Abstract
During summers (May–Aug) 2004–2006, 177 Missouri reservoirs were sampled monthly at open pelagic locations to assess regional patterns in microcystin concentration, frequency of occurrence over successive summer seasons and relations with environmental factors. Microcystin was detected in 58% of Missouri reservoirs and 23% of samples (n = 1402). Total microcystin concentrations, measured by enzyme-linked immunosorbent assay, ranged from ≤ 0.1 to 21 μg/L. Concentrations ≥ 1 μg/L were detected in 10% of reservoirs and exceeded the human health concern limit of 20 μg/L once in a single sample. Microcystin occurred throughout summer, with maximum concentrations in individual reservoirs observed in each month. Occurrence was consistent across years, with about one-half of Missouri reservoirs having detectable microcystin each summer. Eleven reservoirs with microcystin maxima ≥1 μg/L were sampled multiple seasons; of these, 8 had detectable microcystin each summer, which indicates that short-term surveys can identify water bodies with the greatest potential for toxin production. Eutrophic reservoirs in northern Missouri had the greatest microcystin occurrence and concentrations. Reservoirs with detectable microcystin had significantly (p < 0.01) greater nutrient and chlorophyll values and significantly shallower Secchi depths than reservoirs without detection. All correlations, however, had r-values ≤ 0.35, and bivariate plots indicated nonlinear trends. Cylindrospermopsin was measured by enzyme-linked immunosorbent assay in 36 reservoirs once in late summer 2006; 14% had small detectable levels (total concentrations < 1 μg/L). This is the first report of cylindrospermopsin in Missouri.
Microcystins (MC) are a class of cyanobacterial hepatotoxins common in lakes and reservoirs worldwide (CitationChorus and Bartram 1999, Huisman et al. 2005). Regional studies indicate MC occurs frequently in the Midwestern United States and may reach concentrations of human health concern (CitationMcDermott et al. 1995, CitationDodds 1996, CitationGraham et al. 2004, CitationHedman et al. 2008, CitationLindon and Heiskary 2008). Whereas several studies in the United States have assessed regional patterns in MC occurrence (CitationMcDermott et al. 1995, CitationDodds 1996, CitationZimba and Grimm 2003, CitationGraham et al. 2004, CitationBoyer 2007, CitationWilliams et al. 2007, CitationHedman et al. 2008, CitationLindon and Heiskary 2008), few have evaluated frequency of occurrence during successive summer seasons. Likewise, few regional studies have developed empirical relations between environmental factors and MC concentration. Knowledge of MC occurrence during summer and the general environmental conditions associated with elevated concentrations will improve understanding of factors favoring toxic blooms.
Most Missouri reservoirs are located in the agricultural Osage and Glacial Plains, the Ozark Highlands, which are covered by forest and pasture, or the ecotonal Ozark Border section (CitationJones et al. 2008a). Reservoir nutrients are affected by hydraulic flushing and depth but exhibit an increase with cropland and a decrease with forest cover (CitationJones et al. 2004, Citation2008b). An initial survey of particulate MC (net collections > 64 μm) in Missouri reservoirs indicated MC occurrence was more common in the eutrophic Osage and Glacial Plains reservoirs than those in the Ozark Highlands (CitationGraham et al. 2004). A subsequent study of MC in the size fractions of natural plankton communities indicated particulate MC underestimates total toxin concentration because of algal loss in net collections (CitationGraham and Jones 2007). This study reports total MC concentrations in whole water samples collected in conjunction with a statewide inventory of 177 Missouri reservoirs during summers 2004–2006 (CitationJones et al. 2008a). Data on the occurrence and concentration of MC are analyzed to describe regional patterns, detection frequency across three summer seasons and relations with reservoir nutrients and transparency. Also, cylindrospermopsin, another cyanobacterial hepatotoxin (CitationChorus and Bartram 1999), was measured in 36 reservoirs once in late summer 2006 to gather preliminary data on its occurrence; algal taxa capable of producing this toxin (Anabaena, Aphanizomenon and Cylindrospermopsis) can dominate summer algal assemblages in Missouri reservoirs (CitationGraham et al. 2006a, CitationJones et al. 2008a). This information can facilitate human health protection in lakes and reservoirs used for recreational activities and drinking-water supplies.
