Abstract
Kleinheinz, G., A. Coenan, T. Zehms, J. Preedit, M.C. Leewis, D. Becker and C. McDermott. 2009. Effect of aquatic macrophytes on the survival of Escherichia coli in a laboratory microcosm.
Recreational beaches are very important as engines for tourism revenue in many areas of Wisconsin, United States, and microbial contamination of beach water can be very costly. Escherichia coli (E. coli) is the indicator organisms used in the Great Lakes region of the United States for recreational water monitoring. The overall objective of this study was to determine if aquatic macrophytes (Sagittaria sp., Myriophyllum sp.) allow for prolonged E. coli survival in the environment by using a laboratory microcosm as a test system. This study tested whether E. coli survival was greater in lake water alone or in lake water containing a high density of macrophytes (HDM), a low density of macrophytes (LDM), or plastic plant material. Water and plant substrate samples were analyzed for E. coli concentrations. ANOVA analysis indicated a significant difference between all treatments (p < 0.001). The Scheffe test revealed a significant difference between E. coli concentrations in water in the HDM treatment and the plastic plants (p = 0.003) and the controls (lake water only; p < 0.001), as well as differences between the LDM treatments and the plastic plants (p < 0.001) and the controls (p < 0.001). Attachment of E. coli to the macrophytes was rapid and may contribute to the rapid decline of E. coli found in the microcosm water samples. The presence of aquatic macrophytes did not appear to provide growth factors or other substances that could prolong the survival of E. coli in water, but the plants may contribute to bacterial survival by providing a medium for bacterial attachment. To our knowledge this is the first study reporting on the effects of aquatic macrophytes on E coli concentrations in water.
Recreational beaches play a large role in tourism revenues generated for many areas of Wisconsin, especially in the northern parts of the state. Beach closures due to microbial contaminations can be very costly for these areas because many people depend on tourism income for their livelihoods (CitationRabinovici et al. 2004) The indicator organism for fecal contamination events most often used by local governments is Escherichia coli (E. coli) because high levels of this organism may indicate a recent contamination event containing pathogenic bacteria (e.g., Shigella, Salmonella), viruses (e.g., Norovirus), and/or protozoans (e.g., Giardia or Cryptosporidium; (CitationByappanahalli et al. 2003; CitationKinzelman et al. 2003). The U.S. Environmental Protection Agency (USEPA) recommends that water samples should not exceed a single sample limit of 235 E. coli per 100 mL of water (USEPA 1986). The Wisconsin Department of Natural Resources (WI DNR) uses a two-tiered water quality criterion for recreational waters. Samples with E. coli concentrations between 235 and 999 most probable number (MPN)/100mL water result in a poor water quality advisory, and samples with E. coli concentrations > 1000 MPN/100mL result in a beach closure (WI DNR 2001). Both remain in place until measured E. coli concentrations fall below these standards.
Several counties in Wisconsin have more than 1000 inland lakes within their borders, and a problem seen at many of the lakes is thick stands or mats of native aquatic macrophytes (e.g. Potamogeton spp., Elodea canadensis), or invasive species macrophytes (e.g. Myriophyllum spicatum). Unfortunately, mats of these invasives are becoming increasingly common and have become associated with poor water quality issues. Great Lakes beaches can be similarly affected by aquatic macrophytes, although accumulations of nuisance algal species (e.g. Cladophora) have currently received much attention. Recently, researchers have suggested a link between the nuisance alga Cladophora and elevated concentrations of E. coli (CitationByappanahalli et al. 2003; CitationWhitman et al. 2003). No published study, however, has demonstrated a direct link between mats of Cladophora and beach closures. Mats of Cladophora are believed to offer a stable environment for bacteria, shielding them from such things as UV radiation, changes in pH, desiccation, and nutrient depletion (CitationByappanahalli et al. 2003, CitationWhitman et al. 2003). Similarities can be noted between mats of Cladophora and mats of aquatic macrophytes (CitationBaker and Orr 1986). Both mats provide a favorable secondary bacterial habitat, consisting of increased nutrients, protection from the sun, an attachment point for growth, and a stable temperature. Deposition of bacteria within these mats may allow for bacterial survival or even bacterial replication. An increased amount of the indicator organism, E. coli, within and near macrophyte mats could challenge the paradigm that E. coli is indicative of a recent fecal contamination event at beaches.
