Abstract
Liuxihe Reservoir (23°45′50″N; 113°46′52″E) is a valley-type subtropical reservoir in south China. The reservoir behaves as a warm monomictic water body that is stratified throughout the year except for a short mixing period in winter. During stratification, vertical circulation was very weak and the hypolimnion was anoxic. The metalimnion range and thermocline location were affected significantly by the outlet height. Spatial and temporal variations in turbidity indicate that suspended matter was predominantly clay minerals with low organic contents. The behavior of turbid currents entering the reservoir was determined by density differences between inflow and impounded water. Because of particle size, shape of suspended matter, and weak vertical circulation, the suspended matter persisted in the water column for months. The water clarity of the lacustrine zone was affected significantly by water level variation, which is considered a comprehensive effect of stratification, resuspension, landforms, and hydrologic condition of the reservoir. Management recommendations on withdrawal selection and water level regulation are proposed to improve water quality.
Limnological characterization is a fundamental tool for designing conservation and management policies for lakes and reservoirs (CitationPaez et al. 2001). In addition to chemical and biological factors, some physical factors also affect water quality. Studies have found that hydrodynamics play an important role in nutrient flow, related ecological processes, and water quality (CitationAlavian et al. 1992, CitationAn and Jones 2000, de CitationCesare et al. 2001, CitationChen and Wu 2003, CitationRomergo and Imberger 2003, CitationChen et al. 2006), and thermal stratification alters velocity profiles and hinders oxygenation in the hypolimnion, thus affecting reservoir water quality (CitationElci 2008). In addition, turbid water with suspended sediment is known to influence physical, chemical, and biological processes in lakes and reservoirs (CitationLind et al. 1997, CitationFaithful and Griffiths 2000, CitationDoyle and Smart 2001, CitationEffler et al. 2006, CitationMcCullough et al. 2007, CitationCheng and Kao 2008). Turbid water has a large capacity to adsorb nutrients because of large surface-to-area ratios and charged surface characteristics (CitationLind 2003), which can lead to light reduction and sedimentation in the aquatic habitat (CitationParkhill and Gulliver 2002).
There are more than 7000 freshwater reservoirs in Guangdong Province, south China, some of which face pollution and eutrophication problems (CitationJiang et al. 2005). Because of high temperatures, lakes in tropical and subtropical areas are more sensitive than temperate lakes to increases in nutrient supply, and they show larger proportionate changes in water quality and biotic communities in response to eutrophication (CitationLewis 2000). The summer Asian monsoon has a dominant influence on many limnological factors in East Asian reservoirs (CitationAn and Park 2002). In recent years, many researchers have focused on chemical and biological characters of tropical and subtropical reservoirs in south China (CitationLin et al. 2003a, Citation2006, CitationLin, 2009, CitationSun et al. 2010), but basic hydrodynamic processes have not been addressed sufficiently.
The aim of this study was to advance the general understanding of temporal and spatial variations in the main physical descriptors of a typical subtropic reservoir and determine critical controlling factors on hydrodynamic processes for reservoir water quality management. Based on this, some regulatory and management recommendations are proposed to improve water quality in this reservoir.
Study site
Liuxihe Reservoir (23°45′50″N; 113°46′52″E) is a valley-type subtropical reservoir located in Guangdong province, south China, ∼90 km northeast of Guangzhou (). This impoundment, initially filled in 1958, is an important source of drinking water to residents in downstream areas, including Guangzhou (population >14 million). The lacustrine zone has dendritic complexities, and the dam is located at its southwestern end. When full, the reservoir has a surface area of 14.9 km2, with a mean and maximum depth of 22 and 73 m, respectively. Generally, the high water level is 235 m a.s.l. (3.25 × 108 m3 capacity) and the dead water level is 213 m a.s.l. (0.86 × 108 m3 capacity).
The reservoir has 2 major tributaries, the Lvtian and Yuxi rivers, which drain a catchment area of 264.4 km2 and 192.3 km2, respectively, comprising ∼85% of the total watershed area (539 km2; ). The main outlet is the Liuxihe hydropower station intake near the dam, with a bottom elevation of 206.5 m. Mean water retention time is ∼170 d, with shorter retention in the wet season (65 d; CitationLin et al. 2003b). Surveys to determine the nutrient budget, specific composition and structure of phytoplankton and zooplankton were conducted in the reservoir (CitationLin et al. 2003a, Citation2006, CitationLin 2009), which indicated that it is an oligomesotrophic lake (CitationLin et al. 2006), and the growth of phytoplankton is limited strongly by phosphorus (Total Nitrogen to Total Phosphorus [TN/TP]:41; Dissolved Inorganic Nitrogen to Dissolved Inorganic Phosphorus [DIN/DIP]:78; CitationLin 2009).