Methods
Sample collection
Missouri reservoirs (n = 177) were sampled May through August 2004–2006: 94 were sampled one summer season; 45 were sampled 2 summers; and 38 were sampled all 3 summers. Most reservoirs were sampled monthly (n = 3 or 4 per summer), with individual reservoirs sampled on 3–26 occasions during this study. The reservoirs encompassed the full range of landscape, morphological and limnological conditions typical of Missouri reservoirs (; Jones et al. 2004, 2008a, 2008b).
Table 1 Provincial medians (med) and ranges of landscape, morphological, and limnological variables.
Temperature, dissolved oxygen and Secchi transparency were measured at open pelagic locations in each reservoir, typically near the dam. Water samples were composited from the surface layer (0.25–0.5 m) and analyzed for total MC, conductivity (μS), turbidity (NTU), chlorophyll (Chl; uncorrected for degradation products), total phosphorus (TP), total nitrogen (TN), non-volatile suspended solids (NVSS), volatile suspended solids (VSS), total suspended solids (TSS), and dissolved organic carbon (DOC). Whole-water samples underwent 3 freeze-thaw cycles after CitationGraham and Jones (2007) to lyse cyanobacterial cells and allow determination of total MC. In 2004, Envirogard® enzyme-linked immunosorbent assay (ELISA) kits (detection limit 0.1 μg/L; kit is cross-reactive with microcystin-LR, -YR, and -RR and nodularin) were used to measure MC. During 2005–2006, Abraxis® ELISA kits were used (detection limit 0.1 μg/L; -adda specific). Both kits provided similar results, and among-reservoir variability was greater than among-kit variability (J. Graham, unpublished data). Total cylindrospermopsin (CYL) was measured in 36 Missouri reservoirs once in late summer 2006 using Abraxis® ELISA kits (detection limit 0.04 μg/L). DOC was determined colorimetrically (Technicon Method No. 451–76W and Ontario Ministry of the Environment document JC 7501, 1972). Details of other analytical methods and land-use data are presented elsewhere (CitationKnowlton and Jones 1995, CitationGraham et al. 2004, CitationGraham and Jones 2007, CitationJones et al. 2008a, Citation2008b).
Statistical analyses
Reservoir trophic status was determined using geometric means (n = 3–18 per summer), which were averaged across all seasonal means (n = 1–3), and criteria for Missouri reservoirs (CitationJones et al. 2008a). Summary statistics were calculated using all available data; where MC was not detected, values of 0.05 μg/L (one-half the method detection limit) were substituted (CitationSokal and Rohlf 1995). All data were grouped into 3 MC categories based on concentration: no detectable MC; MC between 0.1–1 μg/L; and MC > 1 μg/L. Detections of large MC concentrations were too few to allow creation of additional MC categories above 1 μg/L. Significant differences in environmental variables associated with each MC category were determined using one-way analysis of variance (ANOVA) followed by Tukey's pairwise comparisons (CitationSokal and Rohlf 1995). Relations between total MC concentration and environmental variables were developed using Pearson correlation analysis (CitationSokal and Rohlf, 1995). All data were log10 transformed (land-use data were logit transformed) for ANOVA and correlation analyses (CitationSokal and Rohlf 1995). Statistical analyses were performed using SAS® (9.1), and significance was set at p < 0.05 for all analyses.
Bivariate plots of the relations between total MC concentration and environmental variables showed trends that were not linearized through transformation. Microcystin was undetectable across the range of all variables, and the shape of any given relation was defined by the upper limits created by MC maxima. Nonlinear interval-maxima regression (IMR) (CitationBlackburn et al. 1992, CitationScharf et al. 1998) was used to define the shape of these upper limits after CitationGraham et al. (2004). Each independent variable was divided into equal increments, resulting in 10–16 intervals. The maximum MC concentration and the associated environmental variable value were obtained from each interval and used in nonlinear regression analysis (CitationBlackburn et al. 1992, CitationScharf et al. 1998). Microcystin detection frequency in each interval was calculated by dividing the number of detections in the interval by the total number of samples (n = 1402). We performed IMR using SigmaPlot® (10.0) and set significance at p < 0.05.
