Abstract
High mortality (84–89%) of stocked rainbow trout (Oncorhynchus mykiss) in Twin Lakes, Washington, has been partially blamed on predation from non-native largemouth bass (Micropterus salmoides). We examined the gut-content of 434 largemouth bass and applied a bioenergetics model to determine the consumption demand on hatchery-released rainbow trout and other prey species in the Twin Lakes. Largemouth bass consumed approximately 4915 (95% CI; 2393–13,452) fall stocked rainbow trout in South Twin. No rainbow trout consumption was observed in North Twin, despite a similar stocking regime. Approximately 6.3% (95% CI; 3–17%) of total fall stocked rainbow trout in South Twin were consumed by largemouth bass. Rainbow trout stocked in the fall ranged from 100 to 160 mm total length and were all subject to predation by large largemouth bass ⩾300 mm. Large largemouth bass consumed rainbow trout that reached up to 210 mm in length and 58% of bass body length. No predation was observed on larger rainbow trout (215–370 mm) stocked during the spring. Smaller largemouth bass ⩽299 mm consumed primarily invertebrates, including crayfish, and did not consume rainbow trout in either lake. During spring and summer in South Twin Lake, large largemouth bass consumed principally golden shiner (Notemigonus crysoleucas) and crayfish. In North Twin Lake, golden shiner constituted most of the larger bass diet for the entire year. Differences in macrophyte distribution, bathymetry, temperature and/or predator-prey demographics likely influenced variability in largemouth bass predation between lakes. Largemouth bass predation did not account for overall rainbow trout mortality.
Largemouth bass (Micropterus salmoides) is indigenous to the eastern and midwestern United States and has been widely introduced into the western United States to increase recreational fishing opportunities and control nuisance fish species (CitationFuller et al. 1999). Despite the abundance of recreational largemouth bass fisheries (CitationMoyle 2002, CitationWydoski and Whitney 2003), surprisingly little data are available regarding predation rates and impacts on native species such as salmonids (CitationBonar et al. 2005, CitationChristensen and Moore 2008). In the western United States where trout and salmon fisheries particularly have generally high economic and aesthetic values (CitationMoyle 2002, CitationWydoski and Whitney 2003), bass predation is of special concern. Often, rainbow trout (Oncorhynchus mykiss) are stocked in productive, meso- and eutrophic lakes to supplement trout populations where natural reproduction and survival are already limited due to warm epilimnetic temperatures (⩾20 C) and low hypolimnetic dissolved oxygen (⩽4mg/L; CitationHeidinger 1999, CitationSwales 2006). Largemouth bass has become well established in most productive lowland lakes in the western United States, exacerbating habitat limitations and making predation on hatchery trout a particular management concern (CitationFuller et al. 1999, CitationMoyle 2002, CitationWydoski and Whitney 2003). Bass predation on hatchery-raised trout may diminish catch rates and significantly alter the economic value of the trout fishery. Understanding largemouth bass consumption of hatchery rainbow trout is crucial to developing economically and ecologically sound strategies to improve trout survival and to maintain viable fisheries in productive lakes (CitationFayram and Sibley 2000, CitationBaldwin et al. 2003, CitationFritts and Pearsons 2004, CitationLiao et al. 2004).
Relevant studies of predation effects on salmonids have mostly focused on other piscivores, such as walleye (Sander vitreum), smallmouth bass (Micropterus dolomieu) and northern pikeminnow (Ptychocheilus oregonensis; CitationPoe et al. 1991, CitationBaldwin et al. 2003, CitationFritts and Pearsons 2004, CitationNaughton et al. 2004). These studies all addressed predation impacts on migrating juvenile salmon and trout in reservoir or riverine environments. In contrast, largemouth bass typically prefer macrophyte beds in lowland warmwater lakes, ponds or slow rivers (CitationWydoski and Whitney 2003), reducing the chance of encounter with coldwater salmonids. During periods of cooler temperatures in spring and fall, however, wild and hatchery-stocked salmonids may inhabit productive littoral zones in lakes, where they are potentially exposed to largemouth bass predation (CitationBonar et al. 2005, CitationChristensen and Moore 2008). Recent studies suggest that predation on salmonids may be substantial during juvenile salmon migrations and after small trout are stocked into lakes with largemouth bass populations (CitationBonar et al. 2005, CitationChristensen and Moore 2008).
Mortality of hatchery rainbow trout has been estimated to range from 84 to 89% in North and South Twin lakes, Washington (Christensen and Moore, Washington State University, unpubl. data,). Fishery managers have speculated that largemouth bass predation may be a key component to high trout mortality. Our objective was to estimate the consumption demand of largemouth bass on hatchery-released rainbow trout in North and South Twin lakes. We estimated the number of rainbow trout consumed in relationship to the total number stocked in the lakes and identified ontogenetic and temporal consumption demand of largemouth bass on rainbow trout and other principal prey species. Prey length was explored in relationship to largemouth bass length to evaluate prey-size vulnerability. We also compared largemouth bass consumption dynamics between the lakes, which had contrasting littoral zones, fall littoral temperatures and macrophyte distributions. North Twin Lake had extensive macrophyte monocultures and South Twin Lake had relatively sparse and patchy macrophyte distribution. We estimated the population of largemouth bass in each lake and used a bioenergetics model to estimate consumption demand.
