Abstract
We describe the successful application of a 4-decade analysis of an Index of Biotic Integrity (IBI) demonstrating its assessment value when applied to the fish community of Lake Chapala. We based our analysis on fish community and fishery composition and on the proportion and density of native and exotic species for each decade. Fisheries landings and IBI values document a long-term downward trend since the 1970s. Data from the 1990s and 2000s reveal a dramatic drop in the IBI score to 20 points (out of a possible 100), a rating of very poor. The fisheries collapse and the loss of 11 native species as a consequence of habitat degradation and invasive species have reduced native fish biomass and richness. Fluctuations in rainfall, principally, and water-extraction related to human activities have had a major impact on the lake. Additionally, water quality deterioration has been caused by a lack of wastewater treatment. The fish community has been further modified by large increases in the number and biomass of exotic fishes. Although the exact degree of decline in biotic integrity is impossible to determine because of uncertainty about the representativeness and accuracy of some historical information, the analysis of the temporal changes in the IBI provides compelling evidence for an accelerating drop in ecosystem health. The application of IBI, which is broad in scope and has flexible data requirements, can serve as a template for assessing trends in other water bodies with a history of fisheries data but limited standardized monitoring of the fish community.
Shallow lakes often undergo substantial changes in volume and surface area within and among years, and their fish communities are expected to be adapted to such changes; however, human activities can substantially impact natural water cycles. When coupled with other anthropogenic impacts, there can be major effects on the fish community and associated fisheries. Large, shallow, and tropical Lake Chapala, located in central Mexico, provides a clear example of a human-altered water cycle and serves as a model for assessing fish community responses to extreme hydrologic fluctuations (Mestre Citation1997, Aparicio Citation2001). Lake Chapala has experienced in the last decades a continuing and drastic decline in water quantity and quality as a result of human activities (de Anda and Shear 2001). Consequently, several restoration initiatives have been implemented, and water quality is reported to have improved according to the water quality index (Cloter et al. 2006). Nevertheless, this improvement has not been reflected in the fish community, where long-term decreases in fish species richness and fisheries catches continue (Moncayo-Estrada and Buelna-Osben Citation2001). The lack of response by the fish community to better water quality conditions emphasizes the need to develop and apply biologically based indicators to better assess and understand the overall status and trends in the “health” of shallow lakes such as Chapala that are experiencing major human perturbations.
The Index of Biotic Integrity (IBI) is a multimetric approach that assesses aquatic ecosystem health based on a set of biological community indicators (Karr Citation1981). Ecosystem integrity evaluations made using the IBI integrate the combined effects of a variety of water quality, habitat, and biological factors that are otherwise difficult to quantify. IBI determination and interpretation involves a sequence of activities including biological sample collection, data tabulation, and regional adaptation and calibration of metrics and expectation values. Numerous studies have proven the usefulness of the overall multimetric index framework (Karr and Chu Citation1999).
The application of some versions of the IBI in a long-term analysis is difficult because of their requirement for standardized monitoring data (Miller et al. Citation1988). However, several newer versions have been developed that permit the use of alternative types of nonstandardized or semiquantitative historical information (e.g., historical documents, official fishery statistics, faunal surveys), and these new semiquantitative IBIs can be applied when the availability of standardized monitoring data are limited (Metcalfe Citation1995). Semiquantitative IBIs seem to work best when the available historical nonstandardized data can be pooled over large spatial and temporal scales to give a picture of “typical” biotic integrity conditions over a relatively long time frame (i.e., several decades) and a broad area (i.e., an entire water body) rather than a precise estimate of biotic integrity during a particular short sampling period (i.e., within a single season) and precise location (i.e., a short reach of river or portion of lake shoreline). Semiquantitative IBIs work well on a decadal time scale to evaluate the impacts of long-term historical trends, such as climatic variation, changed fishery exploitation, or establishment of exotic species on the biological community (e.g., Peterson and Kwak Citation1999).
Standardized fish community data collection on most central Mexican lakesbegan only 10–15 years ago, so assessment of longer-term trends in biotic integrity requires use of a semiquantitative IBI. An IBI was developed for these lakes more than 10 years ago (Lyons et al. Citation2000), but until now it has not been fully tested. This IBI allows use of ichthyological studies and faunal surveys going back to the early 20th century that provide critical information on historical fish community composition and species distribution and relative abundance (Jordan and Evermann Citation1896, Cuesta-Terron Citation1925, de Buen 1946, Alvarez del Villar 1970, U de G 1983, Guzman Citation1995). However, some Mexican lakes, including Lake Chapala, do not have enough information to calculate all metrics, our critical reason for modifying the IBI for the present study. We incorporated long-term fishery survey statistics as a means to characterize many aspects of fish community structure. In addition, limnological studies, conducted irregularly as result of different governmental initiatives, provided relevant information on water quantity and quality (Chavez Citation1973, SARH 1981, Lind et al. Citation1992).
Lake Chapala has a well-documented and economically important fish fauna, but a highly altered lakeshore and catchment, making it an appropriate system to test the semiquantitative IBI in an analysis of long-term trends in biotic integrity. We evaluated the biological condition of the Lake Chapala fish community beginning in decades when it was believed to be relatively less influenced by human activities (de Buen 1946) and continuing to the present. In this study, biotic integrity change was described using historical information gathered from government agency fishery statistics coupled with available scientific fish surveys and summarized by decade to determine both initial fish community conditions and trends in the community. The specific research questions were: is there a discernible long-term trend in Lake Chapala's IBI score and integrity rating; and if so, is there a logical relationship between the fish community IBI and anthropogenic impacts? This study describes a useful framework for documenting changes and trends in other lakes where standardized monitoring data are limited or absent.
