Abstract
This experiment was conducted to determine if quagga mussels (Dreissena bugensis) settle and grow on different types of artificial substrates preferentially in Lake Mead, Nevada–Arizona, one of the first known populations of the mussel in the western United States. Six substrates, acrylonitrile butadiene styrene (ABS) plastic, high density polyethylene (HDPE) plastic, concrete underlayment board (CUB), aluminum, stainless steel and fiberglass were cut into 10 cm squares and placed at different depths in the Boulder Basin of Lake Mead for approximately 1 yr in a modified randomized block design. Half the substrates were removed and replaced every 2 mo, and the other half remained in the water for the duration of the study. No preference for substrate type could be determined, but settlement was limited by depth. Mussel settlement on substrates at depths from 6–28 m was significantly greater than on substrates from 32–54 m (F = 5.54; p < 0.001). This divergence in settlement at different depths is likely due to the water quality characteristics at these depths. No relationship was found between mussel settlement on substrates and veliger concentrations in the water column. Mussel settlement was limited when the lake was destratified between January and March 2009. Based on this experiment, materials placed in Lake Mead below 32 m will have greatly reduced mussel settlement, especially if deployed when the lake is destratified.
In January 2007, the quagga mussel (Dreissena bugensis), an invasive species, was found at the Las Vegas Boat Harbor Marina in the Boulder Basin of Lake Mead (CitationLaBounty and Roefer 2007). Since their discovery, quagga mussels have been found in numerous locations in the Lower Colorado River and reservoirs in Southern California and Arizona. This mollusk and its close relative, the zebra mussel (D. polymorpha), have caused severe ecological and economical damage to many water bodies they inhabit and the zebra/quagga mussel has become arguably the most serious nonindigenous biofouling pest introduced into North American freshwater systems (CitationLaBounty and Roefer 2007). In Lake Erie, phytoplankton populations have declined as a result of over filtering of the water by both zebra and quagga mussels (CitationMay and Marsden 1992). Lake Huron's natural food web was seriously altered as a result of fish and zooplankton population declines caused by changes in the system because of the mussel invasion. Salmon, alewife and zooplankton populations have declined, causing an energy shift from pelagic to benthic zones resulting in a $19 million/yr decrease in revenues for Lake Huron sport fisheries (Michigan DNR 2008).
Lake Mead holds more than 37 × 109 m3(9 trillion gallons) of water, is 106 km in length, supplies 80% of Las Vegas’ domestic water needs, provides 3.3 billion kWh of power via hydroelectric generation from Hoover dam and provides a valuable sport fishery to millions of visitors and residents every year (CitationLaBounty and Burns 2005, CitationLaBounty and Roefer 2007). Typically, the epilimnion of Lake Mead ranges from 0–15 m in depth, the metalimnion from 15–35 m and the hypolimnion below 35 m. Determining mussel settling preferences on different substrates will help researchers and resource managers decide how to mitigate mussel colonization on architectural structures, public infrastructures and sensitive environmental areas.
Researchers have monitored veliger, juvenile and adult zebra and quagga mussel population densities in water bodies in the United States and Europe. Studies have demonstrated that mussel settlement on artificial substrates is based on the texture, chemical composition and orientation of the substrate in water column (CitationYankovich and Haffner 1993, CitationMarsden and Lansky 2000, CitationCzarnoleski et al. 2004, CitationKobak 2005). In addition, depth and environmental cues such as light penetration and the presence of a microscopic biofilm appear to play critical roles related to settlement (CitationMarsden 1992, CitationKavouras and Maki 2003). Mussels will grow on almost any shape of material ranging from a tube to a plate (CitationKilgour and Mackie 1993, CitationMarsden and Lansky 2000, CitationKobak 2005). The quantification of mussels on a plate is a more accurate and simple process than counting on all surfaces of a tube. Different types of materials have been evaluated for their suitability for zebra and quagga mussel monitoring including acrylic, aluminum, asbestos, black Pelxiglas, black steel, brass, clear Plexiglas, copper, fiberglass, galvanized iron, galvanized steel, glass, limestone, pine, polypropylene, PVC (polyvinyl chloride), raw steel, resocart, rubber, stainless steel, Teflon™, vinyl, wood and zinc (CitationKilgour and Mackie 1993, CitationMarsden and Lansky 2000, CitationCzarnoleski et al. 2004, CitationKobak 2005). The main objectives of this experiment were to (1) determine if quagga mussels settle and grow preferentially on different substrate materials and (2) evaluate settlement at different depths to determine where minimum and maximum colonization occurs.
