Abstract
In the late 1960s and early 1970s, summer and fall blooms of cyanobacteria began to occur in Newman Lake, Washington (zavg: 5.6 m, zmax: 9.0 m); through the next decade, these blooms intensified and became an annual occurrence. Community efforts in the mid-1980s sparked a Restoration Feasibility assessment of the lake and watershed that indicated total annual gross phosphorus loading of at least 3000 kg, with a major portion (∼83%) attributable to internal recycling associated with summer hypolimnetic oxygen depletion. Implementation activities began September 1989, with watershed controls and a whole-lake alum treatment, followed in 1992 by installation of a Speece cone for hypolimnetic oxygenation and in 1997 by addition of a dual-port, microfloc alum injection system. Average summer volume-weighted total phosphorus has declined from prerestoration levels exceeding 50 μg-P/L to an average of 21 μg-P/L over the past 7 years (15–28 μg-P/L). Most notably, peak annual biovolumes of cyanobacteria and their representation within the phytoplankton community have decreased substantially, with increased prevalence of diatoms, green and golden-brown algae. A clearwater phase following spring blooms of diatom and/or golden-brown algae has occurred during those last 7 years, although this phenomenon was observed in the prior three decades. Overall, the restoration has been a success, and lake response to nutrient reduction at Newman Lake is consistent with worldwide observations that emphasize the need for long-term perspectives and commitment in lake restoration and management. Continuation of internal load controls and increased emphasis on external nutrient abatement will be necessary to continue the positive water quality trends despite future development increases and land use changes.
Since the identification of phosphorus (P) and nitrogen (N) as principal drivers in eutrophication (CitationVallentyne 1957, CitationVollenweider 1971) and recognition of the potential of N/P ratios in regulating phytoplankton community composition (CitationRedfield et al. 1963, CitationSchindler 1977), a majority of efforts to control phytoplankton productivity and standing crop have focused on reducing external and internal phosphorus loading (CitationWetzel 1975, Citation2001, CitationHorne and Goldman 1994). Biomanipulation has also received lesser but significant attention (CitationShapiro et al. 1975, CitationCarpenter et al. 1985, CitationPerrow et al. 1997, CitationMehner et al. 2002). A substantial literature on lake restoration and management has emerged over the past three decades, with availability of a standard text (CitationCooke et al. 2005). Lake restoration is still a relatively new science, however, with much to be learned about long-term physical, chemical and biological responses to nitrogen and phosphorus management (CitationJeppesen et al. 2005, CitationNürnberg 2007, CitationSchindler et al. 2008). Despite many successes, the need for scientific reporting of case studies is still vitally important to advance lake water quality restoration and management (CitationOsgood 2007). Our series of papers describes the ecology and lake restoration and management activities in Newman Lake, Washington, that began in 1989. Part 1 describes the Newman Lake watershed, relevant climatic and environmental events, restoration efforts and overall response of lake chemistry and biota. Subsequent papers describe implementation and responses to specific and relatively unusual treatments employed at Newman Lake, including microfloc alum injection (Part II, this issue) and hypolimnetic oxygenation (Part III, in preparation) with downflow contact bubble technology (Speece cone).
Newman Lake is oligomictic, so that in most years it exhibits a stable summer thermal stratification that begins about mid- to late May and persists through mid-August or early September. The Osgood Index of Mixing (OI = 2.5 m/km) indicates increased potential for mixing of hypolimnetic water into the epilimnion, and consequently for greater influence of internal loading on summer epilimnetic phosphorus and on primary productivity (CitationOsgood 1988). During periods of unseasonably cold temperatures, turnover events and/or entrainment have occasionally been observed in June or July. Such events, along with vertical entrainment and episodes of wind-driven upwelling along the lake margins (CitationBlanton 1973), tend to collaborate implications of the low OI. Therefore, management of Newman and similar lakes must incorporate concepts of both shallow (CitationWelsh and Cooke 1995, CitationWelsh and Jacoby 2001) and deeper lakes (e.g., Effler 1996).
Study area, proceedures, environmental conditions and restoration activities
Watershed, geologic setting and land use
Newman Lake () is located in northeastern Washington, about 25 km east of the city of Spokane. The surface area is about 515 ha (1270 ac). Lake level is regulated by an outlet structure, and volume varies between about 26.5 to 27.1 × 106 m3 (21,500 to 20,000 ac-ft). The lake basin itself was formed by glacial action, which has also exerted major geomorphic control in the watershed.
Figure 1 Bathymetric map of Newman Lake, Washington showing major stream and sample site locations. Depth contours are in feet (after CitationWolcott 1964).
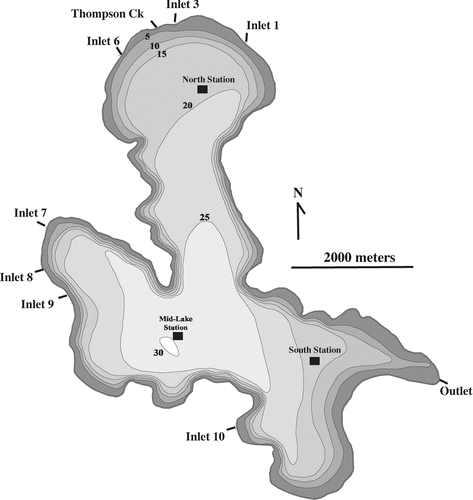
The watershed (area of about 7800 ha [19,000 ac], including the lake) bedrock types are various igneous gneisses. Soils on slopes are principally sands and loams of the Moscow and Spokane series that exhibit moderate to good drainage and moderate to severe erosion hazard. Alluvial deposits of silts, sands and gravels occur in the stream valleys, while poorly drained silt and peat alluvium cover lowland areas immediately surrounding the lake (CitationCopp 1976). Glacial moraines are evident on the southeastern and western lake margins. A peninsular area between two northern arms of the lake is composed of well-sorted sand overlying nonglaciated bedrock outcropping of gneiss; this may be an interlobate moraine or esker, formed by deposition from streams draining converging glaciers.
The only perennial inlet is Thompson Creek, which drains at least 40% of the total watershed and is fed by four perennial tributaries (CitationCopp 1976). Numerous intermittent streams drain the remainder of the watershed (). Almost 86% of the watershed is managed for private, industrial and nonindustrial timber production (CitationBarrentine 2007). Agricultural land use accounts for about 5% of watershed area. About 570 residences occupy about 122 ha (300 ac), less than 2% of the watershed. Residences on septic systems (40% year-round) occupy over 60% of the shoreline (CitationMoore 2007).