Study site
Lake Chapala (20°19’48“N; 103°25’34”W) is the largest natural lake in Mexico located at 1510 m a.s.l (; Guzman 2002). According to biophysical characteristics, this lake is defined as neotectonic, mesotrophic, and tropical with a catchment area of 52,000 km2 (Lind et al. Citation1992). Despite its large surface (90,000 ha), Lake Chapala is shallow with a maximum mean depth of 7 m (Lind and Davalos-Lind Citation2002). The lake is important because it provides 60% of the drinking water to Guadalajara, the second largest city in Mexico (CEA 2010). Lake Chapala is listed as one of the most threatened natural resources in the western hemisphere by the United Nations Environmental Program (Thielcke and Resch Citation2001).
Figure 1 Fish species composition and structure change in Lake Chapala. (a) Number of carnivores, sensitive and exotic species at different decades (pictures from Álvarez 1970). (b) Comparison between native and exotic fish landings as reported by government agency fisheries statistics (CONAPESCA, 2001).
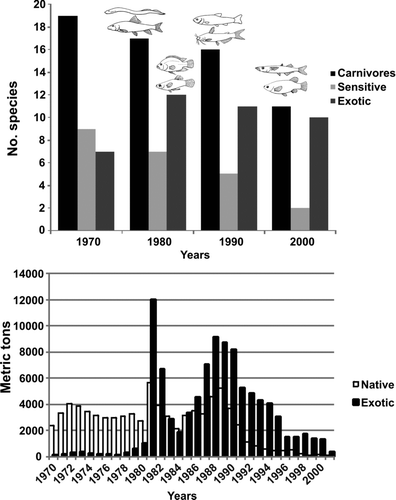
Materials and methods
Index of Biotic Integrity
A semiquantitative modification of the IBI was applied to Lake Chapala data to determine decadal changes in fish composition, structure, and function from the 1970s to the 2000s (Lyons et al. Citation2000). This period was chosen because sufficient data were available on general trends in fishery effort and catch and overall species composition (CONAPESCA 2001). Metric expectations and scoring criteria were determined (). The first 6 metrics, related to species diversity including the number of native and exotic fish, were reviewed, and the expected value was assigned to each according to Moncayo-Estrada and Buelna-Osben (Citation2001). The historic data were obtained from the literature (Chavez Citation1973, U de G 1983, Guzman Citation1995, Citation2002), from museum fish collections, and from comprehensive (but not standardized) lake fish community samples collected in 1978, 1985, and annually from 1997 to 2001 by the Instituto Politecnico Nacional in Mexico.
A metric for the number of nonindigenous species was included in the present study (, metric 3), and the scoring criteria were developed based on the number of species introduced for fisheries purposes and from aquarist activities in different lakes in Mexico. Because of biomass data limitations associated with a long term study, the abundance and condition metrics were based on the analysis of government agency fisheries statistics (CONAPESCA 2001). Percent biomass was calculated from the average fishery capture per decade. We used the number of fishermen as our measure of fishing effort because this was the only statistic with complete information throughout the study period. Catch per unit effort was determined and then utilized to estimate the relative biomass of each species group per decade. We corroborated the relative biomass information with the results of more-detailed studies carried out in specific years (Chavez Citation1973, U de G 1983, Guzman Citation1995, Citation2002). For the percent biomass of tolerant species, charales (small silverside species, Chriostoma app.) were considered tolerant, pescado blanco (large silverside species, mainly Chriostoma lucius) and popocha (native minnow, Algansea popoche) were considered sensitive, and bagre (catfish, Ictalurus dugesi) was considered moderate (, metric 7).
Table 1 Metrics and scoring criteria for the Index of Biotic Integrity (IBI; Karr 1981) as modified for Mexican lakes by Lyons et al. (Citation2000). Expected values (EV) for that metric in the absence of major human degradation, given in parentheses after each metric, were derived from Moncayo-Estrada and Buelna-Osben (Citation2001).
For trophic composition, the biomass metric of native carnivores was limited to only the top predator (3 species of pescado blanco; , metric 9). The scoring criteria were also modified because of the disproportionate capture of charales compared with pescado blanco. The top predator metric provides a measure of trophic complexity where less disturbed systems support a greater prey and predator diversity and a wider variety of habitats in which to find them (Karr et al. Citation1987).
The maximum standard length was evaluated for only one pescado blanco species (Chirostoma lucius) because high quality information was available only for this species (, metric 10). The lower range of the scoring scale was based on the minimum reproductive size of this species (140 mm standard length [SL]; Moncayo-Estrada et al. 2003). The metric related to exotic parasites was not considered because the necessary data were not available. IBI scores were divided into 5 categories: >0–20(very poor), >20–40 (poor), >40–60 (fair), >60–80 (good), and >80 (excellent; Karr Citation1981).
Statistical analysis
We correlated (Pearson coefficient) values of 15 selected physical, chemical, and anthropogenic characteristics of the lake and watershed with the IBI values for each decade to better understand possible explanations for trends in biotic integrity. In addition, we implemented a multiple regression analysis to evaluate the relationships between changes in lake characteristics and precipitation and Rio Lerma inflow (data from 1922 to 2001). The analysis was performed using SPSS for Windows ver. 17 (SPSS Inc., Chicago, IL; http://www.spss.com).
Results
The deterioration of Lake Chapala was evident in the IBI scores that declined steadily from the 1970s to the present (). In the 1970s the IBI score was 75 and rated as good, and 27 native species were present. Although the IBI rating remained good in the 1980s, the score declined by10 points to 65 because of the decreased number of sensitive and carnivore species (Lampetra spadiceus and Moxostoma austrinum) and the increased biomass of exotics, particularly common carp (Cyprinus carpio) and the recently introduced tilapia (Oreochromis aureus; ). Catch of these 2 exotics grew exponentially through the decade as compared with 1970s. At the beginning of the 1980s an increase in fishing effort resulted in the highest catches of all species in the history of the lake's fishery. In the middle of the decade, a major decline in catch occurred because of new regulations designed to conserve fishery resources that restricted the use of the highly efficient seine net (chinchorro). Catches began to recover at the end of the decade because of the second increase in the effort (more gill nets; ).
Table 2 Scores and values (in parentheses) for the 10 metrics from the Lake Chapala fish community decadal analysis.
