Abstract
Limnologists recently have developed an interest in quantifying benthic resource contributions to higher-level consumers. Much of this research focuses on natural lakes with very little research in reservoirs. In this study, we provide a contemporary snapshot of the food web structure of Lake Mead to evaluate the contribution of benthic resources to fish consumers. In addition, we document the available food to fishes on soft sediments and changes to the invertebrate community over 2 time periods. Benthic invertebrate food availability for fishes is greater in Las Vegas Bay than Overton Arm. Las Vegas Bay is dominated by oligochaetes, whose biomass increased with depth, while Overton Arm is dominated by chironomids, whose biomass did not change with depth. Diet and isotopic measurements indicate the fish community largely relies on benthic resources regardless of basin (Las Vegas Bay >80%; Overton Arm >92%); however, the threadfin shad likely contribute more to largemouth and striped bass production in Overton Arm versus Las Vegas Bay. A 2-time period analysis, pre and post quagga mussel establishment and during lake level declines, suggests there is no change in the density of benthic invertebrates in Boulder Basin, but there were greater abundances of select taxa in this basin by season and depth than in other basins. Given the potential of alterations as a result of the expansion of quagga mussel and the reliance of the fishery on benthic resources, future investigation of basin specific, benthic processes is recommended.
Key words:
Ecosystem dynamics and functioning are heavily influenced by the flow of energy across traditionally defined habitat boundaries (CitationPolis et al. 2004). In lake ecosystems, multiple resources (pelagic, terrestrial and benthic) can all play a role in supporting higher-level consumers such as fishes. Research examining food web interactions in these ecosystems, however, has primarily focused on the flow of energy through the pelagic habitat and has only recently examined the flow of energy to higher trophic levels from other habitats (CitationVadeboncoeur et al. 2002, CitationVander Zanden et al. 2005). For example, after a whole-lake isotopic addition, CitationPace et al. (2004) found that consumers in small lakes can derive a substantial fraction of their carbon from terrestrial sources. In addition, benthic primary production has been shown to be a significant proportion of whole-lake primary productivity and supports fish consumers (CitationHecky and Hesslein 1995, CitationSchindler and Scheuerell 2002, CitationVander Zanden and Vadeboncoeur et al. 2002, CitationVadeboncoeur et al. 2002, Citation2003, CitationJeppesen et al. 2003, CitationVander Zanden et al. 2003).
Research from man-made limnetic ecosystems, such as reservoirs and impoundments, suggests plankton and terrestrially derived matter from river inflow, as well as pelagic primary production, are the dominant sources of energy in these ecosystems (CitationKimmel et al. 1990). The role of benthic production in these ecosystems is generally not as well studied and considered to be less important than pelagic production. Fishes in reservoirs, however, while typically composed of those adapted to lentic rather than lotic conditions (CitationFernando and Holčík 1991), may feed on benthic resources even though it has been traditionally thought this habitat may not contribute substantially to whole-lake production.
Lake Mead is a large reservoir in the southwestern United States. Most limnological information focuses on understanding water quality dynamics in the pelagic habitat with no contemporary published information on game fish ecology and the status of benthic food resources. The lake has 11 known game fish species and is home to one endangered species, the razorback sucker (Xyrauchen texanus), a benthic-oriented taxa. The lake's top predators largemouth bass (Micropterus salmoides) and striped bass (Morone saxatilis), have received some attention because they are an important economic resource for the region (CitationMartin et al. 1982). Previous work on piscivorous fishes, striped bass and rainbow trout (Oncorhynchus mykiss), suggests their energy is obtained through the consumption of threadfin shad as well as benthic invertebrates (chironomids and crayfish) during the spring months (CitationWilde and Paulson 1989).
Recently there has been a need to understand lake trophic interactions since the lake has had a series of perturbations that may impact the fishery and consumption of fishes. For example, the introductions of invasive invertebrates, such as the Asian clam (Corbicula fluminea) and quagga mussel (Dreissena bugensis), have resulted in the need to describe the basic ecology of the basins prior to potential future food web alterations. Moreover, contaminant loading in Las Vegas Bay, a portion of Boulder Basin, may impact the endangered razorback sucker, a lake-bottom feeder (CitationHubbs and Miller 1953, CitationJonez and Summer 1954, CitationAllan and Roden 1978). In this study we provide an ecological snapshot of 2 lake regions of varying trophic structure under declining lake levels and before the major establishment of the quagga mussel. Specifically, we (Equation1) document biomass of benthic primary consumers available as a food resource from soft sediments and (Equation2) determine the food web structure of 2 lake regions to understand species-specific, habitat reliance of fishes prior to the expansion of quagga mussel. In addition we (3) determine changes in benthic invertebrate density in soft sediments before and after the initial establishment of quagga mussels from 3 regions and 2 seasons to understand if mussels are changing food availability. Thus, the focus of the paper was to determine how much game fish rely on benthic rather than pelagic resources and determine potential changes in the abundance of invertebrate taxa before and after the initial invasion by quagga mussel. This information is critical for lake managers seeking to sustainably manage the lake's fishery during a time of large changes due to declines in lake level and the recent introduction of invasive mussels and fishes, as well as the continuing introduction of urban runoff and wastewater effluent to the lake.
