Abstract
The ability to predict hypolimnetic dissolved oxygen concentrations in lakes and to track changes in concentrations over time in response to known environmental stressors is critical for effective lake management. The background concentrations of deepwater oxygen, in particular, provide important management benchmarks for assessing the impact of current and future shoreline residential development on water quality. Background can be defined as the conditions that exist in the absence of, or prior to, human influence. We compare 2 models commonly used to predict end-of-summer, volume-weighted hypolimnetic oxygen (VWHO) concentrations in Canadian Shield lakes. The paleoecological and empirical models are evaluated in their ability to predict present-day VWHO concentrations, and then compared in their predictions of background VWHO concentrations and in predictions of changes in VWHO from background to present-day conditions. The predictive power of the 59-lake paleoecological model (jackknifed r2 = 0.51, RMSEP = 2.18 mg/L) is comparable to other models that have used chironomids to predict the degree of hypolimnetic anoxia in lakes but is lower than that produced by the empirical modelling approach (r2 = 0.87, SE = 1.04 mg/L). However, this discrepancy may be offset by the enhanced realism of the paleoecological model, including its ability to predict declines in VWHO over time. The combined use of the paleoecological and empirical modelling approaches may allow lake managers to examine changes in deepwater oxygen concentrations in response to a single “targeted” stressor (e.g., residential shoreline development) and to multiple environmental stressors (e.g., climate change, hydrological management).
The background or “natural” water quality of a lake can be defined as the physical and chemical conditions that exist in the absence of, or prior to, human influence (CitationSmol 2008). Background concentrations of phosphorus and deepwater oxygen, well established measures of a lake's trophic status, provide important management benchmarks for assessing the impact of current and future shoreline residential development on water quality. Impairment of these measures may be quantified as a given threshold beyond background conditions (e.g., background concentrations plus 50% for phosphorus; CitationEnvironment Canada 2004), or as a known physiological boundary that, when crossed, threatens the survival and/or reproduction of a keystone species (e.g., dissolved oxygen concentration < 7 mg/L for lake trout [Salvelinus namaycush]; CitationEvans 2007).
The ability to predict hypolimnetic dissolved oxygen concentrations (DO) in lakes and to track changes in concentrations over time in response to known environmental stressors is critical. Many aquatic organisms require that DO be maintained within narrow physiological ranges (e.g., CitationClark et al. 2004, CitationPollock et al. 2007), and oxygen plays an essential role in the geochemical cycling of redox-sensitive elements or compounds (CitationNealson and Saffarini 1994). Modelling hypolimnetic DO in lakes, however, is not trivial. Spatial variation among lakes in morphometry and mixing regimes, and temporal variability in weather (i.e., air temperature and wind) may affect the strength and duration of stratification and the cycling of oxygen within lakes (CitationBurns 1995). Furthermore, oxygen levels can be both negatively and positively altered by human disturbances, such as the addition of nutrients from shoreline residential development (negatively: CitationDillon and Molot 1996), changes in water clarity associated with climate change and acid deposition (negatively or positively: CitationSchindler et al. 1996, CitationKeller 2007, CitationKeller et al. 2008) and hydrological management for flood control or navigation (positively: CitationQuinlan and Smol 2002).
Over the past 2 decades, quantitative models have been developed to predict present and historical hypolimnetic DO in lakes. An empirical limnological approach involves modelling the modern-day relationship between hypolimnetic DO and other limnological variables (e.g., total phosphorus concentrations), generating estimates of historical changes in these other variables and inserting these values into the predictive model to determine historical changes in hypolimnetic DO (e.g., CitationMolot et al. 1992). The empirical approach assumes a straightforward, linear relationship between total phosphorus and hypolimnetic oxygen (i.e., if phosphorus concentrations increase, hypolimnetic DO declines; CitationLind 1978). A paleoecological approach involves examining subfossil assemblages of nonbiting midges (Order Diptera, Family Chironomidae) in a training set of modern-day sediment samples and relating these assemblages to measured values of hypolimnetic DO to generate an inference model for reconstructing historical values. The paleoecological model is then applied to subfossil chironomid assemblages from pre-industrial sediment samples to infer historical data and to evaluate long-term changes in hypolimnetic DO (CitationLittle and Smol 2001, CitationQuinlan and Smol 2001). The paleoecological approach assumes that the variable being inferred is the most important variable influencing ecological structure in the indicator community (CitationBirks et al. 1990), an assumption that is rigorously tested (e.g., CitationQuinlan and Smol 2001) before making paleoecological inferences.