Results
Descriptive limnology
Missouri reservoirs encompass a broad range of trophic conditions (CitationJones et al. 2008a; ). Most (79%) reservoirs sampled in 2004–2006 were mesotrophic or eutrophic, with 7% oligotrophic and 14% hypereutrophic. Based on TN:TP ratios (4–180), potential phosphorus limitation (TN:TP > 17) or co-limitation (17 < TN:TP > 10) by phosphorus and nitrogen (CitationForsberg and Ryding 1980) was common (93% of samples). Most (88%) samples had Chl:TP ratios substantially < 1, suggesting bloom conditions were uncommon (CitationWhite et al. 1985; ).
Regional patterns in limnology matched those described by CitationJones et al. (2008a), with the greatest nutrient and Chl values and the shallowest Secchi depths occurring in Osage and Glacial Plains reservoirs (). Median nutrient and Chl values in the Plains were 2–3 times greater than in the Ozark Highlands, and the Ozark Border was intermediate; median Secchi depth in the Ozark Highlands was 2 times greater than observed in other physiographic sections, including the Ozark Border ().
Microcystin occurrence and concentration
Overall, 58% of Missouri reservoirs (n = 177) and 23% of samples (n = 1402) had detectable MC ( and ). Total MC concentrations ranged from 0.1 to 21 μg/L but were usually < 1.0 μg/L (; and ). Few Missouri reservoirs (10%) had MC concentrations exceeding the World Health Organization (WHO) finished drinking-water guideline of 1 μg/L (; and ), and the WHO recreational guideline of 20 μg/L (CitationChorus and Bartram 1999) was exceeded once during our study. Of the 36 reservoirs sampled for CYL during late summer 2006, 14% had low detectable levels (0.12–0.81 μg/L).
Table 2 Regional means, medians, and maxima of total microcystin values. Summary statistics are based on samples collected in each region.
Figure 1 Physiographic location of reservoirs sampled in Missouri and total microcystin occurrence and concentration.
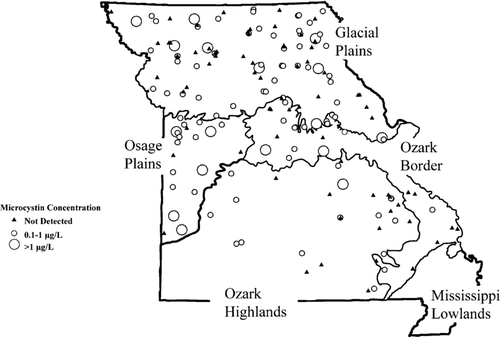
Figure 2 Occurrence and concentration of total microcystin in Missouri reservoirs. The World Health Organization (WHO) microcystin guidelines for finished drinking water and full-body contact recreation are 1 and 20 μg/L, respectively. (A) Occurrence and concentration among samples (n = 1402), (B) occurrence and concentration among reservoirs (n = 177).
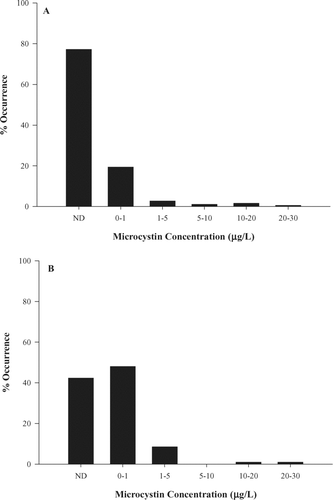
Occurrence and concentration of MC were greatest in eutrophic Osage and Glacial Plains reservoirs (; ). It occurred most frequently in Osage Plains reservoirs (80% of reservoirs, 34% of samples), although the greatest concentrations were collected from Glacial Plains reservoirs (mean = 0.32 μg/L, max = 21 μg/L; ). Despite general regional patterns, MC values > 1 μg/L were observed throughout Missouri, with the exception of the southern Ozark Highlands (; ).