Materials and methods
Study area
Lake Mead is the largest reservoir in the United States and a major water supply for the southwestern part of the country. Previously published literature classifies Lake Mead as mesotrophic (CitationCarlson 1977, CitationLaBounty and Horn 1997); however, recent research has classified multiple basins in Lake Mead as oligotrophic (CitationHoldren et al. 2010). CitationLaBounty and Burns (2005) found differences in classification of Boulder Basin similar to the findings of Holden et al. 2010) that suggests outer Bolder Basin is oligotrophic while inner Bolder Basin (Las Vegas Bay) is mesotrophic. An overall lake classification is difficult due to the 5 basins that make up Lake Mead, the inflow that is associated with each basin and the influence of hydrological processes by dam operations at different times of the year (CitationThornton 1990). The mean annual elevation of Lake Mead during 2007 was 340.5 m a.s.l., which was significantly lower than the level in 1986 of 367.8 m a.s.l. (BOR 2009).
Objective 1: Documentation of benthic invertebrate food availability, and Objective 2: Food web structure between basins of varying nutrient status
Due to physical and ecological differences between lake basins (CitationLaBounty and Horn 1997) and to overlap our sampling with agency (US Fish and Wildlife Service and US Geological Survey) fish collection programs, we chose 2 basins for comparison of fish composition, diet, and available benthic invertebrate food resources. The 2 basins are (Equation1) Las Vegas Bay, a eutrophic basin whose major drainage is from the Las Vegas Valley, which delivers tertiary-treated municipal wastewater effluent along with groundwater, urban runoff, and other point source discharges, and (Equation2) Overton Arm, which does not experience tertiary-treated municipal wastewater and is more oligotrophic than Las Vegas Bay (). Sampling for fishes and benthic biomass available to these fishes occurred over a 1 week period in Las Vegas Bay in March 2007 and November 2008 and in Overton Arm in November 2007. Unfortunately, Overton Arm invertebrates collected in March 2008 were incorrectly preserved and not included in the analysis.
Figure 1 Lake Mead sampling locations, March 2007–March 2008 for zooplankton (black circles), fish electro-shocking routes (solid black lines), and benthic invertebrates (every 5 m along the dashed lines) in Las Vegas Bay and Overton Arm. Stars indicate locations for comparisons between historical (1980s) and contemporary (2007–2008) benthic invertebrate sampling locations to determine potential alterations after quagga establishment. (Base map from US Geological Survey digital data. 1:100,000 scale. 1987: Universal Transverse Mercator Projection, Zone 11).
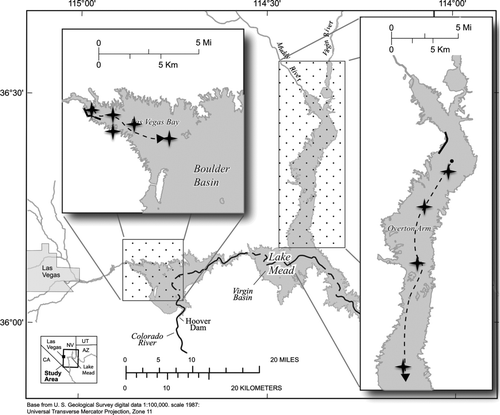
To estimate benthic invertebrate taxonomic composition and food available in soft sediment for each basin, we collected samples in spring and fall (Mar 2007, 2008 and Nov 2007, respectively). Using 3–5 Petite Ponar grabs at approximately 5 m depth intervals collected laterally from the primary inflow (Las Vegas Wash in Las Vegas Bay and the Muddy and Virgin rivers in Overton Arm) to a depth of 30 m (). GPS points were taken during each sampling period; however, depth was the primary parameter for sampling location and comparison due to the declining lake levels between sampling periods. Samples were retained on a 500 μm mesh screen and stored in 70% ethanol for analysis. Preserved invertebrate samples were identified to the lowest taxonomic level possible at the University of Nevada-Reno's Aquatic Ecosystems Analysis Laboratory (usually to family or genus), oven dried, and measured as dry weight per square meter. Potential benthic food availability for fish was estimated by calculating biomass per square meter of all benthic invertebrates in each sampling basin across multiple depths from 3 to 30 m at 5 m intervals.
