Abstract
River systems with impoundments are expected to experience greater nutrient removal as a consequence of enhanced nitrogen (N) loss by denitrification, settling and burial of phosphorus (P), and longer residence times of water relative to a free-flowing river. We evaluated the magnitude of N and P removal across stream reaches and impoundments, including annual and seasonal trends, in two southeastern Michigan rivers that differ in their degree of impoundment and watershed land use patterns. All nutrient fraction concentrations remained statistically unchanged across stream reaches of 2–3 km in length, but dissolved oxygen and pH increased. Impoundment outlets exhibited increases in water temperature and decreases in conductivity, TP, TN, TDP, SRP, TDN, and NO3-N relative to inflowing water. A nutrient load mass balance showed that two connected impoundments removed 18 and 32% of the annual river loads of TN and TP, respectively. Removal rates were seasonably variable, with TP removal most pronounced during winter months when the impoundment received episodic high P loads. Conversely, TN removal was greatest during summer and autumn months when impoundments were stratified and water temperatures were elevated. Strong seasonal differences in N and P transport through the impoundments demonstrated the capacity of impoundments to dramatically alter the timing and stoichiometry of downstream nutrient export. These seasonal differences also highlight the need to consider the entire annual cycle when estimating surface water nutrient dynamics or designing management plans for nutrient abatement.
Nutrient processing in stream reaches, especially in headwater streams, has been shown to influence river nitrogen (N) and phosphorus (P) dynamics, but some uncertainty remains about the degree to which stream reach processes alter the net transport of N and P downstream. Recent research focused on N and P processing (as reviewed by CitationEnsign and Doyle 2006) has shown measurable retention and transformation in stream reaches. However, reach-scale studies in headwater agricultural streams argue for limited nutrient-processing capacity in small streams that receive very high nutrient inputs (CitationRoyer et al. 2004, CitationOpdyke et al. 2006). Nutrient removal mechanisms can become saturated at higher nutrient concentrations (CitationEarl et al. 2006, CitationO'Brien and Dodds 2008) such that the proportion of the nutrient load removed is reduced. Furthermore, modeling efforts that estimate the role of stream channels in nutrient retention at the watershed scale suggest a greater role in nutrient removal for small headwater systems relative to larger tributaries (CitationAlexander et al. 2000). The models also demonstrate the importance of the cumulative impact of nutrient removal along the entire river flow path (CitationSeitzinger et al. 2002) and predict that nutrient removal is reduced during high discharge months (CitationAlexander et al. 2009). However, a disconnect remains between reach-scale experimental data collection and its explicit incorporation into large-scale watershed modeling (CitationAlexander et al. 2009).
While there appears to be a consensus in management planning that impoundments reduce nutrient export downstream, relatively few studies have examined this question in detail at the watershed scale; thus, the quantitative role of impoundments in the downstream transport and processing of nutrients is unclear. We consider impoundments to include all lentic water bodies, including both lakes and reservoirs. Previous impoundment studies have observed a wide range in nutrient processing and consequent reductions in downstream transport (CitationEjsmontkarabin et al. 1993, CitationMaurer et al. 1995, CitationDixit et al. 2000, CitationHarris 2001, CitationDavid et al. 2006). The studies by CitationKelly (2001) and CitationReid and Hamilton (2007) highlighted this variability, finding that some impoundments acted as net sinks and others as net sources of N and P. In a global review of N removal in impoundments, CitationHarrison et al. (2009) reported a range of estimates spanning from 0% to over 100% across the 115 impoundments included in their analysis. Taking measured removal rates of individual impoundments and scaling the cumulative effect of impoundments to the watershed level is a current area of research (CitationBosch 2008), but further direct estimates of nutrient fluxes across impoundments are warranted.
The present study investigated nutrient processing and transport through measurement of net nutrient flux across impoundments and, for comparison, across stream reaches in two southeastern Michigan watersheds. Previous work in these two watersheds showed that impoundments could substantially reduce nutrient loads to downstream waters, as inferred by a watershed budget evaluation (CitationBosch and Allan 2008) and by a modeling study (CitationBosch 2008). We investigated the role of impoundments through a spatially-extensive sampling approach covering multiple impoundments and stream reaches across both watersheds to identify locations and rates of nutrient removal. A time-intensive sampling of one impoundment was used to assess annual and seasonal processing of individual fractions of N and P. This is the first study to our knowledge that has simultaneously examined N and P removal in stream reaches and impoundments and determined the net effects at the watershed scale using chemical mass balances from each season of an annual cycle.
Methods
Study sites
The watersheds of the Huron (2377 km2) and Raisin (2737 km2) rivers drain predominately agricultural lands in southeastern Michigan before entering western Lake Erie (). They are characterized by hilly to moderately undulating topography in their upper watersheds, which are underlain by moraines, till deposits and outwash plains. The lower portions of the watersheds are underlain by sands and clays from glacial Lake Erie (CitationKnutilla and Allen 1975). Both rivers originate in “lake district” areas, but their middle and lower sections differ notably in their degree of impoundment (). These river impoundments are both artificial (created by dams) and natural, and most are controlled through dam operations or lake-level control structures with surface spillways. Land use in the two watersheds is similar, but the Huron has less agriculture and more forest and urban land area compared to the Raisin ().