We evaluated and compared results from 2 models commonly used to predict volume-weighted hypolimnetic oxygen (VWHO) concentrations in Canadian Shield lakes. First, the models were evaluated in their ability to predict present-day hypolimnetic DO by comparing them to measured values of VWHO in 44 Precambrian Shield lakes in Ontario, Canada. Second, the models were compared with their predictions of background VWHO concentrations and to changes in these concentrations from the background to the present-day condition. We discuss the value and limitations of both modelling approaches and present an argument for the combined use of the models in lake management assessments.
Materials and methods
Study region
The Muskoka-Haliburton region of central Ontario (Canada) is located on the southern Precambrian Shield at the southern limit of the Boreal ecozone (44–45°N; 78–79°W). Forest composition in the region ranges from predominantly coniferous (e.g., Pinus strobus, Tsuga canadensis) to mixed hardwood (e.g., Acer saccharum, Fagus grandifolia). Local soils are generally shallow and acidic, overlying granitic (silicate) bedrock, with thicker deposits of clay, sand or gravel occurring locally (CitationJeffries and Snyder 1983). Mean annual air temperature and total annual precipitation are 4.5°C and 1034 mm, respectively (CitationRusak et al. 2002). Long-term monitoring and paleoecological data from the study region indicate that many of the study lakes have undergone significant temporal changes in water quality and ecology over the past few decades (CitationPaterson et al. 2008, CitationQuinlan et al. 2008, CitationYan et al. 2008). These changes are occurring regionally and coherently among lakes, and have been related to regional stressors such as changes in the deposition of strong acids (CitationWatmough and Aherne 2008), climatic forcing (CitationDillon et al. 1997) and human settlement (CitationDillon and Molot 1996). The study lakes vary morphometrically and chemically in accordance with the natural distribution of lakes in the region ().
Table 1 The location (latitude (°N) and longitude (°W)), lake surface areas (SA), maximum depths (Zmax), total phosphorus concentrations from water samples collected at spring or fall overturn (TP), and the mean end-of-summer volume-weighted hypolimnetic dissolved oxygen concentrations (VWHO) for the study lakes used in the development of the paleoecological and empirical models. Dissolved oxygen measurements are based on 1–4 annual profiles collected for each of the study lakes between 15 August and 15 September. VWHO was calculated for each year from profile data and bathymetric maps provided by the Ontario Ministries of Natural Resources and the Environment. TP concentrations were obtained from water samples collected at spring overturn in 1990–1992 or at fall overturn in 1996 or 1998.
Modelling approaches
Two modelling approaches were used to predict the mean VWHO of lakes at the end-of-summer period (i.e., 1 Sep). This metric of deepwater oxygen was selected because other oxygen metrics do not adequately describe end-of-summer conditions (e.g., Areal Hypolimnetic Oxygen Deficit; CitationCornett and Rigler 1979) or describe conditions in only a small portion of the hypolimnion (e.g., “bottom oxygen” 1 m above the sediment-water interface; Quinlan and Smol 2001). In the first approach, a paleoecological model was developed using the fossil remains of chironomids (Diptera: Chironomidae). Chironomids are ubiquitous in freshwater ecosystems and well-preserved in lake sediments. They are sensitive indicators of benthic and lake profundal conditions (CitationBrodersen and Quinlan 2006), as demonstrated in historical reconstructions of hypolimnetic oxygen concentrations (CitationFrancis 2001, CitationQuinlan and Smol 2001) and lake-level fluctuations (CitationKorhola et al. 2000).
Sediment cores were collected from the deepest basin of each lake using a CitationGlew (1989) gravity corer fitted with a 6.35-cm i.d. lucite tube. Cores were sectioned using a CitationGlew (1988) extruder at the lakeshore into 2 sediment sections: the top centimeter (representing present-day lake conditions) and a 1-cm interval taken at a minimum core depth of 20 cm (representing pre-industrial lake conditions). Although the precise date of the bottom sample of each core is unknown, radiometric 210Pb dating (CitationAppleby 2001) of sediment cores from more than 10 of the study lakes in the region have shown that background conditions are consistently reached at core depths of 15–20 cm (CitationClerk et al. 2000, CitationFaulkenham et al. 2003).