Materials and methods
Experimental design
Five substrate samplers were deployed between Sentinel Island and the Boulder Islands in the Boulder Basin of Lake Mead (). All sampling units were located in the same area (approximately 650 m2) following the guidance of CitationMarsden and Lansky (2000) who recommended focusing monitoring experiments on the number of units and not on the number of replication sites in a well-mixed body of water such as Lake Mead. The location was marked by an emerged yellow buoy (GPS coordinates 36°3′13″N; 114°45′04″W) with “Government Property Restricted Access” written on the side. Six substrates were tested, including acrylonitrile butadiene styrene (ABS) plastic, high density polyethylene (HDPE), concrete underlayment board (CUB), aluminum, stainless steel and fiberglass, all purchased from local vendors. Aluminum, concrete, fiberglass and steel were previously confirmed to allow zebra and quagga mussel colonization in Europe and the Great Lakes region (CitationKilgour and Mackie 1993, CitationMarsden and Lansky 2000, CitationKobak 2005).
Figure 1 Location of substrate experiment in Lake Mead indicated by dot with arrow. 1 = Boulder Basin, 2 = Virgin Basin, 3 = Overton Arm, 4 = Temple Basin, 5 = Gregg Basin. Image by Dr. Chad Cross at UNLV.
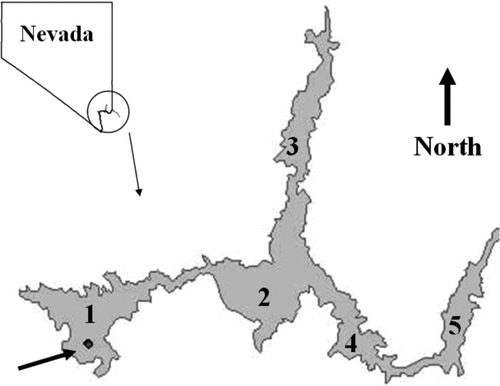
Substrates were cut into 10 cm squares and connected with stainless steel screws, washers and stop nuts to a 15 cm piece of conduit pipe. These substrate units were secured in polypropylene rope every 4.4 m (). The depth at the experimental location was approximately 63 m. Buoys were submerged at least 1.2 m below the surface of the water on each sampler to prevent mishandling by boaters, and they were connected to a rope containing the substrates with approximately 3 m of chain. The anchor was connected to the rope with approximately 7.6 m of chain.
Figure 2 Substrate sampler field design. Odd numbers were permanent substrates; even numbers were removable substrates.
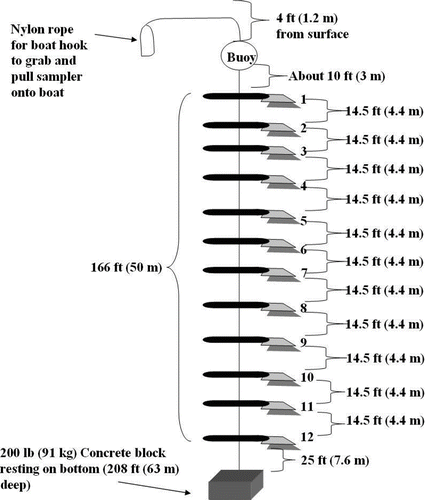
Substrates were connected to the sampler using a modified randomized block design, which assumes that within each block the experimental conditions are homogeneous. Additionally, a permanent substrate was attached at each depth as well as a removable substrate of the same type that was easily removed for bimonthly analysis and replaced with a new substrate. Lake levels were monitored daily on the Bureau of Reclamation's website (http://www.usbr.gov/lc/riverops.html) to ensure that the buoys remained submerged or would be within reach at the next sampling event. The samplers remained in the water for a period of 12 months from 27 March 2008 to 10 March 2009. Although the lake water level varied from 336.63 to 340.73 m between March 2008 and March 2009, there was no change in sample depths from the lake surface because all substrates were suspended in the water column. Samplers were deployed on 27 March 2008 and were removed and inspected approximately every 60 d for 1 yr. Collection dates were 27 May, 30 July, 24 September, 20 November and 10 January in 2008, and the final date was 10 March 2009. One sampler was lost in May 2008 and no replacement was made due to logistic reasons.