To assess the food web structure in each basin, we measured stable isotopes of carbon (δ13C) and nitrogen (δ15N) from fish tissue, as well as from pelagic and benthic primary consumers. Unlike diet data, which can represent diet selectivity but require a number of sampling periods over time to create and accurate assessment of fish energetics, natural abundance isotopic measurements are commonly used to determine temporally integrated resource utilization to a consumer (CitationVander Zanden and Rasmussen 1999). Carbon isotopes were used to differentiate habitat sources (benthic or pelagic) because there is little discrimination as you move toward predators in the food web, allowing researchers to determine the extent of bottom- or open-water energy utilization (CitationVander Zanden and Rasmussen 1999). Nitrogen isotopes infer trophic position or feeding level in the food web (CitationMinagawa and Wada 1984). Dorsal muscle tissue and gut contents were sampled from 7 different fish species; however, not all muscle tissues from each sample were utilized for stable isotope analysis (). Instead, a subset of samples was analyzed due to cost constraints. Fish were collected through coordination with US Geological Survey and US Fish and Wildlife Service biologists using an electroshocking boat at the mouth of the Las Vegas Wash and near the boat launch at Overton Beach. Fish were immediately processed on the shore with the US Geological Survey and US Fish and Wildlife Service. Standard length, weight, sex and stomach contents (total proportional dry weight of individual invertebrates by family) were obtained for each fish. Because fish analyzed for isotopes were collected from shallow waters, we assumed they moved to deeper waters during the year, integrating isotopic values from deeper depths during nonsampling periods and other seasons. When possible, 10 fish from each location, sampling period and size class (small, medium and large, determined from length and weight measurements) were analyzed for stable isotopes.
Table 1 Number of fish per species collected in each location (Las Vegas Bay [LVB] and Overton Arm [OVA]) and total number of fish caught over the entire study. Please note no sampling occurred in OVA in March 2007.
Pelagic isotopic signatures were determined from zooplankton. Zooplankton were obtained using a vertical tow from the 2 basins (), and live zooplankton from each of the dominant species in Lake Mead (Cyclops bicuspidatus, Cyclops vernalis, and Daphnia spp.) were pooled for stable isotope analysis. Benthic invertebrate primary consumers (Oligocheatae and Chironomidae) up to 30 m deep were used to identify the benthic resource isotopic signal. These were collected during benthic invertebrate surveys conducted to determine food availability and were pooled to determine basin specific isotope signals.
All tissue and invertebrate samples were dried at 70 C for 24 h and ground to fine powder using a mortar and pestle. Samples were packed into tin capsules (8 × 5 mm), and carbon and nitrogen were analyzed using a continuous flow isotope ratio mass spectrometer (IRMS, 20–20, PDZ, Europa Science, Sandbach, UK). Samples were combusted to CO2 and N2 occurring at 1000 C in an inline elemental analyzer (PDZEurope Science, ANCA-GSL). A Carbosieve G column (Supelco, Bellefonte, PA, USA) separated the gas before introduction to the IRMS. Standard gases (Pee Dee Belemnite for δ13C and atmospheric N2 gas for δ15N) were injected directly into the IRMS before and after the sample peaks. Isotopic ratios were expressed as a per mil (%) deviation from the standards as defined by the following equations:
Pelagic organisms tend to be isotopically depleted in 13C relative to benthic organisms, and δ13C values tend to be conserved from prey to predator. Thus, we used a simple, 2-source δ13C mixing model to estimate the fractional reliance of a fish population from each basin on pelagic or benthic production using the equation (CitationVander Zanden et al. 1999, 2006)
Objective 3: Comparison of benthic invertebrate density before and after initial quagga mussel establishment
To evaluate the potential impact of quagga mussel to benthic invertebrate abundances, we compared seasonal (late spring/early summer and late summer) densities of benthic invertebrate taxa from 3 different basins (Las Vegas Bay, Boulder Basin, and Overton Arm). Due to the decline in lake levels between 1986 and the current sampling, point to point depth sampling along similar transects were conducted from the historical study conducted in the mid-1980s (Peck et al. 1989). Locations were determined from a database of Lake Mead's only known previous collections, held by Dr. Craig Palmer, University of Nevada-Las Vegas’ Harry Reid Center. Contemporary collections at each point were made during a 1 week period in October 2008 and March 2009 using similar field methods from soft sediments used to determine benthic food available to fishes (see Methods Objectives 1 and 2). Quagga mussels were included in the total invertebrate density calculations. A total of 57 samples from historical locations across 3 basins were comparable to the 223 collections made from the contemporary assessment () after they were pooled for physical habitat from each basin (epilimnion 0–20 m, metalimnion 20–40 m, and hypolimnion 40 m to maximum depth). A one-way ANOVA using Tukey's HSD test was used to compare the mean density of invertebrates collected during the 2 different time periods. Comparisons were made for total invertebrate density and the dominant invertebrates in each sample that included oligochaeta, chironomidae and Corbicula.
Table 2 Comparison of historical (1980s) and contemporary (2008–2009) benthic invertebrate densities by season (October 2008 to late summer, and March 2009 to late spring/early summer) after quagga mussel establishment. A one-way ANOVA using a Tukey's HSD test was used to compare mean densities of invertebrates collected during the 2 time periods.
Results
Objective 1: Primary consumers composition and biomass: potential food available for fish
Oligochaeta and chironomidae dominated the soft sediment benthic assemblage during the collections to determine the food availability for fishes ( and 2b), with no quagga mussel found in benthic collections or stomach samples in 2007 (see Results Objective 2). Combined benthic invertebrate biomass was highest in Las Vegas Bay in November 2007 (649.1 mg dw/m2) in the deepest depth sampled (34 m), with oligochaetes contributing 636.9 mg dw/m2 (98.1%). Chironomidae biomass was greatest in Las Vegas Bay in March 2007, measuring 25.2 mg dw/m2. Chironomidae had the highest biomass in Overton Arm at 123.4 mg dw/m2, while oligochaetes had a maximum biomass of 98.5 mg dw/m2. Oligochaetes displayed a distinct increase with depth, to 300+ mg dw/m2 at 34 m, in Las Vegas Bay in November.