Sampling and procedures
Since 1974, data have been collected in conjunction with multiple studies using various study designs; however, field sampling and laboratory analytical methodologies have been consistent, allowing data comparability. Principal study and restoration efforts at Newman Lake have included:
• | Initial hydrologic and water quality studies, 1974 to 1975 | ||||
• | Phase I diagnostic/feasibility study, summer 1985 through fall 1986 | ||||
• | Phase II implementation study, spring 1989 through winter 1993 | ||||
• | Whole lake alum treatment, September 1989 | ||||
• | Hypolimnetic oxygenator installation, June 1992 | ||||
• | Miscellaneous and intermittent monitoring, 1993 to 1997 | ||||
• | Microfloc alum injection system installation, April 1997 | ||||
• | Lake and inlet monitoring, 1997 to present |
Three sample stations were established in the original 1974–1975 studies and have been utilized since. These stations, designated as North, South and Mid-lake, were selected to represent the northern and southern lobes and main body of lake, respectively. Profiles of temperature, pH, dissolved oxygen (DO) and specific conductivity were taken at 1-m intervals at each lake station using single or multi-parameter instrumentation (either Yellow Springs Instruments single-parameter meters, or Hydrolab Minisonde 4a Water Quality Multiprobe with Surveyor Model 6). National Institute for Standards and Technology (NIST)-traceable reagents and standards were used for all calibrations and for analytical quality assurance. Water transparency was estimated with a standard Secchi disk, and light intensities in profiles were measured occasionally with a Kahl Scientific Photometer. A surface pump collected water samples at half the maximum depth and at 1 m less than the maximum depth at each station, designated as “Top, Middle (Mid) or Bottom (Bot).” Inlet and outlet streams were sampled and gauged on each sampling date in 1985–1986, 1990 and 2004–2006 to quantify nutrients and water entering the lake. Larger streams such as Thompson Creek were gauged using the velocity/cross-section method, as per standard U.S. Geological Survey (USGS) gauging procedures (CitationWahl et al. 1995). Velocity readings were obtained with a Swoffer Velocity Meter (Swoffer Instruments, Seattle, WA). The products of about 20 cross-sectional areas (width × depth) multiplied by corresponding velocities for each section were summed to yield total stream discharge. Staff gauges are located at the outlet and at a regular sampling station on Thompson Creek; rating curves were developed to estimate flows at these locations. Flows in smaller streams were determined by direct collection of the entire inlet volume in a bucket while measuring the time required for collection. Inlet and outlet water samples were taken by grab sampling, and in situ stream measurements were made with same methods as described for lake sampling.
Phytoplankton samples were collected at the same depths as chemical samples and were preserved with gluteraldehyde to maintain algae morphology. Immediately after collection, 2 ml of 3% gluteraldehyde, neutralized to pH 7 with NaOH, were added to each 500-ml sample bottle (CitationAPHA 1998, CitationWetzel and Likens 2000).
Nutrient analyses were completed in the laboratory with the semi-automated, spectrophotometric molybdate method on either a Technicon Auto-Analyzer (pre-1989) or an Alpkem (now: OI Analytical) Rapid Flow Analyzer (RFA) using Win-flow 3000 data capture software. All analytical protocols followed Standard Methods (CitationAPHA 1998 and earlier) as modified for the Technicon or Alpkem instrumentation (Alpken 1997). All reagents and calibrants were NIST-traceable. Total phosphorus (TP) samples (unfiltered) were digested prior to analysis with persulfate (detection limit ∼0.002 mg/L; APHA 1998 and earlier).
Phytoplankton and zooplankton samples were identified and counted in 1-ml Sedgewick-Rafter counting slides using Leica and Nikon stereoscopic microscopes with built-in micrometers, following methods described in CitationEdmonson and Winberg (1971) and CitationWetzel and Likens (2000). Phytoplankton were identified to the lowest possible taxonomic unit using keys of CitationDillard (1999), CitationCanter-Lund and Lund (1995) and CitationPrescott (1978). The numbers of each type of individual were counted, and dimensions of key species were measured with a lens micrometer. Dimensions for at least 30 individuals were recorded for each sample and were used to calculate biovolumes from published relationships (CitationFunk and Moore 1988). Multiple subsamples were taken to confirm that each sample was representative.
Hydrologic and phosphorus budgets
Lake volumes were calculated from hypsographic plots of elevation versus volume (CitationOrsborn and Sood 1975, CitationCopp 1976). Hydrographs were constructed from stream monitoring data for Thompson Creek and for the seasonal inlet streams for 1985–1986 (water year), 1990, 2004, 2005 and 2006 (calendar years, except 2005 and 2006, which were 10-mo budgets). Outlet flows were calculated from rating curves for the control structure (CitationCopp 1976, Spokane County Engineers Office unpublished rating curve). Precipitation was calculated from data collected by volunteers around the lake (Newman Lake Volunteer Monitoring Project, 2004–2006, unpubl. data). For periods when data were not available, precipitation was estimated from a regression of data from Spokane Airport maintained by the National Weather Service with local measurements (CitationOrsborn and Sood 1975, CitationCopp 1976). Evaporation was estimated from a regression of data from Spokane Airport with calculated evaporation rates (CitationOrsborn and Sood 1975). Groundwater flows were calculated by differences in monthly mass water balances (CitationCopp 1976). Seasonal groundwater flow direction, into or out of the lake, were verified by flow net calculations from a network of piezometers installed and monitored from 1989 through about 1993 (CitationBurkett 1991, CitationFunk et al. 1998).
Phosphorus budgets were calculated using a mass balance approach (CitationChapra 1997, CitationCooke et al. 2005). Inlet and outlet phosphorus loading was calculated as the product of weekly integrals of flow-times measured phosphorus concentrations summed over monthly intervals (CitationScheider et al. 1979). Groundwater was calculated as the product of piezometer phosphorus concentrations and monthly flow estimates. For months in which piezometer data were not available, volume-weighted average lake TP for the corresponding time was utilized. Volume weightings were calculated using all data from all stations and from hypsographic profiles. Internal cycling was estimated as the residual term in the phosphorus mass balance on a monthly basis. Changes in Thompson Creek TP over all monitored years were analyzed by ANOVA, and Tukey's post-hoc method of multiple comparisons was used to determine significant differences between yearly concentrations.
Restoration overview
Diagnostic studies
The Newman Lake community originally set the overarching goal for restoration activities as improved water quality, measured by reduced total algae and cyanobacteria standing crop. The principal means for obtaining these goals was a bottom-up control strategy through reducing available lake nutrient concentrations. Standard Selenastrun capricornutum bioassays performed in spring and summer of 1973 and again in 1985 showed greater response to N than P, although the later also stimulated growth (CitationFunk et al. 1976, Citation1988). Response curves to combined N and P spikes were parallel and quantitatively indistinguishable from response curves to N alone. Prerestoration monitoring showed that cyanobacteria tended to dominate summer and fall phytoplankton. These data, along with low N/P ratios, suggested that reduced loading of both nutrients would be necessary to lower primary productivity and algae standing crop in Newman Lake. Although the bioassays indicated N limitation, returning the lake to P-limited conditions by emphasizing P control to increase N/P was seen as the key strategy (CitationWetzel 1975, CitationSchindler 1977) because nitrogen-fixing forms, such as Anabaena flos-aquae and Aphanizomenon flos-aquae, dominated the blue-green community.