Table 1 General characteristics of the Huron and Raisin watersheds.
Hydrology
Both rivers had water residence times in the mainstream channel, excluding impoundments, of about 5.5 days (). With impoundments, however, the Huron had a residence time more than 40-fold that of the Raisin. Residence times for mainstream reaches were estimated using average water velocity measurements from mainstem U.S. Geological Survey (USGS) gage stations on each river. Individual impoundment residence times were estimated using their volumes and average outflow rates. Residence times for all mainstem impoundments with surface areas of at least 10 ha and located more than 50 km downstream from the river origin were summed to obtain total impoundment residence times. Ford and Belleville lakes near the mouth of the Huron River were the largest impoundments in either watershed and were treated as a single unit because they are adjacent. Their combined surface area was 895 ha with an average depth of 6.0 m; each had surface water release at a dam-controlled outlet. The combined residence time for these two impoundments ranged from 33 to 72 days between April 2005 and April 2006 and averaged 47 days, about one-third of the total residence time for the Huron River mainstem under average flow conditions.
Daily mean stream discharge was estimated independently for each of the 14 sampling sites and each sampling event based on data from three USGS gage stations for the Raisin (Manchester 04175600, Adrian 04176000, and Monroe 04176500) and five gage stations for the Huron (Milford 0417000, New Hudson 04170500, Hamburg 04172000, Mill Creek 04173500, and Ann Arbor 04174500). The USGS flow data were used directly for sampling sites located near gages. For sample sites where gage data were not available, discharge was estimated based on drainage area–regression relationships developed from gage data for each watershed on each sampling date (average r2 value was 0.89). Drainage area for each sampling site was estimated using a 30-m resolution digital elevation map and Geographic Information Systems (GIS) software to determine contributing land area. Daily loads were estimated for each sampling date using discharge and assuming that stream nutrient concentrations were representative of the day in which the sample was taken.
Sampling approach and chemical analyses
Net nutrient processing along reaches and impoundments was estimated by longitudinal stream water sampling combined with stream flow measurements to calculate fluxes of N and P at different points along the river length (). By comparing nutrient flux between pairs of upstream–downstream sampling sites, N and P transformation and retention rates for each bounded reach and impoundment were calculated. Only pairs of sites with no major tributary inputs between them were selected. Sites were also chosen based on USGS gage locations in each watershed. The length of stream reaches between pairs of sample sites ranged from 2 to 3 km, which represents an average water transit time of < 2 hr between the two sampling points.
Seven paired sites across the watersheds () were sampled on 10 occasions from May 2003 to August 2005 to examine N and P removal in stream reaches and impoundments as the spatially-extensive portion of this study (). In addition, samples were taken every two weeks above and below the Ford-Bellville impoundment system from April 2005 to April 2006 to examine load change and seasonal differences in nutrient fractions as the time-intensive portion of this study. Sampling dates for the 14-site spatial comparison were chosen intentionally to include seasonal and discharge variation across annual cycles. Systematic sampling events above and below the Ford-Belleville impoundment system were supplemented as needed to include different flow conditions and seasons as well. Paired sites were sampled within 1 h of each other, and all sites for each sampling event were sampled within a 36-h time period.
Table 2 Mean and standard error (SE) of the mean for chemical variable concentrations at outlet sites of impoundments and downstream sites of stream reaches over all sampling events. These data come from sampling two impoundments, three Huron reaches, and two Raisin reaches.
Grab samples were collected from the thalweg of the river at approximately 0.5 m below the water surface. Aliquots of the water were filtered using a 0.2-μ m nylon filter in the field for analysis of dissolved N and P fractions. Filtered and unfiltered water was kept cool and dark for no more than 8 h during transport to the lab, where filtered sample aliquots were frozen for later analyses. Water for total particulate nitrogen (PN) was filtered through precombusted (4 h at 450 C) Whatman GF/F filters and frozen until analysis. Unfiltered water was also digested with potassium persulfate in an autoclave for total phosphorus (TP), and filtered water was analyzed by digestion for total dissolved phosphorus (TDP) and total dissolved nitrogen (TDN; details in following paragraph). In addition, pH, dissolved oxygen (DO), specific conductivity, and temperature were measured using a Hydrolab DataSonde 4a Water Quality Mulitprobe at the time of the grab sample.
