ABSTRACT
We investigated whether chronotype and time-of-day modulate the time course of automatic and controlled semantic processing. Participants performed a category semantic priming task at either the optimal or non-optimal time of day. We varied the prime-target onset asynchrony (100-, 450-, 650-, and 850-ms SOAs) and kept the percentage of unrelated targets constant at 80%. Automatic processing was expected with the short SOA, and controlled processing with longer SOAs. Intermediate-types (Experiment 1) verified that our task was sensitive to capturing both types of processes and served as a reference to assess themin extreme chronotypes. Morning-type and evening-type participants (Experiment 2) differed in the influence of time of testing on priming effects. Morning-types applied control in all conditions, and no performance modulation by time-of-day was observed. In contrast, evening-types were most adversely affected by the time of day to shift from automatic-based to controlled-based responses. Also, they were considerably affected in successfully implementing controlled processing with long intervals, particularly at the non-optimal time of day, with inhibitory priming showing only a marginally significant effect at the longest SOA. These results suggest that extreme chronotypes may be associated with different styles of cognitive control. Morning-types would be driven by a proactive control style, whereas a reactive control style might be applied by evening-types.
Introduction
Human circadian rhythms are physiologically driven by a central pacemaker that is the suprachiasmatic nuclei (SCN) (Miller et al. Citation1996) and connect the organism with the 24 hours of a day on Earth. Nevertheless, circadian functioning may vary from one individual to another. Thus, we refer to the existence of different chronotypes, that is, the preferences that individuals develop for performing their daily life activities and resting earlier or later in the day (Levandovski et al. Citation2013; Schmidt et al. Citation2007). The assessment of chronotype allows individuals to be classified into different circadian profiles that are distributed along a Gaussian curve at the population level. Intermediate-types (i.e. those who do not develop any specific circadian preference), would be located around the centre, while morning-types (i.e. those who prefer to engage in their routines in the early morning hours) and evening-types (i.e. those with a more later-day profile) occupy the extremes of the distribution (Roenneberg et al. Citation2007).
The study of chronotype has become interesting since the continuous development of society has forced individuals not to rely on a 24-h diurnal cycle. For instance, when a doctor must operate at 03:00 h in an emergency, a student must plan her or his study schedule for exams, or an air traffic controller must stand guard at her or his post from 05:00 h, the consideration of chronotypes and how they operate can predict success. Recently, this trait has been linked to aspects of mental health (Walsh et al. Citation2022), effects of shift work (Cheng et al. Citation2021), and school performance (Goldin et al. Citation2020).
Delving into the cognitive level, the mental processes that are primarily underpinned by the prefrontal cortex have proven to be influenced mainly by the chronotype and the time of day, usually showing a performance enhancement at the time of day that matches the individual’s preference (i.e. the optimal time). This effect is known as the synchrony effect (May and Hasher Citation1998), and has been reflected in a wide variety of cognitive tasks (for a review, see Adan et al. Citation2012). Nevertheless, the synchrony effect is often not the same for morning- and evening-types. In contrast to evening-types, morning-types have demonstrated better adjustment and flexibility at non-optimal times (Lara et al. Citation2014; Martínez-Pérez et al. Citation2020; Palmero et al. Citation2022). In this line, it has been argued that personality traits such as impulsivity (Di Milia et al. Citation2011) and even hormonal secretions during the menstrual cycle in women (Palmero et al. Citation2022), may be linked to these chronotype-based differences. Furthermore, morning- and evening-types have revealed general differences in brain connectivity patterns (Facer-Childs et al. Citation2019), and in neuro-cognitive functioning reflected in alpha and theta oscillations recorded via electroencephalography (EEG) (Venkat et al. Citation2020) as well as in fMRI (Orban et al. Citation2020). More specifically, they seem to be unravelling specific patterns in the neural networks activated in response to certain cognitive demands such as conflict resolution or cognitive control (Schmidt et al. Citation2012). These results, together with findings that have put forward the development of specific biological patterns in each of the chronotype-traits, such as the delay in the secretion of activation and relaxation substances (i.e. cortisol and melatonin, Duffy et al. Citation2001; Oginska et al. Citation2010), or peaks and rhythms in body temperature (Kerkhof and Van Dongen Citation1996; Sarabia et al. Citation2008), have prompted researchers to propose that morning- and evening-types are not only the two extremes of a continuum, but also they are independent entities whose functioning, associated conditions and even specific needs, are particular within each group. This issue is explicitly reflected in the existence of specific research on each of the existent chronotype-traits (Facer-Childs et al. Citation2019; Martínez-Pérez et al. Citation2022), and by the fact that they have matured a strictly differentiated pattern of cognitive and biological functioning (Correa et al. Citation2014; Martínez-Pérez et al. Citation2020; Palmero et al. Citation2022; Venkat et al. Citation2020).
Pursuing the matter of the circadian influences on high-order cognitive operations, results obtained both at the behavioural and physiological levels have led researchers to argue that, as a whole, all mental operations that require control are more vulnerable to the time of testing than those that are more automatic in nature (Lara et al. Citation2014; Manly Citation2002; May et al. Citation2005). Automatic and controlled processes refer to the differential modes of processing information in our environment that result in an appropriate response (Schneider and Shiffrin Citation1977). It has commonly been stated that controlled processing is the more adaptive since it prevents responses that may have important negative consequences (Miller and Cohen Citation2001), although the need for automatic processing and the importance of not overcontrolling in certain circumstances have also been highlighted (Bocanegra and Hommel Citation2014).
Concerning people’s preferences for when to perform daily life activities, it is controlled rather than automatic processing that seems to fluctuate throughout the day. For instance, Fisk and Schneider (Citation1981) showed that the decrease in performance typically observed in vigilance tasks occurred specifically when controlled processing was necessary, while decrements in vigilance performance were not observed when automatic processing was involved. Thus, using the Sustained Attention to Response Task (SART), Manly (Citation2002) found differential effects due to the time of testing only in no-go trials, which required cognitive control and therefore controlled processing. In addition, Lara et al. (Citation2014) manipulated two response strategies when participants performed the SART: speed (automatic responding set) vs. accuracy (controlled responding set). Selective synchrony effects were found only when focused on accuracy. Finally, regarding memory, May et al. (Citation2005) and Yang et al. (Citation2007) found a pattern of increased explicit recall (controlled retrieval) at the optimal time of day for both morning- and evening-types. However, although automatic and controlled processes have previously been linked to chronotype and time of day, to our knowledge, studies that have addressed the emergence and time course of both types of processing in relation to circadian rhythms in a single task are rather scarce.
In the current study, we approached this issue using a paradigm that has commonly dissociated automatic and controlled processing: the semantic priming paradigm. In this paradigm, a prime stimulus (e.g. a word) is briefly presented and followed by a target stimulus that requires a quick response by the participant. The target can be a word or a pseudoword if a lexical decision is required. The semantic priming effect refers to the advantage in responding (shorter reaction times, RTs, and better accuracy) in related prime-target trials (e.g. DOCTOR – nurse) compared to unrelated prime-target trials (e.g. DOCTOR – bread) (Meyer and Schvaneveldt Citation1971). Although a discussion of the different models that account for the semantic priming effect is beyond the scope of the present study, semantic priming has been thought to involve the rapid activation of semantic information conveyed by the prime, which spreads to other associated words among which would be the associated target (Collins and Loftus Citation1975). Once the prime has been processed, participants may also consciously generate expectancies about candidate targets in a controlled manner. However, these expectancies require time to build up, and therefore they may only occur if there is enough time between the prime and the target onsets (Becker Citation1980). Thus, the prime-target onset asynchrony (usually referred to as prime-target SOA) is crucial for determining whether semantic processing is mainly due to automatic or controlled processing (Besner and Humphreys Citation1991), with the former being better captured with short prime-target SOAs and the latter with long prime-target SOAs (e.g. longer than 200 ms) (Neely et al. Citation1989). Semantic priming studies usually reveal facilitation in target responses when only the prime-target SOA is manipulated to engage either automatic or controlled semantic processing. Additionally, concerning prime processing, using a mask after its presentation has proven to target either automatic or controlled processes in semantic priming tasks. As such, the immediate appearance of a mask after the prime display would prevent the conscious processing of this stimulus, thereby steering the individual to act based on automatic-driven responses. On the other hand, the delayed presentation of the mask allows the prime to be consciously processed, given the time gap between its appearance and that of the mask, leading most likely to the development and use of controlled strategies (Daza et al. Citation2002; Merikle and Joordens Citation1997).