For the 1985–1986 water-year, total gross phosphorus load was estimated to exceed 3000 kg/yr (lake area load rate of about 610 mg/m2/yr1). Gross load designates total inputs from all sources, including internal, while net loading refers to the remainder of inputs minus losses, such as losses to sediments, groundwater or surface outflows (see CitationNürnberg 1998, CitationNürnberg and LaZerte 2001 for more discussion). Total basin inflows, including precipitation and groundwater, were estimated about 20% of annual gross phosphorus load; therefore, dual strategies were suggested to abate both watershed and internal nutrient sources (CitationFunk and Moore 1988). To address external sources, a Watershed Committee was formed to include as many community stakeholders as possible, and every effort was made to obtain representation for all major land uses and users. Administrative leadership for the restoration efforts was overseen by the Newman Lake Flood Control Zone District (NLFCZD), working through the Spokane County Engineers Office (SCE). This effort was one of the first examples of true public involvement in watershed planning in Washington.
The Watershed Committee identified a wide range of potential nutrient sources including domestic septic disposal and lawn fertilization, cattle grazing in stream corridors and lake margins, timber harvesting, erosion along roadways, new home and driveway construction, and erosion initiated by off-road vehicle (ORV) use, an activity widespread in the Thompson Creek drainage, without authorization from landowners. The committee recommended numerous best management practices (BMP) to abate nonpoint sources and stressed educational efforts to elicit voluntary implementation and compliance with relevant ordinances and statutes.
Watershed controls
Many committee recommendations were implemented. For example, road and stream crossings, riparian buffers and site-specific forestry BMP for erosion control were made on the Thompson Creek forestlands. The committee noted that extreme gully erosion, initiated by ORV damage to soils, was common in the watershed; therefore, controlled access to the Thompson Creek road was implemented in the early 1990s, and ORV use has essentially been excluded from the upper watershed (Dennis Parent, Lands Manager, Inland Empire Paper Company, Spokane, WA, 2008, pers. comm.).
In the 1980s and 1990s, hundreds of cattle were typically maintained in pastures or feed lots within the riparian corridor of lower Thompson Creek and along the northern lake shoreline. The Watershed Committee recommendations limiting cattle access to the stream and lake were largely implemented. Overall, ranching operations have been mostly phased out. Some farming, restricted to hay and oats cultivation, still occurs along the southern end, but surface drainage for these fields is downstream of the lake and probably does not affect the lake (CitationBurkett 1991).
Average concentrations of TP in Thompson Creek have been on a decreasing trend over the past two decades (), with statistically significant lower TP from 2003 to 2006 compared to concentrations observed in the 1980s (CitationAnderson 2007). Loads from other inlets have changed less over the same period, probably because few changes in land use practices that generate phosphorus and sediment loads have occurred (CitationAnderson 2007).
Internal load controls
Available research at the time of the 1985–1986 study indicated that external load control alone was unlikely to reduce P saturation and primary productivity (CitationJacoby et al. 1982); therefore, internal load controls were strongly recommended (CitationFunk et al. 1988). Selected strategies included whole-lake alum treatment coupled with hypolimnetic aeration. Originally, the alum treatment was to be accompanied by hypolimnetic aeration to control increased oxygen demand from materials precipitated with alum floc. A full- or partial-lift Bernhardt aeration system (CitationBernhardt 1974), available as a turnkey, off-the-shelf unit, was specified. However, by the beginning of the Phase II Implementation Project, such units became unavailable, and it was decided to proceed with whole-lake alum treatment alone in September 1989. Alum was applied to the lake surface for 6 days during September 1989 using Loran and Global Positioning System (GPS), to achieve a volume-integrated target aluminum dose of 2.8 mg/L (Tom Eberhardt, Sweetwater Technology, Aiken, MN, 1989, pers. comm.).
Oxygenation system
Analysis of Newman Lake oxygen data and sediment incubations indicated that approximately 1360 kg (3000 lbs) per day of oxygen would be required to maintain oxic conditions at the sediment/water interface (CitationLorenzen and Fast 1977, CitationAshley 1985, CitationAshley et al. 1987, CitationMoore et al. 1996). A downflow contact bubble oxygenation (DCBO) system, also known as a Speece cone design (CitationSpeece 1971), was installed June 1992. In the Newman Lake system, oxygen is supplied by dual molecular sieve units (AirSep Corporation, Buffalo, NY), a dryer module and dual 50 hp compressors, all housed on shore. Oxygen is pumped by the compressors to the in-lake Speece cone that uses a 45 kw (60 hp) submerged pump to drive water through the system. The cone discharges oxygenated water through a 30.5-m long, 0.6-m diameter distribution manifold with fifty 0.8-cm–dia ports. Details of the Newman Lake Speece cone system, operation and performance are presented in Part 3 of this series.
The oxygenation system was operated each year from initial stratification in spring, typically in April or May, through fall turnover in mid-August or early September. Operation during the first year of installation, 1992, was intermittent. From 1993 through 1996, oxygen in early spring was delivered at approximately 50% capacity, using only one of the onshore compressors. Both compressors were brought online in the second half of June because the DO in the hypolimnion was observed to decrease. In 1997 this operational protocol was altered to operate at 100% from initiation of summer stratification, usually in May, through fall turnover. This protocol has been in effect since May 1997, except in July 2000 when the in-lake water pump failed, shutting down both oxygenation and alum systems for most of that summer.
Microfloc alum
In 1997, a microfloc alum injection was installed in a piggyback-configuration on the oxygenation system. Conventional whole lake alum treatments form large aluminum hydroxide flocculants that settle rapidly and very effectively remove particulates from the water column. However, the main object of such treatments is to inactivate phosphorus by chemically bonding within the sediments (CitationCooke and Kennedy 1981, CitationCooke et al. 2005). In contrast, microfloc alum is designed to achieve distribution of ultra-small particles of aluminum hydroxide, substantially increasing residence times and allowing potential removal of soluble as well as particulate phosphorus. The Newman alum system consists of a 23 m3 (6000 gal) alum storage tank, a timer-controlled pump and dual feed lines to the lake. Microfloc is generated by discharging alum syrup into two venturi-shaped outlet cones mounted over discharge ports of the oxygenation system manifold. In this way, the pump from the hypolimnetic oxygenation system is used both to create microfloc and to distribute alum. Details of the microfloc alum system, operation and performance are described in Part 2 of this series.