Objective 2: Food web structure and habitat contribution to fish diets
Seven fish species (n = 423) were collected (64% in Las Vegas Bay; 35% in Overton Arm) in 2007 and 2008 () and analyzed for diet by stomach contents with a subset analyzed for stable isotope analysis. No gizzard shad (Dorsoma cepedianum) were caught during our study. Diet composition in stomach contents varied considerably among species and between the 2 basins (). For example, largemouth bass in Las Vegas Bay consumed bluegill and carp (86%) but in Overton Arm relied heavily on crayfish (Procambarus clarkii; 62%). Bluegill (n = 43) consumed the largest overall diversity of benthic organisms (chironomidae, odonata, trichoptera, and hemiptera), with chironomidae as the dominant food source in both basins (60% in Las Vegas Bay; 83% in Overton Arm). Algae were the most abundant food items (27%) in carp stomach. Due to low numbers of striped bass collected in both Las Vegas Bay and Overton Arm (n = 28), stomach contents were combined and consisted of 56% unidentifiable fish and 36% unknown benthic invertebrates.
Figure 3 Diet analysis (percentage of dry weight) for bluegill, carp, and largemouth bass in Las Vegas Bay and Overton Arm collected in 2007 and 2008.
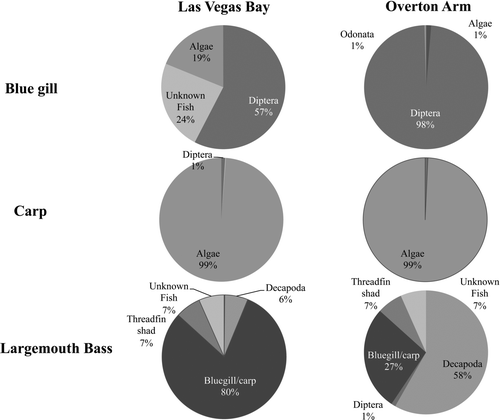
Mean (±SE) δ13C and δ15N for zooplankton, benthic invertebrates and 7 fishes were analyzed (). Because isotopic nitrogen can increase with dietary ontogenetic shifts, we analyzed the size of largemouth bass to determine if we should divide the fishes into different size classes for our analysis. There was no statistical difference between largemouth bass size between the 2 basins in 2007 and 2008 (2007 p = 0.29; 2008 p = 0.15; Overton Arm 2007–2008 p = 0.29; Las Vegas Bay 2007–2008 p = 0.15); thus, largemouth and striped bass were pooled for each species and each basin for this analysis to determine top predator feeding behavior. Pelagic and littoral benthic primary consumer δ13C signals were different within each basin (approximately −29% and −19% for Las Vegas Bay and approximately −29% and −21% for Overton Arm, respectively) and therefore, used as end-member values for our 2-source mixing model to calculate benthic reliance by fish ( and 4b; ). Analysis of δ15N revealed similar trophic positions for predatory fishes between the 2 basins ( and 4b). Largemouth bass and striped bass occupied the highest trophic positions, with benthic invertebrates occupying the lowest trophic positions ().
Figure 4 Stable carbon and nitrogen isotope values (mean ± SE) for biota in a) Las Vegas Bay and b) Overton Arm. B = bluegill, C = carp; CC = channel catfish; GS = green sunfish, LB = largemouth bass, SB = striped bass, TS = threadfin shad, Z = zooplankton, BI = benethic invertebrate. Dotted lines represent the pelagic and littoral-benthic end-members for each basin.
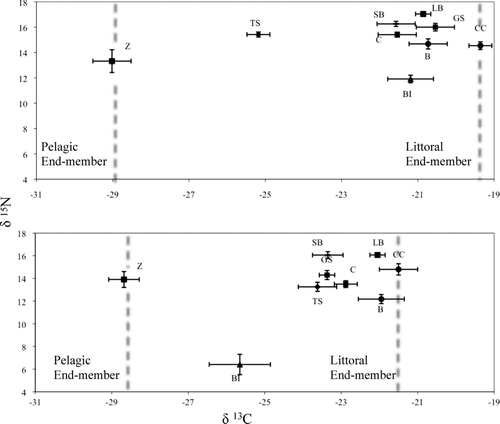
Table 3 Stable carbon and nitrogen isotope values of zooplankton, benthic invertebrates and fish from Las Vegas Bay and Overton Arm basins collected in 2007–2008. The taxa reliance on benthic carbon resources was determined from a 2 end-member mixing model.