Upstream and surrounding landscapes of the reservoir are mainly small hills covered with forest, and low human activity ensures the inflow water is of good condition. The main water body near the dam is part of the Liuxihe National Forest park and is used for recreation.
Materials and methods
Extensive spatial surveys of Liuxihe Reservoir were undertaken almost every month from April 2008 to May 2010. Nine sampling sites were established on the reservoir and the 2 major river arms () extending approximately 11 km from the dam wall. Temperature, turbidity, dissolved oxygen (DO), chlorophyll, and conductivity profiles were measured through the water column at each site using a multiparameter water quality meter AAQ-1183IF (Alec Electronics Co., Japan). Water velocity and discharge of the riverine (S1, S2) and transitional zones (S3) were measured by Acoustic Doppler Coupled Profiler (ADCP), at 1.5 MHz (maximum detectable depth: 25 m; Sontek Inc, USA), and the lacustrine zone (S4, S8) was measured by ADCP at 500 KHz (maximum detectable depth: 120 m; Sontek Inc, USA).
A thermistor chain consisting of 9 Onset HOBO temperature data loggers (accuracy: 0.2 C, Onset Co, USA) attached along a plastic rope at 2 m intervals, was weighted at one end and fitted with a float at the other. For safety of the monitoring devices, the chain was deployed in the south part of the lacustrine zone (23°45′10″N; 113°46′55″E) in the precinct of the national forest park. A temperature, turbidity, and chlorophyll data logger (COMPACT-CLW, Alec Electronics Co, Japan) was also placed 1 m under the surface. These devices were set to record hourly data from 13 May 2008 to 31 December 2009.
A linear regression equation relating suspended sediment concentration (Css, determined by filtration using Whatman® GF/C filters with a nominal pore size of 0.45 μm) to turbidity (measured by AAQ1183IF and COMPACT-CLW) based on 11 samples was used to convert turbidity (Formazin Turbidity Unit, FTU) to Css concentration (AAQ1183-IF: Css = 1.0406 FTU, R = 0.99, P < 0.01; COMPACT-CLW: Css = 1.172 FTU, R = 0.99, P < 0.01).
Table 1 Inflow and water velocity measured by ADCP.
Figure 3 Water temperature of the southeastern lacustrine zone (bottom elevation: 204 m) measured by thermistor chain throughout 2009.
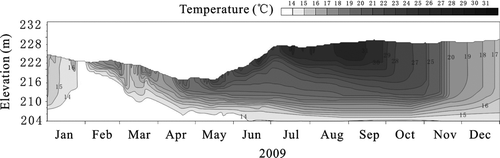
An HOBO meteorological station was deployed on the top of a building near the south shore (23°44′53″N; 113°46′46.2″E) and recorded meteorological variables (solar radiation, air temperature, air pressure, and relative humidity) every 20 min.
Samples of suspended sediments were collected by conventional sediment traps composed of cylindrical polyvinyl chloride (PVC) tubes with an aspect ratio (ht/dia) of 6.5 and 10 cm dia. Traps were deployed vertically in groups of 4, with the openings maintained close to horizontal by subsurface air-filled polyethylene containers. The collected sediments were dispersed in distilled water, and their particle diameters were measured using a particle size analyzer (Mastersizer-2000, Malvern Instruments Ltd, UK). After being air dried and ground to powder, organic matter content was determined by the potassium dichromate method (CSFA 1999), and mineral composition was obtained from X-ray diffraction analysis (Hseung CitationHseung and Chen 1985 using a D/Max- III C diffractometer (Rigaku Co, Japan).
Results and discussion
Hydrological conditions
Hydrological conditions including water level, precipitation, and reservoir outflow rate were recorded at the Liuxihe hydropower station, and the inflow rate was estimated based on the relationship between water level and outflow rate. The water level and inflow volume had clear seasonal variations, with a large interannual difference in inflow between 2008 and 2009 (). As a result of the Asian monsoon climate, ∼79% of annual precipitation (2250 mm) fell in the wet season (Apr–Sep; CitationLin et al. 2003b). The mean inflow rate for the last decade was 35.2 m3/s in the wet season and 8.6 m3/s in the dry season (Oct–Mar).
