Abstract
The partitioning of phosphorus (P) loading to culturally eutrophic lakes according to sources is fundamental information to guide rehabilitation programs and support mathematical models. Patterns of concentrations and loading rates of forms of P are documented for the major tributaries of Onondaga Lake, New York, United States, an urban lake that has recently demonstrated marked recovery from extreme cultural eutrophy as a result of decreased P loading from a domestic wastewater treatment plant (WWTP). This analysis is based on long-term (19 yr) biweekly monitoring of total, dissolved, and soluble reactive forms of P at the mouths of the three largest tributaries, and shorter-term higher frequency monitoring of one of the streams at two sites to resolve rural versus urban contributions. Signatures of anthropogenic sources were identified, including: (1) combined sewer overflows, (2) leaky sewers or other dry weather discharges, and (3) hydrogeologic sediment sources. On an annual average basis, the prevailing tributary and WWTP contributions to the total P load are approximately 70 and 30%, respectively. However, in terms of effective P loading, that which can be used to support primary production during the critical summer months, the tributaries contributed substantially less (∼35%) of the P load. Under the prevailing WWTP loading rate, reductions in tributary loading from aggressive tributary loading management efforts (e.g., 20–30% decrease) would not be expected to yield conspicuous improvements in related features of lake water quality. However, noteworthy improvements are a reasonable expectation from such tributary management efforts following the mandated further reductions in WWTP loading.
Many lakes continue to receive excessive anthropogenic phosphorus (P) loads and suffer water quality impacts associated with cultural eutrophication, despite advancements in wastewater treatment and control of nonpoint sources over the last 30 years (CitationCooke et al. 2005). Partitioning external loads according to the contributions of noteworthy sources of the constituent of interest is fundamental information to guide the development of management strategies to achieve rehabilitation goals (CitationJames et al. 1994, CitationUSEPA 1991, Citation1997). The most common partitioning of external loads, adopted in the “total maximum daily load” (TMDL) process (CitationUSEPA 1991), is separation of point from nonpoint source inputs carried in tributaries.
Phosphorus loading has most often been represented in terms of annual total P loading, based on measurements of total P (TP) concentrations and flow rates of inputs. However, not all forms of P are available to support primary production, the principal driver of water quality impacts associated with cultural eutrophication (CitationDePinto et al. 1981, CitationYoung et al. 1982, Citation1985, CitationAuer et al. 1998). Differences in the bioavailability of the various forms of P are generally considered within the broad groupings provided by the three common measures of P concentration: TP, total dissolved P (TDP), and soluble reactive P (SRP). Two other groupings are represented by residual calculations: particulate P (PP = TP − TDP), and dissolved organic P (DOP = TDP − SRP). Dissolved forms of P support phytoplankton growth. Forms of P analyzed as SRP are considered immediately available (CitationNürnberg and Peters 1984, CitationAuer et al. 1998). Most (CitationYoung et al. 1982), to essentially all (CitationAuer et al. 1998), of the DOP pool is also made available to support phytoplankton growth through enzymatic hydrolysis (CitationGage and Gorham 1985, CitationCurrie et al. 1986) and mineralization processes. The bioavailability of PP is substantially lower and more variable, mediated through an array of processes including decomposition and desorption (CitationYoung et al. 1982, CitationAuer et al. 1998). Within the broad PP grouping, municipal waste constituents are generally more available than terrigenous sources (CitationDePinto et al. 1981, CitationYoung et al. 1982, CitationEffler et al. 2002) because of differences in chemical associations within these matrices. Other factors can diminish the effectiveness of external P loading in supporting in-lake primary production, including (CitationEffler et al. 2002): (1) the seasonality of P loading and lake flushing relative to the intervals of concern for primary production, (2) settling losses of bioavailable PP, and (3) plunging of dense inflows and associated P loads below productive depths.
Urban settings represent special challenges for rehabilitation of culturally eutrophic lakes because of the intensity, dynamics, and multiplicity of anthropogenic effects. The relative importance of tributary inputs to urban lakes increases as point source loads are reduced through increased wastewater treatment. This study documented and evaluated patterns of concentrations and loading rates of forms of P in the tributaries of Onondaga Lake, New York, United States, an urban lake that has recently demonstrated marked recovery from extreme cultural eutrophy as a result of increased P removal from a dominant point-source discharge. The analysis was based on a combination of long-term (19 yr) fixed-frequency and shorter-term higher frequency monitoring. This case study is valuable because of: (1) the diversity of anthropogenic sources of tributary P loading to this system; (2) the conspicuous spatial and temporal signatures imparted by these drivers along an important urban stream; (3) the increasing relative importance of the loading from these tributaries from the recent reduction in point source loading; and (4) the array of factors that diminish the effectiveness of tributary loads to support primary production. Tributary P loading conditions were considered in the context of prevailing and future point source loading levels and related water quality goals for the lake.
Study system
Onondaga Lake tributaries and Metro
Onondaga Lake is an alkaline, hardwater, dimictic lake located (43°06′ 54″N; 76°14′ 34″W) in metropolitan Syracuse, New York (). It has a surface area of 12.0 km2, a volume of 131 × 106 m3 and a maximum depth of 20 m. The lake flushes rapidly, on average ∼4 times per year on a completely-mixed basis, and thus responds rapidly to changes in external loading rates (CitationDoerr et al. 1994).
The streams addressed in this analysis, Onondaga Creek, Ninemile Creek and Ley Creek (), represent 85% of the tributary flow to the lake. Onondaga Creek and Ninemile Creek are the largest tributaries, contributing about 38% each of the total tributary flow; Ley Creek represents ∼9%. Approximately 80% of the Onondaga Creek watershed is located in a largely rural area upstream of Syracuse. The downstream 20% of the watershed is urban and drains a substantial portion of the city (). In 1998 the city sewer system had 70 combined sewer overflows (CSO) that discharged mostly to the urban portion of Onondaga Creek during high runoff intervals when system capacity was exceeded. Onondaga Creek contributes a disproportionate (65% on an annual basis) fraction of the allochthonous sediment received by the lake, primarily associated with inorganic particulate inputs from hydrogeologic sources (“mudboils”) located 33 km upstream of the mouth (CitationPrestigiacomo et al. 2007). Mudboils have been attributed by certain investigators (CitationGetchell 1983, CitationKosinski 1985) to earlier nearby solution mining activity.
The Metropolitan Syracuse Wastewater Treatment Plant (Metro; ) has discharged to the adjoining near-shore zone of the lake since the 1920s. It is designed to treat an average waste flow of 3.5 m3/s (80 million gallons per day [MGD]), and has had a relatively uniform average discharge of ∼3.0 m3/s over much of the last three decades. A small fraction of the discharge from Metro (2% as an annual average) irregularly enters the lake during particularly severe storms (e.g., facility hydrologically overloaded) following only primary treatment (described as the Metro “bypass”). The Metro discharge is extraordinary with respect to its contribution to the overall hydrologic budget for the lake (CitationRucinski et al. 2007), representing nearly 20% of the total on an annual basis, and it is the single largest input in August of most years (CitationEffler 1996).