Water quality characteristics were measured with a YSI 6600 multiparameter water quality probe (YSI Inc., Yellow Springs, OH) every 6 h for the duration of the study at a United States Geological Survey (USGS) water quality monitoring station located within a few hundred feet of the experiment site (GPS coordinates: 36°3’8”N; 114°45’3”W). Characteristics measured every 6 m by the USGS included water temperature, pH, dissolved oxygen and conductivity.
Every 2 mo the samplers were removed from the water column and placed on the deck of the boat, with the substrates submerged in lake water to reduce stress on the mussels. A 2-month period was considered an adequate time for a biofilm to form on the substrate (more than 2 weeks) and for mussels to attach (CitationKavouras and Maki 2003). Every 2 mo each side of the permanent and removable substrates was photographed on a grid-laden platform, and the removable substrates were detached and placed in a plastic bag cooled with ice and transported to the laboratory. The removable substrates were replaced with a new substrate of the same type. After taking photographs, the permanent substrates were placed in a bucket of lake water until returned to the water column.
Each month starting in August 2008, a vertical plankton tow was conducted with a 64-μm plankton net with a 15 cm dia opening to enumerate the number of quagga mussel veligers present in the water near the area of the experiment. Samples were collected by gently lowering the plankton net through the water column at a rate of approximately 1 m/sec (a steady and unhurried hand-over-hand motion), and the net was towed for 60 m. After each tow, the net was washed from the outside from top to bottom with distilled water to rinse veligers into the collection cup. The collection cup side screens were also washed from top to bottom and the contents were emptied into a 500 mL Nalgene bottle. The collection cup was rinsed twice with small amounts of water and emptied into the same bottle. The bottle was labeled with the date and location. Sample bottles were kept on ice while in the field. Once sampling was complete, the plankton net was decontaminated by being soaked in a 5 gallon bucket filled with white vinegar for about 45 min. The plankton net was thoroughly rinsed with clean water after each soaking. The sample was preserved using ethyl alcohol until it comprised 25% of the final sample volume. Samples were refrigerated until analysis.
In the laboratory, the sample was added to an Imhoff settling cone with a venoset delivery system. The veligers were allowed to settle in the Imhoff cone for a minimum of 24 h. Successive aliquots of settled sample were transferred into a centrifuge tube (50 mL) until no sediment remained in the Imhoff cone. This method was developed by the Bureau of Reclamation's Technical Service Center (Denver, CO; Hosler Forthcoming) and is a modified combination of the Standard Method (10200 G) Zooplankton Counting Techniques and the US Army Corps of Engineers (USACE) method for calculating Dreissena veligers. Veligers were quantified in a 1 mL concentrated subsample of the plankton tow using a Sedgwick-Rafter cell with a cross polarizing microscope and reported in number per liter (Zeiss Discovery.V8 stereomicroscope; Carl Zeiss, Inc., Peabody, MA).
Laboratory work
After each sampling event, substrates were taken to the laboratory and stored in a 0 C freezer until analysis, which took no longer than 3 weeks. Mussels were carefully removed from the upper and lower side of the plate (not the edges) and placed in a 9 × 9 cm gridded petri dish with 1.3 cm square grids. Plates were then examined using a Zeiss Discovery.V8 stereomicroscope at 20× power to quantify any mussels that were not visible without magnification. Mussels in the gridded petri dishes were then counted using 6.4–20× power, depending on the number of mussels to be counted. When all mussels were counted on both sides of the plate and in the gridded petri dish, the total number of mussels was divided by 0.01032256 to determine the number of mussels/m2. The mussel density on some plates was so great that counting each individual mussel would have been extremely time-consuming, especially on the permanent plates. In this case, mussels in a 2.7 cm square area were counted from 3 random locations on the plate, averaged, and divided by 0.000027 to determine number of mussels/m2.