Study site
North and South Twin lakes are dimictic, meso-eutrophic water bodies located on the reservation of the Colville Confederated Tribes (CCT) in northeastern Washington. North and South Twin lakes are similar in size (371 and 413 ha, respectively) and depth (). The lakes are connected by a small channel. During summer stratification both lakes experience periods of low hypolimnetic dissolved oxygen (1–4 mg/L) and high summer epilimnetic temperatures (>20 C) (Christensen and Moore, Washington State University, unpubl. data,). In general, North Twin Lake has an extensive littoral zone (depths ⩽2 m deep) comprising 20% (74.2 ha) of the lake surface area and is dominated by expansive monocultures of the macrophyte watershield (Brasenia schreberi). In contrast, South Twin Lake has a smaller littoral area comprising only 9% (37.2 ha) of the lake surface area and has steeper near shore depth contours and a very patchy distribution of watershield.
Figure 1 Map depicting the bathymetry (in meters), macrophyte distribution (shaded), sampling transects (numbered), and location of North and South Twin Lakes, Washington. North Twin Lake transects were utilized for largemouth bass population analysis in 2004 and all transects in both lakes were utilized in the 2005 diet analysis. Arrows represent approximate location of hatchery rainbow trout release sites.
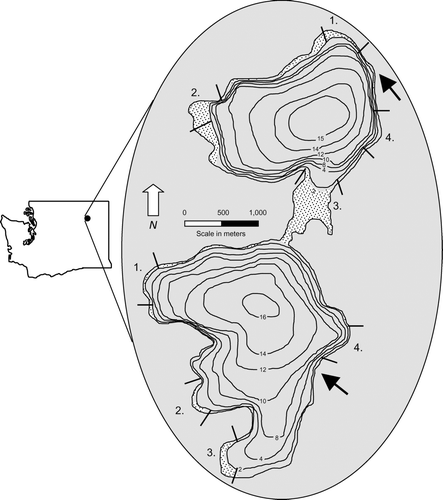
Hatchery trout, rainbow and introduced but naturalized reproducing brook trout (Salvelinus fontinalis), comprise a popular recreational fishery in the Twin Lakes. Since 2003, the CCT have stocked 100,000–150,000 rainbow trout in each lake annually (E. Schallenberger, CCT Fisheries Manager, September 2008, pers. comm.). Approximately 80% of these fish were stocked in the fall (Sep–Oct) and were ⩽160 mm, while fish stocked in the spring and early summer (Apr–Jun) were much larger, ⩾240 mm. Largemouth bass and golden shiner (Notemigonus crysoleucas) were apparently illegally introduced in the 1980s and early 1990s and have become abundant in both lakes. Largemouth bass has become a popular target species for recreational anglers. The Twin Lakes also contain small populations of native species, including redside shiner (Richardsonius balteatus), bridgelip sucker (Catastomus columbianus) and pygmy whitefish (Prosopium coulteri; CitationChristensen and Moore 2008).
Materials and methods
Rainbow trout stocking
The CCT fish hatchery provided and stocked rainbow trout into North and South Twin lakes during 2005. Stocking regimes of rainbow trout did not differ considerably between lakes or from standard Twin Lake stocking protocols (). The number of stocked rainbow trout was estimated gravimetrically as fish were loaded into hatchery trucks. Stocking of both lakes took place within one day of each other. Stocking sites in both lakes were in nonvegetated areas where largemouth bass were presumed to be less abundant. Fish sampling results indicated that stocked trout dispersed throughout the lakes after stocking. Hatchery-raised rainbow trout were identified by the absence of adipose fins that were removed prior to stocking and with visual elastomer tags implanted in the anal fin or near the eye while at the tribal fish hatchery.
Table 1 Hatchery-raised rainbow trout (Oncorhynchus mykiss) stocking rates and size ranges in North and South Twin Lakes for 2005.
Fish collections
Largemouth bass were collected in June, July, August and September 2005 from the littoral zone (depths ⩽2 m) of North and South Twin lakes. Fish were sampled with a Smith-Root model GPP 5.0-DC electrofishing boat (Smith-Root, Inc.). Largemouth bass were sampled at night from 8 stratified littoral transects (4 from each lake) for 30 min of electrofishing time per transect.No largemouth bass were sampled from transects 4 from either lake (). Collected fish were weighed to the nearest 1 g and measured to the nearest 5 mm in total length.
Stomach contents of largemouth bass were sampled by flushing the gut with water from a modified gastric lavage, consisting of a 60 mL syringe attached to catheter tubing (CitationKamler and Pope 2001). For larger fish, 2 syringes were utilized to increase flushing volume. Stomach flushing efficiency was assumed to be approximately 85–90%. Stomach contents were collected from individual fish and preserved separately in 10% formalin. Measurements and stomach flushing were conducted as expeditiously as possible, and fish were quickly released back into the lake to minimize mortalities.