Nutrient concentrations were determined using standard automated colorimetric procedures on a Technicon Auto Analyzer II as detailed by the methods of CitationDavis and Simmons (1979). Ammonia (NH4-N) was determined by the phenate method based on the indophenol blue reduction. Nitrate plus nitrite (reported here as NO3-N) was determined by the cadmium reduction method based on the azo dye reaction. Soluble reactive phosphorus (SRP) was determined by the molybdate-ascorbic acid method based on the formation of a phosphomolybdate blue complex. The TP and TDP (CitationMenzel and Corwin 1965) as well as TDN (CitationD'Elia et al. 1977) were determined as for SRP and NO3-N, respectively, after sample digestion. Prior to analysis, PN filters were thawed and dried at 60 C for 24 h; concentrations were then determined on a Carlo-Erba model 1110 CHN elemental analyzer using regressions against certified NIST standards. Particulate phosphorus (PP) was calculated as the difference between measured TP and TDP. Dissolved organic phosphorus (DOP) concentrations were calculated as the difference between TDP and SRP. Total nitrogen (TN) was calculated as the sum of TDN and PN. Dissolved organic nitrogen (DON) concentrations were determined from the difference between TDN and dissolved inorganic N (NH4-N + NO3-N). All nutrient concentrations are reported as elemental (not compound) weight.
Data analysis
Net nutrient processing was evaluated for each stream reach or impoundment from the change in concentrations and loads between pairs of sampling sites located above and below that reach or impoundment. To test for significant differences in N and P processing between reaches and impoundments over the 10 sampling events and seven pairs of sites of the spatially-extensive sampling, a repeated measures analysis of variance (ANOVA) was conducted with date as the unit of observation and load change or concentration change as the dependent variable (PROC MIXED procedure in SAS System; see CitationLittell et al. 1998). This procedure also included t-tests to determine whether mean differences for sample pairs were significantly different from zero, indicating statistically significant net removal or addition of N and P fractions.
Concentrations and daily loads for all N and P fractions for the time-intensive sampling of the Ford-Belleville impoundment system were analyzed using a paired t-test to compare mean concentrations of each N and P fraction for inflow and outflow sampling sites. Test assumptions were met, and the null hypothesis was no difference in mean concentrations or loads between sampling sites above and below the combined Ford-Belleville system.
Annual loads were estimated for the inlet and outlet of Ford-Belleville impoundments using AutoBeale, an implementation of the Beale Ratio Estimator (CitationRichards 1998). This estimation procedure first computes an average daily load based on dates with concentration data and then adjusts this average daily load according to the proportion of the annual flow included within the subset of dates that had corresponding concentration data. This adjusted average daily load is then multiplied by 365 to obtain an annual load estimate. In addition, AutoBeale breaks the year into several time intervals, each with their own average daily load, to account for seasonal and flow differences throughout the year. Error in annual estimates was evaluated by 95% confidence intervals based on differences between observed and predicted average daily loads within each time interval.
Results
Spatially-extensive sampling
Comparison of paired sites above and below impoundments and for stream reaches revealed pronounced differences in net nutrient processing spanning multiple years and seasons. Water quality variables exhibited different patterns of change across impoundments and reaches (). Water temperature increased significantly and specific conductivity decreased significantly across impoundments, but neither changed significantly across stream reaches. The DO and pH increased along stream reaches but did not change across impoundments. The repeated measures of ANOVA indicated significantly different conductivity changes in comparing impoundments to reaches.
Table 3 Mean, standard error of the mean (SE), and probability values (p-value) for chemical variable changes across impoundments and stream reaches including 7 site pairs over 10 sampling events. Negative mean values indicate that net removal of a chemical variable was observed. Impoundment and reach p-values < 0.05 indicate the means are different from zero according to t-tests performed. ANOVA p-values < 0.05 indicate that concentration and load changes between pairs of sites differ significantly for impoundments versus stream reaches according to the repeated measure ANOVA. Test results in bold indicate significance at the 0.05 level.
Average changes in concentrations and daily loads of nutrient fractions were greater across impoundments than over stream reaches (). In fact, none of the changes in N or P across stream reaches were statistically significant. Nutrient concentrations and daily loads show similar trends, so only concentration data are presented here. Concentrations of TN were significantly reduced across impoundments, but the response among individual N fractions was variable. A significant reduction was observed for NO3-N concentration, while PN and DON showed little change. Concentrations of NH4-N increased slightly, though not significantly. Nitrogen processing was significantly different between impoundment and reach site pairs according to the ANOVA test for TN, TDN and NO3-N. Concentrations of TP were lower at outlets than at inlets of impoundments, as were the TDP and SRP fractions. Furthermore, TP, TDP and SRP also showed significant differences in impoundment versus reach processing according to the repeated measure ANOVA test.
Temporally-intensive sampling
Frequent sampling above and below the Ford-Belleville impoundments showed significant N and P removal and further highlighted seasonal differences in the amounts and fractions of N and P removed. The results are not shown in this paper, but comparison of inflow to outflow concentrations by paired t-test documented significant differences in all N and P fractions except PP. The average concentrations of TP, TDP, DOP, SRP, TN, TDN and NO3-N all were significantly lower at the outlet in comparison with the inlet. In contrast, PN, DON and NH4-N concentrations increased significantly across the Ford-Belleville impoundment system. Concentration of PP was observed to increase, but not significantly, due to increased variance.