Environmental anomalies
Two particularly noteworthy environmental events occurred in winter 1996 and spring 1997. On 19 November 1996, much of the northwestern United States was subjected to a severe ice storm that deposited heavy loads (> 1 cm) of rime ice (National Weather Service) on exposed surfaces. Damage, especially to vegetation, was extreme throughout the Newman Lake watershed. Snow water equivalent accumulation in the Newman watershed during the following winter was the highest on record for February and March (Natural Resource Conservation Service, Quartz Peak Snotel System Data, 1996). At upper elevations, winter 1996–1997 snowpack was 160% over normal levels, and most significant, accumulation was very high (∼ 150% over normal) at lower elevations. In early April 1997, warm temperatures preceded heavy rainfall on the ripe snow pack, causing flooding in Thompson Creek and in the lake outlet. Peak flows in Thompson Creek were estimated to exceed a 100-yr return interval (M. Barrentine, Spokane County Engineers Office, 2008, pers. comm.). Heavy runoff volumes, on top of ice storm-damaged vegetation and soils, yielded extremely turbid and cold runoff. This April flood event was concurrent with ice-off on the lake itself. A stable thermal stratification developed immediately as the cold (∼2 C), highly turbid inlet waters flowed along the lake bottom. Turbidity was so great that the hypolimnion was totally devoid of light; Scuba observations revealed that an underwater torch held to the faceplate could not be seen (B. Moore, pers. observ.). High lake turbidity reduced light penetration and heating so effectively that the thermocline never dropped below 3 m for all of summer 1997, while in most other years the thermocline depth steadily deepens to ∼5–6 m by mid- to late July.
Results and discussion
Phosphorus
Average growing season (Apr–Oct) volume-weighted TP concentrations in Newman Lake from 1985 through 2006 () included three prerestoration years of TP data (1989 TP average includes data only to September, prior to alum treatment). Annual average growing season TP ranged from 43 to 77 μg-P/L (long term average = 55 μg-P/L), with a suggestion of an increasing trend prior to restoration (). A typical problem in lake restoration projects is limited premonitoring data (often only a single year of intensive monitoring), which produces datasets that allow reasonable quantification of annual post-restoration but not prerestoration variability.
Figure 3 Average annual volume-weighted total phosphorus in Newman Lake. Y-axis values are in μg-P/L. 1989 = whole lake alum treatment; 1992 = hypolimnetic oxygenation installed; 1997 = 100-yr flood event; 1997 = microfloc alum installed; 2000 = oxygenation and alum systems out of service (see text for details).
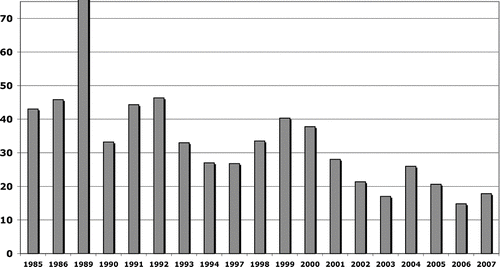
In 1998, the year following whole-lake alum treatment, TP declined to 33 μg-P/L but then increased over the next two years to prerestoration levels, indicating a limited effectiveness of the whole-lake alum treatment. As noted, the original intent was to conduct alum treatment simultaneously with aeration. Failure to do so may very likely account for the limited treatment longevity because increased oxygen demand associated with alum-precipitated materials could have heightened internal P loading, overwhelming P retention capabilities of the alum floc.
Declines in average annual TP are evident in the 2 years for which data are available following the 1992 installation of hypolimnetic oxygenation; however, partial system operation only introduced a fraction of the designed oxygen to the lake. Summer hypolimnetic DO concentrations, especially at the critical sediment/water interface, were < 2 mg/L for significant periods; therefore, full benefits of oxygenation for internal cycling reduction were likely not realized because of operational parameters (CitationMoore et al. 1996, CitationAshley 2000, Citation2002).
Microfloc alum was added to the oxygenation system in spring 1997. Installation actually occurred during the April 1997 100-yr flood event immediately following ice-off. Visual observations indicated extremely high levels of particulates that spring, with high hypolimnetic turbidity throughout summer 1997. Average annual TP increased in 1998 and 1999. High sediment load and accompanying sediment oxygen demand (SOD) likely overwhelmed the design capacity of oxygenation system and alum floc, possibly increasing internal TP load in those years. In early July 2000 a pump impellor failed, shutting both the alum and oxygenation systems down for most of the summer stratification period.
Since 2001, significant decreases in average annual TP have been observed (). This is the only period during which both alum and oxygenation systems have been operated within their design specifications and within the environmental ranges predicted from prerestoration studies. For the 7 years from 2001 to 2007, inclusive, annual volume-weighted total phosphorus ranged from 15 to 28 μg-P/L, averaging 21 μg-P/L.
The TP data were grouped into discrete periods associated with whole-lake alum treatment (1990–1992), flood sediment influences (1997–2000), oxygen/microfloc treatment (2001–2006), and the most recent years (2002–2007; ). Most recent yearly TP concentrations are significantly lower for all stations and depths compared to prerestoration levels (p < 0.05). Changes in TP concentrations have been greatest at the bottom strata, likely reflecting reduced internal cycling related to operation of the microfloc and oxygenation systems (). Hypolimnetic TP concentrations are less variable because the frequency of extremely high concentrations of the prerestoration period decreased. Hypolimnetic TP concentrations of all periods are significantly lower than prerestoration concentrations (p < 0.05). Results for both the epilimnion and metalimnion also indicate similar declining trends; recent year TP concentrations for both depths are statistically different from prerestoration values ( and ). Higher variability for the epilimnion and metalimnion likely reflect greater direct influence of external load, especially for the flood sediment influence period from 1997 to 2000.
Figure 4 Box plots of annual volume-weighted total phosphorus concentrations in Newman Lake, grouped by treatment intervals. Y-axis values are in μg-P/L. Squares = median values; shaded boxes = inner quartiles, including data +/- 25% of medians; whiskers = minimum/maximum lines. (a) Annual volume-weighted, whole-lake TP for all stations and all depths (n = 1420). (b) Annual volume-weighted epilimnion TP (n = 475). (c) Annual volume-weighted metalimnion TP (n = 472). (d) Annual volume-weighted hypolimnion TP (n = 473).