Stomach contents were identified and measured in the lab following procedures outlined by CitationChristensen and Moore (2007). Stomach prey organisms were separated into the following groups; invertebrates (without crayfish), crayfish, golden shiner and rainbow trout. Consumption dynamics based on largemouth bass ontogeny and prey size were explored. We separated largemouth bass into size classes of 100–199 mm (small), 200–299 mm (medium) and ⩾300 mm (large) to identify and quantify consumption demand based on functional feeding groups previously identified by CitationChristensen and Moore (2007).
Population dynamics
The population of largemouth bass in North Twin Lake was estimated in 2004 by a multiple census mark–recapture study using the Schnabel method with Chapman's adjustment, as described in CitationRicker (1975). Negative statistical bias may result from a low number of recaptured individuals (CitationRicker 1975); however, we assumed this bias to be avoided if recaptured fish totaled 4 or more individuals (CitationRicker 1975). Because recaptured fish totaled <25 in our study, we assumed the estimate to be non-normally distributed (Van Den Avyle and Hayward 1999) and used a Poisson frequency distribution chart contained in CitationRicker (1975) to estimate 95% confidence intervals for the population estimate.
North Twin Lake was sampled in June, July, August and September 2004 to obtain the population estimate. Sampling followed methods described previously. All collected largemouth bass were marked with an anal fin hole punch, then released. We assumed (1) marked fish were identifiable at recapture, (2) both marked and unmarked fish had equal probability of capture, (3) mortality was equal for marked and unmarked fish, and (4) marked and unmarked fish were randomly mixed within the lake (Van Den Avyle and Hayward 1999). We only considered fish ⩾100 mm (which are more susceptible to electrofishing) in our population estimate to avoid sampling bias and assumed negligible recruitment or immigration during the study period (Van Den Avyle and Hayward 1999).
We assumed the population of largemouth bass to be similar during both years due to comparable catch-per-unit-effort (CPUE) and consistent spring climatic events during critical spawning periods. Relatively warm and consistent spring temperatures can often stabilize bass recruitment from year to year due to constant temperature and water levels (CitationBonar et al. 2005). The proportion of bass of each size class from the 2005 sample was applied to the population results to estimate relative abundance within the feeding groups. South Twin sample size was 1.9 times larger than North Twin Lake, despite equal sampling efforts, and the bass population in South Twin Lake was estimated by multiplying the North Twin Lake estimate by 1.9.
Bioenergetics modeling
Consumption demand of largemouth bass on rainbow trout, golden shiner, crayfish and invertebrates was estimated with Fish Bioenergetics 3.0 (CitationHanson et al. 1997), a program that applies a mass-balance approach for which consumption input must equal somatic growth, gonadal growth, metabolic cost, and waste excretion for a single fish. Estimates from a single fish are then extrapolated to the entire population. The model estimated consumption for each feeding group using calculated population estimates, mortality rates, diet proportions, predator and prey energy densities, and surface water temperatures. Annual mortality rates were determined by a catch-curve analysis using the program Fishery Analyses and Simulation Tools 2.0.1 (CitationFAST 2001; Auburn University). Age was determined from scale analysis of annuli formation. Scales were removed from the left dorsal region just anterior to the dorsal fin.
Diet proportions were estimated by weight (g) for each feeding group during each sampling excursion. We assumed the diet composition in October, when no stomach analyses were performed, to be similar to September results. Simulations were initiated on June 1 during peak spring trout stocking. Predator and prey energy densities were obtained from published literature values () and were assumed to be constant throughout the year (CitationYule and Luecke 1993). Invertebrates were assumed to be 10% indigestible while fish were 3.3% (CitationYule and Luecke 1993). Simulations ended 18 October, shortly after rainbow trout stocking ceased, when cold temperatures (13 C) were assumed to limit bass predation.
Table 2 Energy density estimates taken from the literature of fish and invertebrates common to the Twin Lakes.
Temperature was measured from the surface to the bottom of each lake at 1 m increments during each sampling excursion. Temperatures varied only slightly from the surface to about 2 m depth, the extent of the littoral zone where largemouth bass were most active and the maximum range of electrofishing efficiency. Measured surface temperatures from the lakes were used for the bioenergetics simulation and ranged from 16.5 C in June, 23.8 C in late July and early August, to 13.3 C in mid-October. During early September, surface water temperatures were 18.1 C in South Twin while only 16.8 C in North Twin Lake.
The mean weights for largemouth bass in each size class during June were used as the simulation starting weights. The model was programmed to fit the p-value (proportion of the maximum consumption) to the consumption estimate of the largemouth bass feeding group (CitationHanson et al. 1997). Estimated p-values were usually near 0.48 and remained constant throughout the simulation. We applied an 8% loss of mass due to spawning in early June for bass ⩾300 mm (CitationHanson et al. 1997).
The bioenergetics model calculated consumption rates for each largemouth bass feeding group in kilograms per day. We calculated the total consumption rate by summing daily estimates and converting the total to metric tonnes (CitationLiao et al. 2004). Confidence intervals (95%) were generated for the total consumption rates by running the upper and lower bounds of the population estimates in the model while all other parameters remained constant. Total numbers of rainbow trout and golden shiner consumed were estimated by dividing total consumption weight by the respective mean weights of individuals of each species sampled from bass stomachs. This was done for each feeding group and then summed to obtain estimates of total trout and shiner consumed by the entire bass population.