Figure 2 Fish species capture data in Lake Chapala as reported by government agency fisheries statistics (CONAPESCA, 2001). Species were divided in 2 graphs because of the difference in the magnitude of capture values.
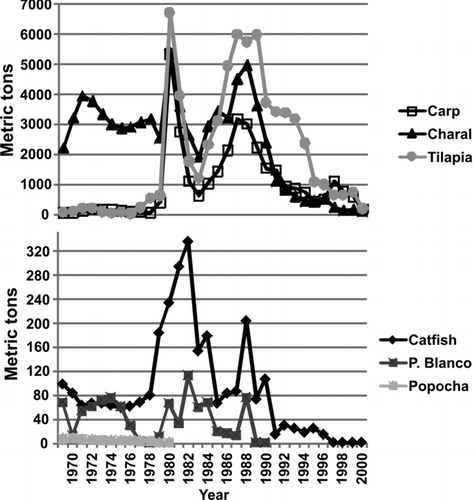
By the 1990s the IBI score had declined 15 additional points to 50 and the rating dropped to fair (). Three native species, including the cyprinid Notropis sallei, the ictalurid Ictalurus ochoterenai, and the goodeid Skiffia multipunctata, were no longer found (). The metric score for native goodeids was reduced 5 points, and its rating dropped from good to fair. In particular, the capture of almost all species in the fishery, except tilapia, was reduced by half despite continued high fishing effort indicating over-exploitation (). Top predator biomass (pescado blanco) decreased to such a low level that it was no longer registered in the official statistics. In the decade of the 2000s, the IBI declined another 30 points to 20, and the rating slipped to very poor (). In 2000, all fisheries in the lake collapsed (). Compared with the 1970s, 11 native species had disappeared from the catch, including one atherinops (Chirostoma cotrerasi) and several goodeids (; ).
Table 3 Fish species in Lake Chapala by decade, with origin, feeding and tolerance classifications following Lyons et al. (Citation2000) criteria. Taxonomy follows Mayden et al. (1992), except for Goodeidae, which follows Webb (1998).
Several lake and human attributes were strongly related to the decline in IBI scores. Scores were significantly positively correlated with lake surface elevation, a measure of lake volume and surface area (Pearson = 0.983, p = 0.017), and the water quality index (Pearson = 0.981, p = 0.019). In comparison, IBI was negatively correlated with total suspended solids (Pearson = −0.995, p = 0.005), hydraulic residence time (Pearson = −0.992, p = 0.008), total phosphorus (Pearson = −0.984, p = 0.016), Guadalajara population (Pearson = −0.980, p = 0.02), and biochemical oxygen demand (Pearson = −0.975, p = 0.025; ). Lake Chapala volume decrease was directly related to the water quality index (Pearson = 0.992, p = 0.008) and precipitation (Pearson = 0.843, p = 0.157), and inversely related to total suspended solids (Pearson = 0.995, p = 0.005), hydraulic residence time (Pearson = −0.994, p = 0.006), and total phosphorus (Pearson = −0.993, p = 0.007). Although lake elevation presented a reduced multiple correlation coefficient value with rainfall and Rio Lerma Inflow (r = 0.354, F = 5.5, p = 0.006), part of the difference was related to river modification upstream and the retention of the water in upstream reservoirs; however, the graphical analysis indicates an important relationship ().
Figure 3 Relationship between (a) the IBI values in different decades with Lake Chapala surface elevation, (b) water quality index, (c) total suspended solids, (d) hydraulic residence time, (e) total Phosphorous, and (f) population growth of Guadalajara city. Graphs include the tendency line with 95.0% Mean Prediction Interval.
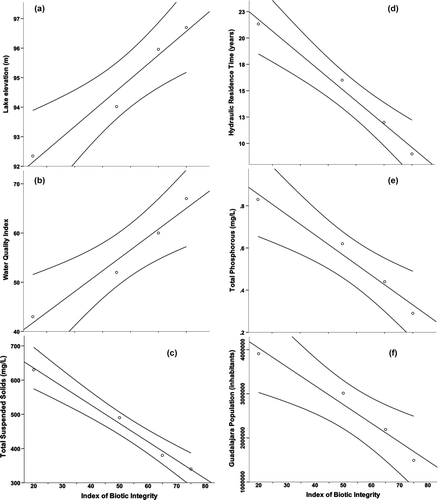
Discussion
The biotic integrity of Lake Chapala decreased dramatically from the 1970s to the 2000s, probably because of a continuously decreasing lake volume, ongoing fishery overexploitation, water quality degradation, and the introduction of exotic species. Similar patterns of human impact are occurring in lakes and rivers elsewhere in Mexico and in other tropical countries, and highlight the urgent need to implement habitat protection and enhancement in nearshore areas and to prevent exotic species introductions (Bence et al. Citation2002, Bronte et al. Citation2003, Mills et al. Citation2003, Mercado-Silva et al. Citation2006). The IBI provides a tool to quantify the effectiveness of such conservation measures.
Biotic integrity and volume reduction
Meteorological conditions were the main driving factors affecting the lake volume change. Of secondary importance are human actions that modify the physical ecosystem properties and presumably the fish community as well. The mean annual rainfall in the 1940s dropped from 750 to 680 mm and, starting in 1945, water levels in the lake declined sharply, from an average of 1523.80 to 1517.60 m a.s.l. in 1954 (6.2 m; de Anda et al. 1998; ). A quick recovery took place in the 1960s as result of constant high rainfall (786 mm) and lasted until the first half of the 1970s.