Our objective was to determine if aquatic macrophytes allow for prolonged E. coli survival in the environment by using a laboratory microcosm as a test system. Specifically, the study was designed to test whether E. coli survival was greater in lake water alone or in lake water containing living or artificial plant material. In addition, adherence of E. coli to plant material was determined. To our knowledge this is the first study reporting on the effects of aquatic macrophytes on E. coli concentrations in water.
Methods
Microcosm set up
E. coli suspension preparation
Lake water from Menominee Park beach on Lake Winnebago, Oshkosh, Wisconsin, was collected on 28 December 2006 and used as the source of lake water for the microcosms. Background E. coli concentrations (determined by the ColilertR Defined Substrate method, described later) in the lake water used for the microcosms were 3 and < 1 most probable number (MPN), respectively. An E. coli isolate recovered from water (isolate number LS057) in Lake Superior during summer 2003 was used to inoculate the lake water microcosms. To prepare the microcosms' inocula, a 10-μ m loopful of the E. coli isolate was placed in 250 mL of nutrient broth and allowed to grow overnight at 37°C. The culture was then centrifuged at 8000 × gravity for 15 min, the supernatant discarded, and the pellet resuspended in 100 mL of PBW (Phosphate Buffered Saline). This mixture was then recentrifuged and resuspended two more times as described above.
Microcosm preparations and growth conditions
We placed 10 mL of a 10− 5 dilution of this culture into the microcosm, composed of a glass tank (30.5 × 15.2 × 20.3 cm; Aqua Clear, Hagen Industries, Montreal, Canada) containing 2.6 L of lake water. A recirculating pump was placed in all microcosms and set to recirculate the water for 30 min in each system twice a day. Duplicate microcosms were used for each treatment. The two high density macrophyte (HDM) microcosms received lake water and 140 g (± 1g) of plant material (wet wt); the low density macrophyte (LDM) microcosms received 66 g (± 1 g) of plant material; the plastic plant treatments received 70 g (± 1 g) of aquarium-grade plastic plants; and the controls contained only lake water. Plastic plants were used to differentiate between the effects of inanimate structures and live macrophytes on E. coli concentrations in the microcosm. In the high and low density plant material treatments, half consisted of Myriophyllum sp. and half of Sagittaria sp. (Carolina Biological Supply Co., Burlington, North Carolina, USA). Plants floated unrooted in the microcosms. The microcosms were held at ambient room temperature (22–23°C) and were exposed to a supplemental lighting regime. Briefly, six 40-watt, full spectrum light bulbs were placed 15 cm above the water within the microcosms. Lights were cycled on for 3 hr then off for 3 hr throughout each day of the experiment.
Laboratory analysis
E. coli analysis for water samples
Aliquots of water were removed from the microcosms daily to assess E. coli concentrations. Because of the relatively large number of E. coli cells originally added, dilution series (1/10, 1/100, 1/1000) using PBW were performed to enumerate bacterial concentrations. Lake water and diluted microcosm samples were analyzed for E. coli using the Colilert defined substrate (DS) testing protocol (IDEXX Corp., Portland, ME; APHA 2005). Incubation and microbial enumeration of samples were conducted following the manufacturer's recommendations. The University of Wisconsin – Oshkosh laboratory used for all analyses is a Wisconsin State-Certified Laboratory for coliform and E. coli analysis with a Quality Assurance plan on file with the Wisconsin State Department of Agriculture and Consumer Protection. All water results were reported as MPN of E. coli per 100 mL of water. Because dilutions of water samples were made, MPN values were adjusted for the dilution factor.
E. coli analysis for plant water
Macrophytes were tested for the presence of E. coli prior to the start of the project, and no E. coli was detected in any samples. In addition, E. coli adhering to live or artificial plant material also was determined. Detachment of E. coli from the surface of the plant material was conducted using the procedures described by CitationByappanahalli et al. 2003 and analyzed using the aforementioned defined substrate test. At each sampling event, approximately 1 g of plant material was removed from each microcosm containing either macrophytes or plastic plants. After E. coli detachment, each sample was dried at 55°C for 72 hr and reweighed, and all results were normalized for the dry weight of the plant sample and used to enumerate E. coli MPN/g of dry weight plant material.