Statistics
To test for spatial independence of the 4 sampling locations a Moran's I was employed. Data were non-normally distributed due to unequal variances, thus a Friedman's nonparametric test for randomized block designs was conducted using SAS version 9.1 (SAS Institute Inc., Cary, NC).
Veliger concentrations were compared with the average number of settled mussels for each date with the Kolmogorov-Smirnov test using SAS version 9.1. The Kolmogrov-Smirnov test was used to determine if the distribution of veliger concentrations throughout the year was different from the distribution of mussels on substrates throughout the year.
To determine if there were any relationships between water quality characteristics and mussel settlement at depth, a nonparametric Spearman correlation was conducted using SPSS. The data collected by the USGS were averaged over the 2-mo sampling periods and compared with overall averages of mussel settlement at each depth. The level of significance for all statistical analyses was set at α= 0.05.
Results
The results of the Moran's I test indicated that the sampler locations were independent of each other (no autocorrelation), making ANOVA an appropriate statistical analysis (I values ranged from −0.59 to 0.53 with p values ranging from 0.322 to 0.495).
Removable substrates
For the removable substrates, exactly 5% of the data were missing, so these data points were removed from analysis as recommended by CitationTabachnick and Fidel (2007). Some substrates were missing when pulled out of the water and others were lost during counting procedures. Raw mussel counts by substrate type and depth were recorded (). Friedman's test found that the block (depth) was significant (F = 5.54; p < 0.001), but there were no differences in mussel settlement between substrate types (Friedman's χ2= 2.7485; p = 0.739). Depths from 10–28 m had significantly greater mussel densities than the lower depths of 37–54 m. The densities per square meter of settled mussels in removable substrates were 342,000 (ABS), 293,000 (aluminum), 189,000 (fiberglass), 150,000 (HDPE), 122,000 (CUB) and 70,000 (stainless steel); however, no significant difference was detected among these substrates, and the overall mean density for all substrates was 194,000 mussels/m2. Overall mussel settlement increased by date from March 2008 to September 2008 but decreased significantly from January 2009 to March 2009 ().
Permanent substrates
Four permanent plates, all CUB, were lost during the experiment and not included in analyses. These concrete substrates were weighted for the analysis to adjust for the loss, and the final number of mussels/m2 was determined from the weighted value based on a prorated year. All of the other substrates remained on the sampler for the duration of the study (approximately 1 yr). On the permanent substrates, there was no significant difference in settlement between substrate types (Friedman's χ2= 6.2165; p = 0.2857), but there was a significant difference among depths (F = 35.04; p < 0.001). The substrate with the highest number of mussels was the same as with the removable substrates: ABS plastic with an average of 2,640,000/m2. The mussel densities in other permanent substrates per square meter were 2,160,000 (fiberglass), 1,440,000 (aluminum), 1,420,000 (HDPE plastic), 1,050,000 (stainless steel), and 647,000 (CUB). No significant difference among different substrate types was detected, and the overall mean density for all substrates was 1,560,000 mussels/m2.
Veligers
The distributions of veliger abundance and mussel abundance were significantly different (KS = 0.5, p = 0.002, indicating to reject the null hypothesis that the 2 distributions were the same; ). The distributions are different mainly because of a lag phase that occurred between veliger presence in the water column and settlement of pediveligers and juveniles on substrates because the proportion of competent veligers ready to settle correlated significantly with mussel settlement (Gerstenberger et al. Forthcoming).