However, a more appropriate way of dissociating the involvement of automatic and controlled processing in a semantic priming task is to find qualitatively different patterns of results associated with each type of process (Merikle and Joordens Citation1997). Whereas the use of associative links between the prime and the target usually produces facilitatory priming effects, both facilitatory and inhibitory effects can be found with category semantic priming tasks when both the relatedness proportion and the prime-target SOA are manipulated (Besner and Humphreys Citation1991; McNamara Citation2005), signalling the emergence of entirely different response strategies according to their inner nature. Facilitatory priming refers to the advantage in performance with related prime-target trials over unrelated prime-target trials and the other way around for inhibitory priming. In the category semantic priming task, the prime is the name of a category (e.g. ANIMAL), and the target can be either an exemplar of the prime category (e.g. cat) in the related condition or an exemplar of a different category (e.g. table) in the unrelated condition. Participants will create expectations about the forthcoming target based on the frequency with which a related target follows a prime category and the interval between the prime and the target (Neely Citation1977). With a low rate of related targets, expectations based on the prime category will favor unrelated targets. Thus, the observation of shorter RTs with unrelated targets compared with related targets (inhibitory priming) will confirm that an expectation has been successfully generated. In contrast, any facilitatory effect, despite the low rate of related targets, will confirm that expectations were not developed; therefore, facilitatory priming would simply reflect the intervention of automatic processing. By using this paradigm, Ortells et al. (Citation2003) showed facilitatory priming effects with 200- and 300-ms prime-target SOAs, whereas the effect became inhibitory with a 500-ms SOA value. Using a similar design with young participants, Langley et al. (Citation2008) found a facilitatory priming effect only with a 100-ms SOA and inhibitory priming effects with 200–500- and 800-ms SOAs, effects being larger as the SOA value increased.
In the current study, we employed a category semantic priming task in which the name of a semantic category served as the prime stimulus and was followed by either a related or an unrelated target exemplar. A low rate of related targets (20%) was used to promote expectancy-based priming effects, and a short prime-target SOA and long prime-target SOAs were used in different blocks of trials to assess the emergence and, as a novelty, the time course of facilitatory (automatic) priming and inhibitory (controlled) priming effects, respectively. Thus, we were interested in the contrast between automatic and controlled processes independently but in the light of the interplay between them (see Ghin et al. Citation2022; Wolff et al. Citation2019). Thus, we aimed to deepen the transition from automatic responses to effective controlled processing, targeting the ability of individuals to reverse an automatic response trend in a context where control had to be recruited to give a correct response.
Experiment 1 involved intermediate chronotype participants, who have been consistently neglected in chronotype-related studies (May and Hasher Citation2017) despite representing 60% of the population (Roenneberg et al. Citation2007). This experiment allowed us to test the suitability of this category semantic priming task to dissociate the two types of processes. In Experiment 2, morning- and evening-type participants, who combined represent 40% of the population and are referred to as extreme chronotypes, performed the task to assess whether chronotype and time of testing are crucial factors that modulate the earlier appearance of automatic processing and the later emergence of controlled processing. Moreover, given the above-mentioned differences between the two chronotypes, our main interest was to observe how this cognitive strategy (i.e. the shift from automatic to controlled processing) may follow a different time course in each chronotype. Based on previous results, we hypothesize facilitatory priming with the short SOA, involving automatic processing. However, the facilitatory effect may likely turn into inhibitory at different moments for each chronotype. Therefore, and based on the results obtained in previous pilot studies with intermediate-types, the time course of automatic processing was addressed based on the division of a 100-ms SOA block into three consecutive subblocks. That division has not been usually done in previous related studies, however, is of special relevance here because participants performed the task in optimal and non-optimal arousal conditions according to their chronotype, which may promote the anticipated use of control-based strategies even in the short prime-target SOA block. Note that the younger adult group in Langley et al.’s (Citation2008) study showed inhibitory effects in the rather short 200-ms SOA. On the other hand, to assess the effects of time of testing on controlled processing, we explored both the emergence and time course of inhibitory priming with longer prime-target SOAs (450, 650, and 850 ms). In this case, based on the differences between the two extreme chronotypes in the ability to overcome the negative influences on performance caused by non-optimal times of day (Lara et al. Citation2014; Martínez-Pérez et al. Citation2020; Palmero et al. Citation2022, Citation2024), we also hypothesized that it is likely that inhibitory priming (also facilitatory priming?) may exhibit a different time course in each chronotype as a function of time of day. Although all participants may be competent to implement control consistently, chronotype and time of day may influence the efficiency and timing with which individuals engage in this sort of response strategy, as it has been shown by Langley et al. (Citation2008) when the authors addressed the time course of both priming effects as a function of the participant’s age.
We also tested the existence of synchrony effects in each chronotype and expected them to generally affect controlled but not automatic processing, but we went further and investigated how the time course of such effects may change as a function of both chronotype and time of testing.
Finally, to ensure the appropriate selection of our sample of extreme chronotypes, we used the psychomotor vigilance task (PVT). This task has proven to be very sensitive to circadian-related performance (Blatter and Cajochen Citation2007) and has been widely used in cognitive research, mainly related to vigilance and sustained attention (Lara et al. Citation2014; Martínez-Pérez et al. Citation2020,Citation2022; Molina et al. Citation2019).
Materials and methods
Participants
Sixty-four undergraduates (Mage = 21.14 years; SDage = 5.45) completed the experiment for course credit. The sample was recruited based on the scores obtained in the reduced version of the Horne and Östberg’s Morningness-Eveningness Questionnaire (rMEQ) standardized by Adan and Almirall (Citation1991) for the Spanish population. This brief questionnaire classifies individuals, depending on their circadian preference, into definite morning- or evening-types and neither or intermediate-types. In this case, we considered the chronotype as a continuum, with the morning- and evening-chronotype groups consisting of moderate and extreme individuals, following previous studies (Lara et al. Citation2014; Martínez-Pérez et al. Citation2020; Palmero et al. Citation2022; Salehinejad et al. Citation2021). We had three chronotype-based groups. Twenty-four students with scores ranging from 12 to 16 were assigned to the intermediate-type group (M = 13.60; SD = 1.66) and participated in Experiment 1. Twenty students with scores between 17 and 25 (corresponding to moderate-to-extreme morning-types) were assigned to the morning-type group (M = 18.50; SD = 1.60), and 20 students with scores between 4 and 11 (corresponding to moderate-to-extreme evening-types) were assigned to the evening-type group (M = 9.25; SD = 1.68). An a posteriori sensitivity analysis using G*Power was performed to corroborate the effect size our study detected, given that our main interest was in testing the synchrony effect. Given two groups (morning and evening), and two measures for each (optimal and non-optimal times), with an alpha level of .05, a statistical power of at least .80, a sample size of n = 40 participants, a correlation between repeated measures of r = .5 and a non-sphericity correction of 1, the effect size f that our design was able to detect was .22. Thus, the sample size that comprised our study warranted the detection of small-to-medium effect sizes. Both morning- and evening-type participants participated in Experiment 2. All participants reported normal or corrected-to-normal vision and the absence of chronic medical conditions. Note that although both groups were composed of moderate and extreme participants, throughout the manuscript, we refer to morning- and evening-types as extreme chronotypes to differentiate them from intermediate-types.
Tasks and design
Two experimental tasks programmed with E-Prime 3 (Psychology Software Tools, Pittsburgh, PA Citation2016; Schneider et al. Citation2012) were used. Stimuli were presented on a 22-inch computer screen with a 1920 × 1080 pixels resolution. Responses were recorded by using a Chronos® device (Psychology Software Tools, Pittsburgh, PA Citation2016).
The PVT assessed the morning-types and evening-types arousal-vigilance states by presenting a red circle (50 pixels in diameter) in the centre of the screen to which the participants had to respond as quickly as possible. The red circle was presented in each trial after a random interval between 2 and 10 s in which the computer screen remained black. The participants were instructed to press the central button of the response box with the index finger of their dominant hand when the stimulus appeared. Once the trial was completed, a new trial began.