Cultural eutrophication, P management, and lake responses
Onondaga Lake was oligo-mesotrophic before European settlement in the late 1770s (CitationRowell 1996) and supported a cold-water fishery during the 1800s (CitationTango and Ringler 1996). Increased inputs of domestic and industrial wastes accompanied development of the watershed and led to degradation and loss of uses, including the cold-water fishery (late 1800s; CitationTango and Ringler 1996). Manifestations of severe cultural eutrophication included: (1) high concentrations of phytoplankton biomass, with occurrences of blooms of nuisance cyanobacteria (CitationMatthews et al. 2001); (2) low water clarity (CitationEffler et al. 2008b); (3) rapid seasonal loss of dissolved oxygen (DO) from the hypolimnion (CitationMatthews and Effler 2006b); (4) accumulation of reduced by-products of anaerobic metabolism (CitationMatthews et al. 2008); and (5) severe depletion of DO in the upper waters during fall mixing associated with oxidation of the reduced constituents (CitationMatthews and Effler 2006a).
The P load from Metro has been the primary driver of cultural eutrophication in the lake (CitationEffler et al. 2005, CitationEffler et al. 2008a). Concentrations of TP in this effluent (TPMetro), and thereby its TP load (TPMetro/L), have decreased in response to multiple management actions, including a ban on high P content detergents within the service area in 1971 (CitationMurphy 1973) and treatment upgrades, particularly in 1979 (secondary treatment), 1981 (tertiary treatment) and 2005 (high rate flocculated settling). In the late 1990s Metro was responsible for ∼85% of the total effective P load (available to support summertime primary production; i.e., bioavailable and reaches productive layers) to the lake (CitationEffler et al. 2002). Despite a 97% reduction in TPMetro/L from the early 1970s to early 2000s, levels of primary production in the lake decreased by only 30–40% (CitationEffler et. al. 2008a). This lack of response was attributed to the great extent to which the lake had been “overloaded”. The previous reductions in TPMetro/L had been inadequate to shift the lake from a nutrient-saturated to a nutrient-limited system (CitationEffler et al. 2008a). Internal loading of P to the productive layers during summer stratification has been minor compared to external loading because of limited vertical transport from the hypolimnion (CitationEffler et al. 2002).
Ongoing rehabilitation efforts to abate the water quality impacts of cultural eutrophication are now being guided by a TMDL analysis for P (CitationNYSDEC 1998). The numeric water quality goal is a summer (mid-May to mid-Sep) average epilimnetic TP concentration (TPepi) of 20 μg/L, a value generally considered to be consistent with the upper bounds of mesotrophy. Based on the TMDL analysis, TPMetro was mandated to be 120 μg/L by 2006 (implemented by 2005) and 20 μg/L by 2012. The TMDL analysis also called for a nearly 50% reduction in tributary loading, to be partially achieved (13%) by an increase in the annual capture of wet weather combined sewage from 62 to 85%. The remaining required decrease in tributary loading (37%) was to be achieved through nonpoint source controls. According to this nomenclature, as adopted here, tributary loading is the sum of nonpoint and CSO loads. Revision of the TMDL analysis for the lake P is planned for 2009 (CitationNYSDEC 1998).
Proportional decreases in TPMetro/L and primary production from 2002 to 2005 indicated a shift from a nutrient-saturated to a P-limited state in response to the mandated treatment upgrade (CitationEffler et al. 2008a). The lake is now poised for further decreases in primary production. Proportionate further decreases from reductions in total external effective P loading are a reasonable expectation (CitationEffler et al. 2008a). This, together with the increased relative contributions from the tributaries associated with the reductions in TPMetro/L, has increased the importance of assessment of the magnitude and origins of the tributary sources to guide of the ongoing rehabilitation program.
Several perturbations and interventions have occurred, or are being contemplated, in the lake's watershed that may have influenced past tributary P loading or could affect future conditions. These include: (1) sewer line breaks and subsequent repairs in urban areas, (2) increased capture of wet weather combined sewage and sewer separation, (3) an underway Best Management Practices (BMP) program to reduce agricultural loads, and (4) reductions in sediment loading from the mudboils (∼90% in the mid-1990s).
Methods
Grab samples for P analyses were collected biweekly over the 1989–2007 period at four tributary sites. Sampling was conducted at the mouths of Onondaga Creek, Ninemile Creek, and Ley Creek, and at an upstream site on Onondaga Creek (). The mouth location is subsequently described as the downstream site for Onondaga Creek. The upstream Onondaga Creek site supports loading estimates for the urban portion of that watershed (CitationHeidtke and Auer 1992, CitationEffler et al. 2002). More temporally intensive sampling was conducted on Onondaga Creek in 1990 and 2007 by the authors and over the 1999–2002 interval by Onondaga County. Samples were collected ∼5 d/week over June–October in 2007 at both the upstream and downstream sites on Onondaga Creek. Hourly collections were made during a single runoff event in July 2007 and for four events in 1990 with automated sampling equipment located at both the upstream and downstream sites on Onondaga Creek. Samples were collected every 2 h by Onondaga County for three events in 1999, two events in 2000, and a single event each in 2001 and 2002 (CitationOnondaga County 2007). Three forms of P were measured according to standard methods (APHA 1992); TP and SRP measurements began in 1989 and TDP measurements began in 1995. Method reporting limits were 2.2 μg/L for TP and TDP and 0.9 μg/L for SRP. We determined PP and DOP through residual calculations. The average coefficient of variation (cv; standard deviation/mean) for TP triplicate field samples was 0.10.
Loading rates of forms of P carried by the tributaries were calculated as products of concentrations and stream flow (Q). Daily average Q values were used for long-term loading estimates based on the biweekly sampling program. Flow has been continuously monitored by United States Geological Survey (USGS) gauges proximate to the stream mouths (Onondaga Creek, USGS site No. 04240010, Ninemile Creek, USGS site No. 04240300, Ley Creek, USGS site No. 04240120) and at the upstream site on Onondaga Creek (USGS site No. 04239000). The gauges were rated good (95% of daily average values with 10% of true value) except at the mouth of Ninemile Creek, where performance was diminished and data were available only as daily average values. Most of the loading rate estimates presented here are for May–September because these inputs largely drive primary production levels in the lake over the designated (CitationNYSDEC 1998) interval of concern (mid-May to mid-Sep; CitationEffler et al. 2002).
Low sampling frequencies represent challenges for the development of precise daily load estimates. Estimates presented here were based on the long-term fixed-frequency monitoring program and calculated according to the FLUX software program (log-transformed concentration-flow regression; CitationWalker 1995). Phosphorus data were pooled into two time segments, 1995–2000 (interval 1), and 2001–2007 (interval 2). This demarcation is roughly consistent with the initiation of programs to reduce tributary P loading. Two flow strata were adopted for each interval, demarcated by the mean flow.
The irregular short-term urban inputs from CSO to Onondaga Creek were assessed separately and added to the fixed-frequency program loading estimates. The CSO events were identified through review of the 15-min USGS flow data from the downstream gauge on Onondaga Creek as abrupt increases, usually of brief duration. The total volume delivered for each of these events (VCSO, m3) was estimated as the area under the hydrograph, above the antecedent flow. Phosphorus loads for monitored CSO events (TPL/CSO, TDPL/CSO, SRPL/CSO; kg) in 1990, over the 1999–2002 interval (8 events for TP, 4 events for TDP, and 7 events for SRP; Onondaga County), and in 2007, were estimated by integrating the area under the pollutographs (loading rates vs. time) for each event. Ratios of the total event P loads to VCSO (TPL/CSO:VCSO, TDPL/CSO:VCSO, and SRPL/CSO:VCSO) were calculated for each of the monitoring events. These ratios formed the basis to estimate P loading for CSO events that were not monitored for P concentrations. Loads for unmonitored events were estimated as the product of VCSO and the average ratio value for the form of P of concern over the 1999–2002 interval, for example TPL/CSO = (TPL/CSO:VCSO)· VCSO. Because TDPL/CSO estimates were only available for four monitored events for that interval, those for unmonitored events were based on the average ratio observed for TDPL/CSO: SRPL/CSO (1.45, cv = 0.10) and SRPL/CSO estimates for each event. Values of PPL/CSO for the CSO events were estimated as the residual TPL/CSO minus TDPL/CSO.