Discussion
Substrate preference
All substrates tested in this experiment provided a suitable place for the mussels to attach and grow. There was no statistically significant difference in mussel settlement between substrate types. In Lake Michigan, 65 d after deployment of artificial substrates of wood, fiberglass, concrete, limestone, aluminum, raw steel and galvanized steel, zebra mussel densities were not significantly different among most of these materials except a significantly lower number of mussels was found on galvanized steel (CitationMarsden and Lansky 2000). One possible explanation why mussels may not have had an overall substrate preference is the stochastic nature of the first settling event as a veliger (CitationMarsden and Lansky 2000). In the post-veliger stage, settlement is dictated more by preference because of their ability to detach byssal threads (CitationMarsden and Lansky 2000).
For the removable and permanent substrates in this study, settlement was highest on ABS plastic but not by a significant margin. The aluminum plates had the second highest densities on the removable substrates (293,000/m2) and the third highest densities on the permanent substrates (1,440,000/m2; ). These results are contrary to CitationKilgour and Mackie (1993) but in agreement with CitationMarsden and Lansky (2000) and CitationKobak (2005). CitationKilgour and Mackie (1993) reasoned that lower densities on aluminum were due to known toxic effects of aluminum on Unionid mussels, which may be more susceptible than Dreissenid mussels. Nevertheless, these results indicate that substrate preference is based more on the depth of the substrate in the water column than the texture or chemical composition of the substrate. The 2 materials with the lowest settlement (i.e., CUB and stainless steel) had the lowest average depth, while both ABS plastic and fiberglass with higher settlement had 3 of their 4 positions in the 3 highest sampling depths. Because depth is critical in determining the mussels’ settlement (see following section), this issue needs to be considered because not all materials were included at each depth ().
Table 1 Substrate sampler experimental design.
Depth
Depths from 6–28 m had significantly greater mussel settlement on both removable and permanent substrates than depths from 32–54 m. There was a 15-fold increase in the average number of mussels on the removable substrates from 10–28 m (337,000/m2) than from mussels on removable substrates from 37–54 m (21,900/m2). On the permanent substrates, there was a 19-fold increase in average number of mussels from 6–23 m (2,890,000/m2) to those from 32–50 m (155,000/m2). Lower temperatures at deeper depths can result in reduced availability of food, lowering the survivability of mussels at these depths, and can delay release and maturation of gametes (CitationWacker and von Elert 2003, CitationFrench et al. 2007). However, future research is needed to address whether temperature and/or other parameters, such as flow velocity and veliger abundance at different depths of the water column, is the main driver in determining location in the water column.
Comparison to other bodies of water
Previous research indicates that mussel settlement at different depths is dependent on the unique characteristics of individual water bodies. In lakes Erie, Michigan and Ontario, zebra mussels had previously established populations before the quagga mussel also invaded (CitationMills et al. 1993, CitationRoe and MacIsaac 1997, CitationPatterson et al. 2005, CitationFrench et al. 2007). These studies found that, in general, quagga mussel densities increased with increasing depth, and zebra mussel densities decreased with increasing depth. One explanation is that quagga mussels are more evolutionarily fit than zebra mussels and thereby more able to survive and reproduce in colder temperatures. In Lake Ontario, both quagga and zebra mussels were found living at depths from 8–110 m, but at 130 m only quagga mussels were found (CitationMills et al. 1993). Another explanation for increased quagga mussel densities with depth is that these deeper depths were not previously colonized by zebra mussels, and the quagga mussels simply settled where no other mussels currently resided.
The overall mean density from CitationMarsden and Lansky's (2000) 65 d experiment in Michigan City of Lake Michigan was about 374,000 mussels/m2, which is about twice the overall mean density of our annual removable substrates (194,000 mussels/m2). However, their data were collected from mid-August to mid-October at water depths of 3 m. The mean mussel density was 1,430,000 mussels/m2 in our September substrates at depth of 10 m (), which is higher than all the values recorded in Lake Michigan ( in Marsden and Lansky 2000). Our findings in Lake Mead are in contrast to the findings in the Great Lakes where quagga mussel density decreased with increase in depth. CitationWittmann et al. (2010) also found the highest quagga mussel densities on muddy and sandy substrates at depths from 12–42 m in Lake Mead. In a reservoir in Spain, CitationNavarro et al. (2006) found the highest densities of zebra mussels in the hypolimnion, but the biomass of the individuals was much less than mussels found in the epilimnion. This study and Wacker and von Elert (2003) both found the lowest densities of mussels in the hypolimnion and the highest densities in the epilimnion.