Results
Population dynamics
Multiple mark-recapture census results () show that the number of largemouth bass sampled in South Twin Lake was nearly double that sampled in North Twin Lake (). A greater proportion of largemouth bass from North Twin were in the medium-size class compared to South Twin, where the greatest proportion of bass was in the smallest size class. Age distribution was determined (), and annual mortality for both lakes was estimated to be near 36%.
Table 3 Schnabel multiple-census population data for North Twin Lake, 2004. Numbers are largemouth bass recaptured (marked), unmarked (captured), and marked (at-large) during each sampling period.
Table 4 Population estimates (N) and confidence intervals (CI) for largemouth bass feeding groups (FG) sampled from North and South Twin Lakes, 2005. Percentages are proportions of the total population for each feeding group.
Table 5 Mean length (mm), range (in parenthesis), and sample size (n) of prey consumed by largemouth bass by feeding groups (FG) in North (NT) and South Twin (ST) lakes, 2005. Age estimates are for largemouth bass.
Marked fish may have been underestimated, violating one of the assumptions previously described regarding mark-recapture techniques. Some anal fins of marked fish were partially regenerated, leaving a distinct oval scar. This made the determination of marked fish more complicated (CitationBonar et al. 2005). However, we felt the number of recaptured fish was satisfactory to obtain a population estimate for use in the bioenergetics model.
Diet dynamics
We examined the stomachs of 222 largemouth bass from North Twin Lake and 212 from South Twin Lake. In North Twin, 50 (23%) of the stomachs were empty, compared to 23 (11%) from South Twin. Details of the relative importance of individual prey taxa for largemouth bass size classes using percent by weight and index of relative importance (IRI; CitationGeorge and Hadley 1979) metrics are presented in CitationChristensen and Moore (2007). Ontogenetic feeding behavior was observed; invertebrates were the most important dietary item for small- and medium-sized largemouth bass, while golden shiner was the most important for large largemouth bass. Large largemouth bass in South Twin principally consumed rainbow trout in September just after the trout were stocked. No rainbow trout were found in largemouth bass stomachs from North Twin, despite similar stocking densities and schedules. No trout were sampled from largemouth bass stomachs from either lake during the spring stocking period. Crayfish also constituted a larger component of the diet of largemouth bass in South Twin than in North Twin Lake.
A positive relationship between largemouth bass length and prey length was observed in both lakes for larger prey organisms such as rainbow trout, golden shiner and crayfish. A weak predator-prey relationship was observed between largemouth bass and invertebrates but was statistically insignificant ( and ). In South Twin, largemouth bass consumed rainbow trout up to 58% of their own body length and golden shiner and crayfish up to 48 and 41% of their body length, respectively. In North Twin Lake, largemouth bass consumed golden shiner and crayfish up to 46 and 53% of their own body length, respectively. Rainbow trout were the largest prey consumed by largemouth bass in the Twin Lakes.
Consumption demand
Consumption demand by weight (kg) for each prey species varied temporally and with largemouth bass ontogeny ( and ). In general, total consumption estimates were greater in South Twin than in North Twin, likely due to higher population estimates in the former. However, consumption of golden shiner was greater in North Twin, while the consumption of rainbow trout was only observed in South Twin Lake. Small and medium largemouth bass in South Twin consumed more crayfish than largemouth bass from North Twin Lake. Larger bass were principally piscivorous, consuming mostly golden shiner in both lakes. Only the larger largemouth bass consumed rainbow trout, and only during September and October when smaller trout were stocked and temperatures were adequate (⩽18 C) for trout to access littoral areas. During summer, higher water temperatures (⩾20 C) likely precluded rainbow trout from most littoral habitats (CitationBiggs 2007). Lower water temperatures approaching 13 C likely reduced largemouth bass activity and consumption rates during the fall.
Figure 3 Daily consumption of crayfish, golden shiner, rainbow trout, and invertebrates by largemouth bass feeding groups (100–199, 200–299, ⩾ 300 mm) in North Twin Lake (left) and South Twin Lake (right) during 2005 as estimated by a bioenergetics model.
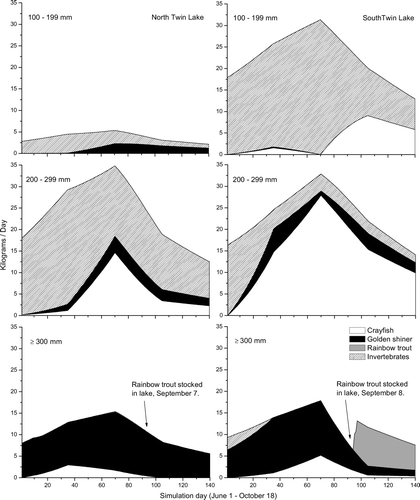
Figure 4 Total consumption in metric tonnes (± 95% confidence interval) from June 1 to October 18 of golden shiner, rainbow trout, crayfish, and invertebrates by largemouth bass feeding groups (100–199, 200–299, ⩾300 mm) in North and South Twin Lakes during 2005 as estimated by a bioenergetics model.