Results and discussion
Onondaga Creek
Leaky sewers
Relatively low flow conditions prevailed during the intensive monitoring interval of June–October 2007. Values of daily average Q were less than the long-term (1971–2006) median for much of the interval (). The median of daily average Q for the interval was ranked the 25th lowest of the 37-year record. A number of modest runoff events occurred during this interval, with the highest Q values observed in late June, mid-July and late October (). The temporal patterns of TP at the upstream and downstream sites tracked each other relatively closely at the daily time scale over most of the interval (). Increases in TP generally coincided with increases in Q; TP exceeded 100 μg/L during several runoff events (). Increases in concentrations of dissolved forms were also observed during the runoff events, particularly at the downstream site ( and ). Systematically higher TDP and SRP levels were observed downstream compared to the upstream site during low flow intervals ( and ).
Figure 2 Time series for two sites on Onondaga Creek that bracket the urban portion of the watershed: (a) Q, with mean daily flow from long-term record for downstream site, (b) TP, (c) TDP, and (d) SRP.
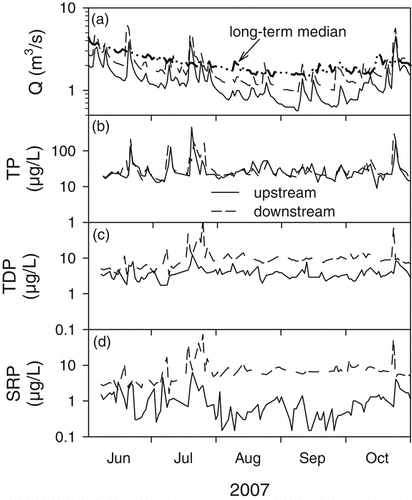
Higher concentrations of dissolved forms of P within the urban portion of the stream throughout the low flow interval of 2007 suggest ongoing inputs of P-rich wastewater. Sources probably include “leaky” adjoining sewer lines and perhaps other uncontrolled waste inputs. Moreover, the enrichment of the stream with dissolved P within the city has a conspicuous chemical signature, preferential increases in SRP compared to DOP. For example, similar increases (∼5.8 μg/L) in median values of TDP and SRP were observed during low flow (designated as daily average Q < 2.8 m3/s at the downstream gauge, a threshold adopted hereafter) portions of the temporally intensive monitoring interval of 2007 ( and ). This was also manifested as a significant (paired t-test, n = 94, p < 0.001) increase in the SRP:TDP ratio from the upstream to downstream sites during low flow intervals in 2007. Domestic waste origins of the urban dissolved P input indicated here are consistent with the recently reviewed increases in fecal coliform bacteria levels over this stream reach during low flow intervals (CitationOnondaga County 2007).
Figure 3 Patterns of dissolved forms of P in Onondaga Creek for dry weather/low flow (< 2.8 m3/s) conditions: (a) distributions of TDP at the upstream and downstream sites during the June–October interval of 2007, (b) distributions of SRP at the upstream and downstream sites during the June–October interval of 2007, and (c) time series of the annual average SRP at the two sites with vertical bars that correspond to ± one standard deviation.
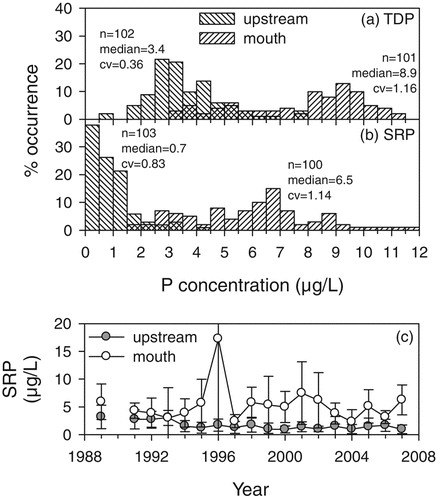
The long-term record of SRP levels at the upstream and downstream sites on Onondaga Creek () during low flow conditions establishes the recurrence and variations of this urban input of dissolved P. This flow-based stratification of the data results in annual populations ranging from 9 to 18 observations for low flow conditions over the 1990–2006 interval, 28 observations in 1989 and 94 in 2007. Note that SRP values have remained relatively low (e.g., usually < 2 μg/L) and uniform upstream of Syracuse (). Concentrations have been higher at the downstream site throughout the 19-year period, and in some years by a wide margin (). Annual average SRP concentrations were significantly higher at the downstream site (t-test on log-transformed data, p < 0.01, df = 33). Interannual differences in Q explained only 22% of the variability in SRP within this low flow stratum (linear regression, p = 0.048); thus, it seems that substantial variations in the urban dry weather load have occurred over this period. The particularly high average SRP reported for the downstream site in 1996 () reflected the effects of high concentrations (25–75 μg/L) during September and October, perhaps associated with a failure of a local sewer line. The drivers of other features of the long-term pattern in the urban source(s) of dissolved P are unknown, though some coupling with the maintenance and functioning of the bordering sewer system is a reasonable explanation. The prevailing urban low flow dissolved P load to Onondaga Creek, estimated as the average of the residuals of daily TDP loads (TDPL) for the downstream and upstream locations in 2007, was about 1.0 kg/d.
Onondaga Creek CSOs
Dry weather, or base, loading is augmented during runoff events of adequate magnitude by additional inputs from the urban area, as illustrated here for a modest event in early July 2007 (). Conspicuous differences in hydrologic and P loading responses to this event were manifested at these two sites, reflecting fundamental differences in these portions of the watershed. The abrupt increase in Q at the downstream site before clear responses occurred at the upstream site () is a recurring manifestation of inputs from CSO (CitationEffler 1996, CitationOnondaga County 2007), although direct inputs from stormwater runoff doubtless also contribute. The abruptness of the response reflects the relatively large area of impervious surfaces within contributing portions of the city. Dramatic increases in TDP () and SRP (not shown) tracked the second of these peaks (samples not collected during first peak) at the downstream site, qualitatively consistent with contributions of domestic waste in the CSO inputs. The combination of increases in both Q and TDP translated to substantial increases in TDPL above low flow levels (). For example, the estimated additional TDPL delivered from the city during the 3 h of the second hydrologic peak was about 2 kg (area under the loading rate time series), corresponding to the magnitude of dry weather inputs received by this stream reach over ∼48 h of low flow conditions. Conditions for TDP () and TDPL () upstream of the city during the subsequent increase in flow were invariant by comparison. Inputs from the urban portion of the stream have dominated loading of dissolved forms of P from this tributary during runoff events (e.g., and ).
Figure 4 Time series for a runoff event in July of 2007: (a) Q, (b), TDP, (c) TDPL, (d) PP, and (e) PPL.