On the other hand, the category semantic priming task assessed performance under automatic or controlled processing. The stimuli were all presented on the center of the screen in black against a white background. The categories used as primes were the Spanish words ANIMAL (animal) and MUEBLE (furniture) (on average: 3.72° in width; 0.71° in height) and were always presented in uppercase letters. The prime was displayed on the screen in all cases for 50 ms. In addition, the target words were familiar exemplars of each of the two categories. For animals, burro (donkey), gato (cat), tigre (tiger), and foca (seal) were used. For furniture, mesa (table), silla (chair), percha (hanger), and cama (bed) were used. The stimuli subtended 2.90° width and 0.57° height on average. All stimuli were presented in Spanish and lowercase letters.
For all participants, in 80% of the trials, the prime and the target stimuli were unrelated, so the target (e.g. seal) did not belong to the category of the prime (e.g. FURNITURE), and in the remaining 20% of trials, the prime (e.g. ANIMAL) preceded a target of the same category (e.g. cat). The time between the onset of the prime stimulus and the onset of the target (i.e. SOA) was manipulated to foster the development of automatic and controlled processing. Four different SOAs were considered. First, a short 100-ms SOA was chosen to promote automatic processing based on previous studies (Langley et al. Citation2008). To assess control processing and the time course of strategy acquisition, we used three long SOAs (450, 650, and 850 ms).
For the presentation of the different SOAs, a blocked design was used. The short-SOA block had 125 trials, from which the first 10 were considered practice. Thus, the automatic-processing experimental block consisted of 115 trials. Subsequently, all long SOAs were randomly presented in a block comprising 300 trials. The first 30 trials were considered practice (approximately 10 trials per SOA). Each long SOA was presented in 90 different trials, bringing the total number of trials to 270. Finally, the task comprised 425 trials, and the design was similar to that used in previous studies (Langley et al. Citation2008; Ortells et al. Citation2003). The order of the blocks was the same for all participants: first, they completed the short-SOA block (reflecting automatic processing) and then the long-SOA block (reflecting controlled processing). The rationale for not using a single block with the four SOAs randomly presented nor counterbalancing the order of the short and long SOA blocks was to avoid applying the control elicited by the long SOA trials to the automatic processing evaluated through the short SOA, as previous studies have shown that the two types of processes can interact during a semantic priming experiment (Balota et al. Citation1992; Lerner et al. Citation2014; Neely et al. Citation2010).
Moreover, to maximize the development of automatic processing and controlled expectancies, a random letter stimulus, “XDGTKSN” (4.30 > width; 0.72 > height), was used as a mask between the prime and the target. Although it was present in all blocks, the delay between the presentation of the prime and the mask varied depending on the SOA. In the short-SOA block, the mask was presented immediately after the prime and lasted 50 ms. In the long-SOA block, the mask length was also 50 ms but was presented with an adapted delay after the prime according to the SOA of each of the trials (i.e. 350, 550, and 750 ms, respectively). It is important to note that using the mask in the short-prime task is meant to promote automatic processing, but by no means does it prevent the participants from being aware of the prime stimulus, and hence it is not designed to promote unconscious priming effects.
The participants were instructed to press two different buttons (the leftmost and rightmost) on the response box to classify the target words. The order of the buttons was counterbalanced between participants. Thus, half of them responded by pressing button 1 to the exemplars of the category ANIMAL and button 5 to the category FURNITURE, and the button assignment was reversed for the other half of the participants. The time to respond to the target was unlimited, so once it was presented, it remained on the screen until the participant’s response. Once a response was made, a new trial began. An example of each type of trial can be seen in .
Figure 1. Sequence of trials in the category semantic priming task with the short 100-ms SOA (left) and the long 450-, 650- and 850-ms SOAs (right).
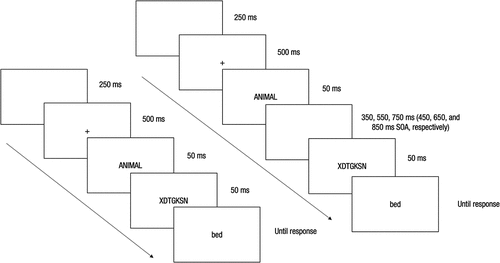
Before the task started, the participants were informed of the relatedness of the prime-target proportion. That information was given to foster the use of expectations based on the prime stimulus from the very beginning of the experimental session. Also, to prioritize an accuracy strategy, we instructed the participants to respond correctly rather than quickly.
General procedure
After being selected based on their scores on the rMEQ, the participants were invited to complete the experimental sessions.
In Experiment 1, the participants completed only one experimental session scheduled from 10:00 h to 16:00 h, as they did not possess specific preferred daytime slots. The experimental session consisted of completing only the category semantic priming task, which lasted approximately 40 min.
In Experiment 2, each participant came to the laboratory twice, with an interval of approximately 7 days between sessions. The sessions were scheduled at 08:00 h (morning) and 20:30 h (evening). This procedure is commonly implemented in studies using the extreme-chronotypes paradigm (Blatter and Cajochen Citation2007; Schmidt et al. Citation2007) and allows us to obtain an assessment of the participant’s performance at both their optimal and non-optimal times of the day according to their chronotype (i.e. to study the potential synchrony effects). The order of the sessions was counterbalanced across participants within each chronotype group so that half of the sample started the experiment at 08:00 h and the other half at 20:30 h. All sessions had the same structure and duration (~1 h). In all cases, the participants in Experiment 2 started the session by performing the PVT and subsequently performed the category semantic priming task. Instructions were given in the same way in all sessions.
Participants were instructed to spend at least five hours of sleep the night before the experimental session, and once in the lab, they were queried on this question to verify they were not sleep-deprived. Also, we monitored the consumption of stimulants so that all participants were asked not to take any stimulant substances such as coffee or tea in the 2 h before the start of the session.
Results
Data were pre-processed with R software (R Core Team Citation2022) and analysed with JASP 0.9.2 (JASP Team Citation2022). Regarding the PVT, the first trial, trials with RTs shorter than 150 ms, and trials with RTs separated by more than 3.5 semi-interquartile ranges from the median value of each participant in each session were considered outliers and discarded (1.77% of trials). Regarding the category semantic priming task, we applied two different filters according to the graphical distribution of the RT raw data. We first established a long-time-interval where most of the participants’ responses were concentrated: 100 ms to 4000 ms. Specifically, only 3 participants made some responses above 4000 ms, and no one made responses below 100 ms. Second, we considered outliers all the RTs that were separated by more than 3.5 semi-interquartile ranges from the median value of each participant in each condition. The percentage of discarded trials did not exceed 1.10% in any experimental condition. Mean RTs were calculated considering only RTs associated with correct responses and after discarding practice trials. Then, RTs statistical data-analysis only covered trials where participants responded correctly. For the accuracy data, the statistical analysis was conducted with the proportion of correct responses. Accuracy rates and mean RTs from all participants in the study are shown in Table S1 in the supplementary material (see category_semantic_priming_data.xlsx).
To facilitate the interpretation of the priming effects shown in the graphs, it is important to consider that priming effects were always obtained by subtracting the performance on unrelated trials from the performance on related trials. With RTs, facilitatory priming produced scores with a positive sign (upper part of the figures), and inhibitory priming produced scores with a negative sign (lower part of the figures). The opposite was true with accuracy data, facilitatory priming produced scores with a negative sign (lower part of the figures), and inhibitory priming produced scores with a positive sign (upper part of the figures).
We adopted a statistical significance level of α = .05 for all statistical analyses. Moreover, we present effect size values for each of our contrasts through the partial eta squared (ηp2) for ANOVA and Cohen’s d (d) for Student’s t-tests.
Demographic data
The analysis of the scores on the rMEQ used to classify individuals according to their chronotype showed a main effect of chronotype, F(2, 62) = 157.40; p < 0.001; ηp2 = .84. Post-hoc tests with Bonferroni correction showed significant differences between the three groups: morning-types vs intermediate-types, t(63) = 9.90, p < 0.001, d = 2.99; evening-types vs intermediate-types, t(63) = 8.79, p < 0.001, d = 2.60; and morning-types vs evening-types, t(63) = 17.73, p < 0.001, d = 5.62. The age analysis showed no statistically significant differences between the groups (p = 0.99). Participants reported sleeping at least five hours as instructed so that no one was eliminated due to sleep deprivation. Moreover, as instructed, none of the participants reported caffeine consumption in the 2 h before the experiment.