Statistical analysis
Statistical analyses including analysis of variance (ANOVA) and Scheffe tests were performed with Systat version 11.0, and graphs were composed with Microsoft Excel. For all analyses the significance threshold was set at α = 0.05.
Results
All microcosms started with an E. coli loading of 9.83 log10MPN/100 mL of water. Neither live macrophytes nor artificial plants had detectable E. coli concentrations prior to addition to the microcosms. The two treatments with living macrophytes present showed the most rapid decline in E. coli concentrations (). The lake water-only control had detectable concentrations of E. coli for the greatest time period. The mean concentration of E. coli in the water with the high and low density macrophyte treatments declined to 3.67 and 3.59 log10MPN/100 mL, respectively, in only five days. Interestingly, it took the plastic plant treatments an average of 38 days to decline to a similar level, 3.58 log10MPN/100 mL. The mean of the lake-water–only control was 5.31 log10MPN/100 mL after 38 days. These trends are mirrored when rates of E. coli decline are calculated for the various treatments (). The ANOVA indicated a significant difference between all treatments (p < 0.001). The Scheffe test revealed no significant difference between the E. coli concentrations in water in HDM and LDM treatments (p = 0.840); however, the Scheffe test revealed a significant difference between E. coli concentrations in water in the HDM treatment and the plastic plants (p = 0.003) and the controls (p < 0.001), as well as differences between the LDM treatments and the plastic plants (p < 0.001) and the controls (p < 0.001). There was no significant difference between the plastic plant treatment and the control (p < 0.001). Using a paired t-test analysis, the rates of E. coli decline () were found to be significantly different between HDM treatments and LDM treatments (p = 0.019), plastic treatments (p < 0.001), and the control microcosms (p < 0.001). LDM treatments were significantly different from plastic treatments (p < 0.001) and control microcosms (p < 0.001). Finally, the control microcosms were found to be different from the plastic treatments (p < 0.001).
Figure 1 E. coli concentrations measured in lake water microcosms with and without the presence of aquatic macrophytes.
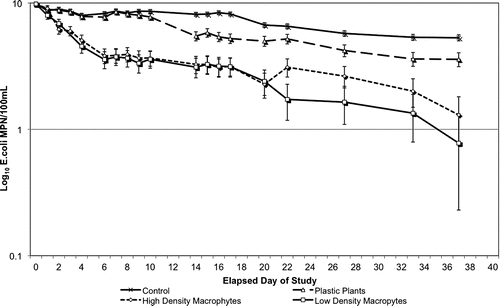
Table 1 Rate of E. coli decline during water sampling of the various microcosms.
To determine if the decline seen in E. coli concentrations was due to attachment of bacteria to substrates within the microcosm (live or plastic plants), plant material was washed of adherent microbes. There was more rapid and early colonization of the natural plant material than the plastic plant material (). After day five, however, and for the remainder of the study, the plastic plant material had greater concentrations of attached E. coli. When analyzing rates of decline in E. coli attached to the plant material (from peak colonization), the plastic plant showed very slow declines and the two natural plant treatments had virtually identical rates of decline that were greater than those for the plastic plants (). The attached E. coli ANOVA results do not suggest any significant differences between treatments (p = 0.098). The Scheffe test also revealed no significant differences between the plastic plants and either the HDM (p = 0.198) or LDM (p = 0.146) treatments. As expected, the Scheffe test found no significant difference between the LDM and HDM treatments (p = 0.984). Using a paired t-test analysis, the rates of E.coli decline () were found to be significantly different between LDM and plastic treatments (p = 0.019) and HDM and plastic treatments (p = 0.029); however, there was no significant difference between the LDM and HDM treatments (p = 0.212).
Figure 2 Adherent E. coli concentrations able to be washed/removed from macrophytes from lake water microcosms.