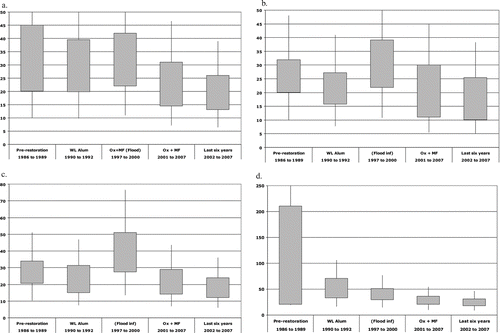
Data frequency distributions, based on 1420 individual TP measurements and grouped for increasing TP levels to indicate trophic state changes, provide information on the shift in Newman Lake TP concentrations (). Cumulative post-restoration data range frequencies were compared to prerestoration frequencies. Prerestoration histograms include all data prior to whole-lake alum treatment in September 1989; post-restoration includes all data since 1990. Frequencies within the 21–30 μg-P/L mesotrophic range are essentially unchanged at about 30%, but the frequency of concentrations > 20 μg-P/L have decreased substantially, from approximately < 25% to > 40% of the post-restoration observations.
Figure 5 Frequency histograms for volume-weighted TP in Newman Lake. Y-axis in percentages of total observations; X-axis in μg-TP/L. (A) Cumulative post-restoration data range frequencies (n = 1345) compared to pre-restoration frequencies (n = 176). (B) Histograms for data stratified into pre-oxygenation years (pre-1992, n = 286), years with partial oxygenation (1992–1996, n = 455), and years with full oxygenation (1997–1999 and 2001–2007, n = 780). (C) Histograms for data stratified into pre-oxygenation years (pre-1992, n = 286), and years operated with full oxygenation within the designed system environmental parameters (2001–2007, n = 780) (see text for details).
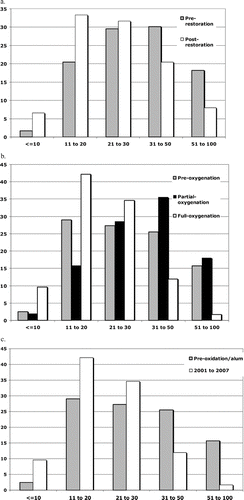
Data histograms are also grouped according to periods of oxygenator system operation compared to prerestoration ( and ). Partial operation years are from 1992 through 1996, inclusive. Full oxygenation histograms include 1997–1999 and 2001–2007, inclusive. The former encompass the flood sediment-influenced and excessive hypolimnetic volume years, while the 7-yr grouping, 2001–2007, are the years in which the system operated within design and operational parameters. A comparison of the 2001–2007 data shows that frequencies within the mesotrophic range are similar to prerestoration years, at 25–30%; however, for the more recent later period, > 50% of TP concentrations have been < 20 μg-P/L. Prior to restoration, about 50% of concentrations exceeded 31 μg-P/L; from 2001 to 2007, less than about 15% of TP observations in were in the higher ranges. Partial oxygenation has been less successful at shifting lake phosphorus distributions, seeming to indicate the importance of operating the systems as designed, but may also reflect the influence of high sediment loading and oxygen demand.
Monitoring overall has focused on the lake, but the 5-yr inlet and outlet monitoring data are sufficient to calculate hydrologic and TP budgets (typically weekly or biweekly flow measurements). These include the prerestoration 1985–1986 water year; 1990, immediately following whole lake alum; and 2004, 2005 and 2006, all 3 years with oxygenation and microfloc alum systems operable. For 2005 and 2006, data only permit 10-month TP budget calculations (). External loading has been highly variable, principally depending on the prevailing annual precipitation quantities, regimens and watershed practices. Even with declining TP concentrations in Thompson Creek, surface external loading ranged from a low of about 200 kg-P/yr to > 1000 kg-P/yr. Summer external surface load, computed as May through September, ranged from about 20 to > 200 kg-P/yr.
Table 1 Total phosphorus nutrient budget, loading rates and coefficients for Newman Lake, calculated for all years in which sufficient inlet and outlet monitoring data are available. Min Gross = minimum gross yearly total phosphorus load calculated as the sum of all positive loads. Net internal = arithmatic sum of all positive and negative loads. Ltot = total yearly phosphorus load divided by watershed and lake areas. Lext = total annual load from inlets divided by lake area. Robs and Rpred are observed and predicted phosphorus retention coefficients, respectively (see Nurnberg 1984 and text for details). qs = annual areal water load. Anoxic factor is calculated per Nurnberg (1987). Summer values are for the four month period, inclusive of June through September, and summer rates are extrapolated to an annual basis. For 2004 and 2005, rates are extrapolated to an annual basis.
Results for internal load calculations are of particular interest in evaluating efficacy of long-term control efforts. Interpretation of internal load estimates calculated by difference in a mass balance should be tempered by measurement uncertainties (CitationWinter 1981, Chapra 1989). Net internal load for the prerestoration 1985–1986-water year was calculated at about 700 kg-P/yr. Net loads for all post-restoration years available have been negative values, indicating an overall shift to net loss of lake phosphorus to the sediment.
Gross internal TP load cannot be measured directly. Direct measurements that would permit estimation of TP settling rates are also not available, and these rates often have high annual variability (CitationRyding and Forsberg 1980, CitationChapra 1997). The net internal load calculation is therefore the best available indication of prevailing TP movement relative to the sediments. However, a minimum gross internal TP load can be estimated as the sum of all positive monthly internal load values in a given year. For the prerestoration year 1985–1986, minimum gross internal load was about five times the external load, constituting over about three-quarters of the total annual TP budget. Minimum gross summer load for that water year exceeded external summer load by about an order of magnitude. While these are very rough estimates, total gross internal loads must, by definition, be higher, so the numbers do give an indication of relative source strengths. For post-restoration years, minimum gross loads have been less than about half of the prerestoration annual estimate; however, these do not give evidence if actual total gross internal TP loads have decreased.
Other indicators of internal loading rates are consistent with the above generalizations. For example, one widely used index of spatial and temporal distribution of anaerobic conditions at the sediment water interface is the anoxic factor (AF), developed by Nürnberg (1995a and b). The AF is calculated as the sediment area underlying anoxic water (defined as DO at < 1 mg/L, measured at 1 m above the sediment) times the duration of anoxia (in days) divided by the lake surface area. Internal phosphorus load may be successfully predicted as the product of AF multiplied times sediment phosphorus release rates determined by laboratory incubations (CitationNürnberg 1984, Citation1995a). Prerestoration AF of Newman Lake was between about 50 and 60 days/yr, which is a typical value for eutrophic, temperate zone dimictic lakes with hypolimnetic summer DO loss. Post-restoration AF for the mass balance years Newman Lake ranged from a high of 56 days/yr prior to oxygenation to < 10 days/yr for years since 2001.