Effects over time
A significant increase in mussel colonization rates over time was due to multiple factors. There was heavy mussel settlement on the rope connecting the substrates to the sampler. The mussels attached to the rope may have detached and then relocated to the substrates, artificially inflating the settlement numbers. Mussels were removed from the rope before placing them back in the water during the sampling events to try and prevent this occurrence, but this cannot be overlooked.
A natural explanation for the increase in mussel settlement from March 2008 to January 2009 is the change in water quality over this time period. Lake Mead destratifies approximately one time per year (monomictic) making the temperatures within the epi- and metalimnion fluctuate throughout the year (). A Spearman correlation was conducted to determine if there were any relationships between mussel settlement throughout the year and 4 water quality characteristics: temperature (C), conductivity (μS/cm), pH and dissolved oxygen (mg/L; ). Temperature was the only characteristic that had a significant correlation with mussel settlement. The low temperatures in the hypolimnion of Lake Mead (11 C) were above the threshold for mussel survival (minimum temperature 2–5 C; Mills et al. 1996), but they may have been low enough to alter mussels’ behaviors and growth patterns.
Table 2 Non-parametric correlations between mussel settlement and water quality characteristics. Only temperature and mussel density were significantly correlated.
High mussel densities in the lower depths (28–37 m) for the 10 March 09 sampling date may be an artifact of lake stratification (). Nonetheless, the difference in mussel settlement among different depths was not significantly different (analysis of variance, p = 0.09). Therefore, the settled mussels distributed equally because the water column was mixed at this time of the year ().
Although CitationMartel et al. (1994) reported a correlation between daily settlement rates and larvae concentrations in the water, CitationSprung (1989) reported that finding correlations between larvae collected in the water column and newly settled mussels is difficult due to the high mortality rates of larvae. The daily collection of larvae could provide an advantage in finding a correlation between larvae in the water column and settled mussels due to the ability to collect larvae before the high mortality rates take effect. Unlike the Great Lakes region, quagga mussel veligers are present year round in Lake Mead. More characteristics such as size of veligers need to be monitored to better understand the relationship between veligers and their settlement behavior.
Limitations of the study
The texture and color of the substrates used in the present study, veliger density at different depths, Secchi depth, flow velocity, food quantity and quality at different seasons, and other physical, chemical and biological factors could affect the settlement of quagga mussel veligers in Lake Mead. Unfortunately, these parameters are not considered in the present study and need to be included for future research. The loss of an entire sampler in May 2008 and changes in lake level make it more difficult to accurately predict veliger settlement with different substrates, though it is clear that veligers did not like settling in deep waters (32 m or below; ).
Suggestions for lake managers and users
Based on the data from this year-long experiment, the lowest colonization rates on substrates occur during January to March, indicating that if an industry needs to place materials in Lake Mead, January to March would be the optimal time, or alternatively, whenever the lake is destratified. Note that when the lake becomes destratified, veligers will settle on substrates at depths deeper than normal because of down-welling. In concordance with CitationAckerman et al. (1996), we observed that mussels had the strongest attachment to natural surfaces (concrete), a fairly strong attachment to metallic surfaces (aluminum and stainless steel) and an even weaker attachment to polymeric surfaces (ABS and HDPE; Mueting SA, pers. observ.). HDPE plates required the least amount of work to remove mussels. Substrate choice by lake managers could have financial implications for the maintenance of equipment deployed in Lake Mead. If materials placed in the lake become colonized, it is easiest to clean materials of a polymeric nature.
Previous literature stressed the importance of a microscopic biofilm to stimulate mussel settlement (CitationMarsden 1992, CitationWainman et al. 1996, CitationKavouras and Maki 2003). Based on a visual assessment at 20× power using a stereomicroscope, a biofilm was not always present on the substrates in this experiment despite existing mussel attachment. Two months in Lake Mead may not be enough time for an appropriate biofilm to form, but mussel settlement did occur in a 2-mo period despite the absence of a biofilm on the substrate. Based on this experiment, quagga mussels in Lake Mead may not be as dependent on the presence of a biofilm as mussels in other bodies of water, but this phenomenon requires further evaluation.