Experiment 1: intermediate-type chronotype
Category semantic priming task
The analyses were conducted separately for the 100-ms SOA and the long SOAs. For the 100-ms SOA, data were subjected to 2 × 3 repeated-measures ANOVAs with relatedness (related and unrelated) and subblock (first, second and third) as within-participants factors. For the long SOAs, data were subjected to 2 × 3 repeated-measures ANOVAs with relatedness (related and unrelated) and prime-target SOA (450, 650 and 850 ms) as within-participants factors.
Accuracy analysis
The results are shown in . For the 100-ms SOA, the main effect of relatedness was not significant, F < 1. Also, priming effects did not vary across the three subblocks of trials (all ps > .24). For the long SOAs, we observed a significant main effect of relatedness, F(1, 25) = 6.75, p = 0.01, ηp2 = .21, indicating higher proportion of correct responses in unrelated (M = .94) than in related trials (M = .90), that is, an inhibitory priming effect. No other effects or interactions were significant (all ps > .61).
Figure 2. Intermediate-type chronotype. Performance at the short (100 ms) and the long (450, 650, and 850 ms) SOAs. (a) Accuracy priming effects. (b) RTs priming effects at the short 100 ms SOA as a function of subblocks. (c) RTs priming effects at the long SOAs. Asterisks indicate statistically significant effects (p < 0.05).
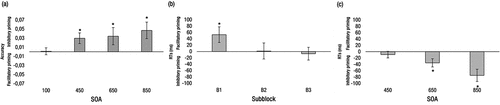
RTs analysis
The results are shown in (short SOA) and (long SOAs). For the 100-ms SOA, the main effect of subblock was significant, F(2, 46) = 3.54, p = 0.04, ηp2 = .07, showing that RTs were longer in the first subblock (M = 784 ms) than in the second and third subblocks (M = 734 and 746 ms, respectively). However, neither the main effect of relatedness nor the relatedness × subblock interaction were significant, (all ps > .15). However, an inspection to reveals that the lack of priming may be due to the facilitatory effect observed in the first subblock of trials, which proved statistically significant (Mpriming = 53 ms), t(23) = 2.14, p = 0.04, d = .44, being counteracted by the lack of effect in the second subblock (Mpriming = 2 ms) (p = 0.95), and the non-significant trend for an inhibitory effect in the third subblock (Mpriming = −7 ms) (p = 0.74). For the long SOAs, the main effects of relatedness and SOA were significant, F(1, 23) = 10.22, p = 0.004, ηp2 = .31 and F(2, 46) = 10.50, p < 0.001, ηp2 = .31, respectively. The relatedness effect showed that, in general, the participants were faster in unrelated (M = 656 ms) than in related trials (M = 695 ms) and the effect of SOA reflected that RTs decreased as SOA increased (M = 691, 669, and 667 ms, for the 450-, 650- and 850-ms SOAs, respectively), In addition, a significant relatedness × SOA interaction was also found, F(2, 46) = 4.24, p = 0.02, ηp2 = .16, which reflected a change in the priming effect across the SOAs. Further interaction analyses showed the progressive development of an inhibitory priming effect. Thus, with the 450-ms SOA, priming effects (M = −9 ms) were not yet significant, t(23) =.71, p = 0.48, d = .14, but inhibitory priming proved to be statistically significant at 650-ms SOA, (M = −35 ms), t(23) = 2.25, p = 0.034, d = .46, and 850-ms SOA (−75 ms), t(23) = 3.85, p < 0.001, d = .78.
An inspection of suggests that intermediate-type participants showed automatic processing at the very beginning of the experimental session, apparent only in the first subblock of the short SOA block. The lack of priming effects in both the two later subblocks of the short SOA block and the 450-ms SOA of the long SOA block suggests that automatic processing dissipated, and controlled processing did not emerge along that interval. With longer prime-target SOAs, only controlled processing took place, fostering the development of appropriate expectations concerning the category of the forthcoming target. This interplay between automatic and controlled processing as a function of prime-target SOA suggests that the pattern of priming effects observed in intermediate-type participants constitutes an appropriate referent to assess the performance of extreme chronotype participants when testing occurs at both their optimal and non-optimal times of day.
Experiment 2: extreme chronotypes
Psychomotor Vigilance Task (PVT)
Mean RTs were subjected to a mixed ANOVA with time-of-day (optimal and non-optimal) as the within-participants factor and chronotype (morning-types and evening-types) as the between-participants factor. The main effect of time-of-day was statistically significant, F(1, 38) = 5.61; p = 0.02; ηp2 = .13, which indicated that, in general, performance at the optimal time produced shorter RTs (M = 319 ms) than at the non-optimal time (M = 341 ms). The difference in performance between the optimal and non-optimal time-of-day according to the different chronotypes is referred to as the synchrony effect, and the current results replicate those obtained in other experiments using the same task (Correa et al. Citation2014; Lara et al. Citation2014; Martínez-Pérez et al. Citation2022). Accordingly, the current synchrony effect confirms the appropriate selection of our sample of extreme chronotypes. No other effects or interactions were significant (all ps > .31).
Category semantic priming task
As with intermediate-types, statistical analyses were conducted separately for the 100-ms SOA and the long SOAs. For the 100-ms SOA, data were subjected to 2 × 2 × 3 × 2 mixed ANOVAs with relatedness (related and unrelated), time-of-day (optimal and non-optimal), and subblock (first, second, and third) as within-participants factors, and chronotype (morning- and evening-types) as the between-participants factor. For the long SOAs, data were subjected to 2 × 2 × 3 × 2 mixed ANOVAs with relatedness (related and unrelated), time-of-day (optimal and non-optimal) and prime-target SOA (450, 650, and 850 ms) as within-participants factors, and chronotype (morning- and evening-types) as the between-participants factor.
Accuracy analysis
For the 100-ms SOA, only the relatedness × chronotype interaction reached statistical significance, F(1, 38) = 5.80, p = 0.021, ηp2 = .13. The interaction analysis proved that only evening-types showed significant facilitatory priming (M = −.016), F(1, 19) = 7.23, p = 0.01, ηp2 = .28, indicating automatic processing. No other effects were statistically significant in either morning- or evening-types. For the long SOAs, the main effect of relatedness was significant, F(1, 38) = 14.78, p < 0.001, ηp2 = .28, indicating better accuracy in unrelated trials (M = .97) than in related trials (M = .92). The relatedness × SOA interaction was also significant, F(2, 76) = 4.12, p = 0.02, ηp2 = .10, indicating that inhibitory priming effects were significant for each SOA, but it was larger for the longest SOA (M = .031, t(43) = 3.07, p = 0.004, d = .46; M = .031, t(43) = 3.08, p = 0.004, d = .46; and M = .051, t(43) = 3.60, p < 0.001, d = .54, for the 450-, 650-, and 850-ms SOAs, respectively). The time-of-day × SOA × chronotype interaction was only marginally significant, F(2, 76) = 2.78, p = 0.068, ηp2 = .07. However, these effects were qualified by the significant relatedness × time-of-day × SOA × chronotype interaction, F(2, 76) = 3.13, p = 0.05, ηp2 = .08. This four-way interaction means that in morning-types the differences in inhibitory priming effects between the optimal and non-optimal time of day (i.e. the synchrony effect), were only observed with 850-ms SOA (M = .042), t(19) = 2.78, p = 0.01, d = .62, while in evening-types, despite being the priming effects observed in all SOA values at the optimal time (M = .043, .056, and .072, for the 450-, 650- and 850-ms SOAs, respectively), and at 850-ms SOA at the non-optimal time (M = .061), they were not affected by the time of day in any case (all ps > .10).
RTs analysis
For the 100-ms SOA, none of the main effects nor the interactions were significant (all ps > .05). For the long SOAs, we observed a significant main effect of relatedness, F(1, 38) = 15.30, p < 0.001, ηp2 = .29. That is, unrelated trials produced shorter RTs (M = 660 ms) than related trials (M = 690 ms), showing an inhibitory priming effect. Also, the main effect of SOA was found to be statistically significant, F(2, 76) = 22.19, p < 0.001, ηp2 = .37. The RTs became shorter as SOA increased (M = 694, 672, and 658 ms, for the 450-, 650- and 850-ms SOAs, respectively). The main effects of time-of-day and chronotype and the relatedness × time-of-day interaction were not significant (all ps > .10). Importantly, we observed a significant relatedness × time-of-day × SOA interaction, F(2, 76) = 5.62, p = 0.005, ηp2 = .13. This three-way interaction indicated that the priming effects were affected by the time of testing (synchrony effect) and varied as a function of SOA. However, contrary to the accuracy analysis, the interaction involving relatedness, time-of-day, SOA, and chronotype was not significant, F < 1.