CitationNürnberg (1984) also tested the hypothesis that differences in predicted and observed P retention rates in lakes with anoxic hypolimnia are related to internal loading, showing that models based on oxic lakes over-predicted observed retention calculated from mass balances in anoxic lakes. This analysis was applied to Newman Lake using the following relationships:
Taking this concept further, the deviation between predicted and observed retention can be used to predict internal load by the equation:
Phytoplankton
Phytoplankton identification, enumeration and biovolume estimates were conducted on Newman Lake from March through October samples for 1989–1995 and 2000–2006. July through October phytoplankton data were collected for 1986. Incidents of blue-green phytoplankton blooms that were reported as annual occurrences in the 1970s and 1980s were no longer evident after the first whole-lake alum treatment (). Peak blooms for 1989 were consistent with anecdotal descriptions of annual prerestoration blooms because blue-greens constituted > 90% of the peak biovolume for that year. The blue-green assemblage was almost exclusively Microcystis sp., with only a small percentage of Chroococcus sp. present on a few dates in 1989. Microcystis sp. continued to be the prevalent blue-green for the next three years, again with some Chroococcus sp., but with increasing numbers of Anabaena flos-aquae, especially in the spring assemblage. Interestingly, peak phytoplankton biovolumes, and the blue-green representation within the whole lake phytoplankton community, declined sharply with the initiation of restoration activities.
Figure 6 Maximum total and cyanobacteria biovolumes in Newman Lake from 1989 through 2007. Maxima are highest observed for each year and are not necessarily concurrent. Y-axis phytoplankton biovolumes are in cm3/m3.
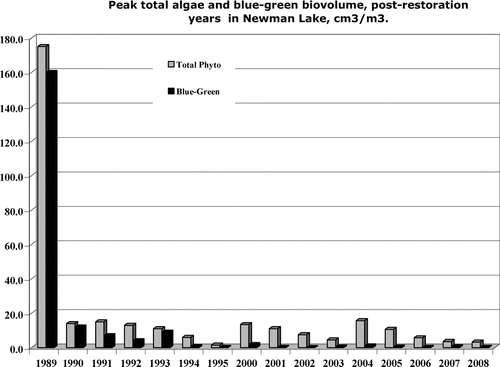
Average summer photic zone phytoplankton biomass change has been less dramatic compared to peak biovolumes, although algae seem to be less for the past six of seven years (). This is consistent with Secchi measurements, which have mostly remained in the range of 2–4 m over the past two decades. Representation of diatoms (Bacillariophyta) has remained between about 50 and 75% of total phytoplankton biomass (). Green algae have accounted for < 15% of total algae biovolume, with the exception of 2002 when they comprised about 22%. Brown algae (Chrysophyta), as a percentage of total plankton biovolume, have increased, as have dinoflagellates (Pyrrhophyta). In recent years, this later group has been most strongly represented by Peridinium sp. Most notably, representation of blue-greens in the phytoplankton community has declined. Since operation of the microfloc and oxygenation systems within design conditions beginning 2001, blue-greens have comprised < 8% of total annual average phytoplankton biomass. In recent years, higher numbers of the Chrysophysean Dinobryon sp. and the diatom Asterionella formosa have been found in spring, appearing immediately after ice-off and persisting through mid- to late May. Since about 2001, the spring algae peaks have been followed by a clearwater phase, with much lower phytoplankton biovolumes.
Table 2a Average growing season (April through October), photic zone algae biomass in Newman Lake for all available data years, 1986 to 2008. Values are in m3/lake. For 1986, data are available only from July through October.
Table 2b Average growing season (April through October), photic zone algae biomass in Newman Lake for all available data years, 1986 to 2008. Values expressed as percent of total phytoplankton biomass.
Overall, the pattern of phytoplankton in Newman Lake has been consistent with a shift from eutrophy to mesotrophy, in both numbers and community composition. The rapid decline in numbers and representation of the blue-greens was probably a response to lower phosphorus availability. Although total nitrogen has not been monitored in the lake, ammonia and nitrate concentrations have generally decreased less than have phosphorus concentrations. More details on inorganic nitrogen are presented in Part 2. As previously noted, algae bioassays from the 1970s and 1980s indicated nitrogen limitation, so the combined restoration efforts and watershed controls have likely disrupted their competitive advantage with respect to higher TP. The slower overall decline in phytoplankton is consistent with the slower reduction in phosphorus and nitrogen levels.
Conclusions
Restoration and management at Newman Lake have reduced ambient TP concentrations. Lower TP has been most evident since about 2001, when combined oxygenation and microfloc alum injection have been fully operational. There is strong evidence for reduced internal P cycling, with greatly reduced TP accumulation in the summer hypolimnion. Modeling and budgets of TP indicate that net direction of annual and summer TP load has shifted from sediment remobilization and recycling to net export to the sediments. Other quantitative indications of internal TP load, including Nürnberg AF, and differences in observed versus predicted P retention rates, support this conclusion. Therefore, restoration and management mechanisms targeted at reducing internal load appear to be having the desired effect.
Reduced TP availability is reflected in the standing crop of algae. Overall biological changes have been positive, especially reduced cyanobacteria biovolumes and a shift in the phytoplankton community from greater proportions of blue-greens to predominantly diatoms and chrysophysean algae. Step-wise reductions in algae correspond with ranges of reduced TP in the lake and correspond with the periods of operation of the oxygenator and microfloc alum systems.
Lake-wide noxious blue-green blooms have not been observed in Newman Lake since the late 1980s. Overall, blue-greens have become a relatively minor component of the phytoplankton community. In dramatic contrast to prerestoration years in which algal growth continued unabated throughout the summer with frequent blue-green dominated blooms, an early summer clear water phase following spring diatoms blooms has been apparent over most of the past 8 years.
Note that frequency distributions are very useful tools for communicating water quality expectations to the public. Often, disappointment in progress of restoration is experienced when perceptions are that water quality improvements will be rapid and linear. The immediate success of whole-lake alum treatments in temporarily clearing the water column can contribute to these perceptions; however, we believe it is important to communicate that the frequency and intensity of poor water quality (i.e., algae blooms) will eventually decrease as nutrient reduction efforts are implemented. This should assist the public in understanding that algae blooms and periods of lowered water clarity may not be immediately eliminated, but that they will not occur every year and should diminish in intensity and frequency as nutrient reduction activities take effect.
Overall, the restoration of Newman Lake has been consistent with observations of TP reduction efforts in many other lakes (CitationJeppesen et al. 2005, Citation2007, CitationSondergaard 2005). Although the phosphorus paradigm is receiving more scrutiny in recent literature (e.g., CitationLewis and Wurtsbaugh 2008, CitationSchindler 2008), the central concept of reducing phosphorus and total nutrient loading to reduce phytoplankton biomass remains sound. A salient point is that lake response to nutrient reduction is a relatively slow process, often requiring decades to achieve results. We agree with CitationCooke (2007) that successful lake managers must realize that public involvement is essential, not only to mobilize economic and social resources, but also to sustain water quality protection efforts in the face of increasing watershed development.