Comparison of annual nutrient loads entering and leaving the Ford-Belleville impoundments also revealed substantial removal of N and P in the impoundment. Annual loads for TN, TDN and NO3-N were reduced between the inlet and outlet of the impoundment by 18, 21 and 30%, respectively (). Consistent with concentration data, there were indications of a very small increase in the annual load of PN and DON across the impoundment. Annual loads of all P fractions decreased from impoundment inflow to outflow (). Specifically, TP and SRP loads were reduced by 32 and 72%, respectively.
Figure 2 Estimates of annual loads for N fractions at the inlet and outlet of the Ford-Belleville impoundment system from April 2005 through April 2006. Error bars represent 95% confidence intervals.
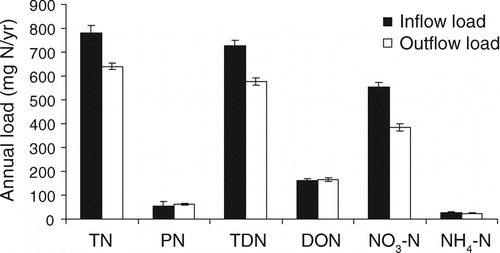
Figure 3 Estimates of annual loads for P fractions at the inlet and outlet of the Ford-Belleville impoundment system from April 2005 through April 2006. Error bars represent 95% confidence intervals.
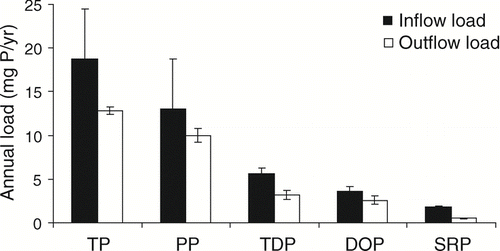
The magnitude of nutrient removal in the Ford-Belleville system varied seasonally for both N and P. Daily TN loads were consistently lower at the outlet than the inlet in the summer and autumn but showed higher and more variable incoming and outgoing loads in the winter months (). Outlet loads of PN, DON and NH4-N were higher than inlet loads during summer and autumn, whereas the opposite was true of NO3-N during this time period (). Loads of NO3-N in the impoundment's outflow were near zero for July through October, despite consistent input loading of NO3-N to the impoundment during this time.
Figure 4 Daily TN loads (kg N/d) at the inlet and outlet of Ford-Belleville impoundment system from April 2005 through April 2006. Daily mean discharge (m3/s) was measured at the impoundment outflow.
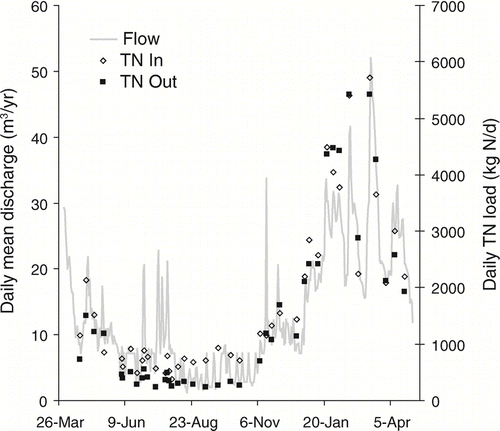
Figure 5 Daily NO3-N loads (kg N/d) at the inlet and outlet of Ford-Belleville impoundment system from April 2005 through April 2006. Daily mean discharge (m3/s) was measured at the impoundment outflow.
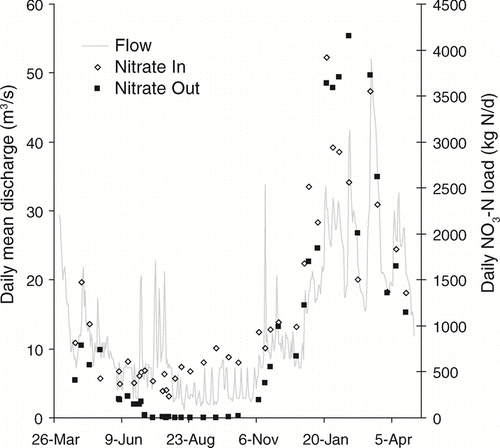
Daily TP loads exhibited even greater seasonal change than those for TN (). Summer loads of TP were consistently lower for the inflow compared to the outflow, resulting in a net export of P. During the winter months, however, incoming loads were often higher than lake export, indicating net removal. During summer and autumn low-flow conditions, PP loads in the outflow were consistently higher than those in the inflow. Outflow loads of TDP, DOP, and SRP exhibited less variability and were consistently lower than inflow loads throughout the year; only the SRP time series is shown ().
Discussion
Nutrient removal in impoundments and stream reaches
This study supports the expectation that the presence of impoundments in a landscape has a significant impact on nutrient transport and processing through the river network and to downstream locations. While 2–3 km stream reaches imposed little change on water quality variables, impoundments raised water temperature and reduced conductivity, TP, TDP, SRP, TN, TDN and NO3-N. Note that net changes in nutrient levels across the reaches in the present study may have been below detection limits due to the short residence time of the reaches.