Microcystin occurred in Missouri reservoirs throughout summer with mean, median and maximum concentrations being similar across summer months during the 3 summer seasons sampled for this study; however, MC was detected about twice as often in June through August compared to May (). Occurrence and concentration of MC were similar across years. Between 42 and 52% of reservoirs and 20–26% of samples had detectable MC each summer (). Mean and median MC concentrations were similar among summers, though 2006 maxima were an order of magnitude less than the others (). Of the 83 reservoirs sampled during 2 or 3 summers, 60% had detectable MC in more than one summer. Within this group, 11 reservoirs had MC maxima ≥ 1 μg/L and 8 (73%) had detectable MC each summer of sample collection, indicating frequent MC occurrence in some reservoirs.
Table 3 Monthly means, medians, and maxima of total microcystin values. Summary statistics are based on samples collected during each month.
Table 4 Annual means, medians, and maxima of total microcystin values. Summary statistics are based on samples collected during each year.
Relation with environmental variables
We detected MC in reservoirs of all trophic status but occurrence and concentration increased across the trophic gradient: 33% of oligotrophic (n = 12), 45% of mesotrophic (n = 40), 60% of eutrophic (n = 100), and 80% (n = 25) of hypereutrophic reservoirs had detectable MC. Maximum concentrations in eutrophic and hypereutrophic reservoirs were 2 orders of magnitude greater than in oligotrophic reservoirs and one order of magnitude greater than in mesotrophic reservoirs ().
Table 5 Trophic class means, medians, and maxima of total microcystin values. Summary statistics are based on samples collected in each trophic class.
As indicated by occurrence and concentration across the trophic gradient (), nutrient status, Chl content and transparency differed among the 3 MC concentration categories (). Mean nutrient and Chl concentrations were greatest in the MC > 1 μg/L category and smallest in the nondetect category; the MC 0.1–1 μg/L category was intermediate. Total N and TP significantly increased by ∼ 2-fold across the MC categories, and Chl by ∼ 4-fold (). Secchi transparency showed the opposite pattern. Mean values decreased significantly by ∼ 2-fold across the MC categories (). Mean TN:TP ratios were significantly greater in the nondetect and MC 0.1–1 μg/L categories (19–20) than the > 1 μg/L category (15), although the difference is not as marked as observed for other variables (). Several landscape and morphological variables also differed among the 3 MC concentration categories, likely reflecting effects on reservoir nutrient values (CitationJones et al. 2004, Citation2008b).
Table 6 Comparison of environmental variables among three microcystin concentration categories: no detectable microcystin (nd), total microcystin concentrations between 0.1–1 μg/L (0.1–1), and total microcystin concentrations greater than 1 (> 1). Significant differences between means were determined using one-way ANOVA and Tukey's pairwise comparisons.
Despite increases in occurrence and concentration with elevated nutrients and reduced transparency ( and 6), correlations between MC and trophic state variables were weak (TN: r = 0.31, TP: r = 0.26, Secchi: r = −0.19; all n = 1402 and all p < 0.01). Correlations of MC concentration with other measured variables in also were weak (all r ≤ 0.35, data not shown). Similar to CitationGraham et al. (2004), MC-TN and MC-TP IMR maxima in Missouri reservoirs were characterized by unimodal curves (r2 = 0.87 and 0.75, respectively; both p < 0.01), and MC-Secchi IMR maxima were characterized by exponential decline (r2 = 0.93, p < 0.01; ). Frequency distributions of MC occurrence along these environmental gradients showed similar patterns. Along the TN gradient the greatest MC values (> 10 μg/L) occurred between 600 and 1900 μg/L; for TP, MC peaked between 30 and 170 μg/L, ranges indicative of eu-hypereutrophic conditions in Missouri reservoirs (CitationJones et al. 2008a; ). Microcystin > 10 μg/L occurred at Secchi depths < 2 m, encompassing meso-hypereutrophic conditions (). The most frequent detection of MC occurred within narrower environmental ranges. We detected MC most frequently at TN between 600 and 1,200 μg/L (eutrophic conditions), TP between 15 and 70 μg/L (meso-eutrophic conditions) and Secchi depths between 0.5 and 1.0 m (eutrophic conditions), a result likely due in part to the large number of samples collected within these ranges ().