Acknowledgements
These papers are dedicated to Dr. William H. Funk, who initiated the Newman Lake restoration efforts, and to his substantial contributions over four decades of service to the cause of lakes, and to NALMS. The authors thank the many residents and property owners at Newman Lake, too numerous to name individually, who have supported restoration and protection of the lake which they love. Marrianne Barrentine and her staff at the Spokane County Engineers Office are thanked for their input and daily work on behalf Newman Lake. The authors thank Gertrud Nürnberg, Dick Osgood, Al Sosiak, and three anonymous reviewers for their valuable comments and critiques.
References
- Alpkem . 1997 . Enviroflow 2.1 Software advanced technology in flow analysis operation manual , College Station , TX : Alpkem Corporation, a Division of O. I. Analytical .
- [APHA] American Public Health Association . 1975 . Standard Methods for the Examination of Water and Wastewater, , 14th Ed , Washington , D. C. : American Public Health Association .
- [APHA] American Public Health Association . 1998 . Standard Methods for the Examination of Water and Wastewater, , 20th Ed , Washington , D. C. : American Public Health Association .
- Anderson , K. A. 2007 . An evaluation of phosphorus models used in the Newman Lake total maximum daily load process , MS thesis Pullman , WA : Washington State University .
- Ashley , K. I. 1985 . Hypolimnetic aeration: Practical design and application . Water Res. , 19 : 735 – 740 .
- Ashley , K. I. 2000 . Recent advances in hypolimnetic aeration design . Verh. Int. Limnol. , 27 : 2256 – 2260 .
- Ashley , K. I. 2002 . Comparative analysis of oxygen transfer in full-lift and downflow bubble contact aerators , PhD dissertation Vancouver , BC : University of British Columbia .
- Ashley , K. I. , Hay , S. and Schoeten , G. H. 1987 . Hypolimnetic aeration: Field test of the empirical sizing method . Water Res. , 21 : 221 – 227 .
- Barrentine , M. 2007 . Land use survey for the Newman Lake Flood Control Zone District , Spokane , WA : Spokane County Engineers Office .
- Bernhardt , H. Ten years experience of reservoir aeration . Seventh Conference on Water Pollution Research . Paris , France.
- Blanton , J. O. 1973 . Vertical entrainment into the epilimnia of stratified lakes . Limnol. Oceanogr. , 18 ( 5 ) : 687 – 704 .
- Burkett , S. E. 1991 . Groundwater and nutrient budget studies of Newman Lake, Washington , MS thesis Pullman , WA : Department of Geology, Washington State University .
- Canter-Lund , H. and Lund , J. W. G. 1995 . Freshwater algae: their microscopic world explored , Bristol , , England : Biopress Limited .
- Carpenter , S. R. , Kitchell , J. F. and Hodgson , J. R. 1985 . Cascading trophic interactions and lake productivity . Bioscience , 35 : 634 – 639 .
- Chapra , S. C. 1997 . Surface water-quality modeling , 844 Boston , MA : McGraw Hill .
- Cooke , G. D. 2007 . History of eutrophic lake rehabilitation in North America with arguments for including social sciences in the paradigm . Lake Reserv. Manage. , 23 ( 4 ) : 323 – 329 .
- Cooke , G. D. and Kennedy , R. H. 1981 . Precipitation and inactivation of phosphorus as a lake restoration technique EPA-600/3-81-012
- Cooke , G. D. , Welch , E. B. , Peterson , S. A. and Nichols , S. A. 2005 . Restoration and management of lakes and reservoirs, , 3rd ed , Boca Raton , FL : Taylor and Francis Group .
- Copp , H. D. 1976 . Investigation to determine extent and nature of nonpoint source enrichment and hydrology of several recreational lakes of eastern Washington. Part I. Hydrologic characteristics of the lake basins , Pullman , WA : Washington State University . State of Washington Water Research Center Report No. 26
- Doke , J. , Funk , W. H. and Moore , B. C. 1995 . Habitat availability and benthic invertebrate population changes following alum treatment and hypolimnetic oxygenation in Newman Lake, Washington . J. Freshw. Ecol. , 10 ( 2 ) : 87 – 102 .
- Dillard , G. E. 1999 . Common freshwater algae of the United States: an illustrated key to the genera (excluding the diatoms) , Berlin : J. Cramer .
- Edmonson , W. T. and Winberg , G. G. 1971 . A manual for the assessment of secondary productivity in fresh waters , Oxford , UK : International Biological Program. Blackwell Scientific Publications . IBP Handbook No. 17
- Effler , S. W. , ed. 1996 . “ Limnological and engineering analysis of a polluted urban lake ” . In Prelude to environmental management of Onondaga Lake, New York , New York : Springer-Verlag .
- Funk , W. H. , Gibbons , H. L. , Morency , D. A. , Bennett , P. J. , Marcley , R. and Bailey , G. C. 1976 . Investigation to determine extent and nature of non-point enrichment and hydrology of several recreational lakes of eastern Washington. Part II. Water quality study , Pullman , WA : Washington State University . State of Washington Water Research Center Report No. 26
- Funk , W. H. and Moore , B. C. 1988 . Newman Lake restoration feasibility study , Pullman , WA : Washington State University . State of Washington Water Research Center Report No. 69
- Funk , W. H. , Moore , B. C. , Burkett , S. K. and Juul , S. T. J. 1998 . Newman Lake Restoration Phase II , Pullman , WA : Washington State University . State of Washington Water Research Center Report No. 87
- Horne , A. J. and Goldman , C. R. 1994 . Limnology, , 2nd ed , New York : McGraw-Hill .
- Jacoby , J. M. , Welch , E. B. , Lynch , D. L. and Perkins , M. A. 1982 . Internal phosphorus loading in a shallow, eutrophic lake . Water Res. , 16 : 911 – 919 .
- Jeppesen , E. , Sondergaard , M. , Jensen , J. P. , Havens , K. E. , Anneville , O. , Carvalho , L. , Coveney , M. F. , Deneke , R. , Dokulil , M. T. Foy , B. 2005 . Lake responses to reduced nutrient loading- an analysis of contemporary long-term data from 35 case studies . Freshwater Biol. , 50 : 1747 – 1771 .
- Jeppesen , E. , Sondergaard , M. , Lauridsen , T. L. , Krovang , B. , Beklioglu , M. , Lammens , E. , Jensen , H. S. , Kohler , J. , Ventela , A-M. , Tarvainen , M. and Tatrai , I. 2007 . Danish and other European experiences in managing shallow lakes . Lake Reserv. Manage. , 23 ( 4 ) : 439 – 451 .
- Lewis , W. M. and Wurstbaugh , W. A. 2008 . Control of lacustrine phytoplankton by nutrients: Erosion of the phosphorus paradigm . Internat. Rev. Hydrobiol. , 93 : 446 – 465 .