From 1979 to the mid-1980s, a second period of substantial water-level decline occurred that coincided with a reduction in the mean annual rainfall (680 mm). At the same time, however, the constructed reservoir storage capacity in the watershed increased from 1817 to 3678 million cubic meters, and the average irrigated area had grown from 250,000 to ∼650,000 ha, mainly due to an increase in groundwater irrigation (Aparicio Citation2001, Wester et al. Citation2001). The main water user in the watershed is irrigated agriculture, with 91% consumptive use of surface water and 69% consumptive use of groundwater (Wester et al. Citation2001). After a modest recuperation in the early 1990s (mean rainfall 745 mm from 1990 to 1993), lake levels declined due to natural droughts with the smallest mean annual rainfall value (630 mm) and decreasing inputs from the Rio Lerma (). In addition, the lake level decline was partly due to an increase in direct water withdrawals to the city of Guadalajara, at which time the lake reached its minimum in 2001 with a loss of more than 80% of its volume (Guzman Citation2002).
Some authors argue that the hydrology of Lake Chapala and its watershed can compensate for anthropogenic losses (Filonov et al. Citation2001), but others disagree and associate the declining water regime in the 1990s to the human use of water in the basin (de Anda and Shear 2001). Two closely related arguments support the role of human water use in reduced lake levels. First, despite an increase in rainfall, the inflow value from the Rio Lerma dropped by more than half due to over exploitation upstream: inflow from 1922 to 1969 was 1646 hm3, and average annual rainfall was 706 mm; inflow from 1970 to 2001 was 738 Mm3, and average annual rainfall was 697 mm (Aparicio Citation2001). Second, reduced inflow is associated with an increase in the hydraulic residence time, with a maximum value of 40 years calculated in 1989 and an average of 15 years for the last 3 decades; the average before the 1970s was only 5 years (de Anda and Shear 2001).
Furthermore, part of this lake-level decline is related to the increasing water demand as a consequence of important population density changes. The population in the watershed has increased significantly, doubling from 2.1 million inhabitants in 1930 to 4.5 million in 1970, and then more than doubling again in the next 30 years to 11 million in 2000 (INEGI 2005). The city of Guadalajara had a population growth from 1970 to 2000 of approximately one million inhabitants per decade and an increasing extraction of water from the lake from 4000 to 7500 L/s, respectively.
Natural fluctuations in Lake Chapala's volume have occurred in the past because of droughts. For example, low precipitation resulted in volume losses of 93% in 1897 and 90% in 1955 (Lind and Davalos-Lind Citation2002). However, we have no evidence that the fish community was permanently modified or fish species disappeared as a result of the earlier droughts (i.e., 1955, as compared with the results from the de Buen study in 1946). In contrast, lake volume changes from the 1970s to the present are associated with the loss of almost 50% of the native fish species of Lake Chapala, and the community structure has changed in irreversible ways (Moncayo- Estrada and Buelna-Osben 2001). Whether these species have truly been eliminated from the lake or still persist in low numbers is unknown because the great size and complexity of Lake Chapala make comprehensive lake-wide surveys of small fishes prohibitively difficult.
The altered water regime of Lake Chapala has influenced 2 potential hydrological aspects that affect the biotic integrity of the fish community. The first relates to the continuous drying of shallow areas near the shorelines that are important habitats for some native small species (goodeids) and spawning sites for others (Algansea and Notropis). The second relates to the reduced inflow limiting species spawning migrations out of the lake, such as Lampetra to the Río Duero, which enters the Rio Lerma about 20 km above the inlet to Lake Chapala.
Biotic integrity and water quality
As lake volume has declined, water quality in Lake Chapala has deteriorated to the detriment of the fish community. Lower lake volumes led to increases in total dissolved solids (TDS) of more than 100% and in phosphorus of almost 400% (Lind et al. Citation1992). In the shallower conditions, wind increased water turbidity by sediment resuspension, modifying trophic relationships (Lind and Davalos-Lind Citation2002). Total suspended solids increased from 340 mg/L in 1970 to 630 mg/L in 2000. In addition, soil erosion transported by the major rivers to Lake Chapala increased 3 times to approximately 1.9 × 106 m3 (SEDUE 1991). de Anda et al. (2004) established a minimal water column height of 5.0 m to meet the USEPA criterion for TDS concentration in a lake. This mean height was only achieved in the1970s (6.52 m), while in the 1990s the average depth of the lake reached just 3.87 m.
Suspended sediments affected the trophic status in the lake because they increased light limitation of water column phytoplankton production, despite substantial increases of nutrients (0.83 mg/L of total phosphorus and 0.24 mg/L of nitrates in 1990; Lind et al. 1992). Based on algal biomass, Lake Chapala has maintained a consistent mesotrophic status during the last decades (Lind and Davalos-Lind Citation2002). The presence of such nutrients, which are highly negatively correlated to the biotic integrity (), promoted a bloom of water hyacinth (Eichornia crassipes) in 1992–1993 that covered 20% of the lake's surface (135 km2), leading to higher rates of evapotranspiration that further reduced lake volume (Lind and Davalos-Lind Citation2002). Further stressing the lake, wastewater analyses found 130,500 t/y biochemical oxygen demand coming from urban waste and 424,260 t/y chemical oxygen demand coming from industrial discharges (Mestre Citation1997).
Another critical aspect involving water quality is the import of toxic elements in Lake Chapala that affect the fish community and threaten human health. The most important industries in the watershed, numbering about 3500 different facilities, concentrate their activities on meat processing, agricultural products, pulp and paper, and petrochemical products, all with little or no wastewater treatment or recycling. A study in the 1990s found high concentrations of metals in water hyacinth (Eichornia crassipes) and cattails (Typha latifolia; Jay and Ford 2001). Copper and lead concentrations in tilapia and carp muscle and liver tissue exceeded action levels for human health (Shine et al. Citation1998). However, a study conducted in charales, smaller and shorter lived than tilapia and carp, concluded that all metals with the exception of mercury were present at levels below US and other international guidelines (Ford et al. Citation2000).