Figure 3 Total microcystin (MC), total nitrogen (TN), total phosphorus (TP) and Secchi bivariate relations. Data shown as grouped into intervals for interval-maxima regression (IMR). Curves were estimated using IMR. Black points indicate the data used for IMR analysis (n = 8–16) and gray points indicate all other data. Bar graphs represent frequency distributions of MC detection within each interval used for IMR. Hash marks on the x-axes indicate oligotrophic/mesotrophic, mesotrophic/eutrophic and eutrophic/hypereutrophic cutpoints for Missouri reservoirs as defined by Jones et al. 2008a. (A) MC–TN relation (r2 = 0.87, p < 0.01, values for line fitted to black points only), (B) MC–TP relation (r2 = 0.75, p < 0.01), (C) MC–Secchi relation (r2 = 0.93, p < 0.01). All n = 1402.
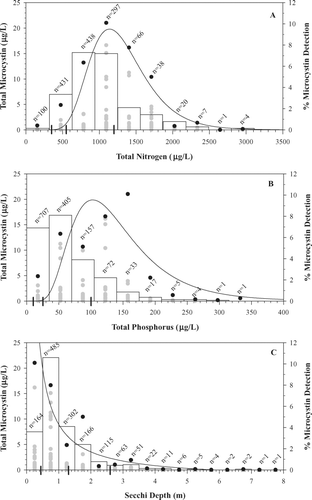
Discussion
Cyanobacteria frequently dominate summer algal biomass in Missouri reservoirs, and Anabaena is the most commonly occurring potential MC producer (CitationJones et al. 2008a). Microcystin was common in Missouri reservoirs, but few concentrations were large enough to cause human health concerns (). Occurrence and concentrations were similar across years, with approximately 50% of reservoirs sampled having detectable MC each summer (). Widespread occurrence with relatively few large values is characteristic of regional MC studies worldwide (CitationChorus and Bartram 1999, CitationGraham et al. 2004, CitationBoyer 2007, CitationWilliams et al. 2007, CitationHedman et al. 2008), a consequence of extreme temporal and spatial variability in MC concentrations (CitationLindholm 1991, CitationKotak et al. 1995, CitationWelker et al. 2003). Among reservoirs with MC maxima ≥1μg/L, 73% had detectable MC each summer, indicating seasonal inventory studies can identify reservoirs with potential for toxin production.
Microcystin maxima in Missouri reservoirs (21 μg/L) were orders of magnitude less than cyanobacterial blooms in Wisconsin (7,600 μg/L) and Minnesota (8,400), although such large values are not necessarily typical of Midwestern blooms (CitationHedman et al. 2008, CitationLindon and Heiskary 2008). The monthly collections from open-water pelagic locations likely missed concentrated surface accumulations of cyanobacteria; therefore, concentrations large enough to cause human-health concerns during recreational activities are likely under-represented (CitationChorus and Bartram 1999, CitationGraham et al. 2008, CitationLindon and Heiskary 2008). Toxic cyanobacterial blooms have been documented in the Midwestern United States since the late 1800s (CitationYoo et al. 1995), and anecdotal reports of animal illness and death after exposure to cyanobacterial blooms are common throughout the region, including Missouri (CitationGraham 2006).
About one-half of reservoirs in this study had detectable MC, similar to the earlier findings of CitationGraham et al. (2004) in Missouri. CitationGraham et al. (2004) sampled particulate MC using a 64-μm mesh plankton net, which may enhance detections but under-represent total concentrations by loss of algal material through the net (CitationGraham and Jones 2007). Maximum MC concentrations were ∼3 μg/L in the CitationGraham et al. (2004) study, compared to 21 μg/L in this dataset. These MC data come from whole-water samples, which better represent the entire cyanobacterial community and more accurately estimate MC concentration (CitationGraham and Jones 2007).
Metalimnetic populations of potential MC producing cyanobacteria occasionally bloom at depth, particularly in mesotrophic systems (CitationLindholm 1991, CitationGraham et al. 2008). Collection of surface samples will miss detection of these subsurface blooms and may result in underestimates of toxin occurrence. In Missouri reservoirs, there is typically little difference in Chl values at the surface and at the Secchi depth, indicating subsurface Chl peaks are uncommon (CitationJones et al. 2008a); therefore, subsurface cyanobacterial blooms likely do not represent a large proportion of microcystin occurrences in Missouri reservoirs.