The velocity of the Lvtian River (S1) was higher than that of the Yuxi River (S2), and the riverine zone velocity was 2–4 times higher than that of the lacustrine zone (S4, S8), especially after heavy rain. Following a period of low rainfall and continuous drawdown, on 18 March 2010 the water velocity was very low both in the riverine and lacustrine zones, and the measured inflow rate were close to the dry season mean value (). During the wet season, the inflow rates increased by 2–3 times as a result of continuous rainfall or sudden strong storms (such as those that occurred at S1, S2 and S3 on 8 May), but in the lacustrine zone (S4, S8), flow rates remained very low (<2 cm/s; ), which may indicate that inflow disturbance is relatively weak in this reservoir.
Thermal and oxygen stratification
Temperature is the major controlling factor in lake stratification that affects lake hydrodynamics by forming vertical density gradients (CitationÇalıskan and Elçi 2009). Liuxihe Reservoir behaves as a warm monomictic water body according to Lewis's lake classification (CitationLewis 1983). The reservoir was stratified for most of the year and mixed only in the last week of January. Its minimum temperature was 13.2 C during mixing (), and its maximum was 31 C in the epilimnion (July 2009, ).
After the short mixing period, the lake water was warmed from the surface downward. With continuous heating, the thermocline formed in the epilimnion, which moved to ∼205 m elevation in late spring and stabilized at this height until further mixing. The hypolimnion (below 205 m) temperature was around 13 C throughout the year ( and ).
This thermal stratification pattern may result from the specific bathymetrical and hydrological conditions of the reservoir. First, because of the shape of such a valley-type reservoir, current hypolimnion capacity (0.683 × 108 m3) accounts for only a small fraction (21%) of the whole reservoir (3.25 × 108 m3). Second, the warm and humid subtropical climate with abundant rainfall leads to a large inflow volume and short residence time, especially in the wet season. The only reservoir outlet (3 m dia) is fixed in the middle layer with an elevation of 208.75 m (bottom elevation 206.5 m, which coincides with the lower boundary of the thermocline). Thus, massive water inflow in spring from the surface migrated to the outlet and warmed the water above the outlet level. Almost no heat exchange occurred between inflow and hypolimnion water, which created the highest temperature gradient near the bottom elevation of withdrawal.
The strong effect of stratification on vertical oxygen distribution can be observed in the seasonal variation (). Because of some technical problems in the instruments, we obtained only 10 DO profiles during the study period, but the main seasonal trend can be obtained nonetheless.
The surface water was well oxygenated in all seasons, but the hypolimnion remained completely anoxic from April to November. In spring, DO decreased gradually from the surface to bottom (Fig. 4a–c and 4h–j), and in summer the DO of the metalimnion decreased significantly because of oxygen consumption by organic matter (). With continuous cooling in autumn, the upper layer mixed thoroughly with the sharp thermocline, and a large DO gradient appeared at the upper boundary of the thermocline ().
In stratified lakes, vertical water movement is hindered and the thermocline behaves as a barrier to DO transport (CitationElci 2008), a condition seen in Liuxihe Reservoir. In addition, the thoroughly mixed period is so short () that DO replenishment by reaeration is not complete, especially in the hypolimnion. In addition to internal mixing, water inflow also plays an important role in providing DO (CitationBellanger et al. 2004, CitationMartin and McCutcheon 1999), but exogenous organic matter entrained by the inflow will increase the consumption of DO in the water column as it settles.
Turbid intrusion
The turbidity of the water column had distinct seasonal and interannual trends in Liuxihe Reservoir (), and correlation of turbidity and inflow volume was significant (CitationWang et al. 2010). This phenomenon is often due to terrigenous particles delivered to lakes and reservoirs (CitationEffler et al. 2002, CitationAnthony et al. 2008).
Except in winter, most turbid water persisted at the epilimnion and metalimnion during the study period and showed a systematic change: turbidity increased in spring, reached its maximum in summer, then declined in autumn (). The turbidity peak first appeared at 215 m a.s.l. in spring and early summer, then at the layer between 205 and 210 m a.s.l., and then decreased gradually with the end of wet season (). In winter, hypolimnetic water had a higher turbidity, but it was much lower than the wet season level (Apr–Sep) in the metalimnion. In addition, the turbidity in the 2008 wet season was much higher than in 2009, which was consistent with differences in inflow volume and precipitation between the 2 years. Multiple peaks in the water column () indicate that vertical mixing was very weak in stratified periods, and with continuous withdrawal the upper water layer moved gradually to the outlet height (206.5 m).