For all species, mean reliance of the fishery on benthic resources () was slightly greater in Overton Arm (84.1%) than Las Vegas Bay (77.7%). Channel catfish relied almost exclusively on the benthic zone in both basins (98.4% Las Vegas Bay and 100.0% Overton Arm). Bluegill relied on the benthic habitat less in Overton Arm (84.5%) than in Las Vegas Bay (94.4%). Carp stomach contents suggested algal dominated their diet, while time-integrated isotopic data also indicated their reliance on benthic habitats in both basins (76.2% Las Vegas Bay and 81.3% Overton Arm). Green sunfish had greater benthic reliance in Las Vegas Bay (86.4%) than in Overton Arm (74.5%). Largemouth bass had greater benthic reliance in Overton Arm (93.0%) than in Las Vegas Bay (83.2%). Striped bass reliance on benthic sources in Las Vegas Bay was 75.8% versus 71.0% in Overton Arm. Threadfin shad had the lowest and most divergent estimates of benthic reliance of all the fishes between basins. In Las Vegas Bay, for example, their benthic reliance was only 39.1% compared with 71.0% in Overton Arm.
Objective 3: Comparison of benthic invertebrate density before and after initial quagga mussel establishment
An analysis of historical versus contemporary invertebrate densities indicates basin and depth (epilimnion, metalimnion and hypolimnion) specific differences during certain time periods (late spring/early summer and late summer). In Boulder basin, for example, there were no significant differences between historical and contemporary total invertebrate densities (). At depths between 0 and 20 m, however, historical chironomid and Corbicula densities were greater than contemporary densities during late summer ( and 5d). Historical Corbicula densities were also were greater than contemporary densities at depths between 21 and 40 m (). At depths >41 m in Boulder Basin, both oligochaeta and chironomidae densities were historically greater during late spring and early summer ( and 5c).
Figure 5 Comparison of historical (1980's) and contemporary (2007–2008) benthic invertebrate densities in three basins by depth (0–20, 21–40, >40 m) where a) is a comparison of total invertebrate density b) oligochaeta density c) chironomidae density and d) Corbicula density. A one-way ANOVA using a Tukey's HSD test was used to compare mean densities between time periods in each basin. Asterisks denote significant differences in mean values. Please note difference in y-axis for chironomidea and Corbicula.
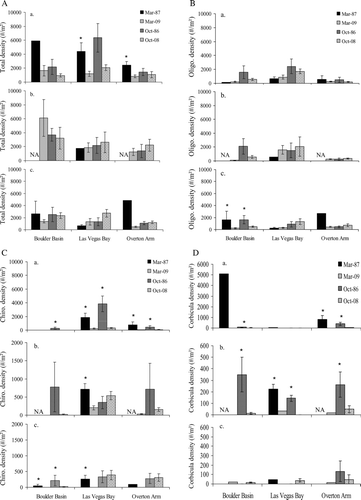
In Las Vegas Bay, chironomid densities were historically greater than contemporary densities during late spring at all depths sampled (). In addition, between 21 and 40 m, Corbicula densities were historically higher during both sampling periods (). Invertebrate densities also varied by season and depth in Overton Arm, where the total density of invertebrates was historically higher during late spring between 0 and 20 m (). At these same depths historical densities of chironomidae and Corbicula were higher during both sampling periods ( and 5d). However, at depths between 21 and 40 m only Corbicula densities in October were historically higher than contemporary densities (). In depths >41 m, there were no significant differences observed between historic and contemporary invertebrate densities in Overton Arm.
Discussion
Lake Mead's soft sediment benthic invertebrate communities collected prior to quagga mussel establishment indicates the basins are dominated by oligochaete and chironomidae, similar to other reservoirs (CitationGladden and Smock 1990, CitationLeslie et al. 1997). Benthic invertebrate biomasses in both basins differed between depths and basins (; Tables and 2b). In Las Vegas Bay, benthic invertebrates were dominated by oligochaetes in both March and November. Overall biomass was much higher in November and increased with depth to 34 m. In Overton Arm, overall benthic biomass in November (the only samples available) was lower than Las Vegas Bay and dominated by chironomidae at all but the deepest sampled depth (34 m). Thus, there is variable biomass of food available to fishes in Overton Arm in November with little biomass available for fishes at the intermediate depths (7, 16 and 20 m). Unfortunately, no environmental parameters (e.g., nutrients, sediment organic concentrations) were obtained from the benthic habitats of these basins to determine the mechanisms that may lead to differential biomass between the basins.
The differences in biomass at different depths and locations, however, could be attributed to the patchy nature of invertebrate abundance in lacustrine ecosystems (CitationStoffels et al. 2005). Previous research from other lacustrine ecosystems suggests algal biomass, habitat structure and temperature at different depths govern the distribution and composition of benthic invertebrates (CitationMoore 1981a, Citation1981b, CitationWeatherhead and James 2001, CitationDetwiler et al. 2002). Other studies have also suggested that fish may distribute in the nearshore where invertebrate biomass is greater (CitationKeast and Harker 1977). In general, eutrophic ecosystems have greater but variable amounts of benthic invertebrate productivity (CitationNorthington et al. 2010); thus, the higher production in Las Vegas Bay should yield greater invertebrate biomass compared with Overton Arm. The quality of algae and sediment combined with effluent coming from Las Vegas Wash may be controlling biomass of invertebrates in the Bay. Moreover, we only measured biomass in this study and do not quantify production or turnover rates for each taxa, which may be greater in Las Vegas Bay due to its more productive nature. Thus, a cautionary interpretation should be taken when trying to understand the drivers behind decreased biomass and availability to fishes, with future studies focusing on production rather than standing stock of inverebrates.