This is the first report of CYL in Missouri. Potential CYL producers, including Anabaena, Aphanizomenon and Cylindrospermopsis, can dominate summer algal assemblages in Missouri reservoirs (CitationGraham et al. 2006a, CitationJones et al. 2008a), but the toxin was detected in few samples and always at concentrations < 1 μg/L. In most of the United States, CYL occurs relatively infrequently (< 10% of samples) and when detected usually at concentrations < 1 μg/L (CitationBoyer 2007, CitationHedman et al. 2008), although in Florida CYL has occurred in up to 30% of samples (CitationWilliams et al. 2007) and at concentrations as large as 200 μg/L (CitationBurns 2008).
In temperate climates, toxic cyanobacterial blooms historically have occurred most frequently during late summer–early fall (CitationChorus and Bartram 1999, Huisman et al. 2005); however, in this study, there were no distinct patterns during summer, and MC maxima were observed anytime between May and August (). Similarly, MC concentrations of concern for recreational exposure were observed from May to September in 12 eutrophic Minnesota lakes (CitationLindon and Heiskary, 2008). Microcystin maxima may have occurred during months other than May–August in Missouri reservoirs. Previous studies in Missouri have documented year-round MC occurrence, and peak concentrations occasionally occur during winter (CitationGraham et al. 2006a, Citation2006b). Thus, MC is a potential recreational concern throughout summer and a potential drinking water concern year-round in Midwestern reservoirs and lakes.
Missouri reservoirs with the greatest MC values had increased nutrients and a reduced light environment (; ), characteristics conducive to cyanobacterial dominance (CitationChorus and Bartram 1999). General patterns in MC with respect to trophic state match these patterns, with peak occurrence and concentrations in eutrophic to hypereutrophic reservoirs (; ). Even so, MC occurred in reservoirs of all trophic status and large nutrient values and shallow Secchi depths were not consistently related to elevated MC values ( and ; ). Because of co-occurrence of toxic and nontoxic cyanobacterial strains (CitationVézie et al. 1998) and the range of factors that may drive cyanobacterial community dynamics (CitationReynolds 1998), regional relations between environmental variables and MC occurrence and concentration are invariably complex (CitationKotak et al. 2000, Chorus 2001, CitationGraham et al. 2004).
Nonlinear relations that describe MC maxima along gradients of nutrients and light () were similar to those described by CitationGraham et al. (2004) for particulate MC in the Midwest; however, TN and TP ranges where MC maxima were encountered in Missouri are shifted to peak at lower nutrient levels and are narrower than those defined for the region (; Graham et al. 2004). Nonlinear interval-maxima regression is limited by the range of MC values and environmental conditions encountered (CitationBlackburn et al. 1992, CitationScharf et al. 1998, CitationGraham et al. 2004). Concentrations of MC in Missouri were orders of magnitude greater, and TN and TP values orders of magnitude less, than in the midcontinent region sampled by CitationGraham et al. (2004). The Midwestern relations are likely more representative of global conditions and are similar to relations described in Germany (Chorus 2001) and Alberta, Canada (CitationKotak et al. 2000). The Missouri relations are local, and may be used to identify conditions under which elevated MC concentrations most frequently occur.
This study demonstrates that MC is common in Missouri reservoirs; most values are low, but concentrations of concern were detected. Though timing of peak concentrations were unique to individual reservoirs, statewide occurrence was relatively consistent among months and years, indicating that including MC as part of routine water-quality monitoring programs may be sufficient to identify reservoirs with frequent MC occurrence; these reservoirs mostly are in the midrange of the trophic continuum. These data enhance understanding of when and where elevated MC concentrations may occur and can lead to more effective identification of human health risks and lake and reservoir management strategies.