The phenomenon of suspended sediments and matter sinking as a turbidity current has been observed in many reservoirs (CitationFischer et al. 1979, CitationMartin and McCutcheon 1999). This is driven by greater tributary density relative to the upper waters of the receiving reservoir (CitationEffler et al. 2006). The turbidity currents supply large quantities of nutrients and organic carbon to the metalimnion. Some of these substances can be advected into the epilimnion and may contribute to a seasonal increase in primary productivity (CitationKim et al. 2000).
In Liuxihe Reservoir, the increased precipitation and resultant increased inflow from stream channels mobilized particulate material from both the surrounding catchment watershed and the riverbed, and thereby increased the suspended matter loading rate during runoff events, which entered the reservoir as a turbid plunging inflow or density current (). In most cases, Yuxi River is more turbid than Lvtian River and acts the main source of suspended matter due to the relatively larger population and more intensive land use in its catchment.
In freshwater reservoirs, water temperature and suspended matter are major factors determining water density and, consequently, hydrodynamics (CitationAlavian et al. 1992, CitationCheng and Kao 2008). In spring, as the warming of lacustrine water lags behind river water, turbid water flows into the epilimnion (). The surface turbid plume was very distinct in 2009 (), and so light attenuation was high. In summer, the turbid inflow water plunged to the metalimnion, and turbidity peaks occurred in the thermocline region because the inflow water was heavier than the surface water and lighter than the hypolimnion water (). In late autumn and winter, cold inflow plunged, continued as underflow, and traveled along the bottom of the reservoir to the dam, so the hypolimnion turbidity increased and was higher than the metalimnion (). Such cold water underflow in the winter can supply oxygen to the hypolimnion. In other deep subtropical reservoirs this underflow has quickly returned the hypolimnion to an oxic condition (CitationChen and Wu 2003) and could increase the fraction of biologically available phosphorous and iron in the total load delivered by runoff (CitationMcCullough et al. 2007). In Liuxihe Reservoir, however, high water level, low inflow volume, and long residence time in winter, led to weakness of inflow-induced reoxygenation in the hypolimnion. After a mixing period, DO of the lower water layer increased but was still anoxic ().
Deposition rate experiments in 2007–2008 showed that more suspended matter was deposited on the bottom of S3 in the wet season (776 g/m2/d) than the dry season (53 g/m2/d), while less was deposited at the bottom of S8 (the dam site) in the wet season (8–15 g/m2/d) than the dry season (50 g/m2/d) (CitationLin 2009). This consistent temporal turbidity variation () indicates that most suspended matter was flushed into the reservoir. After settling in the transitional zone (S3), much matter remained suspended in the water column and flowed from the outlet in the wet season. In the dry season, most suspended matter entrained by underflow (much less than in the wet season) was deposited in the reservoir.
Suspended matter, which was mainly silt and clay, was collected in April and May 2010 (flooding season) by cylindrical traps at 4 critical sites (S1, S2, S3, and S8). Organic matter accounted for only a small proportion (2.4, 2.45, 2.69, and 5.13%, respectively), which indicated that more inorganic matter (sediment) is deposited in the riverine and transitional zones than organic matter during the wet season.
Kaolin (>50%) and mica comprised most of the mineral material in all samples (). Suspended matter in S2 had more kaolin but less quartz and mica than S1, which may reflect the differences in flow and catchment land uses. The differences in mineral composition between S3 and S8 indicated that more mica and quartz are deposited in the lacustrine zone, and the dominance of kaolin in suspended matter at the dam site (S8) was greater than at S3 because of its low settling velocity.
Because of a reduction in flow velocities, coarser particles from river inflows deposit rapidly in reservoirs, but finer ones remain suspended for longer periods and are often present in outflow waters (CitationMarsalek et al. 1997). The suspended matter loading in Yuxi River (S2) was finer than in Lvtian River (S1), with “volume median diameters” (D[v, 0.5]; see CitationRawle 2003) of 3.03 μm and 6.75 μm (), respectively. This can be explained by different terrain: there is a wide shallow river section near the mouth of the Yuxi River (), and a hydropower station with a small impoundment is located ∼2.8 km upstream of S2. In these two parts of the river the water velocity is greatly reduced, and most of the coarser suspended matter is deposited there. Conversely, the Lvtian River has a straight water course with little human intervention, and its velocity is greater than Yuxi River, especially during runoff events (). As expected, D[v, 0.5] of S3 (the confluence area) lies between the 2 rivers (3.83 μm, ), and only finer particles flow through the lake and reach the dam (D[v, 0.5] of S8 is 2.76 μm, ).