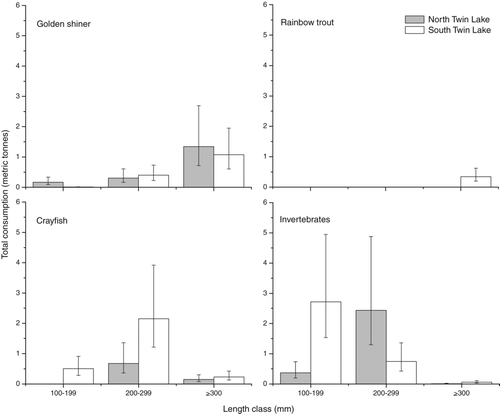
Large largemouth bass in South Twin Lake consumed an estimated 4915 (95% CI; 2393–13,452) hatchery stocked rainbow trout. This consumption represents 6.3% (95% CI; 3–17%) of total fall stocked rainbow trout in South Twin Lake. Largemouth bass consumed an estimated total of 121,942 golden shiner (95% CI; 52,468–375,254) in North Twin and 90,455 golden shiner (95% CI; 43,001–251,236) in South Twin Lake. Small largemouth bass accounted for 28 and 3% of these golden shiner consumed in North and South Twin, respectively. Medium largemouth bass accounted for 31 and 49%, and large bass constituted 41 and 48% of the total golden shiner consumption in North and South Twin, respectively.
The estimate for total prey species consumed takes into consideration variability in prey size consumed by different bass feeding groups. For example, medium-sized largemouth bass may consume more, but smaller, golden shiner compared to larger-sized bass. However, larger largemouth bass consumed larger golden shiners, yielding an overall greater mass.
Discussion
Hatchery-raised rainbow trout were an important part of the diet of largemouth bass in South Twin Lake in fall 2005. Largemouth bass predation on trout, taken with other possible sources for mortality such as angling, low hypolimnetic dissolved oxygen (1–4 mg/L) and high summer epilimnetic temperatures (>20 C; Christensen and Moore, Washington State University, unpubl. data) may have exerted a population-wide influence on rainbow trout abundance. However, a rainbow trout reduction by 6.3% (95% CI; 3–17%) due to largemouth bass predation alone does not account for rainbow trout mortality rates of 84–89%. Also, similar mortality estimates for North Twin Lake, despite an apparent lack of measured bass predation, indicates other more substantial mortality contributors. This suggests that other factors such as angling and habitat limitations may have had a more substantial influence on trout survival in the Twin Lakes. Also, dense macrophyte beds and cooler fall temperatures may have precluded any measurable bass predation on trout in North Twin Lake.
Spatial and temporal habitat overlap between largemouth bass and rainbow trout may be much greater in South Twin than in North Twin, possibly explaining why no trout consumption was observed in North Twin Lake. Patchy macrophyte distribution and steeper depth contours in South Twin, producing increased edge habitats, may be one reason for increased habitat overlap between rainbow trout and largemouth bass (CitationWard and Neumann 1998, CitationChristensen and Moore 2008). Dense macrophyte monocultures in North Twin Lake may have greatly reduced trout access to littoral regions, reducing exposure to largemouth bass predation. Ambush techniques used by largemouth bass in macrophyte beds may have been more successful on golden shiner, supported by greater consumption of golden shiner in North Twin Lake (CitationWard and Neumann 1998). In South Twin Lake, largemouth bass may have developed a more search-and-encounter feeding behavior that increased the probability of interaction with trout and crayfish (CitationChristensen and Moore 2008, CitationWard and Neumann 1998).
Temperature differences between lakes may have influenced largemouth bass predation rates on rainbow trout. Littoral temperatures during September when rainbow trout were stocked were 18.1 C in South Twin while only 16.8 C in North Twin Lake. Largemouth bass bioenergetics is strongly correlated with water temperature. Largemouth bass may have been more active in South Twin Lake during September when rainbow trout were stocked and less active in North Twin Lake due to temperature differences. Also, stocked rainbow trout that generally require cold water may have become lethargic due to warmer water in South Twin Lake, making them more vulnerable to bass predation. Although the 2 lakes are connected, most tributary input and output occur in North Twin Lake, possibly accounting for these temperature disparities.
Habitat variability between the lakes may have influenced largemouth bass consumption rates on crayfish and invertebrates. Habitat complexity may have also influenced the onset of largemouth bass piscivory. Greater open water habitat could have encouraged crayfish populations and increased their exposure to largemouth bass predation in South Twin Lake. However, in North Twin Lake, small largemouth bass were much more piscivorous, suggesting a greater encounter frequency, capture success rate and/or abundance of smaller golden shiner (CitationSavino and Stein 1982, Citation1989, CitationPost 2003). The proportion of medium and large largemouth bass was much greater in North Twin Lake, suggesting an earlier onset to piscivory or greater survival rates (CitationPost 2003). Earlier onset to piscivory, coupled with macrophyte architecture and distribution, may be critical to population structuring of larger largemouth bass (CitationBettoli et al. 1992, CitationDibble and Harrel 1997, CitationGarcia-Berthou 2002, CitationPost 2003).