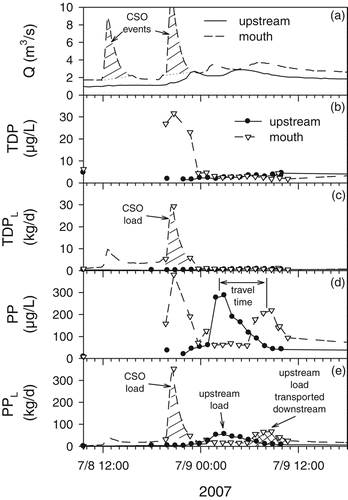
Substantial short-term increases in PP and PPL also occur at the downstream site on Onondaga Creek during CSO events, as depicted for the July 2007 event ( and ). An abrupt increase in PP tracked the timing observed for TDP (); the peak PP approached 400 μg/L (). This increase clearly had an urban origin, attributable primarily to inputs from CSO. The urban input of PP during the second abrupt CSO-based hydrologic increase () was estimated to be ∼20 kg.
Material loading from CSO is complicated by a wide array of difficult-to-quantify factors that can affect its magnitude and drive variability, such as antecedent conditions, runoff intensity and seasonality. The load:volume ratios for TP (TPL/CSO:VCSO; ) and SRP (SRPL/CSO:VCSO; ) demonstrated general uniformity (e.g., similar potency) for different portions of the record. The coefficient of variation (cv) values for TPL/CSO:VCSO for 1990 and the 1999–2002 interval were 0.57 and 0.52, respectively. The decrease in the mean value over this period () was significant (t-test, p = 0.046), suggesting a benefit from management actions over the intervening interval. The single ratio value for 2007 was similar to the 1999–2002 observations (); however, the SRPL/CSO:VCSO was lower for the 2007 event than for the mean of monitored events from 1999 to 2002 ().
Figure 5 Ratios of estimates of Onondaga Creek CSO loading for TP (TPL/CSO) and SRP (SRPL/CSO) and volume (VCSO) for different years or intervals: (a) TPL/CSO:VCSO, and (b) SRPL/CSO:VCSO.
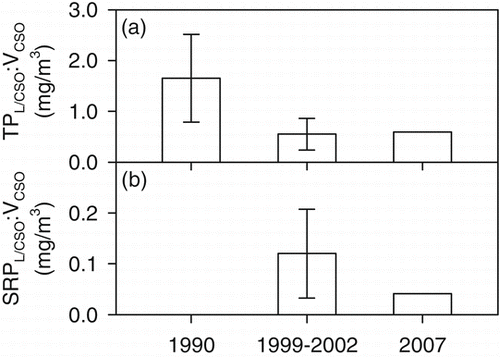
Numerous CSO events were identified in the downstream flow record for the 1995–2007 period. On average, 41 CSO events occurred annually and 25 during the summer (May–Sep) interval, although wide interannual differences have occurred (). More CSO events occurred, and associated P loading was higher (t-test, p = 0.11, df = 10) in summer over the most recent interval (2001–2007) compared to the 1995–2000 period, associated with higher runoff in recent years. Interannual differences in the summed summertime estimates of VCSO, and thereby corresponding CSO phosphorus loads, were strongly positively related to corresponding mean summertime Q at the downstream site (r 2 = 0.76, p = 0.0001; linear least squares regression). Dissolved forms of P represented 31% of the estimated overall P loads. The estimated average annual loading rates from the CSOs for TDP (TDPL/CSO), PP (PPL/CSO), and TP (TPL/CSO) for the entire period were 0.9, 1.9 and 2.8 kg/d, respectively (329, 694, 1203 kg/yr; ). The phosphorus TMDL analysis projected TPL/CSO to decrease by 10.3 kg/d (3760 kg/yr) through increased capture of CSO (CitationNYSDEC 1998). This decrease is nearly 4-fold higher than our estimate of TPL/CSO. Estimates of average summertime loading rates for TDPL/CSO, PPL/CSO and TPL/CSO were > 60% higher than annual rates, 1.4, 3.1 and 4.5 kg/d, respectively. The annual average CSO input of TDP (0.9 kg/d) was similar to the urban dry weather inputs estimated for 2007 (1.0 kg/d).
Table 1 CSOs on an annual and summertime (May–Sep) basis for the 1995–2007 period: number of events, and estimates of VCSO, TDPL/CSO and PPL/CSO.
Upstream contributions to PP L and the mudboils
In sharp contrast to the situation for dissolved forms of P, the upstream portion of the watershed was a major contributor of PPL. For example, a substantial increase in PP (∼300 μg/L; ) occurred in response to the increase in flow at the upstream site during the July 2007 event (). The transfer of this peak to the downstream site was clearly resolved, depicting an approximate travel time under those flow conditions of 5–6 h (). The corresponding PPL values for this peak at the upstream and downstream sites were within 4% of each other, indicating the effective transport of PP from upstream portions of the watershed to the mouth during such events.
Contributions of inorganic sediment from the mudboils, mostly in the form of clay mineral particles, have dominated sediment loading to the upstream site (CitationPrestigiacomo et al. 2007) as well as the lake (CitationEffler et al. 1992). Clay minerals have a high adsorptive capacity for phosphate (CitationFroelich 1988) a fraction of the SRP pool. The high concentrations of these particles that prevail may be responsible for maintaining low SRP levels at the upstream site (, and ). Moreover, these particles may serve to limit SRP levels downstream following runoff events. Desorption could occur in the upper waters of the lake, however, where lower SRP levels generally prevail (CitationEffler 1996). The results of a single bioassay of suspended sediment from the upstream site indicated less than one-third of the associated P was bioavailable (CitationEffler et al. 2002). The effects of these clay mineral particles are expected to continue because the upstream source of sediment does not seem amenable to management. Despite the major reductions in sediment loading from the mudboils, loads carried by the stream have not declined, perhaps a result of continuing inputs form the stream bed and banks from earlier inputs (CitationPrestigiacomo et al. 2007).
On average, upstream sources (PPL/U) represented nearly 50% of the estimated summertime PPL from the stream (downstream, PPL/D) for the 1995–2007 period, though substantial interannual variations have occurred (). The relative contribution from upstream sources increased as Q increased. Variations in the summer mean Q explained 83% of the variability in the ratio of the estimates of the upstream to downstream loads for the 13-year interval, according to linear least squares regression ().
Extent of closure for P sources for Onondaga Creek
The extent of closure of the apparent net inputs for the urban portion of the watershed with the summation of the “leaky” sewer and CSO inputs represents a valuable check on the consistency of the various estimates. These estimates of the urban contributions were based on widely different monitoring approaches with respect to duration and frequency of supporting measurements, influences of year-to-year differences in anthropogenic drivers, and sources and magnitudes of uncertainties in estimation techniques. The TDPL and PPL values for CSO correspond to averages for the 2001–2007 interval, while the leaky sewer estimates were based strictly on conditions in 2007.
Given the limitations in the components supporting the comparisons, reasonably close agreement was observed for both TDPL and PPL ( and ). This supports the general representativeness of the partitioning of the inputs to this stream, although the independently estimated urban components were 70% of the net differences obtained from the upstream–downstream monitoring for both forms of P loading. Alternatively, summation of the CSO, leaky sewer and upstream loads represented 83% of the downstream estimates of both TDPL and PPL based on long-term monitoring (). According to this partitioning, rural inputs, leaky sewers and CSO contributed nearly equally to the TDPL delivered to the lake ().