Fine suspended particles are not only an aesthetic problem but are also known to be enriched with nutrients that create water quality control problems (CitationChen and Wu 2004) because of their higher exchange capacity and greater surface area (CitationProve 1991). Some studies have found that in-reservoir impacts of runoff events largely disappear within 4–5 d (CitationEffler et al. 2006). In Liuxihe Reservoir, suspended particles entrained by runoff events stayed in the water column for the whole flooding season in 2008 ().
The low velocity of the lacustrine zone () caused coarser particles to be deposited near the river mouth, and only finer particles with lower settling velocity entered the lake. Kaolin, the main component of the suspended matter (), has more surface area because of its disk shape; therefore, it settles more slowly than other particle types because of increased drag compared to equivalent spherical diameters (CitationRawle 2003). Furthermore, with small particles (<2 μm) Brownian motion hinders gravitational settling (CitationRawle 2003). In addition, aggregation and coagulation is negligible because of the low organic content of the suspended matter, low salinities, and lack of mixing energy in deep reservoir environments (CitationChung et al. 2009). Therefore, in the lacustrine zone, fine particles remain in the water column for a relatively long time as a result of low settling rate and may influence the aquatic ecosystem.
Water clarity
Water clarity is an indicator used widely to describe a lake's overall water quality (CitationLi and Li 2004, CitationWu et al. 2009 Clarity affects the primary productivity (CitationMcCullough et al. 2007) and recreation function of lakes and reservoirs. Because deep nutrient-poor lakes are rarely affected by phytoplankton blooms, water clarity can be subject to variability with changes in influx of suspended sediments (CitationSchwarz and Hawes 1997), which is also observed in the oligomesotrophic Liuxihe Reservoir (CitationLin et al. 2006).
Daily mean turbidity of the lacustrine surface layer increased after intensive rains, while the Secchi disk transparency declined () because of erosion in the watershed. In the long term, decreasing water clarity was more closely synchronized with lowering of lake level than with storms (). In the 2008 wet season, high turbidity levels following heavy rain lasted only a few days, and surface water clarity returned to prerain status. When the water level was low (Apr–Jun 2009), surface turbidity persisted at a relative high level for nearly 3 months until returning to a low level (below 5 FTU). In the dry season, no significant turbidity fluctuations occurred, but turbidity was a little higher in 2008 ().
Many researchers attribute this phenomenon to enhanced sediment resuspension from the lake bottom when bottom shear exceeds a critical shear stress for the sediment bed (CitationDirnberger and Weinberger 2005, CitationEffler et al. 2006, CitationSánchez-Carrillo et al. 2007). Although Liuxihe Reservoir is a deep water body, resuspension effects may occur in its shallow margins.
Specific landforms and hydrologic conditions of Liuxihe Reservoir also contribute to suspended matter. In the last decade (2001–2010) the water level of the reservoir was above 220 m most of the time, except some days in spring (2002: 54 d; 2008: 29 d; and 2009: 68 d). The lowest elevations of Lvtian and Yuxi rivers are 217.63 m (S1) and 218.2 m (S2), respectively, with a constant depth of 5–8 m. The velocity of the 2 rivers (S1, S2) is less than 7 cm/s in wet season and 3 cm/s in the dry season (). Much sediment is deposited on the river bed close to the reservoir because of the velocity reduction. When the water level falls to <220 m, inflow water will flush the river bed close to the reservoir, remobilizing the earlier deposited sediments and entraining them to the reservoir as a turbid plume because of the shallow depth of the stream channel close to the reservoir. In April 2009, the water level fell to <217 m (), which lead to a more distinct surface turbid plume than in the same period in 2008 (), although the inflow volumes of March and April were very similar ().
Thermal stratification plays an important role in surface clarity. Weak turbulence is expected in water layers with a high vertical density gradient for stabilization of the water column, which leads to high turbidity (CitationElci 2008). After March, the thermocline reached outlet level (205–210 m) and stayed there until mixing occurred (). Lower water level leads to a higher vertical density gradient in the metalimnion under the same meteorological conditions. In this case suspended matter settled more slowly, and low clarity surface water persisted for a long period ().
Summary and management recommendations
Liuxihe Reservoir is a subtropical reservoir with hydrological and ecological characters typical of south China. As the main water resource for Guangzhou, its water quality requires regulation and management.
The reservoir is a warm monomictic lake with summer stratification and winter circulation. Influenced by tropical and subtropical climate, the complete mixing period is very short (about 1 week), and hypolimnion water is anoxic most of the year. Oxygen supply mainly results from winter mixing. The range of the metalimnion is controlled by outlet height, below which is the hypolimnion with a stable temperature of 13 C throughout the year.