The lack of a significant four-way interaction in the RTs analysis deserves further comment. Regarding priming, the differences between the two chronotypes were expected to be subtle rather than extreme (i.e. mainly in the time when the effects emerge). Importantly, previous studies have shown that the differences in performance between the optimal and non-optimal times of day are only pronounced for evening-types, while a greater stabilization of performance throughout the day is only found in morning-types (Lara et al. Citation2014; Martínez-Pérez et al. Citation2020; Palmero et al. Citation2022). Thus, it should be expected differences in the emergence and time course of controlled priming effects between the two chronotypes to be mainly observed at the non-optimal time-of-day. This is further supported by the significant relatedness × SOA × chronotype interaction when performance was assessed only at the non-optimal time-of-day, F(2, 76) = 3.40, p = 0.039, ηp2 = .082. Thus, we consider that there is a strong case for separate analyses for each chronotype to assess both the emergence and time course of automatic and controlled processing in both accuracy and RT data. Additionally, this non-optimal time analysis also revealed two significant main effects of SOA, F(2, 76) = 12.68, p < 0.001, ηp2 = .25, indicating a significant decrease in RTs as SOA increased (MRTs = 714 ms, MRTs = 687 ms, and MRTs = 676 ms, for the 450-, 650- and 850-ms SOAs, respectively), and relatedness, F(1, 38) = 5.66 p = 0.02, ηp2 = .13, showing faster responses in unrelated trials (M = 681 ms) than in related trials (M = 703 ms).
For the 100-ms SOA, given that subblock did not show any effect either as a main effect or in the interaction with the other factors, that factor was omitted in the accuracy and RTs analyses for each chronotype. Thus, accuracy and RTs data were subjected to 2 × 2 repeated measures ANOVAs with relatedness (related and unrelated) and time-of-day (optimal and non-optimal) as within-participants factors. For the long SOAs, data were subjected to 2 × 2 × 3 repeated-measures ANOVAs with relatedness (related and unrelated), time-of-day (optimal and non-optimal), and SOA (450, 650, and 850 ms) as within-participants factors.
Automatic and controlled processes in morning-type chronotype
Accuracy analysis
The results are shown in . For the 100-ms SOA, none of the main effects nor the interactions reached statistical significance (all ps > .30). For the long SOAs, we observed a significant main effect of relatedness, F(1, 19) = 6.90; p = 0.02; ηp2 = .27. That is, the proportion of correct responses was higher in unrelated (M = .98) than in related trials (M = .94). Moreover, the relatedness × time-of-day × SOA interaction was statistically significant, F(2, 38) = 4.67; p = 0.01; ηp2 = .20. The interaction was due to a significant synchrony effect (the difference in priming between the optimal and non-optimal time of day) only with the 850-ms SOA, t(19) = 2.79, p = 0.01, d = .62. At both 450- and 650-ms SOAs, the synchrony effects proved not to be statistically significant, t(19) = .28, p = 0.78, d = .06, and t(19) = 2.12, p = 0.13, d = .35, respectively.
Figure 3. Morning-type participants. Performance at the short (100 ms) and the long (450, 650, and 850 ms) SOAs during optimal and non-optimal times of day. (a) Accuracy priming effects. (b) RTs priming effects. Priming effects did not vary for the three subblocks of the 100-ms SOA; consequently, they are not shown. The letter “a” indicates marginally significant effects (p < 0.10), and asterisks indicate statistically significant effects (p < 0.05).
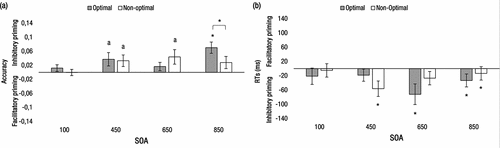
RTs analysis
The results are shown in . For the 100-ms SOA, neither the main effects nor the interactions reached statistical significance (all ps > 20). For the long SOAs, the main effects of relatedness and SOA were statistically significant, F(1, 19) = 8.32; p = 0.009; ηp2 = .30 and F(2, 38) = 12.38; p < 0.001; ηp2 = .39, respectively. RTs were longer for related (M = 750 ms) than for unrelated trials (M = 711 ms), reflecting an inhibitory priming effect. Also, RTs reflected a general decrease as SOA increased (M = 733, 707, and 694 ms, for the 450-, 650- and 850-ms SOAs, respectively). However, the relatedness × time-of-day × SOA interaction did not reach statistical significance, F(2, 38) = 2.74; p > 0.05; ηp2 = .13. Thus, we did not find any modulation of priming due to time of testing for the morning-type participants in the RTs analysis.
Automatic and controlled processes in evening-type chronotype
Accuracy analysis
The results are shown in . For the 100-ms SOA, we observed a main effect of relatedness, F(1, 19) = 7.23; p = 0.015; ηp2 = .28, indicating a higher proportion of correct responses in related (M = .97) than in unrelated trials (M = .95), reflecting automatic facilitatory priming. There were no other statistically significant main effects nor interactions (all ps > .40). For the long SOAs, we also observed a main effect of relatedness, F(1, 19) = 7.91; p = 0.01; ηp2 = .29, indicating higher proportion of correct responses in unrelated (M = .96) than in related trials (M = .91). There were no other statistically significant main effects nor interactions (all ps > .14).
Figure 4. Evening-type chronotype. Performance at the short (100 ms) and the long (450, 650, and 850 ms) SOAs during optimal and non-optimal times of day. (a) Accuracy priming effects. (b) RTs priming effects. Priming effects did not vary for the three subblocks of the 100-ms SOA; consequently, they are not shown. The letter “a” indicates marginally significant effects (p < 0.10), and asterisks indicate statistically significant effects (p < 0.05).
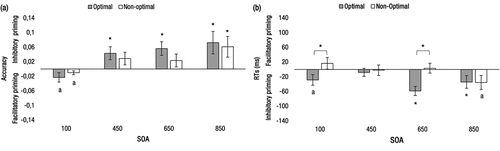
RTs analysis
The results are shown in . For the 100-ms SOA, only the relatedness × time-of-day interaction was marginally significant, F(1, 19) = 3.89; p = 0.06; ηp2 = .17. The analysis of the interaction showed a lack of facilitatory priming effects (M = 16 ms) at the non-optimal time of day, t(19) = 1.05, p = 0.31, d = .23, and a trend for inhibitory priming effects (M = −29 ms) at the optimal time of day, t(19) = 2.00, p = 0.06, d = .45. In order to test the statistical significance of this qualitative change in participants’ response trend (i.e. from facilitation at non-optimal times to inhibition at optimal times), we conducted a t-test contrasting the difference between both priming effects, which proved statistically significant, t(19) = 1.97, p = 0.03, d = .44.
For the long SOAs, we observed significant main effects of relatedness, F(1, 19) = 7.53; p = 0.013; ηp2 = .28, and SOA, F(2, 38) = 9.99; p < 0.001; ηp2 = .34. RTs were larger for related (M = 651 ms) than for unrelated trials (M = 628 ms) and decreased as SOA increased (M = 656, 639, and 623 ms, for the 450–650- and 850-ms SOAs, respectively). Importantly, the relatedness × time-of-day × SOA interaction reached statistical significance, F(2, 38) = 5.46; p = 0.008; ηp2 = .22. The three-way interaction analysis showed that at the optimal time of day, significant inhibitory priming was observed at both the 650-ms SOA, t(19) = 4.74, p < 0.001, d = 1.06, and the 850-ms SOA, t(19) = 2.01, p = 0.05, d = .45. At the non-optimal time of day, no priming was observed at any SOA value (ps > .05). However, at the 850-ms SOA it was marginally significant (p = 0.08).