Comparative features of tributaries and overall loading estimates
Substantial differences in composition of the P pools have prevailed for the four sites, as represented by the ratios TDP:TP (, , , , ) and SRP:TDP (, , , , ). Signatures of anthropogenic effects are manifested in these ratios. The ratio TDP:TP is an indicator of the relative bioavailability of these inputs; higher values indicate greater bioavailability (CitationEffler et al. 2002). The ratio SRP:TDP reflects the immediately available fraction of the TDP pool and can serve as an indicator of domestic waste.
Figure 8 Distribution of ratios of forms of P for Onondaga Lake tributaries for the May–September interval: (a) TDP:TP, upstream site on Onondaga Creek, (b) TDP:TP, downstream site on Onondaga Creek, low flow conditions, (c) TDP:TP, downstream site on Onondaga Creek, high flow conditions, (d) TDP:TP, mouth of Ninemile Creek, (e) TDP:TP, mouth of Ley Creek, (f) SRP:TDP, upstream site on Onondaga Creek, (g) SRP:TDP, downstream site on Onondaga Creek, low flow conditions, (h) SRP:TDP, downstream site on Onondaga Creek, high flow conditions, (i) SRP:TDP, mouth of Ninemile Creek, and (j) SRP:TDP, mouth of Ley Creek.
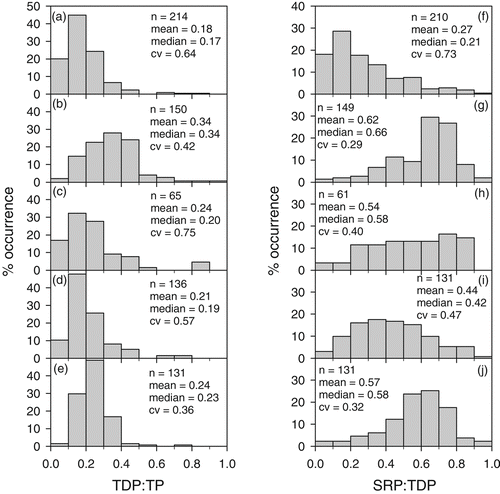
The TDP:TP ratio was relatively low at the upstream Onondaga Creek site (median = 0.17; ) but increased significantly (paired t-test, p < 0.001) downstream during dry weather (median = 0.34; ), apparently from domestic waste inputs. Under higher flow conditions the ratio shifted to lower values at the downstream site (median = 0.20; ,), at least in part due to increased loading of mudboil-based PP from upstream. The indicated relative bioavailability of the pool at the mouth of Ninemile Creek () is similar to that for the mouth of Onondaga Creek during high runoff. The median TDP:TP for Ley Creek (0.24; ) was about 20% higher.
The relative contribution of SRP to the TDP pool increased nearly 3-fold from the upstream site on Onondaga Creek to the stream mouth during low flow conditions ( and ), and about 2-fold during higher flows ( and ), associated with SRP-enriched urban inputs. Values of the ratio at the mouth of Ninemile Creek (median = 0.42; ) were generally intermediate between those for the upstream and downstream Onondaga Creek sites. Values of the ratio for the mouth of Ley Creek () were generally more similar to the mouth of Onondaga Creek during low flows () than Ninemile Creek (). The differences in the composition of the P pools at the mouths of these tributaries indicate differences in their relative impacts on primary production in the lake (e.g., per unit TP load).
One approach to identify changes in loading potential that accommodates variations in flow is evaluation of the extent of differences in concentration versus Q relationships between designated intervals (CitationPrestigiacomo et al. 2007). Observations and best fit relationships, partitioned according to the low and high flow ranges, for the two designated intervals for TDP at the mouth of Onondaga Creek () represent the bioavailable load from the largest tributary source. Despite considerable scatter during both periods, the slope of the best-fit relationship for low flows was significantly lower (p = 0.015, intercepts not significantly different) during interval 2 (2001–2007). This suggests a decrease in loading potential for TDP during low flows at this site, consistent with implementation of rehabilitation measures over that interval. The best-fit relationship was shifted lower for the recent interval under high flow conditions (). Such relationships were not manifested for all the tributaries and forms of P; however, the result for TDP at the mouth of Onondaga Creek suggests that decreases in loading potential may have been masked by the generally higher flows in the latter interval (). Flow-weighted TDP concentrations decreased from 12.1 μg/L during interval 1 to 9.5 μg/L during interval 2 under low flow conditions. A larger decrease, from 19.4 to 11.2 μg/L, occurred under high flow conditions. Trends in tributary concentrations and loads are difficult to document because of natural fluctuations in flows driven by climatic variability that have a large stochastic component (CitationRichards and Baker 1993, Citation2002). There are alternate, and perhaps more robust, trend analysis techniques that deserve consideration in further evaluations of shifts in loading potential of these tributaries in the future (CitationRichards and Baker 1993, Citation2002).
Table 2 Average flow and phosphorus loading rates to Onondaga Lake.
Figure 9 Evaluation of TDP vs. stream flow (Q) relationships for the downstream site on Onondaga Creek for two stream flow strata and two time intervals, 1995–2000 (interval 1) and 2001–2007 (interval 2).
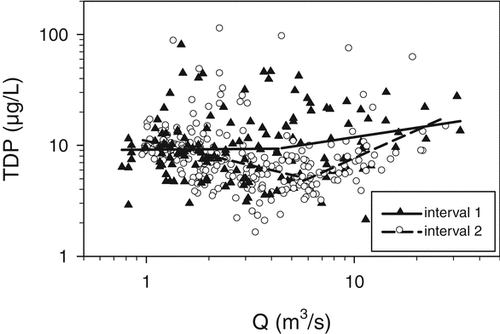
Stratification of the interval of loading calculations to match the seasonal aspects of the water quality goal (CitationEffler et al. 2002) had a pronounced effect on the magnitude of these estimates (). Annual average loading rate estimates were systematically higher (∼50%) for these three tributaries than the summer average values, primarily as a result of the higher annual versus summer flows (), a feature common to this region (CitationEffler 1996). The total annual average loading rate estimates for the three streams exceeded the summer average values (1995–2007) of PPL by 73% and TDPL by 53%.
Onondaga Creek has represented 50% of PPL and 52% of TDPL of the summed summertime loads from these three tributaries, while Ninemile Creek contributed 33 and 31%, respectively (). Ley Creek has made disproportionately large contributions to P loading representing 17% of PPL and 17% of TDPL, despite representing only ∼11% of the summertime flow. Four other minor tributaries, not reported here, represent < 10% of the total tributary load of TP to Onondaga Lake (CitationEffler 1996).
Synthesis and management perspectives
Point versus tributary loading
The partitioning of P loading according to forms and sources is central to identification of appropriate targets for future reductions and for setting reasonable expectations for implemented actions. Five different cases of such partitioning are considered here for Onondaga Lake to (1) demonstrate potential pitfalls in evaluating the relative roles of point versus tributary inputs; (2) provide a reasonable representation of prevailing conditions; and (3) identify information gaps for development of more definitive partitioning. Phosphorus loading is widely represented in terms of annual TP loading rates (TPL), presented here as case 1. Accordingly, the lake tributaries presently represent 70% of the prevailing (average for 2001–2007 interval) annual TPL (case 1; ). The concentration of the Metro effluent (TPMetro) and discharge flow were assumed to be 120 μg/L and 3.0 m3/s in this partitioning and for the following three cases (cases 2, 3 and 4).