Macrophyte architecture and littoral habitat complexity have been studied extensively and found to influence largemouth bass predation on prey such as bluegill (Lepomis macrochirus), fathead minnows (Pimephales promelas) and crayfish (CitationSavino and Stein 1982, Citation1989, CitationBettoli et al. 1992, CitationDibble and Harrel 1997, CitationTrebitz et al. 1997, CitationWard and Neumann 1998, CitationValley and Bremigan 2002). However, little is known how littoral habitat complexity influences largemouth bass predation on salmonids. CitationBonar et al. (2005) found predation on juvenile salmon by largemouth bass to be extensive in shallow lakes with widespread littoral regions. Predation correlated to migrational movements and to densities of juvenile salmon. The authors suggested that spatial segregation in larger lakes and reservoirs with less extensive littoral regions and macrophyte distribution may limit spatial overlap and largemouth bass densities. However, CitationWard and Neumann (1998) found that largemouth bass consumed more crayfish and pelagic fishes in a lake with a small littoral zone, steep depth contours and patchy macrophyte distribution as apposed to a shallow lake dominated by macrophytes.
The findings from CitationWard and Neumann (1998) were similar to those reported here. The correlation of largemouth bass predation with increased densities of stocked rainbow trout in our study is similar to natural pulses of migrating salmon described in CitationBonar et al. (2005). Therefore, prey densities, predator densities and habitat complexity (including temperature) all appear to play an important role in the structuring of largemouth bass diets (CitationCarpenter and Kitchell 1993, CitationWard and Neumann 1998, CitationBonar et al. 2005).
Note that the spatial segregation between salmonids and largemouth bass discussed in CitationBonar et al. (2005) was focused on large lakes and reservoirs of the Pacific Northwest with little to no macrophyte and littoral development. These conditions could severely limit establishment of largemouth bass and reduce their potential for predation on salmonids. Although the South Twin littoral is less extensive than that of North Twin, adequate habitat is available for largemouth bass populations to exist in both lakes. Therefore, a mosaic of macrophyte beds adjacent to deeper water and open-substrate habitats could increase frequency of encounter between largemouth bass and rainbow trout but requires further examination.
Prey and predator length also seemed to influence largemouth bass consumption in the Twin Lakes. It was apparent that largemouth bass consumed larger prey as the bass increased in length. Prey fish may escape the window of predation vulnerability as they grow in length; however, vulnerability to predation based on length is likely dependent on the size distribution of large predators and their relative abundance. We found golden shiner to be exposed to predation by all bass functional feeding groups. Golden shiner seemed to be most vulnerable to predation until they reached about 190 mm in length. The largest golden shiner sampled from the lakes was 225 mm (Christensen and Moore, Washington State University, unpubl. data), suggesting they are exposed to some level of predation by largemouth bass for most, if not all of their lives. In contrast, rainbow trout were only consumed by largemouth bass ⩾300 mm. The length range of consumed rainbow trout was 130–210 mm, which is the approximate length range of rainbow trout stocked in the fall by the CCT fish hatchery. Rainbow trout >210 mm would have a greater chance at escaping predation by large largemouth bass than would smaller fish, and most stocked rainbow trout would not be threatened by largemouth bass <300 mm. Naturally recruited rainbow trout, or fish stocked at a total length <130 mm, could be exposed to predation by smaller largemouth bass feeding groups, increasing the influence predation may have on the Twin Lake trout populations. During the spring, larger rainbow trout (240–370 mm) were stocked, and we did not document any largemouth bass predation on salmonids during that time, suggesting that these larger fish were beyond the vulnerability range of largemouth bass consumption or that habitat separation was greater for these larger trout.
Predation vulnerability also has temporal and spatial components that interact based on the time that consumable rainbow trout spend within or near the littoral region. Elevated temperatures (⩾20 C) during the summer may preclude rainbow trout from utilizing littoral habitats at the time when largemouth bass are most active (CitationChristensen and Moore 2008). This spatial segregation may minimize rainbow trout annual mortality. Our model predictions suggest temperatures <16 C can greatly reduce the consumption demand on trout by largemouth bass. In September, when rainbow trout were stocked, South Twin Lake surface waters were warmer (18.1 C) than North Twin Lake (16.8 C), possibly elevating largemouth bass metabolism and feeding activity. These differences in temperature could have caused the disparity in predation estimates between the lakes. Due to metabolic constraints, largemouth bass may stop feeding completely for extended periods of time when temperatures drop below 10 C and drastically reduce predation rates on rainbow trout or other prey species (CitationWydoski and Whitney 2003).
CitationCarpenter and Kitchell (1993) found that in a similar lake, largemouth bass caused a decline in the abundance of stocked rainbow trout and other prey fishes, initiating top-down mediated trophic shifts. Researchers found in 3 shallow Washington State lakes that largemouth bass accounted for 98% of the predation of juvenile coho salmon (CitationBonar et al. 2005). In Long Lake Reservoir, >50% of the migrating juvenile coho salmon in 1999–2000 were estimated to have been consumed by largemouth bass (CitationBonar et al. 2005); however, largemouth bass predation on rainbow trout in South Twin Lake was not nearly as extensive as that reported in CitationBonar et al. (2005) for other salmonid species.