Much suspended matter is entrained by water entering the reservoir, which is composed mainly of clay minerals with low organic content. The turbid water flows into a layer of equivalent density (mainly determined by temperature) and moves along that layer. In early spring, such turbid intrusion manifests as a surface plume, in late autumn and winter as an underflow, and at other times the turbid current plunges to the metalimnion and spreads along the layer with the highest density gradients near the thermocline. Because of reduction of water velocity in the reservoir, most coarse particles deposit in the transitional zone and only finer particles enter the lacustrine zone. Because of the small particle size, the specific disk shape of kaolin (the main component of suspended matter), and weak vertical circulation, the suspended matter can stay in the water column for a long time. The surface clarity of the lacustrine zone is affected significantly by water level variation and other hydrological conditions, stratification, sediment resuspension, and landforms. These characteristics will influence water quality and aquatic ecosystem in many ways.
For sound reservoir management, we recommend the following:
First, to reduce the impact of low clarity caused by spring surface plumes, we suggest that water level should be maintained ≥220 m a.s.l., which would reduce erosion of the river bed and increase the settling rate of suspended matter. In the last decade, the mean lowest annual water level was 221.4 m a.s.l., and in some years (e.g., 2009) the level was below 220 m, which may lead to low water clarity. Maintaining the water level at 220 m a.s.l. is feasible with respect to downstream water demand under normal precipitation conditions. For example, the precipitation of 2003 (1586.5 mm) was similar to 2009 (1582.6 mm), but its lowest water level was 223.38 m a.s.l., which was much higher than for 2009 (216.84 m a.s.l.)
Second, selective withdrawal is advisable for improving reservoir water quality, although most reservoirs in China adopt singular fixed withdrawal. Hypolimnetic withdrawal has been shown to be an effective way to remove nutrients and electrochemically reduced substances in many stratified lakes, but the timing of withdrawal operation is critical, with late summer and autumn considered the preferred times (CitationNürnberg, 2007). However, the downstream effects of selective withdrawal should be considered before it is enacted. In Liuxihe Reservoir, surface withdrawal is not suggested in spring because it may enhance the stability of surface water, which can lead to low settling rate of suspended matter and eventually affect the water clarity.
In addition, water and soil conservation in the whole catchment is essential. Excessive human activities should be controlled in this drinking water conservation area to reduce the discharge of pollutants and garbage into the reservoir.
Future research will address the integration of reservoir physical factors with chemical and biological dynamics. This will further our understanding of reservoir systems and strengthen decision-making capability for reservoir regulation and management.
Acknowledgments
The work is supported by the Key Project of Unite Program of NSFC (National Natural Science Foundation of China) and Guangdong Province (U0733007) and National Basic Research Program of China (973 Program, 2008CB418003).
Notes
*Measured discharge of site 3 was considered the reservoir inflow rate. Daily average inflow was calculated as described above.
Reference
- An , K G and Jones , J R . 2000 . Temporal and spatial patterns in salinity and suspended solids in a reservoir influenced by the Asian monsoon . Hydrobiologia. , 436 : 179 – 89 .
- An , K G and Park , S S . 2002 . Indirect influence of the summer monsoon on chlorophyll–total phosphorus models in reservoirs: a case study . Ecol Model. , 152 : 191 – 203 .
- Anthony , R , Effler , S W , O’Donnell , D M , Smith , D G and Pierson , D . 2008 . Turbidity and temperature patterns in a reservoir and its primary tributary from robotic monitoring: Implications for managing the quality of withdrawals . Lake Reservoir Manag. , 24 ( 3 ) : 231 – 243 .
- Alavian , V , Jirka , G H , Denton , R A , Johnson , M C and Stefan , H G . 1992 . Density currents entering lakes and reservoirs . J Hydraulic Eng. , 118 ( 11 ) : 1464 – 1489 .
- Bellanger , B , Huon , S and Steinmann , P . 2004 . Oxic-anoxic conditions in the water column of a tropical freshwater reservoir (Pena-Larga dam, NW Venezuela) . Appl Geochem. , 19 : 1295 – 314 .
- Çalıskan , A and Elçi , S . 2009 . Effects of selective withdrawal on hydrodynamics of a stratified reservoir . Water Resour Manage. , 23 : 1257 – 1273 .