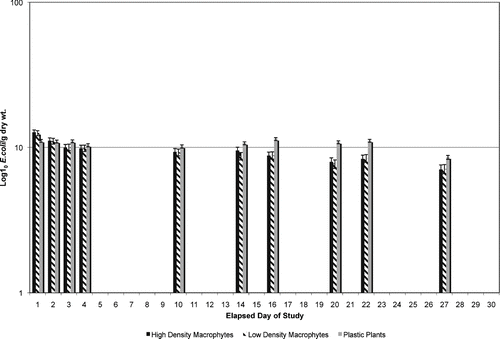
Table 2 Rate of E. coli decline after maximum attachment to plant material in microcosms.
Discussion
This study showed that E. coli was detectable in lake water alone longer than in lake water containing plants, either living or artificial. While numbers of viable E. coli in lake water alone initially decreased, they then remained relatively static for about two weeks, and then gradually declined until the end of the study (5 weeks; ). This indicates that E. coli can survive in lake water for relatively long periods with only modest reductions in numbers. Once live plants are added to the lake water, however, E. coli numbers in water declined rapidly and were substantially reduced from those found in lake water alone by the end of the study period. Addition of artificial plants to lake water microcosms resulted in reduction of E. coli numbers in water, less than that seen in microcosms with live plants but greater than in those with lake water alone (). This would indicate that addition of plants either caused the death of E. coli in lake water or (more likely) that E. coli was able to adhere to plant material; thus, a reduction in E. coli concentrations in “free water” seemed to occur. To investigate this question, E. coli adhering to plant material also were enumerated. The amount of E. coli that adhered to (i.e., can be washed off from) plant material was great (Fig 2). Both HDM and LDM (live plant) treatments resulted in similar adherent E. coli concentrations. Adhesion of E. coli to these live plants appeared to occur quickly but began to decline within about a week and continued to decline throughout the experimental period. Plastic plants were colonized by E. coli as well, but colonization occurred more slowly, and adherent E. coli numbers remained relatively constant throughout the experiment ().
Several hypotheses can account for the loss of adherent E. coli from the live plant material. First, E. coli may adhere loosely to the plant material and be released back into the “free” water over time. If this were true, however, the E. coli concentrations in “free” water would be expected to increase as amount of adherent E. coli decreased. This did not occur (). Likewise, adherent E. coli could be released from the plant material because bacteria were killed by plant defenses. Again, this is not a likely scenario because most toxic plant secondary metabolites are released only when the plant has been wounded by pathogens or by insects (CitationTaiz and Zeiger 2006). No obvious damage was noted on living plant material. A third possibility is that the E. coli in lake water adheres to biofilms that had previously formed on the plant material, or E. coli begins to form a biofilm, if none was previously present. This third possibility most easily explains the data from this study. Biofilm formation occurs as a step-wise process, with a slow attachment of bacteria to surfaces that previously are free of biofilm matrix (CitationWilley et al. 2008). This explains the slow attachment of E. coli to the artificial plant material, which was purchased new and lacked established biofilm formation on the plastic surfaces. Once the E. coli were able to adhere and begin biofilm formation, the number of organism that could be washed from the plastic surfaces remained constant throughout the experimental period.
Living plants, however, are different from artificial plants in many ways. Living aquatic plants have been grown under nonsterile conditions and likely are covered by an established biofilm. The presence of an existing biofilm matrix would make adherence of additional bacteria an easier and more rapid process (CitationWilley et al. 2008; ). Biofilms form on surfaces, and living plant material should have a far greater surface area (stems, leaves, and roots) than do artificial plants made of plastic. The presence of a previously established biofilm and a greater surface area in living plants could account for the rapid colonization by E. coli and for the decline in numbers of E. coli able to be washed from the living plant material. Once thick biofilms have formed on surfaces, ability to dislodge bacteria from the tenacious matrix should become difficult. The “apparent” reduction in E. coli adherent to the living plant material over time is more likely an inability to release the tightly adherent organisms from the well-established biofilm. On plastic plants, the biofilm is newly formed, and surface area to which the matrix is attached is less than that on living plants. This could account for the slower colonization and the ease of removal from the artificial material. Sand and other particulate beach substrates also have been shown to “hold and release” bacteria (CitationWhitman and Nevers 2003; CitationIshii et al. 2006), and may play a role in the elevation of E. coli concentrations in beach water without a recent fecal contamination.