If largemouth bass predation on hatchery trout is a significant problem, fishery managers should consider adjusting stocking regimens to reduce unnecessary mortality by largemouth bass. For example, it has been suggested that stocking trout in the fall increases survival because warm- and cool-water predators such as largemouth bass and walleye become less active (CitationWiley et al. 1993, CitationYule et al. 2000). However, we observed largemouth bass predation on rainbow trout to occur only during the fall due to spatial habitat overlap and stocking of vulnerable sized fish. By mid-October, however, low temperatures reduced largemouth bass predation rates on rainbow trout. Predation during the fall could be further reduced if trout are stocked at larger sizes and/or later during the fall or early winter when largemouth bass are more lethargic due to cold temperatures. In the Twin Lakes, water temperatures are optimal for rainbow trout during the late fall and early winter, reducing stress and increasing the amount of habitat available for feeding, in addition to the reduced risk of predation (CitationChristensen and Moore 2008).
Stocking larger rainbow trout during the spring seemed to prevent largemouth bass predation. Stocking smaller trout earlier in the spring when water temperatures are cold enough to reduce largemouth bass activity could be effective. These smaller trout may disperse sufficiently as the water warms to avoid predation. Stocking increased numbers of smaller trout may mitigate predation but could be an uneconomical alternative (CitationChristensen and Moore 2007). Targeted fishing regulations, such as slot-limits or increasing bass limits, may be an effective tool to reduce bass predation on trout. In severe cases, it may become necessary to develop control programs for unwanted largemouth bass using approved mechanical and/or chemical methodologies (CitationWydoski and Wiley 1999).
Preventive, instead of reactive, management at the regional scale could preclude further introductions of largemouth bass into waters where they would be detrimental to native and/or economically important salmonid fisheries. CitationBonar et al. (2005) suggested prioritizing where largemouth bass predation is most detrimental in order to maintain important nonthreatening largemouth bass fisheries and to control populations damaging to valuable salmonid populations. Most lowland lakes in Washington and Oregon contain populations of largemouth bass (CitationBonar et al. 2005), both legally and illegally introduced (CitationFuller et al. 1999). Identifying priority areas and preventing the unnecessary transplantation of largemouth bass is necessary to protect salmonids in lowland lakes and should be a part of any predator management plan.
Acknowledgments
We would like to thank the Colville Confederated Tribes (CCT) for assistance and funding for this project. Additionally, we wish to thank Dan Fairbank and Jerry Marco of the CCT for their expertise. Assistance with field sampling by Marvin Bob, Allen Hammond and Levi Morris was especially helpful. We also thank 3 anonymous reviewers who provided necessary insight into manuscript revision.
Notes
References
- Baldwin , C M , McLellan , J G , Polacek , M C and Underwood , K . 2003 . Walleye predation on hatchery releases of kokanees and rainbow trout in Lake Roosevelt, Washington . N Am J Fish Manage. , 23 : 660 – 676 .
- Bettoli , P W , Maceina , M J , Noble , R L and Betsill , R K . 1992 . Piscivory in largemouth bass as a function of aquatic vegetation abundance . N Am J Fish Manage. , 12 : 509 – 516 .
- Biggs , M J . 2007 . Seasonal habitat use and movement by Columbia Redband Trout in the Twin Lakes, Washington , MS Thesis Pullman , WA : Washington State University .
- Bonar , S A , Bolding , B D , Divens , M and Meyer , W . 2005 . Effects of introduced fishes on wild juvenile coho salmon in three shallow Pacific Northwest lakes . Trans Am Fish Soc. , 134 : 641 – 652 .
- Carpenter , S R and Kitchell , J F . 1993 . The trophic cascade in lakes , Cambridge , , UK : Cambridge University Press .
- Christensen , D R and Moore , B C . 2007 . Differential prey selectivity of largemouth bass functional feeding groups in Twin Lakes, Washington . Lake Reserv Manage. , 23 : 39 – 48 .
- Christensen , D R and Moore , B C . 2008 . Diet composition and overlap in a mixed warm- and coldwater fishery . J Freshw Ecol. , 23 ( 2 ) : 195 – 204 .
- Cummins , K W and Wuychek , J C . 1971 . Caloric equivalents and investigations in ecological energetics . Verh Internat Verein Limnol. , 18 : 1 – 151 .
- Dibble , E D and Harrel , S L . 1997 . Largemouth bass diets in two aquatic plant communities . J Aquat Plant Manage. , 35 : 74 – 78 .
- Fayram , A H and Sibley , T H . 2000 . Impact of predation by smallmouth bass on sockeye salmon in Lake Washington, Washington . N Am J Fish Manage. , 20 : 81 – 89 .
- [FAST] Fishery Analyses and Simulation Tools . 2001 . Version 2.0.1 , Auburn , AL : Alabama Auburn University .
- Fritts , A L and Pearsons , T N . 2004 . Smallmouth bass predation on hatchery and wild salmonids in the Yakima River, Washington . Trans Am Fish Soc. , 133 : 880 – 895 .