- Lorenzen , M. W. and Fast , A. W. 1977 . A Guide to Aeration/Circulation Techniques for Lake Management , Ecological Research Series USEPA 600/3-77-004
- Mehner , T. , Benndorf , J. , Kasprzak , P. and Koschel , R. 2002 . Biomanipulation of lake ecosystems: successful applications and expanding complexity in the underlying science . Freshwater Biol. , 47 : 2453 – 2465 .
- Moore , B. C. , Chen , P. H. , Funk , W. H. and Yonge , D. 1996 . A model for predicting lake sediment oxygen demand following hypolimnetic aeration . Water Resour. Res. , 32 : 723 – 731 .
- Moore , D. L. 2007 . Survey of Newman Lake property owners , Pullman , WA : Social and Economic Science Research Center, Washington State University .
- Nürnberg , G. K. 1984 . The prediction of internal phosphorus load in lakes with anoxic hypolimnia . Limnol. Oceano. , 29 ( 1 ) : 111 – 124 .
- Nürnberg , G. K. 1995a . Quantifying anoxia in lakes . Limnol. Oceano. , 40 ( 6 ) : 1100 – 1111 .
- Nürnberg , G. K. 1995b . The anoxic factor, a quantitative measure of anoxia and fish species richness in central Ontario Lakes . Trans. Am. Fish. Soc. , 124 : 677 – 686 .
- Nürnberg , G. K. 1998 . Prediction of annual and seasonal phosphorus concentrations in stratified and polymictic lakes . Limnol. Oceano. , 43 : 1544 – 1552 .
- Nürnberg , G. K. 2007 . Low-Nitrate-Days (LND), a potential indicator of cyanobacteria blooms in a eutrophic hardwater reservoir . Water Qual. Res. J. Can. , 42 ( 4 ) : 269 – 283 .
- Nürnberg , G. K. and LaZerte , B. D. 2001 . “ Predicting lake water quality ” . In Managing lakes and reservoirs , Edited by: Holdren , C. , Jones , W. and Taggart , J. 139 – 163 . Madison , WI : North American Lake Management Society and the Terrene Institute in cooperation with Office of Water Assessment, Watershed Protection Division, United States Environmental Protection Agency .
- Orsborn , J. F. and Sood , M. 1975 . Hydrographic atlas and technical supplement-Spokane River Basin , 115 Pullman , WA : State of Washington Water Research Center. Washington State University .
- Osgood , R. A. 1988 . Lake mixes and internal phosphorus dynamics . Arch. Hydrobiol. , 113 : 629 – 638 .
- Osgood , R. 2007 . Planning for better lakes . Lake Line , 27 ( 1 ) : 11 – 12 .
- Perrow , M. R. , Meijer , M.-L. , Dawidowicz , P. and Coops , H. 1997 . Biomanipulation in shallow lakes: State of the art . Hydrobiologia , 342/343 : 355 – 365 .
- Prescott , G. W. 1978 . How to know the freshwater algae, , 3rd ed , Boston , MA : McGraw-Hill .
- Redfield , A. C. , Ketchum , B. H. and Richards , F. A. 1963 . “ The influence of organisms on the chemical composition of seawater ” . In The seas: ideas and observations on progress on the studies of seas. Vol. 2: The composition of seawater, comparative and description oceanography , Edited by: Hill , M. N. 26 – 77 . New York : Interscience .
- Ryding , S. O. and Forsberg , C. 1980 . “ Short-term load-response relationships in shallow, polluted lakes ” . In Hypereutrophic ecosystems. Developments in Hydrobiology Series, Vol. 2 , Edited by: Barica , J. and Mur , L. R. The Hague , , Netherlands : Dr. W. Junk Publishers .
- Scheider , W. A. , Moss , J. J. and Dillon , P. J. 1979 . “ Measurement and uses of hydraulic and nutrient budgets ” . In Lake Restoration 77 – 83 . USEPA 440/5-79-001
- Schindler , D. W. 1977 . The evolution of phosphorus limitation in lakes . Science , 195 : 260 – 263 .
- Schindler , D. W. , Hecky , R. E. , Findlay , D. L. , Stainton , M. P. , Parker , B. R. , Paterson , M. J. , Beaty , K. G. , Lyng , M. and Kasian , S. E. M. 2008 . Eutrophication of lakes cannot be controlled by reducing nitrogen input: Results of a 37-year whole-ecosystem experiment . Proc. Nat. Acad. Sci. , 105 : 11254 – 11258 .
- Shapiro , J. A. , LaMarra , V. and Lynch , M. 1975 . “ Biomanipulation: An ecosystem approach to lake restoration ” . In Symposium on water quality management and biological control , Edited by: Brezonik , P. L. and Fox , J. L. 85 – 96 . Gainesville , FL : University of Florida .
- Sondergaard , M. , Jensen , J. P. and Jeppeson , E. 2005 . Seasonal response of nutrients to reduced phosphorus loading in 12 Danish lakes . Freshwater Biol. , 50 : 1605 – 1615 .
- Speece , R. E. 1971 . Hypolimnetic aeration . J. Am. Water Works Assoc. , 63 : 6 – 9 .
- Vallentyne , J. R. 1957 . Principles of modern limnology . Am. Sci. , 45 : 218 – 244 .
- Vollenweider , R. A. 1971 . Scientific fundamentals of the eutrophication of lakes and flowing waters, with particular reference to nitrogen and phosphorus as factors in eutrophication , Paris , , France : Organization for Economic Cooperation and Development .
- Wahl , K. L. , Thomas , W. O. and Hirsch , R. M. 1995 . Stream Gauging Program of the U S Geological Survey , 1123 Denver , CO : United States Geological Survey Circular .
- Welsh , E. B. and Cooke , G. D. 1995 . Internal phosphorus loading in shallow lakes: Importance and control . Lake Reserv. Manage. , 11 : 273 – 281 .
- Welsh , E. B. and Jacoby , J. M. 2001 . On determining the principle source of phosphorus causing summer algal blooms in Western Washington lakes . Lake Reserv. Manage. , 17 : 55 – 65 .
- Wetzel , R. G. 1975 . Limnology, , 1st ed , Philadelphia , PA : W. B. Saunders .
- Wetzel , R. G. 2001 . Limnology: Lake and River Ecosystems, , 3rd ed , San Diego , CA : Academic Press .
- Wetzel , R. G. and Likens , G. E. 2000 . Limnological Methods, , 3rd ed , New York , NY : Springer-Verlag .
- Winter , T. C. 1981 . Uncertainties in estimating the water balance of lakes . Water Res. Bull. , 17 ( 1 ) : 82 – 115 .
- Wolcott , E. E. 1964 . Lakes of Washington. Vol. II Eastern Washington , Olympia , WA : Dept. of Conservation, Division of Water Resources . Water Supply Bulletin No. 14