Impoundments create conditions that favor N and P removal through denitrification, and settling and burial, which are likely to be enhanced by longer water residence times. Studies have found denitrification rates in sediments to increase when NO3-N concentrations increase, DO concentrations decrease, and water temperatures increase (CitationInwood et al. 2005, CitationWall et al. 2005, CitationSmith et al. 2006). These general conditions were observed in the Huron and Raisin watersheds, as the impoundments tended to increase temperature and reduce oxygen concentrations in the water passing through them (). In addition, the many impoundments of the Huron River increase its water residence time markedly (), favoring nutrient processing and removal (CitationStanley and Doyle 2002). Even if N and P removal mechanism rates were similar for reaches and impoundments, the additional water residence time of impoundments would result in more N and P removal than in streams.
Nitrogen removal in stream reaches and impoundments has been estimated from a relationship between water depth and time of travel (CitationSeitzinger et al. 2002), with fairly strong correlation (r2 = 0.73). With additional data, CitationDavid et al. (2006) developed an improved equation with higher correlation (r2 = 0.86) between the dependent and independent variables. We added the reach and impoundment data from the present study based on 10 sampling events, as well as more reach data from CitationOpdyke et al. (2006), and found close agreement to both models. Our observed differences in N removal between impoundments and river reaches are highly consistent with these relationships, strengthening our claim that impoundments are important locations for N removal in a stream network ().
Figure 8 Relationship between depth (m) divided by time of travel (y) and N removed (% of inputs) for a given impoundment or stream reach. Figure adapted from CitationDavid et al. (2006). Data are taken from the present study, CitationSeitzinger et al. (2002), CitationDavid et al. (2006), CitationJossette et al. (1999), CitationRoyer et al. (2004), and CitationOpdyke et al. (2006). Regression equations are from CitationSeitzinger et al. (2002) and CitationDavid et al. (2006). Single data point with error bars is the Ford-Belleville impoundment system, and range bars represent extreme removal estimates based on summer-only (49%) and winter-only (6%) sampling.
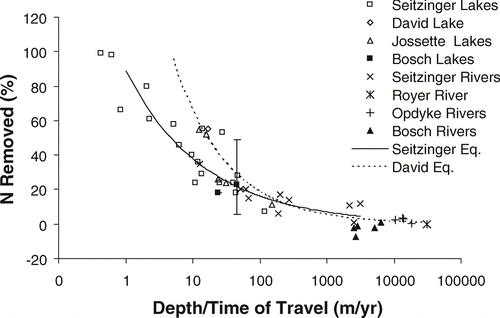
Annual nutrient load removal in impoundments
Quantification of annual N and P removal from the water passing through impoundments highlights their importance in reducing nutrient loading to downstream receiving waters. Loads of TN and TP decreased substantially (18% TN and 32% TP) from the inlet to the outlet of the Ford-Belleville impoundments. CitationKosek (1993) and CitationOwen (1991) reported reductions in annual TP loads by Ford Lake alone of 17, 36 and 37% in 1972, 1990 and 1992, respectively. An extensive study of 23 natural lakes and 27 reservoirs in Ohio, Indiana and Illinois (CitationWalker and Kuhner 1978) found consistent annual removal of N and P in these impoundments similar to removals observed in the Ford-Belleville impoundments. Median TP removal for these Midwestern natural lakes and reservoirs was 33 and 47%, and median TN removal was 24 and 23%, respectively. A comparison of impoundment inputs to riverine export in Lake Shelbyville, Illinois, found 19% of annual TN inputs were removed (CitationDavid et al. 2006). Previous work in similar impoundments corroborates the proportions of TP and TN removal that we observed in the present study.
Examining the removal of specific P fractions allows further insight into potential mechanisms responsible for impoundment P load reductions and implications for downstream systems. The SRP exhibited the most dramatic load reductions in the Ford-Belleville impoundment system. Removal of SRP in impoundments is expected because of its bioavailability and the high biological production common in impoundments. Thus, the downstream impact of P removal in the impoundment is heightened because the most removed fraction is the most bioavailable to immediately downstream systems. While no significant net annual change in PP was observed from the impoundment inlet to the outlet, rapid biological uptake of SRP during the summer months () should result in a corresponding increase in organic PP loads. A shift from the settling of inorganic PP (mostly P adsorbed to sediment particles) to the export of organic PP produced in the lake likely resulted in little annual change in total PP concentrations. This type of shift in the form of PP was observed in a study of four impoundments in southeastern Germany (CitationPaul 2003).