- Fuller , P L , Nico , L G and Williams , J D . 1999 . Nonindigenous fishes introduced into inland waters of the United States , Bethesda , MD : American Fisheries Society . Special Publication 27
- George , E L and Hadley , W F . 1979 . Food and habitat partitioning between rock bass (Ambloplites rupestris) and smallmouth bass (Micropterus dolomieui) young of the year . Trans Am Fish Soc , 108 : 253 – 261 .
- Garcia-Berthou , E G . 2002 . Ontogenetic diet shifts and interrupted piscivory in introduced largemouth bass (Micropterus salmoides) . Int Rev Hydrobiol. , 87 : 353 – 363 .
- Hanson , P C , Johnson , T B , Schindler , D E and Kitchell , J F . 1997 . Fish bioenergetics 3.0 , Madison , WI : University of Wisconsin-Madison, Center for Limnology .
- Heidinger , R C . 1999 . “ Stocking for sport fisheries enhancement ” . In Inland Fisheries Management in North America. , 2nd ed , Edited by: Kohler , C C and Hubert , W A . 375 – 401 . Bethesda , MD : American Fisheries Society .
- Kamler , J F and Pope , K L . 2001 . Nonlethal methods of examining fish stomach contents . Rev Fish Sci. , 9 : 1 – 11 .
- Liao , H , Pierce , C L and Larscheid , J G . 2004 . Consumption dynamics of the adult piscivorous fish community in Spirit Lake, Iowa . N Am J Fish Manage. , 24 : 890 – 902 .
- Moyle , P B . 2002 . Inland fishes of California , Berkley , CA : University of California Press .
- Naughton , G P , Bennett , D H and Newman , K B . 2004 . Predation on juvenile salmonids by smallmouth bass in the Lower Granite Reservoir system, Snake River . N Am J Fish Manage. , 24 : 534 – 544 .
- Poe , T P , Hansel , H C , Vigg , S , Palmer , D E and Prendergast , L A . 1991 . Feeding of predaceous fishes on out-migrating juvenile salmonids in John Day Reservoir, Columbia River . Trans Am Fish Soc. , 120 : 405 – 420 .
- Post , D M . 2003 . Individual variation in the timing of ontogenetic niche shifts in largemouth bass . Ecology , 84 : 1298 – 1310 .
- Ricker , W E . 1975 . Computation and interpretation of biological statistics of fish populations . B Fish Res Board Can. , 191 : 96 – 100 .
- Savino , J F and Stein , R A . 1982 . Predator-prey interaction between largemouth bass and bluegills as influenced by simulated, submersed vegetation . Trans Am Fish Soc. , 111 : 255 – 266 .
- Savino , J F and Stein , R A . 1989 . Behavior of fish predators and their prey: habitat choice between open water and dense vegetation . Environ Biol Fish. , 24 : 287 – 293 .
- Swales , S . 2006 . A review of factors affecting the distribution and abundance of rainbow trout (Oncorhynchus mykiss Walbaum) in lake and reservoir systems . Lake Reserv Manage. , 22 : 167 – 178 .
- Trebitz , A , Carpenter , S , Cunningham , P , Johnson , B , Lillie , R , Marshall , D , Martin , T , Narf , R , Pellett , T Stewart , S . 1997 . A model of bluegill-largemouth bass interactions in relation to aquatic vegetation and its management . Ecol Model. , 94 : 139 – 156 .
- Valley , R D and Bremigan , M T . 2002 . Effects of macrophyte bed architecture on largemouth bass foraging: implications of exotic macrophyte invasions . Trans Am Fish Soc. , 134 : 234 – 244 .
- Van , B en , Avyle , M J and Hayward , R S . 1999 . “ Dynamics of exploited fish populations ” . In Inland Fisheries Management in North America, , 2nd ed , Edited by: Kohler , C C and Hubert , W A . 127 – 166 . Bethesda , MD : American Fisheries Society .
- Ward , S M and Neumann , R M . 1998 . Seasonal and size-related food habits of largemouth bass in two Connecticut lakes . J Freshw Ecol. , 13 : 213 – 220 .
- Wiley , R W , Whaley , R A , Satake , J B and Fowden , M . 1993 . Assessment of stocking hatchery trout: a Wyoming perspective . N Am J Fish Manage. , 13 : 160 – 170 .
- Wydoski , R S and Whitney , R R . 2003 . Inland fishes of Washington, , 2nd ed , Bethesda , MD : American Fisheries Society .
- Wydoski , R S and Wiley , R W . 1999 . “ Management of undesirable species ” . In Inland fisheries management in North America, , 2nd ed , Edited by: Kohler , C C and Hubert , W A . 403 – 426 . Bethesda , MD : American Fisheries Society .
- Yule , D L and Luecke , C . 1993 . Lake trout consumption and recent changes in the fish assemblage of Flaming Gorge Reservoir . Trans Am Fish Soc. , 122 : 1058 – 1069 .
- Yule , D L , Whaley , R A , Mavrakis , P H , Miller , D D and Flickinger , S A . 2000 . Use of strain, season of stocking, and size at stocking to improve fisheries for rainbow trout in reservoirs with walleyes . N Am J Fish Manage. , 20 : 10 – 18 .