Acknowledgments
We thank Steven Heiskary, Minnesota Pollution Control Agency, and Keith Loftin, U.S. Geological Survey, for reviews that provided valuable input and improved the quality of this paper, and D. Obrecht, C. Radcliffe and K. Veum for field and laboratory assistance. Funding and support for this project was provided by Missouri Department of Natural Resources, Food and Agriculture Policy Research Institute, Missouri Agricultural Experiment Station, and the U.S. Geological Survey. Any use of trade, product, or firm names is for descriptive purposes only and does not imply endorsement by the U.S. Government.
Notes
a ,
b ,
c Indicate significant differences in mean values for each variable. variables without significant differences (p > 0.05) are not shown.
References
- Blackburn , T. M. , Lawton , J. H. and Perry , J. N. 1992 . A method of estimating the slope of upper bounds of plots of body size and abundance in natural animal assemblages . Oikos , 65 : 107 – 112 .
- Boyer , G. L. 2007 . The occurrence of cyanobacterial toxins in New York lakes: Lessons from the MERHAB-Lower Great Lakes program . Lake Reserv. Manage. , 23 : 153 – 160 .
- Burns , J. 2008 . “ Toxic cyanobacteria in Florida freshwaters ” . In Cyanobacterial harmful algal blooms. Advances in experimental medicine and biology , Edited by: Hudnell , H. K. 127 – 137 . Springer .
- Chorus , I. , ed. 2001 . Cyanotoxins: occurrence, causes, consequences , 357 Berlin : Springer-Verlag .
- Chorus , I. and Bartram , J. , eds. 1999 . Toxic Cyanobacteria in Water , 416 WHO . E & FN Spon
- Dodds , W. K. 1996 . Assessment of blue-green algal toxins in Kansas , 36 Kansas Water Resources Research Institute . Contribution Number: G2020–02. Lawrence, KS
- Forsberg , C. and Ryding , S.-O. 1980 . Eutrophication parameters and trophic state indices in 30 Swedish waste-receiving lakes . Arch. Hydrobiol. , 89 : 189 – 207 .
- Graham , J. L. 2006 . Harmful algal blooms , 2006 – 3147 . U. S. Geological Survey Fact Sheet .
- Graham , J. L. and Jones , J. R. 2007 . Microcystin distribution in physical size class separations of natural plankton communities . Lake Reserv. Manage. , 23 : 161 – 168 .
- Graham , J. L. , Jones , J. R. , Jones , S. B. and Clevenger , T. E. 2006a . Spatial and temporal dynamics of microcystin in a Missouri reservoir . Lake and Reserv. Manage. , 22 : 59 – 68 .
- Graham , J. L. , Jones , J. R. and Jones , S. B. 2006b . Microcystin in Midwestern lakes . LakeLine. , 26 : 32 – 35 .
- Graham , J. L. , Jones , J. R. , Jones , S. B. , Downing , J. A. and Clevenger , T. E. 2004 . Environmental factors influencing microcystin distribution and concentration in the Midwestern United States . Water Res. , 38 : 4395 – 4404 .
- Graham , J. L. , Loftin , K. A. , Meyer , M. T. and Ziegler , A. C. 2008 . Cyanobacteria in lakes and reservoirs—Toxin and taste-and-odor sampling guidelines (ver. 1.0) , U. S. Geological Survey Techniques of Water-Resources Investigations . http://pubs.water.usgs.gov/twri9A/ Book 9, Chapter A7, Section 7.5; available online only from
- Hedman , C. J. , Krick , W. R. , Karner , D. A. , Perkins , E. A. Harrahy and Sonzogni , W. C. 2008 . New measurements of cyanobacterial toxins in natural waters using high performance liquid chromatography coupled to tandem mass spectrometry . J. Environ. Quality , 37 : 1817 – 1824 .
- Huisman , J. , Matthijs , H. C. P. and Visser , P. M. , eds. 2005 . Harmful cyanobacteria , 241 Springer .
- Jones , J. R. , Knowlton , M. F. , Obrecht , D. V. and Cook , E. A. 2004 . Importance of landscape variables and morphology on nutrients in Missouri reservoirs . Can. J. Fish. Aquat. Sci. , 61 : 1503 – 1512 .
- Jones , J. R. , Obrecht , D. V. , Perkins , B. D. , Knowlton , M. F. , Thorpe , A. P. , Watanabe , S. and Bacon , R. R. 2008a . Nutrients, seston, and transparency of Missouri reservoirs and oxbow lakes: an analysis of regional limnology . Lake Reserv. Manage. , 24 : 155 – 179 .