Discussion
It is well established that automatic processes occur without the need to invest cognitive resources, whereas controlled processes require cognitive control. A key question is whether both types of processes can be modulated by the time of testing in extreme chronotypes, that is, whether the development of attention-based strategies can be different by the fact that people perform cognitive tasks at the optimal or non-optimal time of day according to their chronotypes. Although the influence of chronotype and time of day on automatic and controlled processing has been addressed separately in most studies, it is important to note that both processes coexist in most of our daily activities, and the interplay between them gives us the ability to deal with relatively complex demands. Thus, in the present study, we used a single-category semantic priming task in which a high proportion of unrelated prime-target pairs aimed to promote control-based expectancies. Moreover, the variation of time intervals (i.e. SOAs) between the prime and target respective appearances, allowed us to assess with finer detail the chronotype influence on the emergence and time course of automatic and controlled processing.
In Experiment 1, the pattern obtained with intermediate-chronotypes, which represent the largest part of the population (Roenneberg et al. Citation2007), allowed us to verify that our task was sensitive to capturing both automatic and controlled processing. Thus, at short SOAs, the prime stimulus activated the semantic network automatically, and the participants showed facilitatory priming. However, an automatic influence was apparent only with low experience with the task since the facilitatory effect vanished quickly (after the first subblock of trials) as the task progressed. A transition period occurred after the first subblock of trials in which automatic activation dissipated, and the controlled process was not developed enough to produce inhibitory priming effects. The early emergence and dissipation of automatic processing may have been promoted by the instructions given to the participants at the beginning of the experimental session about the low rate of related trials and the emphasis on accuracy, which surely fostered the development of controlled processes. At longer prime-target SOAs, intermediate-type participants could progressively apply the controlled expectancy about the category of the forthcoming target. Accordingly, inhibitory priming effects increased as a function of prime-target SOA, indicating effective controlled processing. Thus, the results of Experiment 1 with intermediate-types confirm that our task could capture both processing styles, replicating those observed in some previous studies (Langley et al. Citation2008; Ortells et al. Citation2003).
In addition to the potential for dissociating automatic and controlled processes, the category semantic priming task used here proved to be sensitive to the effects of time of day, according to the results obtained with extreme-chronotypes, replicating related studies that used conflict attentional paradigms such as the Stroop task (Schmidt et al. Citation2012). Based on the important differences that distinguish the two chronotypes regarding physiological and cognitive measures and how the two are differentially affected by the time of testing, we discuss the results observed in each group separately.
Concerning morning-type participants, their performance showed a trend for inhibitory priming even with a short prime-target interval (100 ms SOA), suggesting that they were adept at quickly disengaging their attention from the prime category to anticipate a target from the other semantic category. Moreover, their performance was not modulated as a function of the time of day, proving their ability to reverse automatic activation into controlled expectancies to be robust and unaffected by diurnal variations. Regarding the time course of controlled processing, we did not find any modulation by either the SOA length or the time of testing, as morning-type participants showed significant inhibitory priming effects in all conditions (although accuracy data showed a synchrony effect just at the longest SOA). This performance pattern leads us to infer that morning-types are consistent in their performance and minimally affected by the time of day. However, we estimate that this lack of time-of-day modulation of their performance deserves further explanation. In this sense, it has been suggested that circadian modulation of performance depends on the difficulty of the task (Lara et al. Citation2014; Manly Citation2002; May et al. Citation2005; Yang et al. Citation2007) such that synchrony effects usually appear in those tasks that demand high levels of cognitive resources. Therefore, notwithstanding the evident efficiency in controlled processing shown by morning-types it may be argued that the category semantic priming task used here was not truly challenging for this group of participants, so the implementation of control-based expectancies would have been relatively easy and remained constant at both the optimal and non-optimal time of day. This approach is directly supported by the absence of facilitatory priming effects at short SOAs and the large inhibitory priming effects observed at longer SOAs.
Concerning evening-types, despite the small facilitatory priming effects usually observed in this type of task, a time-of-day modulation of the time course of automatic processing occurred in these participants in the RTs analysis. Although we must admit that such modulation is based on facilitatory priming that did not achieve statistical significance and inhibitory priming that was only marginally significant, the difference between the two priming effects was significant. Therefore, although with some caution, we suggest that the time of day influenced the mode of response executed by the evening-type participants. This result marks a qualitative difference from morning-types, whose performance was always guided by effectively applying the controlled strategy. As far as we know, this is the first evidence of such modulation by chronotype and time of day.
Concerning controlled processing, we observed that evening-types were considerably affected by the time of testing in implementing controlled expectancies. Note that at the optimal time of day, inhibitory priming effects appeared at 650 ms SOA onwards, whereas at the non-optimal time, priming was no longer significant (although it was marginally significant at the longer 850 ms SOA). These results show that these participants, at the non-optimal time, could not implement the controlled expectancy even with the longest interval allowed. However, the fact that the inhibitory priming effect was marginally significant at that long interval suggests that they might require more time for the controlled expectative to be fully implemented. Future studies manipulating longer intervals than those in the present study may probe the plausibility of such a suggestion.
The results obtained in the present study reveal a dissociation between the magnitude of the effect based on controlled processes (i.e. inhibitory priming effects) and the difficulty of the task. Theories on which this study was based have pointed out that time-of-day modulation occurs most explicitly in tasks that require the involvement of controlled processing. However, we found that the strongest evidence of controlled processing (i.e. occurring in morning-type individuals throughout almost the whole task) was paralleled by the absence of time-of-day modulation. Nevertheless, evening-type participants, whose performance reflected less controlled processing, were more negatively influenced by the time of day in both automatic and controlled processing than morning-type participants. This pattern of results suggests that the difficulty of the task varied for the two extreme chronotypes, being harder for the evening-types than for the morning-types.
In this vein, it is also important to address the qualitative differences found in the performance of the category semantic priming task between the two extreme chronotypes. Our results align with studies suggesting better adaptation by morning-types at non-optimal times (Lara et al. Citation2014; Martínez-Pérez et al. Citation2020; Palmero et al. Citation2022). However, we go further by suggesting that it is plausible that their cognitive-control styles may be substantially different. Thus, following Braver’s (Citation2012) theory on the dual-mechanism framework of cognitive control, the proactive control style refers to the maintenance of active response strategies so that the individual can anticipate the occurrence of a conflict or a cognitively demanding event and resolve it without producing noticeable negative effects. On the other hand, the reactive control mode is related to a “late correction,” which suggests that the conflict resolution takes place once it has occurred and has been detected. Both styles are variable at the intraindividual level, but interestingly, it has been suggested that there are differences at the interindividual level, which places cognitive control as an individual trait driving the way different people deal with and resolve tasks that demand cognitive control. In this sense, the response style observed in the evening-types may be associated to a greater extent with the reactive style, while the consistent application of attention-based cognitive control from the very beginning of the task by the morning-types might constitute evidence of a proactive style of response in these individuals. In addition, the development of reactive control strategies has been linked with anxiety traits (Fales et al. Citation2008), to which evening-types are selectively associated (Antypa et al. Citation2016). The study of cognitive control styles in morning- and evening-types constitutes a line of research that deserves further attention.
Limitations and future directions
The present study has certain limitations that need to be mentioned. The main conclusions regarding the different pattern of results shown by the two extreme chronotypes should be taken with caution, as when entering chronotype as a between-participants factor in the overall ANOVAs, the four-way interaction was statistically significant only with the accuracy data but not with the RTs. However, further analysis with RTs showed that the interaction was significant when performance was assessed only at the non-optimal time of day, probably because morning-types showed greater performance stabilization throughout the day than evening-types.
A second limitation is that our task could not capture large automatic semantic priming effects (see Langley et al. Citation2008), which may have made it challenging to observe a clearer circadian modulation of automatic processing. Instructions about the low rate of related trials and the emphasis on accuracy might have promoted the activation of controlled processing earlier than in previous studies.
A third limitation concerns the split of the 100-ms SOA into three subblocks of trials. Although the interaction between relatedness and subblock was not statistically significant, likely due to the small number of trials in each subblock, the simple main effect analyses confirmed that the facilitatory priming effect had already dissipated in the second subblock. Consequently, the priming results in these analyses should be taken with caution. However, we consider it essential to include this analysis so that future studies may address this issue with more trials per condition and more appropriate tasks to tap automatic processing, as the present results with the shortest SOA suggest that the time course of automatic processing can differ significantly with time-on-task and time of testing in each chronotype.