Approximately 3–52 g (wet weight) of sediment was processed for the identification of chironomid head capsules following CitationQuinlan and Smol (2002). Glass microscope slides of chironomid head capsules were prepared using Euparal© mounting medium, and specimens were identified using a Leitz Dialux compound light microscope at 125–630× magnification. Taxonomy followed CitationWiederholm (1983) and CitationWalker (1988). A total of 6801 chironomid head capsules were recovered and identified from the surface sediment of 86 study lakes in the study region. Of these sites, 59 were included in the development of the paleoecological model because they were thermally-stratifying lakes (and thus developed hypolimnia) and yielded a suitable number of head capsules to develop meaningful quantitative inferences (CitationQuinlan and Smol 2001). Following data screening and removal of rare taxa, 44 chironomid taxa remained for model development. Further information regarding taxonomic identifications and quality control are found in Quinlan and Smol (2001, 2002).
In the second approach, 2 models were linked to estimate background hypolimnetic oxygen profiles for each lake. First, Ontario's Lakeshore Capacity Model (LCM) was used to calculate the phosphorus budget for each lake based on natural and anthropogenic inputs of phosphorus, hydrologic and morphometric data, assumptions or estimates (CitationPaterson et al. 2006). The model output, the total phosphorus concentration of the lake during the spring overturn period, was subsequently used as an input variable in the oxygen submodel (described later). The background phosphorus concentration of each lake was modelled by setting anthropogenic inputs of phosphorus to zero (e.g., shoreline residential development including dwellings, resort units and camp sites, % watershed as pasture, % watershed as agriculture, % watershed as urban).
Using the equations presented in CitationMolot et al. (1992), the oxygen submodel was used to calculate hypolimnetic oxygen profiles for each lake at the end-of-summer period. The oxygen model was based on empirical relationships between oxygen concentration at specific depths in the lake, lake morphometry (e.g., the stratum volume/sediment surface area ratio), the total phosphorus concentration of the lake during spring overturn and the initial or spring oxygen concentration (see CitationMolot et al. 1992 for equations). In our calculations, we assumed no change in lake morphometry from background to present-day conditions. When combined with hypolimnetic volumes derived from bathymetric maps, oxygen concentrations were used to calculate the end-of-summer VWHO for each lake. Input data for the empirical models were available for 44 of the study lakes.
Predicted values from both models were compared to measured oxygen concentrations obtained from oxygen/temperature profiles taken at 1- or 2-m intervals using an analog YSI dissolved oxygen meter. One to 4 annual profiles were collected for each of the study lakes between 15 August and 15 September. Measured VWHO was calculated for each year from profile data and bathymetric maps provided by the Ontario Ministries of Natural Resources and the Environment.
Results and discussion
Comparing models: Predicted versus measured VWHO
The paleoecological and empirical approaches performed well in their prediction of mean end-of-summer VWHO, and correlations of predicted versus measured VWHO were significant for both models (). The predictive ability of the 59-lake paleoecological model (jackknifed r2 = 0.51, root mean square error of prediction [RMSEP] = 2.18 mg/L; ) is comparable to other models that have used chironomids to predict the degree of hypolimnetic anoxia (i.e., anoxic factor: CitationNürnberg 1995a, CitationQuinlan et al. 1998; bootstrapped r2 = 0.54), and the average summer dissolved oxygen concentration in lakes (CitationLittle and Smol 2001; jack-knifed r2 = 0.58). In our model, as has been reported in other studies (e.g., CitationLittle and Smol 2001), a bias in the prediction of VWHO exists at the ends of the environmental gradient, where measured values are over-predicted at the low end of the gradient and under-predicted at the high end. Referred to as an edge effect, this bias is common to models created using a weighted-averaging regression approach and is caused by a truncation of the species response curves near the ends of the environmental gradient. At the low end, this bias is further created by the zero value, which provides a finite lower limit to the VWHO gradient. However, as described by CitationLittle and Smol (2001), changes in the chironomid species assemblage may occur beyond this point as species tolerant to severe anoxia are replaced by littoral taxa.
Figure 1 Regression models of (a) chironomid-inferred mean end-of-summer volume-weighted hypolimnetic oxygen concentrations (VWHO) and (b) VWHO predicted using the empirical modelling approach versus VWHO calculated from measured profile data averaged over at least 3 years. The dotted lines represent 1:1. r2 jack and RMSEPjack are the jackknifed coefficient of determination and the jackknifed root mean square error of prediction, respectively. SE is the standard error.