Biotic integrity, fisheries and exotic species
The relatively high IBI value in the 1970s indicated good ecosystem conditions in Lake Chapala when the lake volume was near capacity and there was relatively low fishing effort. However, at the beginning of the 1980s, fishing effects increased because of greater fisheries effort (i.e., more fishers and technological net improvements; Orbe et al. Citation2001). During the 1980s, more than 80% of the inland commercial fish catches recorded in the state of Jalisco came from Lake Chapala (Perez et al. Citation1998). This increased effort resulted from a government policy that promoted increases in production from different productive sectors such as agriculture and fisheries (de Anda and Shear 2001). The increased fishing effort strongly affected the fish community, evidenced by the fishery collapse in the 2000s, and could have begun the process of species extirpation; 2 of the 12 species targeted by fishers in the 1970s have not been captured recently (Moncayo-Estrada and Buelna-Osben Citation2001).
However, the majority of species that seem to have been lost from Lake Chapala have little direct economic value, and we believe that their potential disappearance was at most only indirectly related to fishery overexploitation, perhaps through by-catch. Some studies relate another indirect effect in the case of Lampetra spadicea to the reduction in size of large species that were the host of this parasitic fish (Lyons et al. Citation1994). We hypothesize that species reduction has been mainly caused by the combined effects of environmental degradation and the establishment of many exotic species.
Exotic fish species were present in Lake Chapala long before the 1970s. Common carp (Cyprinus carpio) and largemouth (black) bass (Micropterus salmoides) were introduced in the 1920s and 1930s, respectively (Cuesta-Terron Citation1925, de Buen 1946). However, over the last 30 years the number of exotic species has increased greatly, and their populations have been maintained by regular addition of juveniles (i.e., 850,000 carp fry were introduced in 1997; Perez et al. 1998). As consequence, exotic species now dominate the fishery and the fish community in the lake. The introduction of blue tilapia (Oreochromis aureus) in the 1970s is particularly significant. This species is one of the most competitive invaders because of its parental care and territoriality (FAO 1996), and it has the potential to alter the population dynamics of many fish species (Mair Citation2003).
Tilapia is now one of the ecologically dominant fishes in Lake Chapala. Given their biological characteristics and huge increase in abundance, we think that tilapia have had an impact on the native fish populations. For example, introduced Oreochromis aureus can literally occupy all available habitats with their spawning sites and exclude native fish from prime breeding grounds with severe consequences (Canonico et al. Citation2005). Trophic interactions are also important; tilapias consume the eggs and larvae of other fish species, and even small fishes (Arthington and Blühdorn Citation1995). In other tropical lakes, the feeding habits of this species have eliminated all submerged and floating aquatic macrophytes, which coincided with significant declines in populations of common and abundant native fish species (McKaye et al. Citation1995). Nonetheless, direct effects of tilapia are almost impossible to determine because of the myriad of other changes that have also occurred in the lake during the same period.
Additional and important biological threats, exotic parasites, were introduced along with exotic fishes, and these parasites have been increasing since the 1970s. In 1968, the cestod tapeworm Bothriocephalus acheilognathi was found in the herbivorous carp (Ctenopharingodon idellus; Lopez-Jimenez 1981). A survey of the fishery of Lake Chapala in the 1980s reported the presence of 6 exotic parasites in native fishes: Bothriocephalus acheilognathi, Ligula intestinalis, Myzobdella lugubris, Lernaea cyprinacea, and Clinostomum sp. (Olmos Citation1992).
Implications for monitoring and management
It is impossible to assign a single specific factor as the primary cause of the decline in IBI scores through the decades. Unfortunately, the degradation we have documented in Lake Chapala results from a combination of meteorological events (droughts) and the 6 categories of shallow lakes human impacts, both in the lake and in its catchment: (1) induced volume change, (2) enrichment, (3) toxins, (4) overfishing, (5) introduction of exotic species, and (6) physical habitat alteration. The fish community was a recipient of these impacts, as evidenced by the disappearance of many native species, reduced abundances of the remaining native species, increased numbers and abundance of exotic species, and a decrease in the sizes of large carnivores. Each of these community changes is a symptom (and exotic species are likely also a cause) of the rapid and alarming decline in ecosystem health of Lake Chapala.
The semiquantitative lake IBI provided a valuable framework and ecological benchmark for evaluating the long-term (decadal) biological community interactions, all responses to environmental degradation brought about by a combination of interacting anthropogenic forces, especially agriculture, urbanization, industry, and exotic species introductions. Given the importance of these forces as driving variables for lake water quality and quantity and overall biotic community structure and function elsewhere in Mexico, the semiquantitative lake IBI is likely a useful tool for detecting trends in ecosystem health in other lakes of Mexico and beyond (Lammert and Allan Citation1999, Lyons et al. Citation2000). Agriculture is the principal economic activity in central Mexico and covers the majority of the watersheds of most natural lakes and reservoirs (de Leon et al. 2001). Mexican lakes have experienced greatly decreased storage volumes over the past 40 years, and population density and demand for water is growing rapidly (Alcocer Citation2002). Additionally, historical information to estimate the changing composition of the fish community is available for many central Mexican lakes.
Semiquantitative IBIs similar in structure to the Mexican lake IBI would likely also be useful in other tropical regions where data on the biological community, although often voluminous when considered collectively, are scattered, have not been collected or organized in a standardized framework, and may not always be completely quantitative. A semiquantitative IBI is built specifically to accept such data and focuses on robust community measures and scoring criteria that are relatively insensitive to the type and amount of sampling effort. Such data are typically imprecise and often incomplete, so the ability of a semiquantitative IBI to detect or track short-term or subtle changes in the community is limited. Inferences from semiquantitative IBIs improve as the amount and variety of data included is increased, so they are best applied over long-time frames, such as decades in this case, and broad spatial areas, such as an entire large lake as in this case.
In addition to documenting broad-scale change and detecting long-term trends, another potential use of semiquantitative IBIs is to guide management activities. The decline in quality of an ecosystem is often readily apparent, but the exact nature of the degradation and the steps necessary for mitigation or restoration may be unclear. The IBI provides a specific biological framework in which specific aspects of ecosystem degradation can be elucidated, management strategies for mitigation or restoration can be formulated and applied, and the success of the application of these strategies can be evaluated. For example, in Lake Chapala, one important type of ecosystem degradation has been the decline of native species, mainly related to habitat quality reductions from a combined effect of loss of nearshore areas because of lake volume reduction and water quality deterioration.