Finally, more information regarding sleep in our sample, such as sleep quality and the total hours of sleep the night before the experiment, would have allowed for a more detailed explanation of the main differences observed between the two chronotypes. In this sense, as hours of sleep have previously been connected to automatic and controlled processing (for some examples, see Harrison and Horne Citation1999; Horne Citation1993), a further leap linking this circadian rhythms variable with both automatic and controlled processing would be paramount.
Conclusions
The present results are framed within the literature that has studied the modulation of cognitive processes by chronotype and time of day. Although it has usually been considered that only controlled processing is modulated by time of day, our study reflects a qualitative distinction between the two extreme chronotypes not only in controlled processing but also in automatic processing. Thus, while morning-types were able to apply control quickly and easily to reverse the automatic process, evening-types were more influenced by the time of day, so that they could reverse the automatic process only at the optimal time of day. This pattern of results points to the need to further study the differences between the extreme chronotypes and to consider them as distinct groups, given that their cognitive style of functioning seems to be different. In addition, we highlight the need to understand circadian modulations based on the difficulty experienced by individuals in performing the task, which directly connects to their unique cognitive traits.
Authors’ contributions
LBP: Conceptualization, Methodology, Investigation, Software, Formal analysis, Data curation, Writing – original draft, Visualization MT: Methodology, Investigation, VMP: Methodology, Investigation ASL: Methodology, Investigation GC: Conceptualization, Methodology, Software, Supervision LJF: Conceptualization, Writing-Original draft preparation, Writing-Reviewing and Editing, Supervision, Visualization, Funding acquisition. All authors read and approved the final manuscript.
Ethics approval and consent to participate
Written informed consent was obtained from all participants prior to their participation. Data obtained from participants have been fully anonymized under their acknowledgment. The study was approved by the Ethics Committee of the University of Murcia and was carried out in accordance with the ethical standards of the Declaration of Helsinki.
Supplemental Material
Download MS Excel (20.5 KB)Disclosure statement
No potential conflict of interest was reported by the author(s).
Data availability statement
The dataset generated and analyzed during the current study as well as the R scripts used to preprocess the data are available in OSF repository (peer-review link): https://osf.io/kjhna/?view_only=16bc0c55949d4fa5ad800c60e0a692ec.
Supplementary material
Supplemental data for this article can be accessed online at https://doi.org/10.1080/07420528.2024.2312806.
Additional information
Funding
References
- Adan A, Almirall H. 1991. Horne & Östberg morningness-eveningness questionnaire: a reduced scale. Pers Indiv Differ. 12:241–253. doi: 10.1016/0191-8869(91)90110-W.
- Adan A, Archer SN, Hidalgo MP, Di Milia L, Natale V, Randler C. 2012. Circadian typology: a comprehensive review. Chronobiol Int. 29:1153–1175. doi: 10.3109/07420528.2012.719971.
- Antypa N, Vogelzangs N, Meesters Y, Schoevers R, Penninx BWJH. 2016. Chronotype associations with depression and anxiety disorders in a large cohort study: research article: chronotypes in depression and anxiety. Depress Anxiety. 33:75–83. doi: 10.1002/da.22422.
- Balota DA, Black SR, Cheney M. 1992. Automatic and attentional priming in young and older adults: reevaluation of the two-process model. J Exp Psychol Hum Percept Perform. 18:485–502. doi: 10.1037//0096-1523.18.2.485.
- Becker CA. 1980. Semantic context effects in visual word recognition: an analysis of semantic strategies. Mem Cognit. 8:493–512. doi: 10.3758/BF03213769.
- Besner D, Humphreys GW. 1991. Basic processes in reading: Visual word recognition. L. Erlbaum Associates. http://site.ebrary.com/id/10630126.
- Blatter K, Cajochen C. 2007. Circadian rhythms in cognitive performance: methodological constraints, protocols, theoretical underpinnings. Physiol Behav. 90:196–208. doi: 10.1016/j.physbeh.2006.09.009.
- Bocanegra BR, Hommel B. 2014. When cognitive control is not adaptive. Psychol Sci. 25:1249–1255. doi: 10.1177/0956797614528522.
- Braver TS. 2012. The variable nature of cognitive control: a dual mechanisms framework. Trends Cogn Sci. 16:106–113. doi: 10.1016/j.tics.2011.12.010.
- Cheng W-J, Puttonen S, Vanttola P, Koskinen A, Kivimäki M, Härmä M. 2021. Association of shift work with mood disorders and sleep problems according to chronotype: a 17-year cohort study. Chronobiol Int. 38:518–525. doi: 10.1080/07420528.2021.1885431.
- Collins AM, Loftus EF. 1975. A spreading-activation theory of semantic processing. Psychol Rev. 82:407–428. doi: 10.1037/0033-295X.82.6.407.
- Correa Á, Molina E, Sanabria D. 2014. Effects of chronotype and time of day on the vigilance decrement during simulated driving. Accident Anal Prev. 67:113–118. doi: 10.1016/j.aap.2014.02.020.
- Daza MT, Ortells JJ, Fox E. 2002. Perception without awareness: further evidence from a Stroop priming task. Percept Psychophys. 64:1316–1324. doi: 10.3758/BF03194774.
- Di Milia L, Smolensky MH, Costa G, Howarth HD, Ohayon MM, Philip P. 2011. Demographic factors, fatigue, and driving accidents: an examination of the published literature. Accident Anal Prev. 43:516–532. doi: 10.1016/j.aap.2009.12.018.
- Duffy JF, Rimmer DW, Czeisler CA. 2001. Association of intrinsic circadian period with morningness–eveningness, usual wake time, and circadian phase. Behav Neurosci. 115:895–899. doi: 10.1037/0735-7044.115.4.895.
- Facer-Childs ER, Campos BM, Middleton B, Skene DJ, Bagshaw AP. 2019. Circadian phenotype impacts the brain’s resting-state functional connectivity, attentional performance, and sleepiness. Sleep. 42:zsz033. doi: 10.1093/sleep/zsz033.
- Facer-Childs ER, Middleton B, Skene DJ, Bagshaw AP. 2019. Resetting the late timing of ‘night owls’ has a positive impact on mental health and performance. Sleep Med. 60:236–247. doi: 10.1016/j.sleep.2019.05.001.
- Fales CL, Barch DM, Burgess GC, Schaefer A, Mennin DS, Gray JR, Braver TS. 2008. Anxiety and cognitive efficiency: differential modulation of transient and sustained neural activity during a working memory task. Cogn Affect Behav Neurosci. 8:239–253. doi: 10.3758/CABN.8.3.239.
- Fisk AD, Schneider W. 1981. Control and automatic processing during tasks requiring sustained attention: a new approach to vigilance. Hum Factors. 23:737–750. doi: 10.1177/001872088102300610.
- Ghin F, Stock A-K, Beste C. 2022. The importance of resource allocation for the interplay between automatic and cognitive control in response inhibition – an EEG source localization study. Cortex. 155:202–217. doi: 10.1016/j.cortex.2022.07.004.
- Goldin AP, Sigman M, Braier G, Golombek DA, Leone MJ. 2020. Interplay of chronotype and school timing predicts school performance. Nat Hum Behav. 4:387–396. doi: 10.1038/s41562-020-0820-2.
- Harrison Y, Horne JA. 1999. One night of sleep loss impairs innovative thinking and flexible decision making. Organ Behav Hum Decis Process. 78:128–145. doi: 10.1006/obhd.1999.2827.
- Horne JA. 1993. Human sleep, sleep loss and behaviour: Implications for the prefrontal cortex and psychiatric disorder. Brit J Psychiat. 162:413–419. doi: 10.1192/bjp.162.3.413.
- JASP Team. 2022. JASP (Version 0.16.3)[Computer software]. [Software]. https://jasp-stats.org/.
- Kerkhof GA, Van Dongen HPA. 1996. Morning-type and evening-type individuals differ in the phase position of their endogenous circadian oscillator. Neurosci Lett. 218:153–156. doi: 10.1016/S0304-3940(96)13140-2.
- Langley LK, Saville AL, Gayzur ND, Fuentes LJ. 2008. Adult age differences in attention to semantic context. Aging Neuropsychol Cognit. 15:657–686. doi: 10.1080/13825580802036928.