Seasonality of nutrient removal in impoundments
Seasonal trends in P inflow and outflow loads of the Ford-Belleville system further support inferences about the importance of material settling in impoundments. Daily TP loads in the impoundment outflow remained fairly consistent throughout the year, but inflow loads showed more variation with coefficients of variation of 0.6 and 1.4, respectively. Removal of TP was most pronounced during winter months when high episodic P loads were observed entering the lake. During summer months, inlet TP loads were low and the impoundments exported more TP than they received. This same pattern was previously shown by CitationOwen (1991). Production of TP in impoundments during summer has been reported elsewhere (CitationHillbricht-Ilkowska 1999, CitationTeodoru and Wehrli 2005). The rate of internal dissolved P loading from remineralization and flux from sediments along with riverine P input to the impoundment during this time was greater than dissolved plus particulate P removal rates, leading to a net export of P from the impoundment. Episodic, high inlet P loading events contrasted to relatively constant and lower outlet P loading, supporting the inference that material settling in impoundments can remove large proportions of incoming P (CitationStanley and Doyle 2002).
Nitrogen removal, NO3-N in particular, was most pronounced during the summer and autumn months when conditions favored biological assimilation and denitrification. Biological assimilation and later settling of the organic material may have allowed transport of N to the anoxic hypolimnion or sediments where denitrification can occur. Alternatively, entering stream waters were colder and more dense than lake water () and could have directly entered the hypolimnion and supplied NO3 to the sediments for denitrification. Relative to the rest of the year, July through October had higher inlet NO3 concentrations, higher lake water temperatures and lower lake DO concentrations, which would favor greater denitrification. Using daily NO3 loads in the Ford-Belleville outflow () as an approximation of whole-lake NO3 conditions, the time series fits expectations of denitrification-dominated N removal. Outflow NO3 remains low throughout the summer and early autumn when conditions for denitrification are favorable, followed by a moderate increase in NO3 loads in November. In December and January, NO3 increases more abruptly (seen also for SRP) during the period prior to ice formation when the dimictic lakes in the area completely mix. Replenishment of oxygen in bottom waters may have abruptly reduced denitrification during this time period. Nitrification of ammonium produced by the mineralization of decaying organic particles would lead to the re-introduction of NO3 into the water column and result in the observed increases in NO3. These seasonal observations support the inference that denitrification is the major process that accounts for N removal in this impoundment, as has been concluded in similar studies (CitationHillbricht-Ilkowska 1999, CitationWall et al. 2005, CitationDavid et al. 2006).
Seasonal changes in N and P removal have important implications for downstream systems in addition to simply reducing overall annual loads. The seasonal changes observed for P and N are uncoupled in their timing because P in the system is mostly particulate and N is mostly in dissolved inorganic forms. Because seasonal changes in N and P loads are uncoupled, N:P stoichiometric ratios change throughout the year and may represent dissimilative and physical removal processes rather than algal uptake (Fig 9). The changes in the N:P ratios are abrupt, and there appears to be a strong influence of discharge because periods of higher or lower discharge often coincide with changes in the N:P inflow versus outflow balance. Despite this variability over the year, the N:P ratios never approach or drop below the Redfield ratio of 16:1 (), and NO3 and NH4 concentrations () are sufficient such that the system appears to remain consistently P-limited. One implication of these seasonal differences and uncoupling is that impoundments can substantially alter the delivery and timing of N and P fractions to downstream ecosystems.
Figure 9 N:P ratios of daily TN (mol N/d) and TP (mol P/d) loads at the inlet and outlet of Ford-Belleville impoundment system from April 2005 through April 2006. Daily mean discharge (m3/s) was measured at the impoundment outflow.
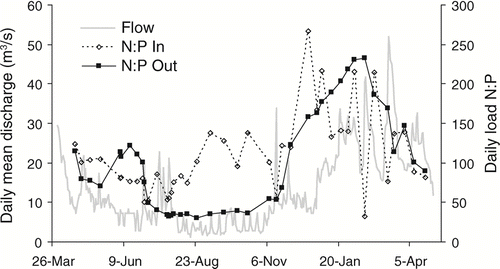
Our seasonal analysis of N and P removal has relevance to interpreting results of previous research as well as for the development of sampling programs to determine N and P transport through aquatic systems. If we had only sampled during specific 3-month seasons to estimate annual loads for inlet and outlet, N removal estimates would be 49% for summer, 46% for autumn, 6% for winter and 8% for spring sampling periods. Furthermore, seasonal plots for Ford-Belleville (, , , ) show net removal and production of different nutrient fractions at different times of the year, so any sampling effort that does not quantify seasonal variation will inaccurately predict the annual influence on downstream systems. Thus caution must be used when interpreting seasonal data on nutrient processing, and annual data should be used in estimating the role of impoundments in developing watershed management plans for nutrient removal.
Potential management implications
The work presented in this paper represents one of three related efforts to better understand the role of impoundments in the Huron and Raisin watersheds. The first paper described a catchment-scale budgeting approach, which showed that the more heavily impounded Huron watershed retained a larger proportion of the N and P inputs than it received compared to the less-impounded Raisin (CitationBosch and Allan 2008). Second, an application of the Soil and Water Assessment Tool (SWAT) model to the Huron and Raisin watersheds (CitationBosch 2008) predicted that annual river exports of TP and TN from the Huron watershed were respectively 41 and 54% below that expected in the absence of impoundments. Furthermore, model scenario runs predicted that more numerous smaller impoundments near nutrient sources were most effective at nutrient removal. The extensive nutrient sampling presented in the present paper confirms these previous efforts by identifying impoundments as hotspots for nutrient removal in these watersheds.