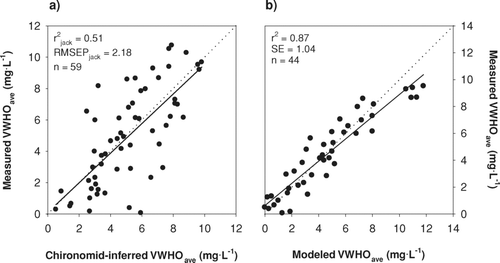
The empirical model produced a higher coefficient of prediction and a lower standard error than the paleoecological model in its prediction of mean end-of-summer VWHO (). With the exception of 5 large deep lakes, in which the empirical model over-predicted the observed VWHO, the predictions were close to the one-to-one line and showed minimal bias. Morphometry (i.e., lake shape and size), an input parameter that can be determined with a high degree of accuracy, accounted for the majority of the explained variance in the predicted end-of-summer values (CitationMolot et al. 1992) and thus may explain the close relationship between measured and predicted VWHO. In contrast to the paleoecological model, where the chironomid assemblage of a given lake will be shaped by many factors including oxygen concentrations, temperature, habitat structure and quality, and top-down trophic interactions (CitationBrodersen and Quinlan 2006), the empirical model was specifically calibrated to predict changes in oxygen concentrations in response to changes in total phosphorus concentrations only. Thus, the empirical model is conceptually much simpler, and a stronger relationship between measured and predicted values was expected. It is also possible that the predictive ability of the paleoecological inference model would be improved by increasing the number of lakes, thus strengthening estimates of species' optima and tolerances to VWHO concentrations, and by improving the taxonomic resolution of taxa, many of which are currently identified to the genus level.
Predicting background and long-term changes in VWHO
A comparison of paleoecological and empirical model predictions of present-day and background VWHO revealed a significant correlation between the 2 models across the region (). In general, the range of predicted values was smaller using the paleoecological model, again due to the edge effect discussed above. Despite significant agreement between the models, the 2 approaches provided very different interpretations of long-term changes in VWHO (). For the majority of lakes, the chironomid model inferred changes within the RMSEP, with approximately half the lakes showing a rise in VWHO and half showing a decline relative to background (pre-industrial) conditions. In contrast, the empirical model inferred either no change in VWHO (in lakes with no shoreline residential development) or a decrease in VWHO relative to background concentrations ().
Figure 2 A comparison of mean end-of-summer volume-weighted hypolimnetic oxygen concentrations (VWHO) predicted using the paleoecological and empirical modelling approaches. The models are compared in their prediction of present-day (left panel) and background (right panel) VWHO. The dotted lines represent 1:1.
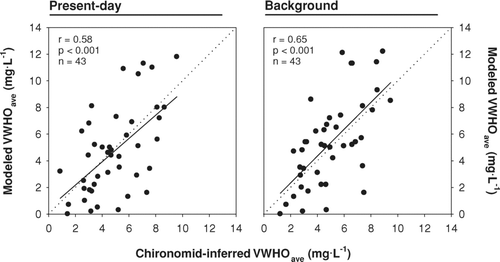
Model comparisons: Strengths and limitations
The models presented here use very different methods to predict the mean, end-of-summer VWHO concentration of lakes on the Canadian Shield. Although both models are limited by the range of lakes in which they were calibrated, they provide a distinct set of strengths and limitations in their predictions. The paleoecological model, for example, is more expensive and labor-intensive than the empirical approach. It requires the collection of sediment cores, sample preparation and the detailed taxonomic identification of chironomid remains in addition to the collection and synthesis of monitoring data required to test and calibrate the model. However, these limitations are offset by the enhanced realism of the paleoecological model, particularly in the evaluation of changes in VWHO over time or relative to background conditions.
Concentrations of VWHO in lakes are a function of the dissolved oxygen concentrations at depth, and the volume of the hypolimnia (CitationQuinlan et al. 2005). These measures, in turn, are influenced by many environmental factors that, when altered, may positively or negatively affect the end-of-summer VWHO (CitationQuinlan and Smol 2002). For example, oxygen depletion rates (and consequently end-of-summer VWHO) may be affected by hypolimnetic temperature, which influences metabolic rates of bacteria at the sediment-water interface (CitationCharlton 1980). A warmer climate may also result in earlier ice-off in spring, increasing the strength and duration of thermal stratification (CitationSchindler et al. 1996). If oxygen depletion rates are equivalent, a lake experiencing an extended period of thermal stratification would experience lower end-of-summer VWHO. Changes in lake depth may also influence VWHO by changing the volume of the hypolimnion, thus changing the mass of oxygen available for bacterial consumption during the period of stratification (CitationCharlton 1980, CitationCornett and Rigler 1987). Because chironomids respond to changes in the ambient oxygen levels regardless of the mechanism or direction of change (i.e., increases or decreases over time; ), the paleoecological model provides greater realism and a more complete picture of long-term environmental change (CitationSmol 2008).