Based on our IBI analysis, it is important to establish a minimum lake volume for maintenance of biotic integrity. One specific criterion for TDS concentration was established previously with a minimum mean depth of 5 m and 4644.50 Mm3. According to the present analysis, a similar value of 4.6 m (4409.79 Mm3) represents a critical water level to maintain biotic integrity (related to the 1980s volume). This can be achieved only to the extent that Lake Chapala is given priority in the water management system and that improvements are made in water use related to agricultural, urban, and industrial sectors. Also important is the recognition that water quality degradation in the lake environment must be changed through mandated controls on phosphorus and nitrogen loading, imposing a target level by wastewater treatment in the immediate watershed. In addition, restoration of vegetated littoral zones could help in the absorption and transformation of such nutrients, as well as provide habitat regeneration for native small species (Crisman et al. Citation2005).
A different indicator of reduced lake health is the decline of top predators, so a logical restoration strategy for the lake would be to increase native top predators. This could be achieved through a combination of more stringent fisheries regulations in cooperation with fishers, applied research on top predator life history and ecology, and aquaculture activities to raise and stock native top predators to enhance populations. All 3 of these approaches have been undertaken in Lake Chapala, and one benchmark of restoration success for the lake will be the extent to which native top predators increase during the coming decade. Restoration of Lake Chapala's fish community has often been considered a signal of a healthy lake ecosystem. Reestablishment of the native species in the lake would satisfy those who desire an ecosystem that supports a self-reproducing, diverse fish community, enhanced food web, and energy partitioning in different habitats.
Environmental change associated with biological “pollutants” such as invasive species is likely irreversible. Once established, exotics rarely disappear, although their role in the food web may change significantly with time (Mills et al. Citation2003). When the introduced species become overwhelmingly successful in their adapted environment, as in Lake Chapala, they can outcompete and exclude the native species and promote shifts in the structure and trophic organization of communities (Kennish Citation2005). A direct population control through specific fishing effort, according to morphological and behavioral characteristics of the species, is an alternative approach to reduce exotic species impacts.
Conservation of Lake Chapala is of great importance because it represents historically a center of adaptive radiation of fish species and recently was declared an Internationally Important Wetland by the Ramsar Convention (Alvarez del Villar 1970, Global Nature Fund 2010). Additionally, the lake controls the regional climate and integrates several critical elements related to water use and demand (Lind and Davalos-Lind Citation2002). Unfortunately, important impacts have already affected this ecosystem, such as the drying of the adjacent wetlands while modifying the transformation and storage of watershed exports (Chavez Citation1973). We expect that the implementation of different restoration strategies, monitored by the increase on the IBI value, will promote the health and biotic integrity of the lake, implementing horizontal management as a critical component in the rehabilitation process (Crisman et al. Citation2005).
Acknowledgments
We thank J. Morales-Castellanos for extremely helpful support in field work. The research upon which this paper is based was supported by the Bob Gardner Memorial Grant Award and the Jack and Norma Folmar Endowment Fund Research Award from Baylor University, and The Explorers Club Research Award to R. Moncayo-Estrada. Rodrigo Moncayo-Estrada and Carlos Escalera-Gallardo are supported by the Comisión de Operación y Fomento de Actividades Académicas (COFAA) and Estímulo al Desempeño Académico (EDI).
References
- Alcocer , J. 2002 . Surface water- major challenges in Mexico . LakeLine , 22 : 28 – 31 .
- Alvarez del Villar , J. 1970 . Peces mexicanos (claves) , Mexico City , , Mexico : Instituto Nacional de Investigaciones Biológico-Pesqueras y Comisión Nacional de Pesca; Secretaría de Industria y Comercio .
- Aparicio , J. 2001 . “ Hydrology of the watershed ” . In The Lerma-Chapala Watershed. Evaluation and Management , Edited by: Hansen , A M and van Afferden , M . 3 – 30 . New York (NY) : Kluwer Academic Press .
- Arthington , A H and Blühdorn , D R . 1995 . Improved management of exotic aquatic fauna: R&D for Australian rivers . LWRRDC Occasional Paper No. 4/95
- Bence , J R , Madenjian , C P , Rutherford , E S , Fahnenstiel , G L , Lavis , D S , Johengen , T H , Robertson , D M , Nalepa , T F , Jude , D J Vanderploeg , H A . 2002 . Dynamics of the Lake Michigan food web, 1970–2000 . Can J Fish Aquat Sci. , 59 : 736 – 753 .
- Bronte , C R , Ebener , M P , Schreiner , D R , DeVault , D S , Petzold , M M , Jensen , D A , Richards , C and Lozano , S J . 2003 . Fish community change in Lake Superior, 1970–2000 . Can J Fish Aquat Sci. , 60 : 1552 – 1574 .
- Canonico , G C , Arthington , A , McCrary , J K and Thieme , M L . 2005 . The effects of introduced tilapias on native biodiversity . Aquatic Conserv. , 15 : 463 – 483 .
- Chavez , EA. 1973 . Datos hidrobiologicos del lago de Chapala . Rev Soc Mex Historia Natl , XXXIV : 125 – 146 .
- Cloter , H , Mazari-Hiriiart , M and de Anda , J. 2007 . Atlas de la cuenca Lerma-Chapala. Construyendo una visión conjunta , Mexico City , , Mexico : Instituto Nacional de Ecología, Secretaría de Medio Ambiente y Recursos naturales, Universidad Autónoma de México, Instituto de Ecología .