From this work, we can identify several implications for management of watersheds and their impoundments. As dam removal becomes more common in the Midwestern United States, our studies document the nutrient removal capacity of impoundments and suggest that these benefits should be considered when prioritizing dams for removal. Our work also has implications for dam operations and management of lake-level control structures. This work has shown that increasing the residence time of water is a major factor in the effectiveness of impoundments to remove nutrients. By reducing water levels during low-flow periods and holding back water during high-flow events, managers could increase water residence time and allow greater chance for nutrient removal in impoundments. A third management implication would be future planning for development. A watershed with numerous impoundments to remove nutrients would be a better targeted area for future development plans rather than an adjacent watershed with no impoundments and likely less capacity for nutrient removal.
Acknowledgments
This work was supported by grants and fellowships from the University of Michigan School of Natural Resources and Environment, a U.M. Rackham Dissertation Award, and NSF-DEB 0423385. We are grateful to the NOAA Great Lakes Environmental Research Lab for the use of their facilities for nutrient analyses. Ken Guire and the Center for Statistical Consultation and Research at the University of Michigan provided helpful assistance with statistical analysis of data. We would also like to thank Bill Currie and Pete Richards for their insights related to this work.
References
- Alexander , R. B. , Smith , R. A. and Schwarz , G. E. 2000 . Effect of stream channel size on the delivery of nitrogen to the Gulf of Mexico . Nature , 403 ( 6771 ) : 758 – 761 .
- Alexander , R B , Bohlke , J. K. , Boyer , E. W. , David , M. B. , Harvey , J. W. , Mulholland , P. J. , Seitzinger , S. J. , Tobias , C. R. , Tonitto , C. and Wollheim , W. M. 2009 . Dynamic modeling of nitrogen losses in a river networks unravels the coupled effects of hydrological and biogeochemical processes . Biogeochemistry , 93 : 91 – 116 .
- Bosch , N. S. 2008 . The influence of impoundments on riverine nutrient transport: An evaluation using the Soil and Water Assessment Tool . J. Hydrol. , 355 ( 1-4 ) : 131 – 147 .
- Bosch , N. S. and Allan , J. D. 2008 . The influence of impoundments on nutrient budgets in two catchments of Southeastern Michigan . Biogeochemistry , 87 ( 3 ) : 325 – 338 .
- D'Elia , C. F. , Steudler , P. A. and Corwin , N. 1977 . Determination of total nitrogen in aqueous samples using persulfate digestion. Limnol . Oceanogr. , 22 ( 4 ) : 760 – 764 .
- David , M. B. , Wall , L. G. , Royer , T. V. and Tank , J. L. 2006 . Denitrification and the nitrogen budget of a reservoir in an agricultural landscape. Ecol . Appl. , 16 ( 6 ) : 2177 – 2190 .
- Davis , C. O. and Simmons , M. S. 1979 . Water chemistry and phytoplankton field and laboratory procedures , Ann Arbor : Great Lakes Research Division, University of Michigan .
- Dixit , A. S. , Hall , R. I. , Leavitt , P. R. , Quinlan , R. and Smol , J. P. 2000 . Effects of sequential depositional basins on lake response to urban and agricultural pollution: a palaeoecological analysis of the Qu'Appelle Valley, Saskatchewan . Freshwater Biol. , 43 ( 3 ) : 319 – 337 .
- Earl , S. R. , Valett , H. M. and Webster , J. R. 2006 . Nitrogen saturation in stream ecosystems . Ecology , 87 ( 12 ) : 3140 – 3151 .
- Ejsmontkarabin , J. T. , Weglenska , T. and Wisniewski , R. J. 1993 . The effect of water-flow rate on zooplankton and its role in phosphorus cycling in small impoundments . Water Sci. Technol. , 28 ( 6 ) : 35 – 43 .
- Ensign , S. H. and Doyle , M. W. 2006 . Nutrient spiraling in streams and river networks . J. Geophys. Res.-Biogeosci. , 111 ( G4 )
- Harris , G. P. 2001 . Biogeochemistry of nitrogen and phosphorus in Australian catchments, rivers and estuaries: effects of land use and flow regulation and comparisons with global patterns . Mar. Freshwater Res. , 52 ( 1 ) : 139 – 149 .
- Harrison , J. A. , Maranger , R. J. , Alexander , R. B. , Giblin , A. E. , Jacinthe , P. , Mayorga , E. , Seitzinger , S. P. , Sobota , D. J. and Wollheim , W. M. 2009 . The regional and global significance of nitrogen removal in lake and reservoirs . Biogeochemistry , 93 : 43 – 147 .