Considering the typical context of water quality modelling (i.e., to evaluate and maintain ecological integrity in aquatic ecosystems), the enhanced realism of the paleoecological approach is important. Biological communities respond not only to low hypolimnetic DO, but also to the duration of low DO (CitationNürnberg 1995b, CitationLittle and Smol 2001). Thus, different taxa may be similarly affected by brief periods of anoxic or hypoxic conditions but have differing survivability during extended periods of anoxia (CitationHeinis and Davids 1993). Because chironomids are relatively long-lived (1–2 years in the profundal zone of temperate lakes; CitationOliver 1971), their subfossil assemblages preserved in lake sediments represent not only end-of-summer VWHO but also integrate the effects of the duration of anoxia experienced.
A rise in phosphorus concentrations with increased shoreline residential development, resulting in a decline in VWHO relative to background conditions, is one of many possible outcomes for lake ecosystems, but it is the only possible outcome predicted by the empirical model. A scenario may exist, for example, where current VWHO concentrations are higher than background levels (, Scenario 1). As discussed earlier, this could be caused by the construction of a dam for hydrological management that increased the volume of the hypolimnion (CitationQuinlan and Smol 2002), or by long-term declines in total phosphorus concentrations caused by drought (CitationSchindler et al. 1996), reforestation, and/or soil acidification (CitationHall and Smol 1996, CitationQuinlan et al. 2008). In this scenario, it is also possible that an increase in shoreline residential development resulted in a decline in VWHO not detectable relative to the increase in VWHO resulting from other environmental stressors (, Scenario 1). Indeed, a long-term monitoring record from Chub Lake in central Ontario suggests that this scenario is possible (). Despite an increase in shoreline residential development in this lake since the late-1970s, total phosphorus concentrations have declined and VWHO has increased over time (). This outcome cannot be predicted using the empirical model.
Figure 4 Conceptual diagrams showing the theoretical changes in mean end-of-summer volume-weighted hypolimnetic oxygen concentrations (VWHO) from background to current (present-day) levels. In Scenario 1 (left panel), a net increase in VWHO is caused by a multiple stressor effect resulting in an increase in VWHO (e.g., a rise in water levels that increases the volume of the hypolimnion) minus a decrease in VWHO associated with increased shoreline residential development (i.e., single stressor effect). In Scenario 2 (right panel), a net decline in VWHO is caused by a multiple stressor effect resulting in a decrease in VWHO (e.g., climate change resulting in increased strength and duration of stratification) and an increase in shoreline residential development (i.e., single stressor effect).
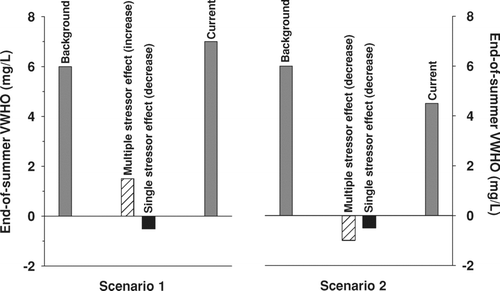
Figure 5 Long-term monitoring data (1976–2005) from Chub Lake in central Ontario showing a long-term decline in total phosphorus concentrations, concurrent with a long-term increase in mean end-of-summer volume-weighted hypolimnetic oxygen concentrations (bottom panel). These changes have occurred despite an increase in the number of shoreline residential developments from 1978 to 1997 (top right).
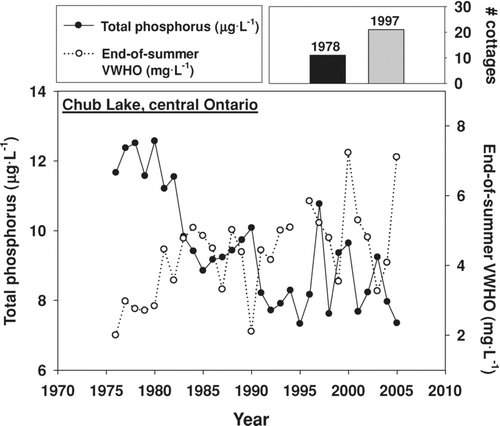
A second scenario may exist where climatic forcing has resulted in a shorter period of spring turnover, resulting in lower initial (spring) oxygen concentrations and a lower end-of-summer VWHO. Shoreline residential development may also have contributed to this decline, but because the net decrease in VWHO is caused by multiple factors it may be difficult to attribute cause (e.g., shoreline residential development) to the observed effect (i.e., decline in VWHO relative to background conditions; , Scenario 2).