- Jones , J. R. , Knowlton , M. F. and Obrecht , D. V. 2008b . Role of land cover and hydrology in determining nutrients in mid-continent reservoirs: implications for nutrient criteria and management . Lake Reserv. Manage. , 24 : 1 – 9 .
- Knowlton , M. F. and Jones , J. R. 1995 . Temporal and spatial dynamics of suspended sediment, nutrients, and algal biomass in Mark Twain Lake, Missouri . Arch. Hydrobiol. , 135 : 145 – 178 .
- Kotak , B. G. , Lam , A. K.-Y. and Prepas , E. E. 1995 . Variability of the hepatotoxin microcystin-LR in hypereutrophic drinking waters . J. Phycol. , 31 : 248 – 263 .
- Kotak , B. G. , Lam , A. K.-Y. , Prepas , E. E. and Hrudey , S. E. 2000 . Role of physical and chemical variables in regulating microcystin-LR concentration in phytoplankton of eutrophic lakes . Can. J. Fish. Aquat. Sci. , 57 : 1584 – 1593 .
- Lindholm , T. 1991 . Recurrent depth maxima of the hepatotoxic cyanobacterium Oscillatoria agardhii . Can. J. Fish. Aquat. Sci. , 48 : 1629 – 1634 .
- Lindon , M. J. and Heiskary , S. A. 2008 . Blue-green algal toxin (microcystin) levels in Minnesota lakes 12p Minnesota Pollution Control Agency Environmental Bulletin No. 11
- McDermott , C. M. , Feola , R. and Plude , J. 1995 . Detection of cyanobacterial toxins (microcystins) in waters of Northeastern Wisconsin by a new immunoassay technique . Toxicon , 33 : 1433 – 1442 .
- Reynolds , C. S. 1998 . What factors influence the species composition of phytoplankton in lakes of different trophic status? . Hydrobiologia , 369/370 : 11 – 26 .
- Scharf , F. S. , Juanes , F. and Sutherland , M. 1998 . Inferring ecological relationships from the edges of scatter diagrams: comparison of regression techniques . Ecology , 79 : 448 – 460 .
- Sokal , R. R. and Rohlf , F. J. 1995 . Biometry: the principles and practice of statistics in biological research, , 3rd ed. , 887 New York : W. H. Freeman and Company .
- Vézie , C. , Brient , L. , Sivonen , K. , Bertru , G. , Lefeuvre , J.-C. and Salkinoja-Salonen , M. 1998 . Variation of microcystin content of cyanobacterial blooms and isolated strains in Lake Grand-Lieu (France) . Microb. Ecol. , 35 : 126 – 135 .
- Welker , M. , Von Döhren , H. , Täuscher , H. , Steinberg , C. E. W. and Erhard , M. 2003 . Toxic Microcystis in shallow lake Müggelsee (Germany) – dynamics, distribution, diversity . Arch. Hydrobiol. , 157 : 227 – 248 .
- White , E. , Law , K. , Payne , G. and Pickmere , S. 1985 . Nutrient demand and availability among planktonic communities – an attempt to assess nutrient limitation to plant growth in 12 central volcanic plateau lakes . N. Z. J. Mar. Freshwat. Res. , 19 : 49 – 62 .
- Williams , C. D. , Aubel , M. T. , Chapman , A. D. and D'aiuto , P. E. 2007 . Identification of cyanobacterial toxins in Florida's freshwater systems . Lake Reserv. Manage. , 23 : 144 – 152 .
- Yoo , R. S. , Carmichael , W. W. , Hoehn , R. C. and Hrudey , S. E. 1995 . Cyanobacterial (blue-green algal) toxins: a resource guide , 229 AWWA Foundation and the American Water Works Association .
- Zimba , P. V. and Grimm , C. C. 2003 . A synoptic survey of musty/muddy odor metabolites and microcystin toxin occurrence and concentration in southeastern USA channel catfish (Ictalurus punctatus Ralfinesque) production ponds . Aquaculture , 218 : 81 – 87 .