Similar to previous studies from natural lakes that highlight the role of benthic habitats in the flow of energy to higher trophic levels, our snapshot of diet and isotopic measurements indicates the fish communities from both basins rely on benthic more than pelagic habitats for their energy, regardless of their nutrient structure (CitationHecky and Hesslein 1995, CitationSchindler and Scheuerell 2002, CitationVander Zanden and Vadeboncoeur 2002, CitationVander Zanden et al. 2006). Unfortunately, there have been no previously published studies on the dietary habits of the fish community from different basins in Lake Mead. CitationWilde and Paulson (1989), however, did find that one of the lake's top predatory game fish, striped bass, rely to some degree on chironomid larvae and crayfish. In their study, threadfin shad were thought to be an important link to higher-level consumers during summer months with some utilization in spring and fall periods. This would suggest that to understand and predict game fish production, a focused study on threadfin shad should be conducted at the lake.
If we evaluate basin-specific food web structure as conducted in the current study, the top predatory fishes (largemouth and striped bass) receive their energy through the consumption of threadfin shad to a greater extent in Overton Arm than in Las Vegas Bay, where bluegill or shiners support their energetic needs. Our diet and isotopic analysis confirm a heavy reliance for these game fishes on the benthic resources, specifically chironomids in the spring and fall, while only a small proportion of their diets consist of prey fish such as threadfin shad. Thus, basin-specific studies are likely necessary if managers are interested in linking intermediate consumers such as threadfin shad or other forage fish to game fish production. In comparison, carp, a more cosmopolitan benthic feeder, seems to consistently feed on benthic primary consumers and algae in both basins. Please note, this is only a one-time snapshot of the dietary behavior and energetics of the fish community in the basin. Future monitoring should be conducted to determine the intra- and interannual variability of fish energetics and body condition over time, especially given the recent establishment and spread of quagga mussel in the lake.
While there is limited information available on the reliance of benthic fishes from manmade reservoir ecosystems, CitationJohnson et al. (2002) utilized isotoptic collections to determine the food web structure of 2 reservoirs in Colorado. The game fish in one reservoir were largely supported from resources from the bottom of the lake; however, the addition of one nonnative invertebrate species greatly altered the structure of a similar reservoir whose top predators fed mostly in the pelagic zone. Thus, the addition of a single species can create competitive interactions at similar trophic levels in the food web to the determinant of other taxa, resulting in a shift in the flow of energy from different habitats (CitationChess and Stanford 1998, CitationJassby 1998). Thus, understanding how quagga mussel may alter the future benthic productivity in each basin will likely be important if trying to predict fisheries production.
Recent research suggests that quagga mussels can alter benthic invertebrate communities abundance and diversity (CitationWard and Ricciardi 2007) and alter food-web production pathways and energetics by means of physical and biological changes throughout lake ecosystems (CitationLodge et al. 1994, MacIsaac 1996, CitationKaratayev et al. 2002). Our analysis suggests that there has been a large change in the densities of select invertebrates over time from Lake Mead, but these alterations may be basin- and seasonally specific. For example, it does not appear that the overall densities of invertebrates in Boulder Basin have shifted since historical collections were made; however, other basins have had greater densities during the early spring. Furthermore, historically the samples collected from the lake contained greater numbers of certain taxa including larger numbers of Corbicula and, depending on the season, oligochaetes and chironomids. Thus, while today there seems to be a decline in benthic invertebrates in select basins and depth zones, the reason for this decline is not clear. Even though quagga mussels are in the early establishment phase and may impact the density and diversity of invertebrates, the lake level has also declined dramatically over this time period. We believe it is too early to assess from the data collected in this study whether mussels are directly impacting native densities. Future studies should focus on understanding the interannual aspects of the invasions dynamics on soft sediments and the role they may play in shifting invertebrate biomass. Moreover, the quality of food available as a result of shifts from soft-bodied invertebrates to mussel should be considered and tied into the body condition of fishes in each basin.
In conclusion, this is the first contemporary snapshot of Lake Mead's food web structure before the widespread establishment of quagga mussel and assessment of the benthic invertebrate community on soft sediments over time. Our findings indicate a strong reliance of the fish community on benthic resources with intermediate consumers (e.g., threadfin shad) playing a variable role in game fish production, depending on the basin. To understand future changes to the fishery due to disturbances such as declining water levels, contaminant loading or invasive species spread, we recommend a benthic monitoring and assessment program be initiated at the basin level and across multiple years.