In these examples, the empirical model can be used to isolate the impact of a single stressor (e.g., shoreline residential development) on mean end-of-summer VWHO, a distinct advantage for lake managers. Furthermore, the empirical model allows resource managers to predict possible future scenarios resulting from increased shoreline residential development or the conversion of forested catchments to intensive agriculture or urban land (CitationPaterson et al. 2006). Even more powerful, perhaps, the combined use of the empirical and paleoecological models allows lake managers to examine changes in deepwater oxygen concentrations in response to both a single targeted stressor and multiple environmental stressors, thus encapsulating the hypothetical scenarios presented above (). Moreover, when combined with other models, additional environmental indicators (e.g., diatoms in sediment cores to reconstruct phosphorus concentrations in lakes; CitationSmol 2008) and experimental work, resource managers may begin to tease apart the relative importance of the many environmental factors that affect oxygen concentrations in lakes and their impacts on biological communities. These efforts are particularly important when one considers that the protection of coldwater fisheries communities, typical keystone species in many aquatic ecosystems, is an integral part of ecosystem management (CitationGunn and Pitblado 2004).
Acknowledgments
We thank J. Glew, R. Hall, K. Neill, J. Havelock and S. Clerk for assistance with fieldwork. We also thank P. Dillon, N. Hutchinson, N. Yan and D. Galloway for advice on lake selection and logistical support. Measured oxygen and temperature profile data were provided by the Ontario Ministries of the Environment and Natural Resources. J. Little, J Kao, S. Clerk and K. Neill assisted with the sorting of samples for chironomid subfossils. Elements of this project were funded by an Ontario Ministry of the Environment Research Advisory Council Grant, NSERC Grants to J. Smol and R. Quinlan, and an Ontario Graduate Scholarship to R. Quinlan. We thank G. Nürnberg and an anonymous reviewer for valuable comments on this manuscript.
References
- Appleby , P. G. 2001 . “ Chronostratigraphic techniques in recent sediments ” . In Tracking environmental change using lake sediments, Volume 1: Basin analysis, coring, and chronological techniques , Edited by: Last , W. M. and Smol , J. P. 171 – 204 . Dordrecht , , Netherlands : Kluwer Academic Publishers .
- Birks , H. J. B. , Line , J. M. , Juggins , S. , Stevenson , A. C. and ter Braak , C. J. F. Diatoms and pH reconstruction . Phil. Trans. R. Soc. Lond. B. , 327 263 – 278 .
- Brodersen , K. P. and Quinlan , R. 2006 . Midges as palaeoindicators of lake productivity, eutrophication and hypolimnetic oxygen . Quat. Sci. Rev. , 25 : 1995 – 2012 .
- Burns , N. M. 1995 . Using hypolimnetic dissolved oxygen depletion rates for monitoring lakes . N. Z. J. Mar. Freshw. Res. , 29 : 1 – 11 .
- Charlton , M. N. 1980 . Hypolimnion oxygen consumption in lakes: discussion of productivity and morphometry effects . Can. J. Fish Aquat. Sci. , 37 : 1531 – 1539 .
- Clark , B. J. , Dillon , P. J. and Molot , L. A. 2004 . “ Lake trout (Salvelinus namaycush) habitat volumes and boundaries in Canadian Shield lakes ” . In Boreal Shield watersheds: Lake trout ecosystems in a changing environment , Edited by: Gunn , J. M. , Steedman , R. J. and Ryder , R. A. 111 – 117 . Boca Raton , FL : Lewis Publishers .
- Clerk , S. , Hall , R. I. , Quinlan , R. and Smol , J. P. 2000 . Quantitative inferences of past hypolimnetic anoxia and nutrient levels from a Canadian Precambrian Shield lake . J. Paleolimnol. , 23 : 319 – 336 .
- Cornett , R. J. and Rigler , F. H. 1979 . Hypolimnetic oxygen deficits: their prediction and interpretation . Science. , 205 : 580 – 581 .
- Cornett , R. J. and Rigler , F. H. 1987 . Vertical transport of oxygen into the hypolimnion of lakes . Can. J. Fish Aquat. Sci. , 44 : 852 – 858 .