Figure 10 Contributions to P loading, Metro and tributaries: (a) case 1, TPL on an annual basis, TPMetro = 120 μg/L; (b) case 2, TPL for the May-September interval, TPMetro = 120 μg/L; (c) case 3, PPL adjusted for bioavailability, TPMetro = 120 μg/L; (d) case 4, effective (available to support primary production) P loading from tributaries, TPMetro = 120 μg/L; and (e) case 5, effective P loading from tributaries and Metro bypass, TPMetro = 20 μg/L, estimated manageable fraction of tributary loads indicated. Adjustments for effective P loading as described by CitationEffler et al. (2002). Metro bypass included within TPMetro/L for all cases. Total loading rates for each case appear below the respective pie charts.
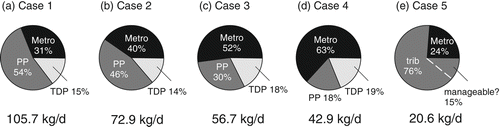
The time segmentation of “annual” in such calculations is inappropriate for this rapid-flushing rate lake (CitationEffler et al. 2002). Inputs received outside of the interval designated for the water quality goal, mid-May through mid-September, have substantially diminished influence on the lake P pool and associated metabolism during this summer interval. Thus, case 2, which considers only the loading over the May–September interval (), is inherently more representative in the context of the water quality goal (CitationNYSDEC 1998). In this case, the contribution of the tributaries decreases to 60% (). The remaining cases all consider loading levels for the summer interval.
Case 3 represents the limited bioavailability of PPL from tributaries, based on the earlier bioassay experiment results (samples collected in 1996; CitationEffler et al. 2002). The tributary TDPL is assumed to be completely bioavailable (CitationAuer et al. 1998, CitationEffler et al. 2002). The Metro TP load is assumed to be completely bioavailable for this and subsequent cases and is a conservative, or worst case, assumption. For example, based on characterizations (including a bioassay experiment) of P in the Metro effluent before the most recent treatment upgrade, approximately 60% of the PP from this source was found be bioavailable (CitationEffler et al. 2002). However, such information is not available for the prevailing Metro discharge (i.e., following the most recent treatment upgrade). Related P measurements (e.g., paired TP and TDP, routinely) and bioassay experiments (CitationDePinto 1982, CitationAuer et al. 1998) are recommended for this effluent to fill this information gap. Updated and more temporally comprehensive bioassay experiments for the primary tributaries are also recommended. The 100% bioavailable assumption for Metro is a prudent management position in the absence of the above measurements and in light of the fundamental change in treatment. The contribution of the tributaries diminishes further in case 3, associated with the limited bioavailability of PPL (52%; CitationEffler et al. 2002), to 48% of the total (case 3; ).
Case 4 considers the effects of seasonal plunging of tributaries below productive lake depths and settling losses of PPL that act to further diminish the availability of the tributary P loads (CitationEffler et al. 2002). Plunging is promoted in Onondaga Lake from seasonally lower stream temperatures and both natural and anthropogenic saline inputs to the two largest tributaries and has been represented for a single year through application of a tested hydrodynamic/stratification model (CitationEffler et al. 2002). This plunging acts to diminish both the bioavailable PPL and TDPL components of P loading during intervals of its occurrence. The situation with respect to depth(s) of entry of the Metro discharge is strikingly different. The Metro effluent usually enters the upper well-mixed productive layers during summer because of both its higher temperature and lower salinity, supporting the greater “effectiveness” of this P input (CitationEffler et al. 2002). Further, potentially bioavailable PP can be lost to deposition before the associated P is converted to an available form. Based on comparison of the rate constants determined in PP bioavailability (bioassay) experiments and assumed settling rates of tributary particles, it has been estimated that ∼75% of the bioavailable PP from Ninemile Creek and ∼40% from Onondaga Creek would be lost through deposition before it could release the available P. Based on the greater contribution of organic material to TSS in the Metro effluent, settling losses of bioavailable PP from this input were estimated to be smaller (∼10%; CitationEffler et al. 2002) before the most recent treatment upgrade. The bioavailable tributary loads were adjusted in the calculations for case 4 according to factors reported previously for plunging and deposition; the overall adjusted values are described as “effective” loads (CitationEffler et al. 2002). In sharp contrast, the P load from Metro was assumed to be 100% effective for this case, again a prudent management assumption in the absence of more detailed characterization of the effluent. Accordingly, the contribution of the tributaries decreases further to 37%, and Metro continues to be the dominant source (case 4 in ).
Substantial uncertainty is acknowledged in the comparative extent of plunging of Onondaga Creek and Ninemile Creek and the coupled effects in diminished external P loads, which should be eliminated through the conduct of appropriate hydrodynamic experiments (CitationMartin and McCutcheon 1999). Moreover the lack of comprehensive characterization of the particulate fraction of the present Metro effluent also limits quantitative partitioning of the loading between the discharge and the tributaries. A series of measurements is recommended for both Metro and the tributaries to describe the comparative settling features of PP from these sources, including: (1) particle size distributions and gross chemical composition (CitationPeng et al. 2007); (2) partitioning of P according to particle classes; and (3) settling velocity measurements according to size class (CitationPerkins et al. 2007). Such studies, in concert with the recommended bioassay experiments, could support less conservative representation of TPMetro/L and a decrease in its contribution.
Simple empirical analyses of the dependence of TP concentrations in the epilimnion (TPepi) on external P loading support the dominant role the Metro input has played in regulating changes over the monitored period and the minor role of the tributary component, particularly PPL (). Changes in the average TPMetro/L for the May–September interval explained 69% (p = 0.0004) of the observed variations in TPepi over the 1995–2007 period (). This relationship becomes stronger when the period is extended back to 1989 (r 2 = 0.83, p < 0.0001; Upstate Freshwater Institute, unpublished). Representation of the driving load instead as the sum of TPMetro/L and TDPL () results in a nearly equally strong empirical relationship (r 2 = 0.67, p = 0.0006; ). The lack of a significant effect by the inclusion of TDP L is consistent with the small magnitude of the variations in TDPL relative to those for TPMetro/L over the same period. Inclusion of the effects of variations in PPL over the same period, by representing the driving P load as the sum of TPMetro, TDPL and PPL, results in a weaker model (). This is consistent with the position that only a small fraction of the tributary PPL contributes to the TP pool of the lake's epilimnion, a point depicted in the progression of decreasing contributions of PPL from cases 1–4 ( and ). Thus, the rather wide interannual variations in PPL have not been important in driving the variations in TPepi among years, and when included in the external load cause an overall weaker model ().
Figure 11 Evaluation of the dependence of TPepi on summer average external loads of P, 1995–2007: (a) TPMetro/L, (b) summation of TPMetro/L and TDPL, and (c) summation of TPMetro/L, TDPL, and PPL.
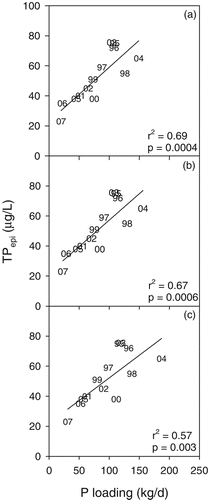
Mechanistic water quality models concerned with cultural eutrophication of lakes need to represent the partitioning of external P loading according to PPL and TDPL because of their relative differences in potency in supporting primary production and in-lake behavior. Moreover, the comparative effectiveness of the P loads in supporting primary production, particularly the PP fraction, needs to be described in models for this lake. This second step entails quantification of the various factors that diminish the effectiveness of the external TP loads, such as bioavailability and settling of PP and plunging of tributary inputs. The tributary loads developed here as time series for each of the years in support of this analysis, in combination with available Metro loading information (e.g., CitationEffler et al. 2008a), represent appropriate external loading inputs to drive dynamic nutrient-phytoplankton models for the 1995–2004 period. However, the lack of more robust characterization of P in the Metro effluent for 2005–2007 limits representation for this critical interval in such models. Moreover, the models for this lake will need to be robust with respect to representation of entry and in-lake behavior of the individual noteworthy sources of P to properly adjust the external loads to deliver appropriate “effective loads.” The issues of bioavailability and overall effectiveness of external P loads are of broad importance for culturally eutrophic systems.
