv.2016.02.003
- Smolensky MH, Hermida RC, Reinberg A, Sackett-Lundeen L, Portaluppi F. 2016. Circadian disruption: new clinical perspective of disease pathology and basis for chronotherapeutic intervention. Chronobiol Int. 33:1101–1119. doi:10.1080/07420528.2016.1184678
- Smolensky MH, Sackett-Lundeen LL, Portaluppi F. 2015. Nocturnal night pollution and underexposure to daytime sunlight: complementary mechanisms of circadian disruption and related diseases. Chronobiol Int. 32:1029–1048. doi:10.3109/07420528.2015.1072002
- Sobiczewski W, Wirthwein M, Gruchala M, Kocic I. 2014. Mortality in hypertensive patients with coronary heart disease depends on chronopharmacotherapy and dipping status. Pharmacol Rep. 66:448–452. doi:10.1016/j.pharep.2013.12.009
- Sothern RB, Vesely DL, Kanabrocki EL, Hermida RC, Bremner FW, Third JLHC, Boles MA, Nemchausky BM, Olwin JH, Scheving LE. 1995. Temporal (circadian) and functional relationship between atrial natriuretic peptides and blood pressure. Chronobiol Int. 12:106–120. doi:10.3109/07420529509064506
- Stolarz AJ, Rusch NJ. 2015. Gender differences in cardiovascular drugs. Cardiovas Drugs Ther. 29:403–410. doi:10.1007/s10557-015-6611-8
- Stranges PM, Drew AM, Rafferty P, Shuster JE, Brooks AD. 2015. Treatment of hypertension with chronotherapy: is it time? Ann Pharmacother. 49:323–334. doi:10.1177/1060028014563535
- Sturrock NDC, George E, Pound N, Stevenson J, Peck GM, Sowter H. 2000. Non-dipping circadian blood pressure and renal impairment are associated with increased mortality in diabetes mellitus. Diabet Med. 17:360–364. doi:10.1046/j.1464-5491.2000.00284.x
- Sun Y, Yu X, Liu J, Zhou N, Chen L, Zhao Y, Li X, Wang J, Cui L. 2016. Effect of bedtime administration of blood-pressure lowering agents on ambulatory blood pressure monitoring results: a meta-analysis. Cardiol J. 23:473–481. doi:10.5603/CJ.a2016.0027
- Thien T, Keltjens EBM, Lenders JWM, Deinum J. 2015. Should blood pressure be measured with the cuff on a bare arm? Blood Press Monit. 20:320–324. doi:10.1097/MBP.0000000000000142
- Umemura S, Arima H, Arima S, Asayama K, Dohi Y, Hirooka Y, Horio T, Hoshide S, Ikeda S, Ishimitsu T, et al. 2019. The Japanese society of hypertension guidelines for the management of hypertension (JSH 2019). Hypertens Res. 42:1235–1481. doi:10.1038/s41440-019-0284-9
- Unger T, Borghi C, Charchar F, Khan NA, Poulter NR, Prabhakaran D, Ramirez A, Schlaich M, Stergiou GS, Tomaszewski M, et al. 2020. 2020 International Society of Hypertension global hypertension practice guidelines. Hypertension. 75:1334–1357. doi:10.1161/HYPERTENSIONAHA.120.15026
- Verdecchia P, Porcellati C, Schillaci G, Borgioni C, Ciucci A, Battistell M, Guerrieri M, Gatteschi C, Zampi I, Santucci A, et al. 1994. Ambulatory blood pressure: an independent predictor of prognosis in essential hypertension. Hypertension. 24:793–801. doi:10.1161/01.hyp.24.6.793
- Wang JG. 2015. Chinese hypertension guidelines. Pulse. 3:14–20. doi:10.1159/000382025
- Whelton PK, Carey RM, Aronow WS, Casey DE Jr, Collins KJ, Himmerfarb CD, DePalma SM, Gidding S, Jamerson KA, Jones DW, et al. 2018. 2017 ACC/AHA/AAPA/ABC/ACPM/AGS/APhA/ASH/ASPC/NMA/PCNA Guideline for the Prevention, Detection, Evaluation, and Management of High Blood Pressure in Adults: A Report of the American College of Cardiology/American Heart Association Task Force on Clinical Practice Guidelines. J Am Coll Cardiol. 71:e127–e248. doi:10.1016/j.jacc.2017.11.006
- Williams B, Mancia G, Spiering W, Rosei EA, Azizi M, Burnier M, Clement DL, Coca A, de Simone G, Dominiczak A, et al. 2018. 2018 ESC/ESH Guidelines for the management of arterial hypertension: the Task Force for the management of arterial hypertension of the European Society of Cardiology (ESC) and the European Society of Hypertension (ESH). Eur Heart J. 39:3021–3104. doi:10.1093/eaurheartj/ehy339
- Zhang Z, Cajochen C, Khatami R. 2019. Social jetlag and chronotypes in the chinese population: analysis of data recorded by wearable devices. J Med Internet Res. 21:e13482. doi:10.2196/13482
- Zhao P, Xu P, Wan C, Wang Z. 2011. Evening versus morning dosing regimen drug therapy for hypertension. Cochrane Database Syst Rev. 10:CD004184. doi:10.1002/14651858.CD004184.pub2