- Dillon , P. J. and Molot , L. A. 1996 . Long-term phosphorus budgets and an examination of a steady-state mass balance model for central Ontario lakes . Water Res. , 30 : 2273 – 2280 .
- Dillon , P. J. , Molot , L. A. and Futter , M. 1997 . The effect of El Niño-related drought on the recovery of acidified lakes . Environ. Monitor Assess. , 46 : 105 – 111 .
- Environment Canada . 2004 . Canadian Guidance Framework for the management of phosphorus in freshwater systems 133 Report No. 1–8. ISBN 0–662-36111–3
- Evans , D. O. 2007 . Effects of hypoxia on scope-for-activity and power capacity of lake trout (Salvelinus namaycush) . Can. J. Fish Aquat. Sci. , 64 : 345 – 361 .
- Faulkenham , S. E. , Hall , R. I. , Dillon , P. J. and Karst-Riddoch , T. 2003 . Effects of drought-induced acidification on diatom communities in acid-sensitive Ontario lakes . Limnol. Oceanogr. , 48 : 1662 – 1673 .
- Francis , D. R. 2001 . A record of hypolimnetic oxygen conditions in a temperate multi-depression lake from chemical and chironomid remains . J. Paleolimnol. , 25 : 351 – 365 .
- Glew , J. R. 1988 . A portable extruding device for close interval sectioning of unconsolidated core samples . J. Paleolimnol. , 1 : 229 – 234 .
- Glew , J. R. 1989 . A new trigger mechanism for sediment samplers . J. Paleolimnol. , 2 : 241 – 243 .
- Gunn , J. M. and Pitblado , R. 2004 . “ Lake trout, the Boreal Shield, and the factors that shape lake trout ecosystems ” . In Boreal Shield Watersheds: Lake trout ecosystems in a changing environment , Edited by: Gunn , J. M. , Steedman , R. J. and Ryder , R. A. 3 – 19 . Boca Raton , FL : Lewis Publishers .
- Hall , R. I. and Smol , J. P. 1996 . Paleolimnological assessment of long-term water-quality changes in south-central Ontario lakes affected by cottage development and acidification . Can. J. Fish Aquat. Sci. , 53 : 1 – 17 .
- Heinis , F. and Davids , C. 1993 . Factors governing the spatial and temporal distribution of chironomid larvae in the Maarsseveen Lakes with special emphasis on the role of oxygen conditions . Neth. J. Aquat. Ecol. , 27 : 21 – 34 .
- Jeffries , D. S. and Snyder , W. R. 1983 . Geology and geochemistry of the Muskoka-Haliburton study area , Ontario Ministry of the Environment . Data Report No. DR 83/2
- Keller , W. 2007 . Implications of climate warming for Boreal Shield lakes: a review and synthesis . Environ. Rev. , 15 : 99 – 112 .
- Keller , W. , Paterson , A. M. , Somers , K. M. , Dillon , P. J. , Heneberry , J. and Ford , A. A . 2008 . Relationships between dissolved organic carbon concentrations, weather, and acidification in small Boreal Shield lakes . Can. J. Fish Aquat. Sci. , 65 : 786 – 795 .
- Korhola , A. , Olander , H. and Blom , T. 2000 . Cladoceran and chironomid assemblages as quantitative indicators of water depth in subarctic Fennoscandian lakes . J. Paleolimnol. , 24 : 43 – 54 .
- Lind , O. T. 1978 . Interdepression differences in hypolimnetic areal relative oxygen deficits in Douglas Lake, Michigan . Verh. Int. Verein. Limnol. , 20 : 2689 – 2696 .
- Little , J. L. and Smol , J. P. 2001 . A chironomid-based model for inferring late-summer hypolimnetic oxygen in southeastern Ontario lakes . J. Paleolimnol. , 26 : 259 – 270 .
- Molot , L. A. , Dillon , P. J. , Clark , B. J. and Neary , B. P. 1992 . Predicting end-of-summer oxygen profiles in stratified lakes . Can. J. Fish Aquat. Sci. , 49 : 2363 – 2372 .
- Nealson , K. H. and Saffarini , D. D. 1994 . Iron and manganese in anaerobic respiration: environmental significance, physiology, and regulation . Ann. Rev. Microbiol. , 48 : 311 – 343 .
- Nürnberg , G. K. 1995a . Quantifying anoxia in lakes . Limnol Oceanogr. , 40 : 1100 – 1111 .
- Nürnberg , G. K. 1995b . The Anoxic Factor, a quantitative measure of anoxia and fish species richness in central Ontario lakes . Trans. Am. Fish. Soc. , 124 : 677 – 686 .