Materials placed in the water below 32 m deep will have much lower colonization rates than materials placed above this depth. If an agency wanted to test different types of substrates or coatings that would not allow mussels to settle, the most efficient depths to conduct this would be from the surface to 32 m. For example, Lake Tahoe, a large and deep (about 500 m) freshwater lake between Nevada and California, is currently quagga mussel-free. If agencies are conducting early detections with artificial substrates, our data suggest that the substrates should be in depths shallower than 32 m to have a more efficient and cost-effective monitoring program. The most important finding from this study was that most mussels will settle and grow on surfaces located above a depth of 32 m.
Acknowledgments
This project was funded by the National Park Service Lake Mead National Recreation Area and the Southern Nevada Water Authority. The authors thank the park service employees who helped design, implement and complete this experiment: Wen Baldwin, Melissa Cheung, Mitch Urban, Rigby Ough, Art Gunzel, Bryan Moore, Jessie Rinella, Emily Lehman and Tom Culler; Alan Sims at SNWA for use of his microscope; and Ron Veley of USGS for the water quality data. Thanks are also due to Dr. Steve Weber and Dr. Chad Cross for review of the manuscript. We appreciate the comments from 3 anonymous reviewers that significantly improved the quality of this manuscript. Mussels kept in the laboratory were in accordance with Nevada Department of Wildlife (NDOW) possession permit #S30712. This project was approved by the NPS and awarded the study number LAME-00139 and the protocol number LAME-2008-SCI-0008.
References
- Ackerman , J D , Cottrell , C M , Ethier , C R , Allen , D G and Spelt , J K . 1996 . Attachment strength of zebra mussels on natural, polymeric, and metallic materials. . J Environ Eng. , 122 ( 2 ) : 141 – 148 .
- Czarnoleski , M , Michalczyk , L and Pajdak-Stos , A . 2004 . Substrate preference in settling zebra mussels Dreissena polymorpha. . Arch Hydrobiol. , 159 ( 2 ) : 263 – 270 .
- French , J RP , Adams , J V , Craig , J , Stickel , R G , Nichols , S J and Fleischer , G W . 2007 . Shell-free biomass and population dynamics of dreissenids in offshore Lake Michigan, 2001–2003. . J Great Lakes Res. , 33 ( 3 ) : 536 – 545 .
- Gerstenberger , S L , Mueting , S A and Wong , W H . “ Forthcoming ” . In Veligers of invasive quagga mussels (Dreissena bugensis) in Lake Mead, Nevada-Arizona Veliger
- Hosler , D M . “ Forthcoming ” . In Early Detection of dreissenid species: zebra/quagga mussels in water systems Aquat Invasions
- Kavouras , J H and Maki , J S . 2003 . Effects of biofilms on zebra mussel postveliger attachment to artificial surfaces. . Invert Biol. , 122 ( 2 ) : 138 – 151 .
- Kilgour , B and Mackie , G . 1993 . “ Colonization of different construction materials by the zebra mussel (Dreissena polymorpha). ” . In Zebra mussels: biology, impacts, and control , Edited by: Schloesser , T and Nalepa , D . 167 – 173 . Boca Raton (FL) : Lewis Publishers .
- Kobak , J . 2005 . Recruitment and small-scale spatial distribution of Dreissena polymorpha (Bivalvia) on artificial materials. . Arch Hydrobiol. , 160 ( 1 ) : 25 – 44 .
- LaBounty , J F and Burns , N M . 2005 . Characterization of boulder basin, Lake Mead, Nevada-Arizona, USA - Based on analysis of 34 limnological parameters. . Lake Reserv Manage. , 21 ( 3 ) : 277 – 307 .
- LaBounty , J F and Roefer , P . 2007 . Quagga Mussels Invade Lake Mead. . LakeLine , Fall : 17 – 22 .