- [CEA] Comisión Estatal del Agua de Jalisco . 2010 . Lago de Chapala; Guadalajara Jalisco , , Mexico [cited 17 Dec 2010]. Available from http://www.ceajalisco.gob.mx/chapala.html
- [CONAPESCA] Comisión Nacional de Acuacultura y Pesca . 2001 . Anuario estadístico de pesca 2001 Mexico City , , Mexico [cited 2 May 2008]. Available from http://www.conapesca.sagarpa.gob.mx/wb/cona/cona_anuario_estadistico_de_pesca
- Crisman , T L , Mitraki , C and Zalidis , G . 2005 . Integrating vertical and horizontal approaches for management of shallow lakes and wetlands . Ecol Eng. , 24 : 379 – 389 .
- Cuesta-Terron , C. 1925 . La fauna ictiológica y malacológica comestible del lago de Chapala, Jal., y su pesca . Mem Rev Soc Científica Antonio Alzate , 44 : 39 – 67 .
- de Anda , J , Quiñones-Cisneros , S E , French , R H and Guzmán , A M . 1998 . Hydrologic balance of Lake Chapala (Mexico) . J Am Water Resour As , 34 : 1319 – 1331 .
- de Anda , J and Shear , H . 2001 . “ Nutrients and eutrophication in Lake Chapala ” . In The Lerma-Chapala watershed. Evaluation and management , Edited by: Hansen , A M and van Afferden , M . 183 – 198 . New York (NY) : Kluwer Academic Press .
- de Anda , J , Shear , H , Maniak , U and Zárate-del Valle , P F . 2004 . Solids distribution in Lake Chapala, Mexico . J Am Water Resour As. , 40 : 97 – 109 .
- de Buen , F . 1946 . Investigaciones sobre ictiología mexicana III. La ictiofauna del lago de Chapala con descripción de una nueva especie. (Haustor ochoterenai De Buen) . Anal Inst Biol Univ Nac, Mexico , 17 : 261 – 281 .
- de Leon , B , Medina-Mendoza , R and Gonzalez-Casillas , A . 2001 . “ Natural resources management in the Lerma-Chapala Basin ” . In The Lerma-Chapala watershed. Evaluation and management , Edited by: Hansen , A M and van Afferden , M . 59 – 92 . New York (NY) : Kluwer Academic Press .
- Filonov , A E , Tereshchenko , I E and Monzon , C O . 2001 . “ Hydro-meteorology of Lake Chapala ” . In The Lerma-Chapala Watershed. Evaluation and management , Edited by: Hansen , A M and van Afferden , M . 151 – 182 . New York (NY) : Kluwer Academic Press .
- [FAO] Food and Agriculture Organization of the United Nations . 1996 . : 350 Precautionary approach to capture fisheries and species introduction. Rome: FAO Fisheries Technical Paper No
- Ford , T E , Ika , R , Shine , J P , Davalos-Lind , L and Lind , O T . 2000 . Trace metal concentrations in Chirostoma spp. From Lake Chapala, Mexico: Elevated concentrations of mercury and public health implications . J Environ Sci Heal A , 35 : 313 – 325 .
- Global Nature Fund. 2010. Lake Chapala, Mexico; [cited 17 Dec 2010]. Available from http://www.globalnature.org/25800/LIVING-LAKES/America/Lake-Chapala/February-2007/02_vorlage.asp.
- Guzman , AM. 1995 . La pesca en el lago de Chapala: Hacia su ordenamiento y explotación racional , Guadalajara (Mexico) : Universidad de Guadalajara y Comisión Nacional del Agua .
- Guzman , AM. 2002 . “ El lago de Chapala ” . In Lagos y presas de México , Edited by: de la Lanza-Espino , G and García-Calderón , J L . 129 – 145 . Mexico City (Mexico) : Centro de Ecología y Desarrollo, A. C .
- [INEGI] Instituto Nacional de Estadística y Geografía . 2005 . Conteo de Población y Vivienda 2005 Mexico City , , Mexico [cited 2 May 2008]. Available from http://www.inegi.org.mx/est/contenidos/proyectos/ccpv/cpv2005/Default.aspx
- Jay , J A and Ford , T M . 2001 . “ Bioaccumulation, and human helath implications of heavy metals ” . In The Lerma-Chapala watershed. Evaluation and management , Edited by: Hansen , A M and van Afferden , M . 123 – 136 . New York (NY) : Kluwer Academic Press .
- Jordan , DS and Evermann , BW . 1896 . The fishes of North and Middle America: A descriptive catalogue of the species of fish-like vertebrates found in the waters of North America, north of the Isthmus of Panama , Washington , DC : GPO .
- Karr , JR. 1981 . Assessment of biotic integrity using fish communities . Fisheries , 6 : 21 – 27 .
- Karr , JR and Chu , EW . 1999 . Restoring life in running waters: Better biological monitoring , Washington (DC) : Island Press .
- Karr , J R , Yant , P R , Fausch , K D and Schlosser , I J . 1987 . Spatial and temporal variability of the Index of Biotic Integrity in three mid-western streams . T Am Fish Soc. , 116 : 1 – 11 .
- Kennish , MJ. 2005 . “ Estuaries, anthropogenic impacts ” . In Encyclopedia of Coastal Science Developed Coasts Edited by: Jackson , N L and Nordstrom , K F . 434 – 436 .
- Lammert , J and Allan , D . 1999 . Assessing biotic integrity of streams: Effects of scale in measuring the influence of land use/cover and habitat structure on fish and macroinvertebrates . Environ Manage. , 23 : 257 – 270 .
- Lind , O T and Davalos-Lind , L . 2002 . Interaction of water quantity with water quality: The Lake Chapala example . Hydrobiologia. , 467 : 159 – 167 .
- Lind , O T , Doyle , R , Vodopich , D S , Trotter , B G , Gualberto-Limon , J and Davalos-Lind , L . 1992 . Clay turbidity: Regulation of phytoplankton production in a large, nutrient-rich tropical lake . Limnol Oceanogr. , 37 : 549 – 565 .
- Lopez-Jimenez , S. 1981 . Cestodos de peces I. Bothriocephalus (Clestobothrium) acheilognathi (Cestoda: Bothriocephalidae) . An Inst Biol Univ Nac Auton Mex Ser Zool , 51 : 69 – 84 .