- Chen , Y J and Wu , S C . 2003 . Effects of sediment phosphorus release associated with the density current on water quality of a sub-tropical and deep reservoir in Taiwan . Water Sci Technol. , 48 : 151 – 158 .
- Chen , Y J and Wu , S C . 2004 . “ Effects of the phosphorus forms of the watershed on the speciation of phytoplankton: A case study of the two subtropical deep reservoirs in Taiwan ” . In Proceedings of World Water and Environmental Congress; June 27-July 1, 2004. Environmental and Water Resources Institute (EWRI) of American Society of Civil Engineers , Edited by: Gerald , Sehlke , Hayes , D F and Stevens , D K . 1 – 10 . Salt Lake City , UT : ASCE press .
- Chen , Y J , Wu , S C , Lee , B S and Hung , C C . 2006 . Behavior of storm-induced suspension interflow in subtropical Feitsui Reservoir, Taiwan . Limnol Oceanogr. , 51 : 1125 – 33 .
- Cheng , W F and Kao , S J . 2008 . Effects of climate events driven hydrodynamics on dissolved oxygen in a subtropical deep reservoir in Taiwan . Sci Total Environ. , 393 : 326 – 332 .
- Chung , S W , Hipsey , M R and Imberger , J . 2009 . Modelling the propagation of turbid density inflows into a stratified lake: Daecheong Reservoir, Korea . Environ Modell Softw. , 24 : 1467 – 1482 .
- [CSFA] Chinese State Forestry Administration . 1999 . Determination of organic matter in forest soil and calculation carbon-nitrogen ratio. Beijing LY/T 1237–1999. Chinese
- de Cesare , G , Schleiss , A and Hermann , F . 2001 . Impacts of turbidity currents on reservoir sedimentation . J Hydraulic Eng. , 127 ( 1 ) : 6 – 16 .
- Dirnberger , JM. and Weinberger , J. 2005 . Influences of lake level changes on reservoir water clarity in Allatoona Lake, Georgia . Lake Reserv Manag. , 21 ( 1 ) : 24 – 29 .
- Doyle , R D and Smart , R M . 2001 . Impacts of water column turbidity on the survival and growth of Vallisneria americana winterbuds and seedlings . Lake Reserv Manag. , 17 ( 1 ) : 17 – 28 .
- Effler , S W , Matthews , D A and Kaser , J W . 2006 . Run off event impacts on a water supply reservoir suspended sediment loading, turbid plume behavior, and sediment deposition . J Am Water Resour As. , 42 ( 6 ) : 1697 – 1701 .
- Effler , S W , Perkins , M G , Ohrazda , N , Matthews , D A , Gelda , R K , Peng , F , Johnson , D L and Stepczuk , C L . 2002 . Tripton, transparency and light penetration in seven New York reservoirs . Hydrobiologia. , 468 : 213 – 232 .
- Elci , S. 2008 . Effects of thermal stratification and mixing on reservoir water quality . Limnology. , 9 : 135 – 142 .
- Faithful , J W and Griffiths , D J . 2000 . Turbid flow through a tropical reservoir (Lake Dalrymple, Queensland, Australia): Responses to a summer storm event . Lakes Reserv Res Manag. , 5 : 231 – 247 .
- Fischer , H B , List , E J , Imberger , J , Koh , R C and Brooks , H N . 1979 . Mixing in inland and coastal waters , New York , NY : Academic Press .
- Hseung , Y and Chen , J F . 1985 . Soil Colloid, Vol. 2, Methods for soil colloid , 74 – 193 . Beijing (China) : Science Press . Chinese
- Jiang , T , Chen , X H , Wang , Z L , Shi , L S and Zhang , L . 2005 . Assessment of reservoir eutrophication in Guangdong Province . Hupo kexue. , 17 ( 4 ) : 378 – 382 . Chinese
- Kim , B , Choi , K , Kim , C , Lee , U H and Kim , Y H . 2000 . Effects of the summer monsoon on the distribution and loading of organic carbon in a deep reservoir, Lake Soyang, Korea . Water Res. , 34 ( 14 ) : 3495 – 3504 .
- Lewis , W M Jr . 1983 . A revised classification of lakes based on mixing . Can J Fish Aquat Sci. , 40 : 1779 – 1787 .
- Lewis , W M Jr . 2000 . Basis for the protection and management of tropical lakes . Lakes Reserv Res Manag. , 5 : 35 – 48 .
- Li , R and Li , J . 2004 . Satellite remote sensing technology for lake water clarity monitoring: an overview . Environ Inform Arch. , 2 : 893 – 901 .