- Marsden , J E . 1992 . Standard protocols for monitoring and sampling zebra mussels Illinois Natural History Survey Biological Notes 138
- Marsden , J E and Lansky , D M . 2000 . Substrate selection by settling zebra mussels, Dreissena polymorpha, relative to material, texture, orientation, and sunlight. . Can J Zool. , 78 ( 5 ) : 787 – 793 .
- Martel , A , Mathieu , A F , Findlay , C S , Nepszy , S J and Leach , J H . 1994 . Daily settlement rates of the zebra mussel, Dreissena polymorpha, on an artificial substrate correlate with veliger abundance. . Can J Fish Aquat Sci. , 51 ( 4 ) : 856 – 861 .
- May , B and Marsden , J E . 1992 . Genetic identification and implications of another invasive species of Dreissenid mussel in the Great Lakes. . Can J Fish Aquat Sci. , 49 ( 7 ) : 1501 – 1506 .
- Michigan , D NR . 2010 . Changes in Lake Huron's ecosystem and foodweb cause Chinook salmon collapse summer 2010 Available at: http://www.michigan.gov/documents/LakeHuronNewEcosystem-foodweb_122463_7.pdf. Accessed Dec 2010
- Mills , E L , Dermott , R M , Roseman , E F , Dustin , D , Mellina , E , Conn , D B and Spidle , A P . 1993 . Colonization, ecology and population structure of the quagga mussel (Bivalvia, Dreissenidae) in the lower Great Lakes. . Can J Fish Aquat Sci. , 50 ( 11 ) : 2305 – 2314 .
- Mills , E L , Rosenberg , G , Spidle , A P , Ludyanskiy , M , Pligin , Y and May , B . 1996 . A review of the biology and ecology of the quagga mussel (Dreissena bugensis), a second species of freshwater dreissenid introduced to North America. . Am Zool. , 36 ( 3 ) : 271 – 286 .
- Navarro , E , Bacardit , M , Caputo , L , Palau , T and Armengol , J . 2006 . Limnological characterization and flow patterns of a three-coupled reservoir system and their influence on Dreissena polymorpha populations and settlement during the stratification period. . Lake Reserv Manage. , 22 ( 4 ) : 293 – 302 .
- Patterson , M WR , Ciborowski , J JH and Barton , D R . 2005 . The distribution and abundance of Dreissena species (Dreissenidae) in Lake Erie, 2002. . J Great Lakes Res , 31 : 223 – 237 .
- Roe , S L and MacIsaac , H J . 1997 . Deepwater population structure and reproductive state of quagga mussels (Dreissena bugensis) in Lake Erie. . Can J Fish Aquat Sci. , 54 ( 10 ) : 2428 – 2433 .
- Sprung , M . 1989 . Field and laboratory observations of Dreissena polymorpha larvae-abundance, growth, mortality and food demands. . Arch Hydrobiol. , 115 ( 4 ) : 537 – 561 .
- Tabachnick , B G and Fidell , L S . 2007 . Using multivariate statistics , Boston , MA : Pearson .
- Wacker , A and von Elert , E . 2003 . Settlement pattern of the zebra mussel, Dreissena polymorpha, as a function of depth in Lake Constance. . Arch Hydrobiol. , 158 ( 3 ) : 289 – 301 .
- Wainman , B C , Hincks , S S , Kaushik , N K and Mackie , G L . 1996 . Biofilm and substrate preference in the dreissenid larvae of Lake Erie. . Can J Fish Aquat Sci. , 53 ( 1 ) : 134 – 140 .
- Wittmann , M E , Chandra , S , Caires , A , Denton , M , Rosen , M R , Wong , W H , Tietjen , T , Turner , K , Roefer , P and Holdren , C . 2010 . Early invasion population structure of quagga mussel and associated benthic invertebrate community composition on soft sediment in a large reservoir. . Lake Reserv Manage , 26 ( 4 )
- Yankovich , T L and Haffner , G D . 1993 . “ Habitat selectivity by the zebra mussel (Dreissena polymorpha) on artificial substrates in the Detroit River. ” . In Zebra mussels: biology, impacts, and control , Edited by: Schloesser , T and Nalepa , D . 175 – 183 . Boca Raton (FL) : Lewis Publishers .