- Lyons , J , Cochran , P A , Polaco , O J and Merino-Nambo , E . 1994 . Distribution and abundance of the Mexican lampreys (Petromyzontidae; Lampetra, subgenus Tetrapleurodon) . Southwest Nat. , 39 : 105 – 113 .
- Lyons , J , Gutiérrez-Hernández , A , Díaz-Pardo , E , Soto-Galera , E , Medina-Nava , M and Pineda-López , R . 2000 . Development of a preliminary index of biotic integrity (IBI) based on fish assemblages to assess ecosystem condition in the lakes of central Mexico . Hydrobiologia. , 418 : 57 – 72 .
- Mair , G. 2003 . Tilapia: A species for Indian aquaculture? . Aquacult Asia , VIII : 22 – 23 .
- McKaye , K R , Ryan , J D , Stauffer , J R Jr , Lopez Perez , L J , Vega , G I and van den Berghe , E P . 1995 . African tilapia in Lake Nicaragua—ecosystem in transition . BioScience. , 45 : 406 – 411 .
- Mercado-Silva , N , Lyons , J , Díaz-Pardo , E , Gutiérrez-Hernández , A , Pedraza-Lara , C , Ornelas-García , C P and Vander Zanden , M J . 2006 . Long-term changes in the fish assemblage of the Laja River, Guanajuato, central Mexico . Aquat Conserv. , 16 : 533 – 546 .
- Mestre , JE. 1997 . “ Case Study VIII - Lerma-Chapala Basin, Mexico ” . In Water pollution control - A guide to the use of water quality management , Edited by: Richard Helmer , R and Hespanhol , I . London (UK) : Thomson Professional .
- Metcalfe , SE. 1995 . Holocene environmental change in the Zacapu Basin, Mexico: a diatom-based record . Holocene. , 5 : 196 – 208 .
- Miller , DL , Leonard , PM , Hughes , RM , Karr , JR , Moyle , PB , Schrader , LH , Thompson , BA , Daniels , RA , Fausch , KD , Fitzhugh , GA , Gammon , JR , Halliwell , DB , Angermeier , PL and Orth , DJ . 1988 . Regional applications of an index of biotic integrity for use in water resource management . Fisheries , 13 : 12 – 20 .
- Mills , E L , Casselman , J M , Dermott , R , Fitzsimons , J D , Gal , G , Holeck , K T , Hoyle , J A , Johannsson , O E , Lantry , B F Makarewicz , J C . 2003 . Lake Ontario: food web dynamics in a changing ecosystem (1970–2000) . Can J Fish Aquat Sci , 60 : 471 – 490 .
- Moncayo-Estrada , R and Buelna-Osben , H R . 2001 . “ Fish fauna of Lake Chapala. ” . In The Lerma-Chapala watershed. Evaluation and management , Edited by: Hansen , A M and van Afferden , M . 215 – 242 . New York (NY) : Kluwer Academic Press .
- Moncayo-Estrada , R , Escalera-Gallardo , C and Segura-Garcia , V . 2003 . “ Los pescados blancos del lago de Chapala, caracteristicas generales ” . In Historia y avances del cultivo del pescado blanco , Edited by: Rojas-Carrillo , P and Fuentes-Castellanos , D . 51 – 78 . Mexico City (Mexico) : Instituto Nacional de la Pesca-SAGARPA .
- Olmos , ME. 1992 . Situacion actual y perspectivas de las pesquerias derivadas de la acuicultura en México . FAO-Programa Cooperativo Gubernamental. Documento de Campo No 1 GCP/RLA/102/ITA
- Orbe , A M , Hernandez , D and Acevedo , J . 2001 . Lago de Chapala . : 821 – 836 . Cisneros MA, Meléndez LF, Zarate E, Gaspar MT, Lopez L, Saucedo C, Tovar J, editors. Sustentabilidad y pesca responsable en Mexico: Evaluacion y manejo. Mexico City (Mexico): Instituto Nacional de la Pesca-SAGARPA
- Perez , M , Jara , E , Lopez , E and Arriaga , V M . 1998 . Sistema productivo, subsistema pesca. Guadalajara (México): Universidad de Guadalajara, Reporte Técnico No. 73
- Peterson , JT and Kwak , TS . 1999 . Modeling the effects of land use and climate change on riverine smallmonth bass . Ecol. Appl , 9 : 1391 – 1404 .
- [SARH] Sectretaría de Agricultura y Recursos Hidráulicos . 1981 . Batimetría de Lago de Chapala. Información en Dirección Lerma-Chapala-Santiago, CONAGUA . Secretaría de Recursos Hidráulicos. Guadalajara, México.
- [SEDUE] Secretaría de Desarrollo Urbano y Ecología . 1991 . Plan integral de desarrollo urbano de la ribera del lago de Chapala, Jal. (1ra. Etapa medio físico natural) , Guadalajara (México) : Gobierno del Estado de Jalisco .
- Shine , J P , Ryan , D K and Ford , T E . 1998 . Annual cycle of heavy metals in a tropical lake-Lake Chapala, Mexico . J Environ Sci Heal A. , 33 : 23 – 43 .
- Thielcke , G and Resch , J . 2001 . Living Lakes: a Survey of the Living Lakes of the world. Constanz (Germany): Stadler Verlag
- [U de G] Universidad de Guadalajara . 1983 . Lago de Chapala; investigación actualizada . Guadalajara (México): Instituto de Geografía y Estadística e Instituto de Astronomía y Meteorología
- Wester , P , Burton , M and Mestre-Rodríguez , E . 2001 . “ Managing the water transition in the Lerma-Chapala Basin, Mexico ” . In Intersectoral Management of River Basins. Proceedings of an International Workshop on Integrated Water Management in Water-Stressed River Basins in Developing Countries: Strategies for Poverty Alleviation and Agricultural Growth Edited by: Abernethy , C . 161 – 181 . Colombo (Sri Lanka) IWMI