- Lin , GE. 2009 . The dynamics and budget of nitrogen and phosphorus in Reservoir Liuxihe. [Master's thesis] , [Guangzhou (P.R.China)] : Jinan University . Chinese
- Lin , Q Q , Duan , S S , Hu , R and Han , B P . 2003a . Zooplankton distribution in tropical reservoirs, South China . Int Rev Hydrobiol. , 23 ( 6 ) : 602 – 613 .
- Lin , Q Q , Gu , J G and Han , B P . 2006 . “ Spatial and temporal variation of plankton in Liuxihe Reservoir ” . In Reservoir ecology and water quality management in China. Beijing (PRC) , Edited by: Han , B P , Shi , Q C and Chen , X W . 3 – 20 . Science Press . Chinese
- Lin , Q Q , Hu , R and Han , B P . 2003b . Effect of hydrodynamics on nutrient and phytoplankton distribution in Liuxihe Reservoir . Acta Ecol Sinica. , 23 ( 11 ) : 2278 – 2284 . Chinese
- Lind , O T . 2003 . Suspended clay's effect on lake and reservoir limnology . Arch Hydrobiol Suppl. , 139 ( 3 ) : 327 – 360 .
- Lind , O T , Chrzanowski , T H and Lind , L D . 1997 . Clay turbidity and the relative production of bacterioplankton and phytoplankton . Hydrobiologia , 353 : 1 – 18 .
- McCullough , G K , Barber , D and Cooley , P M . 2007 . The vertical distribution of runoff and its suspended load in Lake Malawi . J Great Lakes Res. , 33 : 449 – 465 .
- Marsalek , J , Watt , W E , Anderson , B C and Jaskot , C . 1997 . Physical and chemical characteristics of sediments from a stormwater management pond . Water Qual Res J Can. , 32 : 89 – 100 .
- Martin , J L and McCutcheon , S A . 1999 . Hydrodynamics and transport for water quality modeling , Boca Raton (FL) : Lewis Publishers Inc .
- Nürnberg , GK. 2007 . Lake responses to long-term hypolimnetic withdrawal treatments . Lake Reserv Manage. , 23 ( 4 ) : 388 – 409 .
- Paez , R , Ruiz , G , Márquez , R , Soto , L M , Montiel , M and Lopez , C . 2001 . Limnological studies on a shallow reservoir in western Venezuela (Tulé reservoir) . Limnologica. , 31 ( 2 ) : 139 – 145 .
- Parkhill , K L and Gulliver , J S . 2002 . Effect of inorganic sediment on whole-stream productivity . Hydrobiologia , 472 : 5 – 17 .
- Prove , BG. 1991 . A study of the hydrological and erosional processes under sugar cane culture on the wet tropical coast of north-eastern Australia , [Townsville (AU)] : James Cook University . [Dissertation]
- Rawle , A . 2003 . Basic of principles of particle-size analysis . Surface coatings international, Part A , 86 ( 2 ) : 58 – 65 .
- Romergo , J R and Imberger , J . 2003 . Effects of a flood underflow on reservoir water quality: data and three-dimensional modeling . Arch Hydrobiol. , 157 ( 1 ) : 1 – 25 .
- Schwarz , A M and Hawes , I . 1997 . Effects of changing water clarity on characean biomass and species composition in a large oligotrophic lake . Aquat Bot. , 56 : 169 – 181 .
- Sánchez-Carrillo , S , Alatorre , L C , Sánchez-Andrés , R and Garatuza-Payán , J . 2007 . Eutrophication and sedimentation patterns in complete exploitation of water resources scenarios: An example from northwestern semi-arid Mexico . Environ Monit Assess. , 132 : 377 – 393 .
- Sun , Y P , Wang , X H , Hu , R and Han , B P . 2010 . Seasonal variation of phytoplankton communities in Xiangang Reservoir, a tropical shallow and high-yield fishery reservoir in south China . Chinese J Appl Environ Biol. , 16 ( 2 ) : 228 – 234 . Chinese
- Wang , S , Qian , X and Han , B P . Modeling the effects of inflow and outflow volume on turbidity variation in Liuxihe Reservoir . 4th International Conference on Bioinformatics and Biomedical Engineering, 18–20 June 2010 . Chengdu , China. pp. 1 – 4 . Piscataway, NJ: IEEE Computer Society .
- Wu , G F , de Leeuw , J and Liu , Y L . 2009 . Understanding seasonal water clarity dynamics of Lake Dahuchi from in situ and remote sensing data . Water Resour Manage. , 23 : 1849 – 1861 .