- Hillbricht-Ilkowska , A. 1999 . Shallow lakes in lowland river systems: Role in transport and transformations of nutrients and in biological diversity . Hydrobiologia , 409 : 349 – 358 .
- Inwood , S. E. , Tank , J. L. and Bernot , M. J. 2005 . Patterns of denitrification associated with land use in 9 midwestern headwater streams . J. N. Am. Benthol. Soc. , 24 ( 2 ) : 227 – 245 .
- Jossette , G. B. , Leporcq , B. , Sanchez , N. and Philippon , X. 1999 . Biogeochemical mass-balances (C, N, P, Si) in three large reservoirs of the Seine Basin (France) . Biogeochemistry , 47 ( 2 ) : 119 – 146 .
- Kelly , V. J. 2001 . Influence of reservoirs on solute transport: a regional-scale approach . Hydrol. Proc. , 15 ( 7 ) : 1227 – 1249 .
- Knutilla , R. L. and Allen , W. B. 1975 . Water resources of the River Raisin Basin, southeastern Michigan , Reston , VA : U. S. Geological Survey .
- Kosek , S. 1993 . A phosphorus loading analysis and lake model for Ford and Belleville Lakes, Washtenaw County, January-December, 1992 , Lansing , MI : Surface Water Quality Division, Michigan Department of Natural Resources .
- Littell , R. C. , Henry , P. R. and Ammerman , C. B. 1998 . Statistical analysis of repeated measures data using SAS procedures . J. Animal Sci. , 76 ( 4 ) : 1216 – 1231 .
- Maurer , W. R. , Claflin , T. O. , Rada , R. G. and Rogala , J. T. 1995 . Volume loss and mass-balance for selected physicochemical constituents in Lake Pepin, Upper Mississippi River, USA . Regul. Rivers , 11 ( 2 ) : 175 – 184 .
- Menzel , D. W. and Corwin , N. 1965 . The measurement of total phosphorus liberated in seawater based on the liberation of organically bound fractions by persulfate oxidation. Limnol . Oceanogr , 10 : 280 – 281 .
- O'Brien , J. M. and Dodds , W. K. 2008 . Ammonium uptake and mineralization in prairie streams: chamber incubation and short-term nutrient addition experiments . Freshwater Biol. , 53 ( 1 ) : 102 – 112 .
- Opdyke , M. R. , David , M. B. and Rhoads , B. L. 2006 . Influence of geomorphological variability in channel characteristics on sediment denitrification in agricultural streams . J. Environ. Qual. , 35 ( 6 ) : 2103 – 2112 .
- Owen , A. L. 1991 . Limnological study including total phosphorus and total organic carbon budgets of Ford Lake reservoir, 1990-1991 , MS thesis Eastern Michigan University .
- Paul , L. 2003 . Nutrient elimination in pre-dams: results of long term studies . Hydrobiologia , 504 ( 1-3 ) : 289 – 295 .
- Reid , N. J. and Hamilton , S. K. 2007 . Controls on algal abundance in a eutrophic river with varying degrees of impoundment (Kalamazoo River, Michigan, USA) . Lake Reserv. Manage. , 23 : 219 – 230 .
- Richards , R. P. 1998 . Estimation of pollutant loads in rivers and streams: A guidance document for NPS programs , Denver , CO : U. S. Environmental Protection Agency .
- Royer , T. V. , Tank , J. L. and David , M. B. 2004 . Transport and fate of nitrate in headwater agricultural streams in Illinois . J. Environ. Qual. , 33 ( 4 ) : 1296 – 1304 .
- Seitzinger , S. P. , Styles , R. V. , Boyer , E. W. , Alexander , R. B. , Billen , G. , Howarth , R. W. , Mayer , B. and Van Breemen , N. 2002 . Nitrogen retention in rivers: model development and application to watersheds in the northeastern USA . Biogeochemistry , 57 ( 1 ) : 199 – 237 .
- Smith , L. K. , Voytek , M. A. , Bohlke , J. K. and Harvey , J. W. 2006 . Denitrification in nitrate-rich streams: Application of N-2: Ar and N-15-tracer methods in intact cores . Ecol. Appl. , 16 ( 6 ) : 2191 – 2207 .
- Stanley , E. H. and Doyle , M. W. 2002 . A geomorphic perspective on nutrient retention following dam removal . Bioscience , 52 ( 8 ) : 693 – 701 .
- Teodoru , C. and Wehrli , B. 2005 . Retention of sediments and nutrients in the Iron Gate I Reservoir on the Danube River . Biogeochemistry , 76 ( 3 ) : 539 – 565 .
- Walker , W. W. and Kuhner , J. An empirical analysis of factors controlling eutrophication in midwestern impoundments . International Symposium on the Environmental Effects of Hydraulic Engineering Works . Knoxville : University of Tennessee .
- Wall , L. G. , Tank , J. L. , Royer , T. V. and Bernot , M. J. 2005 . Spatial and temporal variability in sediment denitrification within an agriculturally influenced reservoir . Biogeochemistry , 76 ( 1 ) : 85 – 111 .