Acknowledgments
The project was supported by funds generated from the Southern Nevada Public Lands Management Act to the National Park Service (Cooperative Agreement Number 08WRAR0007- US Geological Survey and the Great Basin Institute) and a second award to S. Chandra. We thank Jorge Arufe and Chuck Fisher (US Geological Survey) as well as Annie Caires, Marianne Denton, Jason Barnes, and undergraduate students from the Aquatic Ecosystems Analysis Laboratory at the University of Nevada-Reno for field collections and identifications. We would like to give a special thank you to Steven Goodbred for development of this project and collection of fish samples. Carla Weiser and Reynaldo Patiño assisted with field collection and provided fish samples. Conversations with the Interagency Quagga Mussel working group were very helpful, especially those with Todd Tietjen and Peggy Roefer (Southern Nevada Water Authority), Kent Turner (National Park Service), and Chris Holdren (USBR). Reviews of earlier versions of the manuscript by USGS reviewers Steve Lawrence and Lari Knochemnus, and by Michael Parker (Southern Oregon University) greatly improved the manuscript.
References
- Allan , C R and Roden , L D . 1978 . “ Fish of Lake Mead and Lake Mohave ” . In Nevada Department of Wildlife. Base Fisheries Data Boulder Canyon to Davis Dam Biological Bulletin No. 7.
- [BOR] Bureau of Reclamation . 2009 . Lake Mead database http://www.hprcc.unl.edu/nebraska/lake-mead-all-data.html. Accessed 15 March 2009
- Carlson , R E . 1977 . A trophic state index for lakes. . Limnol Oceanogr , 22 : 361 – 369 .
- Chess , D W and Stanford , J A . 1998 . Comparative energetics and life cycle of the opossum shrimp (Mysis relicta) in native and non-native environments. . Freshwater Biol , 40 : 783 – 794 .
- Detwiler , P M , Coe , M F and Dexter , D M . 2002 . The benthic invertebrates of the Salton Sea: distribution and seasonal dynamics . Hydrobiologia , 473 : 1573 – 5117 .
- Fernando , C H and Holčík , J. 1991 . Fish in reservoirs . Int Rev Ges Hydrobiol , 76 : 149 – 167 .
- Gladden , J E and Smock , L A . 1990 . Macro invertebrate distribution and production on the floodplains of two lowland headwater streams. . Freshwarer Biol , 24 : 533 – 545 .
- Hecky , R E and Hesslein , R H . 1995 . Contributions of benthic algae to lake food webs as revealed by stable isotope analysis. . J North Am Benthol Soc , 14 : 631 – 653 .
- Holdren , G C and Turner , K . 2010 . Characteristics of Lake Mead, Arizona–Nevada. . Lake Reserv Manage , 26 : 229
- Hubbs , C L and Miller , R R . 1953 . Hybridization in nature between the fish genera Catostomus and Xyrauchen . Pap. Michigan Acadamy of Science, Arts, and Letters , 38 : 207 – 233 .
- Jassby , A D . 1998 . Interannual variability at three inland water sites: implications for sentinel ecosystems. . Ecol Appl , 8 : 277 – 287 .
- Jeppesen , E , Jensen , J P , Jensen , C , Faafeng , B , Hessen , D O , Sondergaard , M , Lauridsen , T L , Brettum , P and Christoffersen , K . 2003 . The impact of nutrient state and lake depth on top down control in the pelagic zone of lakes: a study of 466 lakes from the temperature zone to the Arctic. . Ecosystems , 6 : 313 – 325 .
- Johnson , B M , Martinez , P J and Stockwell , J D . 2002 . Tracking trophic interactions in coldwater reservoirs using naturally occurring stable isotopes. . Trans Am Fish Soc , 131 : 1 – 13 .
- Jonez , A and Summer , R C . 1954 . Lake Mead and Mohave investigations , Nevada Fish and Game Commission . Final Report. D-J Project F-1-R, 186
- Karatayev , A Y , Burlakova , L E , Molly , D P , Volkova , L K and Volosyuk , V V . 2002 . Field and laboratory studies of Ophryoglena sp. (Cilitata: Ophryoglenidae) infection in zebra mussels, Dreissena polymorpha (Bivalvia: Dreissenidae). . J Invertebr Pathol , 79 : 80 – 85 .
- Keast , A and Harker , J . 1977 . Fish distribution and benthic invertebrate biomass relative to depth in an Ontario Lake. . Environ Biol Fish , 2 : 235 – 240 .
- Kimmel , B L , Lind , O T and Paulsen , L J . 1990 . “ Chapter 6: Reservoir primary production. ” . In Reservoir limnology: ecological perspectives , Edited by: Thornton , K W , Kimmel , B L and Payne , F E . NewYork , NY : John & Wiley Sons, Inc .
- LaBounty , J F and Burns , N M . 2005 . Characterization of Boulder Basin, Lake Mead, Nevada-Arizona, USA- based on analysis of 34 limnological parameters. . Lake Reserv Manage. , 21 : 277 – 307 .
- LaBounty , J F and Horn , M J . 1997 . The influence of drainage from the Las Vegas Valley on the limnological of Boulder Basin, Lake Mead, Arizona-Nevada. . Lake Reserv Manage , 13 : 95 – 108 .
- Leslie , A J , Thomas , L C , Joseph , P P and Ewel , K C . 1997 . Benthic macro invertebrates of small Florida pond-cypress swamps and the influence of dry periods. . Wetlands , 17 : 447 – 455 .
- Lodge , D M , Kershner , M W , Aloi , J E and Covich , A P . 1994 . Ecology , 55 : 1265 – 1281 .