- Lara T, Madrid JA, Correa Á, van Wassenhove V. 2014. The vigilance decrement in executive function is attenuated when individual chronotypes perform at their optimal time of day. PLoS One. 9:e88820. doi: 10.1371/journal.pone.0088820.
- Lerner I, Bentin S, Shriki O. 2014. Integrating the automatic and the controlled: strategies in semantic priming in an attractor network with latching dynamics. Cogn Sci. 38:1562–1603. doi: 10.1111/cogs.12133.
- Levandovski R, Sasso E, Hidalgo MP. 2013. Chronotype: a review of the advances, limits and applicability of the main instruments used in the literature to assess human phenotype. Trends Psychiatry Psychother. 35:3–11. doi: 10.1590/S2237-60892013000100002.
- Manly T. 2002. Coffee in the cornflakes: time-of-day as a modulator of executive response control. Neuropsychologia. 40:1–6. doi: 10.1016/S0028-3932(01)00086-0.
- Martínez-Pérez V, Palmero LB, Campoy G, Fuentes LJ. 2020. The role of chronotype in the interaction between the alerting and the executive control networks. Sci Rep. 10:11901. doi: 10.1038/s41598-020-68755-z.
- Martínez-Pérez V, Tortajada M, Palmero LB, Campoy G, Fuentes LJ. 2022. Effects of transcranial alternating current stimulation over right-DLPFC on vigilance tasks depend on the arousal level. Sci Rep. 12:547. doi: 10.1038/s41598-021-04607-8.
- May CP, Hasher L. 1998. Synchrony effects in inhibitory control over thought and action. J Exp Psychol Hum Percept Perform. 24:363–379. doi: 10.1037//0096-1523.24.2.363.
- May CP, Hasher L. 2017. Synchrony affects performance for older but not younger neutral-type adults. Timing Time Percept. 5:129–148. doi: 10.1163/22134468-00002087.
- May CP, Hasher L, Foong N. 2005. Implicit memory, age, and time of day: paradoxical priming effects. Psychol Sci. 16:96–100. doi: 10.1111/j.0956-7976.2005.00788.x.
- McNamara TP. 2005. Semantic priming: Perspectives from memory and word recognition. Hove, England: Psychology Press. doi: 10.4324/9780203338001.
- Merikle PM, Joordens S. 1997. Parallels between perception without attention and perception without awareness. Conscious Cogn. 6:219–236. doi: 10.1006/ccog.1997.0310.
- Meyer DE, Schvaneveldt RW. 1971. Facilitation in recognizing pairs of words: evidence of a dependence between retrieval operations. J Exp Psychol. 90:227–234. doi: 10.1037/h0031564.
- Miller EK, Cohen JD. 2001. An integrative theory of prefrontal cortex function. Annu Rev Neurosci. 24:167–202. doi: 10.1146/annurev.neuro.24.1.167.
- Miller JD, Morin LP, Schwartz WJ, Moore RY. 1996. New insights into the mammalian circadian clock. Sleep. 19:641–667. doi: 10.1093/sleep/19.8.641.
- Molina E, Sanabria D, Jung T-P, Correa Á. 2019. Electroencephalographic and peripheral temperature dynamics during a prolonged psychomotor vigilance task. Accident Anal Prev. 126:198–208. doi: 10.1016/j.aap.2017.10.014.
- Neely JH. 1977. Semantic priming and retrieval from lexical memory: roles of inhibitionless spreading activation and limited-capacity attention. J Exp Psychol. 106:226–254. doi: 10.1037/0096-3445.106.3.226.
- Neely JH, Keefe DE, Ross KL. 1989. Semantic priming in the lexical decision task: roles of prospective prime-generated expectancies and retrospective semantic matching. J Exp Psychol Learn Mem Cogn. 15:1003–1019. doi: 10.1037//0278-7393.15.6.1003.
- Neely JH, O’Connor PA, Calabrese G. 2010. Fast trial pacing in a lexical decision task reveals a decay of automatic semantic activation. Acta Psychol. 133:127–136. doi: 10.1016/j.actpsy.2009.11.001.
- Oginska H, Fafrowicz M, Golonka K, Marek T, Mojsa-Kaja J, Tucholska K. 2010. Chronotype, sleep loss, and diurnal pattern of salivary cortisol in a simulated daylong driving. Chronobiol Int. 27:959–974. doi: 10.3109/07420528.2010.489412.
- Orban C, Kong R, Li J, Chee MWL, Yeo BTT. 2020. Time of day is associated with paradoxical reductions in global signal fluctuation and functional connectivity. ne Biol. 18:e3000602. doi: 10.1371/journal.pbio.3000602.
- Ortells JJ, Daza MT, Fox E. 2003. Semantic activation in the absence of perceptual awareness. Percept Psychophys. 65:1307–1317. doi: 10.3758/BF03194854.
- Palmero LB, Martínez-Pérez V, Tortajada M, Campoy G, Fuentes LJ. 2022. Mid-luteal phase progesterone effects on vigilance tasks are modulated by women’s chronotype. Psychoneuroendocrinology. 140:105722. doi: 10.1016/j.psyneuen.2022.105722.
- Palmero LB, Martínez-Pérez V, Tortajada M, Campoy G, Fuentes LJ. 2024. Testing the modulation of self-related automatic and others-related controlled processing by chronotype and time-of-day. Conscious Cogn. 118:103633. doi: 10.1016/j.concog.2023.103633.
- Psychology Software Tools, Pittsburgh, PA. 2016. Psychology software tools, inc. [E-Prime 3.0]. [ Software]. https://support.pstnet.com/.
- R Core Team. 2022. A language and environment for statistical computing. R Foundation for Statistical Computing, Vienna, Austria. [ Software]. https://www.R-project.org/.
- Roenneberg T, Kuehnle T, Juda M, Kantermann T, Allebrandt K, Gordijn M, Merrow M. 2007. Epidemiology of the human circadian clock. Sleep Med Rev. 11:429–438. doi: 10.1016/j.smrv.2007.07.005.
- Salehinejad MA, Wischnewski M, Ghanavati E, Mosayebi-Samani M, Kuo M-F, Nitsche MA. 2021. Cognitive functions and underlying parameters of human brain physiology are associated with chronotype. Nat Commun. 12:4672. doi: 10.1038/s41467-021-24885-0.
- Sarabia JA, Rol MA, Mendiola P, Madrid JA. 2008. Circadian rhythm of wrist temperature in normal-living subjects. Physiol Behav. 95:570–580. doi: 10.1016/j.physbeh.2008.08.005.
- Schmidt C, Collette F, Cajochen C, Peigneux P. 2007. A time to think: circadian rhythms in human cognition. Cogn Neuropsychol. 24:755–789. doi: 10.1080/02643290701754158.
- Schmidt C, Peigneux P, Leclercq Y, Sterpenich V, Vandewalle G, Phillips C, Berthomier P, Berthomier C, Tinguely G, Gais S, et al. 2012. Circadian preference modulates the neural substrate of conflict processing across the day. PLoS One. 7:e29658. doi: 10.1371/journal.pone.0029658.
- Schneider W, Eschman A, Zuccolotto A. 2012. E-Prime user’s guide. Pittsburgh: Psychology Software Tools, Inc. [ Software].
- Schneider W, Shiffrin RM. 1977. Controlled and automatic human information processing: I. Detection, search, and attention. Psychol Rev. 84:1–66. doi: 10.1037/0033-295X.84.1.1.
- Venkat N, Sinha M, Sinha R, Ghate J, Pande B. 2020. Neuro-cognitive profile of morning and evening chronotypes at different times of day. Ann Neurosci. 27:257–265. doi: 10.1177/0972753121990280.
- Walsh NA, Repa LM, Garland SN. 2022. Mindful larks and lonely owls: the relationship between chronotype, mental health, sleep quality, and social support in young adults. J Sleep Res. 31. doi: 10.1111/jsr.13442.
- Wolff N, Chmielewski W, Buse J, Roessner V, Beste C. 2019. Paradoxical response inhibition advantages in adolescent obsessive compulsive disorder result from the interplay of automatic and controlled processes. NeuroImage Clin. 23:101893. doi: 10.1016/j.nicl.2019.101893.
- Yang L, Hasher L, Wilson DE. 2007. Synchrony effects in automatic and controlled retrieval. Psychon Bull Rev. 14:51–56. doi: 10.3758/BF03194027.