- Oliver , D. R. 1971 . Life history of the Chironomidae . Ann. Rev. Entomol. , 16 : 211 – 230 .
- Paterson , A. M. , Dillon , P. J. , Hutchinson , N. J. , Futter , M. N. , Clark , B. J. , Mills , R. B. , Reid , R. A. and Scheider , W. A. 2006 . A review of the components, coefficients and technical assumptions of Ontario's Lakeshore Capacity Model . Lake Reserv. Manage. , 22 : 7 – 18 .
- Paterson , A. M. , Winter , J. G. , Nicholls , K. H. , Clark , B. J. , Ramcharan , C. W. , Yan , N. D. and Somers , K. M. 2008 . Long-term changes in phytoplankton composition in seven Canadian Shield lakes in response to multiple anthropogenic stressors . Can. J. Fish Aquat. Sci. , 65 : 846 – 861 .
- Pollock , M. S. , Clarke , M. S. J. and Dubé , M. G. 2007 . The effects of hypoxia on fishes: from ecological relevance to physiological effects . Environ. Rev. , 15 : 1 – 14 .
- Quinlan , R. , Hall , R. I. , Paterson , A. M. , Cumming , B. F. and Smol , J. P.JP . 2008 . Long-term assessments of ecological effects of anthropogenic stressors on aquatic ecosystems from paleoecological analyses: challenges to perspectives of lake management . Can J Fish Aquat Sci. , 65 : 933 – 944 .
- Quinlan , R. , Paterson , A. M. , Smol , J. P. , Douglas , M. S. V. and Clark , B. J. 2005 . Comparing different methods of calculating volume-weighted hypolimnetic oxygen (VWHO) in lakes . Aquat. Sci. , 67 : 97 – 103 .
- Quinlan , R. and Smol , J. P. 2001 . Chironomid-based inference models for estimating end-of-summer hypolimnetic oxygen from south-central Ontario shield lakes . Freshwater Biol. , 46 : 1529 – 1551 .
- Quinlan , R. and Smol , J. P. 2002 . Regional assessment of long-term hypolimnetic oxygen changes in Ontario (Canada) shield lakes using subfossil chironomids . J. Paleolimnol. , 27 : 249 – 260 .
- Quinlan , R. , Smol , J. P. and Hall , R. I. 1998 . Quantitative inferences of past hypolimnetic anoxia in south-central Ontario lakes using fossil midges (Diptera: Chironomidae) . Can. J. Fish Aquat. Sci. , 55 : 597 – 596 .
- Rusak , J. A. , Yan , N. D. , Somers , K. M. , Cottingham , K. L. , Micheli , F. , Carpenter , S. R. , Frost , T. M. , Paterson , M. J. and McQueen , D. J. 2002 . Temporal, spatial and taxonomic patterns of crustacean zooplankton variability in unmanipulated north-temperate lakes . Limnol. Oceanogr. , 47 : 613 – 625 .
- Schindler , D. W. , Bayley , S. E. , Parker , B. R. , Beaty , K. G. KG , Cruikshank , D. R. , Fee , E. J. , Schindler , E. U. and Stainton , M. P. 1996 . The effects of climatic warming on the properties of boreal lakes and streams at the Experimental Lakes Area, northwestern Ontario . Limnol. Oceanogr. , 41 : 1004 – 1017 .
- Smol , J. P. 2008 . Pollution of lake and rivers: A paleoenvironmental perspective. , 2nd ed. , Oxford , , England : Blackwell Publishing .
- Walker , I. R. 1988 . Late Quaternary palaeoecology of Chironomidae (Diptera: Insecta) from lake sediments in British Columbia , PhD dissertation Burnaby, BC , , Canada : Simon Fraser University .
- Watmough , S. A. and Aherne , J. 2008 . Estimating calcium weathering rates and future lake calcium concentrations in the Muskoka-Haliburton region of Ontario . Can. J. Fish Aquat. Sci. , 65 : 821 – 833 .
- Wiederholm , T. 1983 . Chironomidae of the Holarctic region: keys and diagnoses. Part 1 – Larvae . Entomol Scand Suppl. , 19 : 1 – 457 .
- Yan , N. D , Paterson , A. M. , Somers , K. M. and Scheider , W. A. 2008 . An introduction to the Dorset special issue: transforming understanding of factors that regulate aquatic ecosystems on the southern Canadian Shield . Can. J. Fish Aquat. Sci. , 65 : 781 – 785 .