- Mac Isaac , J H . 1996 . Potential abiotic and biotic impacts of Zebra Mussels on the inland waters of North . Am Zool , 36 : 287 – 299 .
- Martin , W E , Bollman , F H and Gum , R L . 1982 . Economic value of the Lake Mead fishery. . Fisheries , 7 : 20 – 24 .
- Minagawa , M and Wada , E . 1984 . Stepwise enrichment of N15 along food-chains - further evidence and the relation between delta-n-15 and animal age. . Geoch Cosmochim Acta , 48 : 1135 – 1140 .
- Moore , J W . 1981a . Inter-species variability in the consumption of algae by Oligochaetes . Hydrobiologia , 83 : 241 – 244 .
- Moore , J W . 1981b . Factors influencing the species composition, distribution and abundance of benthic invertebrates in the profundal zone of a eutrophic northern lake . Hydrobiologia , 83 : 1573 – 5117 .
- Northington , R M , Keyse , M D , Beaty , S R , Whalen , S C , Sokol , E R and Hershey , A E . 2010 . Benthic secondary production in eight oligotrophic arctic Alaskan lakes. . J North Am Benthol Soc , 29 : 465 – 479 .
- Pace , M L , Cole , J J , Carpenter , S R , Kitchell , J F , Hodgson , J R , Van de Bogert , M C , Bade , D L , Kritzberg , E S and Bastviken , D . 2004 . Whole-lake carbon-13 additions reveal terrestrial support of aquatic food webs. . Nature , 427 : 240 – 243 .
- Peck , S K , Pratt , W L , Pollard , J E , Paulson , L J and Baepler , D H . 1987 . Benthic invertebrates and crayfish of Lake Mead , Las Vegas (NV) : University of Nevada-Las Vegas, Environmental Research Center . Final Report
- Polis , G A , Power , M E and Huxel , G R . 2004 . Food webs at the landscape level , Chicago(IL) : University of Chicago Press .
- Schindler , D E and Scheuerell , M D . 2002 . Habitat coupling in lake ecosystems. . Oikos , 98 : 177 – 189 .
- Stoffels , R J , Clarke , K R and Closs , G P . 2005 . Spatial scale and benthic community organization in the littoral zones of large oligotrophic lakes: potential for cross-scale interactions. . Freshwater Biol , 50 : 1131 – 1145 .
- Thornton , K W . 1990 . “ Perspectives on reservoir limnology. ” . In Reservoir Limnology: Ecology Perspectives , Edited by: Thornton , K W , Kimmel , B L and Payne , F F . 1 – 14 . New York (NY) : John Wiley and Sons .
- Vadeboncoeur , Y , Jeppesen , E , Vander Zanden , M J , Schierup , H H , Christoffersen , K and Lodge , D M . 2003 . From Greenland to green lakes: cultural eutrophication and the loss of benthic energy pathways in lakes. . Limnol Oceanogr , 48 : 1408 – 1418 .
- Vadeboncoeur , Y , Vander Zanden , M J and Lodge , D M . 2002 . Putting the lake back together: Reintegrating benthic pathways into lake food web models. . Bioscience , 52 : 44 – 54 .
- Vander Zanden , M J , Chandra , S , Allen , B C , Reuter , J E and Goldman , C R . 2003 . Historical food web structure and restoration of native fish communities in Lake Tahoe (CA-NV) basin. . Ecosystems , 3 : 274 – 288 .
- Vander Zanden , M J , Chandra , C , Park , S , Vadeboncoeur , Y and Goldman , C R . 2006 . Efficiencies of benthic and pelagic trophic pathways in a subalpine lake. . Can J Fish Aquat Sci , 63 : 2608 – 2620 .
- Vander Zanden , M , Essington , T E and Vadeboncoeur , Y . 2005 . Is pelagic top-down control in lakes augmented by benthic energy pathways? . Can J Fish Aquat Sci , 62 : 1422 – 1431 .
- Vander Zanden , M J , Lester , N P , Shuter , B J and Rasmussen , J B . 1999 . Application of stable isotope techniques to studies of age-0 smallmouth bass. . Trans Am Fish Soc , 127 : 729 – 739 .
- Vander Zanden , M J and Rasmussen , J B . 1999 . Primary consumer delta13C and delta15N and the trophic position of aquatic consumers. . Ecology , 80 : 1395 – 1404 .
- Vander Zanden , M J and Vadeboncoeur , Y . 2002 . Fishes as integrators of benthic and pelagic food webs in lakes. . Ecology , 83 : 2152 – 2161 .
- Ward , J M and Ricciardi , A . 2007 . Impacts of Dreissena invasions on benthic marcroinvertebrate communities: a meta-analysis. . Divers Distrib , 13 : 155 – 165 .
- Weatherhead , M A and James , M R . 2001 . Distribution of macroinvertebrates in relation to physical and biological variables in the littoral zone of nine New Zealand lakes. . Hydrobiologia , 462 : 115 – 129 .
- Wilde , G R and Paulson , L J . 1989 . Food habits of subadult striped bass in Lake Mead, Arizona-Nevada. . Southwest Nat , 34 : 118 – 123 .