Management targets, now and in the future
This analysis demonstrates that tributary P loading to this urban lake is influenced by multiple anthropogenic drivers, including: (1) CSO; (2) leaky sewers or other dry weather discharges; and (3) mudboils. At least three important factors need to be considered in placing the tributary P-loading estimates presented here in a management context: (1) the prevailing relative apportionments of contributions according to source; (2) systematic changes that would result from implementation of other components of the existing management plan (CitationNYSDEC 1998); and (3) the extent to which the various sources are manageable. The prevailing dominant source of P, the Metro discharge (see case 4; ), is completely manageable (e.g., through diversion to the Seneca River). Diversion of Metro had been the leading rehabilitation plan before the loss of assimilative capacity for oxygen demanding wastes of the Seneca River from the zebra mussel invasion (CitationEffler et al. 2004), an alternative that is to be revisited if the P goal for the lake cannot be reached (CitationNYSDEC 1998). Recently a dual discharge strategy was proposed that would effectively result in a partial diversion to the river; for example., the effluent would be routed to the river except when oxygen standards may be compromised, in which case the effluent would be discharged to the lake (CitationRucinski et al. 2007). While partial diversion of the Metro effluent would decrease P loading to the lake, it would also affect the hydrology of the lake through reductions in inflow. The mandated future (2012) upgrade in treatment (TPMetro = 20 μg/L), with continued discharge to the lake, will result in an additional 75% reduction in the loading rate from the facility (includes the continuing contribution from the bypass at Metro).
The most uncertain of the tributary loading information presented here is the CSO component of the Onondaga Creek contribution. Implementation of the plan (underway) to capture more (85%) of the CSO flow annually (CitationNYSDEC 1998) will undoubtedly result in decreased loading from the urban portion of this watershed; however, the assumed decrease in TPL from CSOs in the TMDL analysis has been demonstrated here to be unrealistically high. The results of this study are valuable for the ongoing management program for P for Onondaga Lake, and will provide guidance for the forthcoming (2009) revision of the phosphorus TMDL analysis. The manageable fraction and its magnitude for the tributary inputs are much smaller compared to prevailing loads from Metro following its recent upgrade (). Based on analysis of tributary loading rates from the 1990s, normalized for contributing watershed areas (unit area loads), these inputs were described as poor targets for substantive reductions from rehabilitative methods (CitationEffler et al. 2002). The lack of reductions in tributary loading over the two coarse time segments addressed here () is at least superficially consistent with that position. Differences in runoff between these intervals could have masked systematic decreases in the loading potential of these streams, however, particularly Onondaga Creek. For example, decreased loading potential from CSOs () and for TDP from Onondaga Creek () have been reported here. More robust trend analyses (e.g., CitationRichards and Baker 2002) are recommended to further elucidate and quantify these potential changes in response to management actions.
A range of 20 to 30% is a reasonable upper bound for recently implemented and future reductions in tributary loading. This magnitude of loading reduction in the context of the prevailing Metro load would be relatively minor (e.g., 8–12% decrease overall, case 4; ). Accordingly, expectations for the benefits of tributary management efforts in the absence of implementation of further reductions in loading from Metro (e.g., increased treatment or partial diversion) would be limited. Interannual variations in runoff could mask such modest changes from tributary management. However, this range of reduction in effective P loading from the tributaries will become substantially more meaningful under the future loading conditions that will result from the reduction in TPMetro to 20 μg/L (case 5; ) or diversion. Under future conditions associated with increased treatment, the potential manageable portion of the tributary loading would represent from 16 to 24% of the total, which would probably result in more demonstrable (e.g., manifested in most years) improvements in the lake.
This analysis has embraced the concept of effective P loading, that portion of the load available to support summertime primary production. Accordingly, several factors may act to diminish the effectiveness, or availability, of the more commonly considered annual external TP load, including: (1) bioavailability, (2) the seasonality of P loading and lake flushing, (3) settling losses of bioavailable PP, and (4) plunging of dense inflows below productive depths. These factors reflect processes that are broadly occurring, although their relative importance is expected to be system-specific. A number of recommendations have been made to better quantify these effects for Onondaga Lake to guide ongoing rehabilitation initiatives. The extra level of effort necessary to implement the effective P loading approach is justified for systems severely impacted by cultural eutrophication targeted for costly related rehabilitation programs such as Onondaga Lake.
Acknowledgment
Funding for portions of this work was provided by USEPA and the Onondaga Nation.
Notes
+Coefficient of variation, in parenthesis.
**Period covered by FLUX loading calculations.
*1995–2000, first of two periods for FLUX loading calculations.
+2001–2007, second of two periods for FLUX loading calculations.
xCoefficient of variation, in parenthesis.
xxA = annual, S = summer.
*Contribution No. 269 of the Upstate Freshwater Institute
References
- [APHA] American Public Health Association . 1992 . Standard methods for the examination of Water and Wastewater, , 18th edition Washington , DC
- Auer , M. T. , Tomasoski , K. A. , Babiera , M. J. , Needham , M. , Effler , S. W. , Owens , E. M. and Hansen , J. M. 1998 . Phosphorus bioavailability and P-cycling in Cannonsville Reservoir . Lake Reserv Manage , 14 : 278 – 289 .
- Cooke , G. D. , Welch , E. B. , Peterson , S. A. and Nichols , S. A. 2005 . Restoration and management of lakes and reservoirs , Boca Raton , FL : Taylor and Francis, CRC Press .
- Currie , D. J. , Bentzen , E. and Kalff , J. 1986 . Does algal-bacterial partitioning vary among lakes? A comparative study of orthophosphate uptake and alkaline phosphatase activity in freshwater . Can J Fish Aquat Sci , 43 : 311 – 318 .
- DePinto , J. V. 1982 . An experimental apparatus for evaluating kinetics of available phosphorus release from aquatic particulates . Water Res , 16 : 1065 – 1070 .
- DePinto , J. V. , Young , T. C. and Martin , S. C. 1981 . Algal-available phosphorus in suspended sediments from lower Great Lakes tributaries . J Great Lakes Res , 7 : 311 – 325 .
- Doerr , S. M. , Effler , S. W. , Whitehead , K. A. , Auer , M. T. , Perkins , M. G. and Heidtke , T. M. 1994 . Chloride model for polluted Onondaga Lake . Water Res , 28 : 849 – 861 .
- Effler , S. W. 1996 . “ Limnological and engineering analysis of a polluted urban lake ” . In Prelude to environmental management of Onondaga Lake, New York , New York , NY : Springer-Verlag .
- Effler , A. J. P. , Gelda , R. K. , Effler , S. W. , Matthews , D. A. , Field , S. D. and Hassett , J. M. 2008a . Decreases in primary production in Onondaga Lake from reductions in point source inputs of phosphorus . Fundam Appl Limnol , 172 : 239 – 253 .