Regardless of the mechanism by which E. coli numbers decreased in the lake water microcosms containing live or artificial plants, it seens that E. coli concentrations in free water are not being elevated when plant material is present. While it has been shown that algal blooms and sand (CitationByappanahalli et al. 2003, CitationWhitman et al. 2003, CitationWhitman and Nevers 2003) may harbor E. coli, allow for its survival and replication, and may release these organisms back into beach water (falsely indicating a recent fecal input to the water), this does not seem to be the case for aquatic macrophytes and E. coli. The presence of plant material, both living and artificial, in a lake water microcosm resulted in lower E. coli concentrations than found in a lake-water–only microcosm. While E. coli did adhere to the macrophytes, the plant material did not readily release the E. coli back into the lake water. Further studies should be conducted to examine this phenomenon in the natural lake environment. While microcosm water was mixed throughout the study, there was a lack of wind and wave action that can occur in nature. Extensive agitation of plant material in a natural lake system may act to dislodge bacteria from biofilms and could be a source of elevated E. coli concentrations in beach water.
Acknowledgments
Funding for this project was provided in-part by University of Wisconsin Sea Grant Program.
References
- APHA . 2005 . “ Microbiological Examination ” . In Standard methods for examination of water & wastewater, , 21st ed. , Edited by: Clescerl , L. S. , Greenberg , A. E. and Eaton , A. D. 9-72 – 9-74 . Washington , D. C. : American Public Health Association .
- Baker , J. H. and Orr , D. R. 1986 . Distribution of epiphytic bacteria on freshwater plants . J. Ecol , 74 ( 1 ) : 155 – 165 .
- Byappanahalli , M. , Shively , D. , Nevers , M. , Sadowsky , M. and Whitman , R. 2003 . Growth and survival of Escherichia coli and Enterococci populations in the macro-alga Cladophora (Chlorophyta) . FEMS Microbiol. Ecol. , 46 ( 2 ) : 203 – 211 .
- Ishii , S. , Ksoll , W. B. , Hicks , R. E. and Sadowsky , M. J. 2006 . Presence and growth of naturalized Escherichia coli in temperate soils from Lake Superior watersheds . Appl. Environ. Microbiol. , 72 ( 1 ) : 612 – 621 .
- Kinzelman , J. , Clement , N. , Jackson , E. , Gradus , S. and Bagley , R. 2003 . Enterococci as indicators of Lake Michigan recreational water quality: Comparison of two methodologies and their impacts on public health regulatory events . Appl. Environ. Microbiol. , 69 ( 1 ) : 92 – 96 .
- Rabinovici , S. J. , Bernknopf , R. L. , Wein , A. M. , Coursey , D. L. and Whitman , R. L. 2004 . Economic and health risk trade-offs of swim closures at a Lake Michigan beach . Environ. Sci. Technol. , 38 ( 10 ) : 2737 – 2745 .
- Taiz , L. and Zeiger , E. 2006 . “ Secondary metabolites and plant defense ” . In Plant Physiology, , 4th ed. , Sunderland , MA : Sinauer Associates . Chapter 13
- USEPA . 1986 . Ambient water quality criteria for bacteria , Washington , D. C. : US Environmental Protection Agency, United States Office of Water. Environmental Protections, Regulations, Agency Criteria and Standards Divisions . EPA 440/5-84-002
- Whitman , R. L. and Nevers , M. 2003 . Foreshore sand as a source of Escherichia coli in nearshore water of a Lake Michigan beach . Appl. Environ. Microbiol. , 69 ( 9 ) : 5555 – 5562 .
- Whitman , R. L. , Shively , D. , Pawlik , H. , Nevers , M. and Byappanahalli , M. 2003 . Occurrence of Escherichia coli and Enterococci in Cladophora (Chlorophyta) in nearshore water and beach sand of Lake Michigan . Appl. Environ. Microbiol. , 69 ( 8 ) : 4714 – 4719 .
- Willey , J. , Sherwood , L. and Woolverton , C. 2008 . Prescott's principles of microbiology , 148 – 149 . Boston , MA : McGraw Hill .
- WI DNR . 2001 . Water quality standards for Wisconsin surface waters , Madison : Wisconsin Department of Natural Resources . NR/102