- Effler , S. W. , Gelda , R. K. , Field , S. D. , Effler , A. J. P. , Wallenberg , E. B. and Matthews , D. A. 2005 . Changes in primary production in Onondaga Lake, NY: Magnitude, metrics, and drivers . Lake Reserv Manage , 21 : 49 – 60 .
- Effler , S. W. , Gelda , R. K. , Perkins , M. G. , Peng , F. , Hairston , N. G. and Kearns , C. M. 2008b . Patterns and modeling of the long-term optics record of Onondaga Lake, New York . Fundam Appl Limnol , 172 : 217 – 237 .
- Effler , S. W. , Johnson , D. L. , Jiao , J. and Perkins , M. G. 1992 . Optical impacts and sources of suspended solids in Onondaga Creek, USA . Water Resour Bull , 28 : 252 – 262 .
- Effler , S. W. , Matthews , D. A. , Matthews , C. M. , Perkins , M. G. , Siegfried , C. A. and Hassett , J. M. 2004 . Water quality impacts and indicators of metabolic activity of the zebra mussel invasion of the Seneca River . J Am Water Resour Assoc , 40 : 737 – 754 .
- Effler , S. W. , O'Donnell , S. M. , Matthews , D. A. , Matthews , C. M. , O'Donnell , D. M. , Auer , M. T. and Owens , E. M. 2002 . Limnological and loading information and a phosphorus total maximum daily load analysis for Onondaga Lake . Lake Reserv Manage , 18 : 87 – 108 .
- Froelich , P. N. 1988 . Kinetic control of dissolved phosphate in natural rivers and estuaries: A primer on the phosphate buffer mechanism . Limnol Oceanogr , 33 : 649 – 668 .
- Gage , M. A. and Gorham , E. 1985 . Alkaline phosphatase activity and cellular phosphorus as an index of the phosphorus status of phytoplankton in Minnesota Lakes . Freshwater Biol , 15 : 227 – 233 .
- Getchell , F. A. 1983 . Subsidence in the Tully Valley, New York , MS thesis Syracuse , NY : Syracuse University .
- Heidtke , T. M. and Auer , M. T. 1992 . Partitioning phosphorus loads: Implications for lake restoration . J Water Res Plan Manage , 118 : 562 – 579 .
- James , R. T. , O'Dell , K. and Smith , V. H. 1994 . Water quality trends in Lake Tohopekaliga, Florida, USA: Responses to watershed management . Water Resour Bull , 30 : 531 – 546 .
- Kosinski , A. J. 1985 . Analytical critique of summary consultants report: Relationship of brining operations in the Tully Valley to the behavior of groundwater and geological resources , Albany , NY : New York State Department of Environmental Conservation .
- Martin , J. L. and McCutcheon , S. C. 1999 . Hydrodynamics and transport for water quality modeling , Boca Raton , FL : Lewis Publishers .
- Matthews , D. A. and Effler , S. W. 2006a . Long-term assessment of the oxygen resources of a recovering urban lake, Onondaga Lake, NY . Lake Reserv Manage , 22 : 19 – 32 .
- Matthews , D. A. and Effler , S. W. 2006b . Long-term changes in the areal hypolimnetic oxygen deficit (AHOD) of Onondaga Lake: Evidence of sediment feedback . Limnol Oceanogr , 51 : 702 – 714 .
- Matthews , D. A. , Effler , S. W. , Matthews , C. M. , Siegfried , C. A. and Spada , M. E. 2001 . Responses of Onondaga Lake, New York, to early stages of rehabilitation: Unanticipated ecosystem feedbacks . Water Environ Res , 73 : 691 – 703 .
- Matthews , D. A. , Effler , S. W. , O'Donnell , S. M. , Driscoll , C. T. and Matthews , C. M. 2008 . Electron budgets for the hypolimnion of a recovering urban lake, 1989-2004: Response to changes in organic carbon deposition and availability of electron acceptors . Limnol Oceanogr , 53 : 743 – 759 .
- Murphy , C. B. 1973 . Effect of restricted use of phosphate-based detergents on Onondaga Lake . Science , 181 : 379 – 381 .
- Nürnberg , G. K. and Peters , R. H. 1984 . Biological availability of soluble reactive phosphorus in anoxic and oxic freshwaters . Can J Fish Aquat Sci , 41 : 757 – 765 .
- [NYSDEC] New York State Department of Environmental Conservation . 1998 . Total maximum daily loads for phosphorus in Onondaga Lake , Albany , NY : Division of Water .
- Onondaga County . 2007 . Onondaga Lake Ambient Monitoring Program 2006 Annual Report , Syracuse , NY : Onondaga County Department of Environmental Protection .
- Peng , F. , Effler , S. W. , O'Donnell , D. M. , Perkins , M. G. and Weidemann , A. D. 2007 . Role of minerogenic particles in light scattering in lakes and a river in Central New York . Appl Optics , 46 : 6577 – 6594 .
- Perkins , M. G. , Effler , S. W. , Peng , F. , Pierson , D. , Smith , D. G. and Agrawal , Y. C. 2007 . Particle characterization and settling velocities for a water supply reservoir during a turbidity event . J Environ Eng , 133 : 800 – 808 .
- Prestigiacomo , A. R. , Effler , S. W. , Hassett , J. M. and Michelanko , E. M. 2007 . Turbidity and suspended solids levels and loads in a sediment enriched stream: Implications for impacted lotic and lentic ecosystems . Lake Reserv Manage , 23 : 231 – 244 .
- Richards , R. P. and Baker , D. B. 1993 . Trends in nutrient and suspended sediment concentrations in Lake Erie tributaries, 1975–1990 . J Great Lakes Res , 19 : 200 – 211 .
- Richards , R. P. and Baker , D. B. 2002 . Trends in water quality in LEASEQ rivers and streams (Northwestern Ohio), 1975–1995 . J Environ Qual , 31 : 90 – 96 .
- Rowell , C. 1996 . Paleolimnology of Onondaga Lake: The history of anthropogenic impacts on lake water quality . Lake Reserv Manage , 12 : 35 – 45 .
- Rucinski , D. K. , Auer , M. T. , Watkins , D. W. , Effler , S. W. , O'Donnell , S. M. and Gelda , R. K. 2007 . Accessing assimilative capacity through a dual discharge approach . J Water Res Plan Manage , 133 : 474 – 485 .
- Tango , P. J. and Ringler , N. H. 1996 . The role of pollution and external refugia in structuring the Onondaga Lake fish community . Lake Reserv Manage , 12 : 81 – 90 .
- [USEPA] United States Environmental Protection Agency . 1991 . Guidance for water quality-based decisions: The TMDL process , Washington , DC : Office of Water . EPA 440-4-91-001
- [USEPA] United States Environmental Protection Agency . 1997 . Compendium of tools for watershed assessment and TMDL development , Washington , DC : Office of Water . EPA 841-B-97-006
- Walker , W. W. 1995 . FLUX: Stream Load Computations Version 4.5 , Vicksburg , MS : Environmental Laboratory, USAE Waterways Experiment Station .
- Young , T. C. , DePinto , J. V. , Flint , S. E. , Switzenbaum , S. M. and Edzwald , J. K. 1982 . Algal availability of phosphorus in municipal wastewater . J Water Pollut Control Fed , 54 : 1505 – 1516 .
- Young , T. C. , DePinto , J. V. , Martin , S. C. and Bonner , J. S. 1985 . Algal-available particulate phosphorus in the Great Lake basin . J Great Lakes Res , 11 : 434 – 446 .