Abstract
Fishes inhabiting shallow, glacial lakes of the Prairie Pothole Region in the United States and Canada periodically experience hypoxia in severe winters that can lead to extensive fish mortality resulting in high biomasses of dead fish. However, the role of carcass-derived nutrient subsidies in shallow, eutrophic lakes translocated to pelagic primary producers is not well documented. This study quantified the influence of winterkill events on nutrient contributions from decaying fish carcasses of common carp (Cyprinus carpio) and the phytoplankton response among pre- and postwinterkill years and compared seasonal patterns of nutrient limitation and phytoplankton community composition between winterkill and nonwinterkill lakes. We found that fish carcasses contributed an estimated 2.5–4.3 kg/ha of total (Kjeldahl) nitrogen (N) and 0.3–0.5 kg/ha of total phosphorus (P) to lakes that experienced winterkill conditions. Nutrient bioassays showed that winterkill lakes were primarily N limited, congruent with the low N:P ratios produced by fish carcasses corrected for the disproportionate release of N and P (8.6). Nutrient subsidies translocated from decomposed fish to pelagic primary producers seemed to have little immediate influence on the seasonal phytoplankton community composition, but total N and subsequent chlorophyll-a increased the year following the winterkill event. Cyanobacteria density varied seasonally but was higher in winterkill lakes, presumably due to the integration of nutrients released from fish decomposition. This study provides evidence that large inputs of autochthonous fish-derived nutrients contribute to nutrient availability within winterkilled systems and increase the maximum attainable biomass of the phytoplankton community.
Ecologists have long recognized the importance of nutrients derived from the marine environment and spatially translocated to oligotrophic streams and lakes via semelparous salmonids (Stockner and Ashley Citation2003, Schindler et al. Citation2005). These fish-derived nutrients support healthy ecosystem function by increasing productivity and biodiversity (Stockner and Ashley Citation2003). Increased nutrients have been linked to higher algal biomass and higher invertebrate abundances through a bottom-up effect on higher trophic levels (McQueen et al. Citation1986, Carpenter et al. Citation1992, Quamme and Slaney Citation2003).
Although the origin of the nutrients (allochthonous vs. autochthonous inputs) and productivity of the systems (oligotrophic vs. eutrophic) differ, a similar nutrient phenomenon occurs in lakes when nonmigratory fishes experience pulsed mortality imposed by either natural (e.g., winter or xsummerkill) or anthropogenic (e.g., lake renovation) conditions. Fertile, shallow lakes in the Northern Great Plains in the United States and Canada periodically experience winterkill conditions during prolonged periods of ice and snow cover. Hypoxia induced by a lack of mixing with the atmosphere and the reduction in photosynthetically active radiation due to persistent ice and snow cover can result in fish kills (Greenbank Citation1945, Cooper and Washburn Citation1949, Johnson Citation1965). Winterkills are major, natural perturbations that have both direct and indirect effects on food web functions and nutrient dynamics within these systems (Clark et al. Citation1991, Isermann et al. Citation2004, Tonn et al. Citation2004). A large portion of a lake's nutrient pool can be stored in fish biomass, and those nutrients become available to pelagic primary producers following a fish winterkill in the form of a nutrient subsidy (Kitchell et al. Citation1975, Citation1979, Parmenter and Lamarra Citation1991, Schindler et al. Citation1993, Schindler and Eby Citation1997). The term “subsidy” in this paper describes an autochthonous release of nutrients from fish carcasses to pelagic primary producers. Hypothetically, the magnitude of the phytoplankton response should be in concert with the percent increase in newly available nutrients translocated from decomposing fish carcasses but may differ with trophic state. Further, the rate of carcass decomposition, as influenced by water temperature, depth, and the presence of scavengers, may confound the interaction between carcass-derived nutrients and pelagic primary producers (Chidami and Amyot Citation2008, Premke et al. Citation2010). In addition, the rates of release during decomposition have been found to differ among individual nutrients (Parmenter and Lamarra Citation1991).
The abundance and diversity of phytoplankton can be limited by many factors including nutrient (phosphorus, nitrogen, and silicon) and light availability, while water temperature, turbidity, invertebrate grazing pressure, wind, macrophytes and the fish community can complicate the competitive interactions between phytoplankton taxa (Van Donk et al. Citation1993, Schindler and Eby Citation1997, Scheffer Citation1998). The shallow, wind-swept glacial lakes of the Prairie Pothole Region can be characterized as productive, turbid, nitrogen (N)-limited systems that therefore favor proliferation of cyanobacteria during summer (Raney Citation1991, Salm et al. Citation2009). Because most cyanobacteria are low-light tolerant, can fix N2, tolerate warm temperatures (optimum growth at >20 C), and experience low grazing pressure, they often outcompete and attain a higher biomass than green algae, particularly under N-limited conditions (Tilman et al. Citation1982, Tilman Citation1986, Sigee Citation2005). In comparison, other algae taxa usually dominate phytoplankton communities at cooler temperatures and when phosphorus (P) is limiting (Scheffer Citation1998, Sigee Citation2005). The ratio of available N:P resources can have an important influence on phytoplankton composition and abundance, and large nutrient fluxes may change the limiting nutrient depending on the relative changes to N:P availability (Tilman et al. Citation1982, Schindler et al. Citation1993, Schindler and Eby Citation1997, Scheffer Citation1998). The increased release rate of N from decomposing fish carcasses may increase N:P and influence the phytoplankton community composition because N, located in the soft tissues of fishes, is released at a faster rate and ultimately in greater quantity than P, which is bound in bones and scales (Parmenter and Lamarra Citation1991). Alternatively, large nutrient fluxes could affect the composition of the phytoplankton community without changing the limiting nutrient because pulsed nutrient availability has been shown to increase phytoplankton diversity (Sommer Citation1985, Grover Citation1988). The composition of the phytoplankton community could also be altered by a decrease of benthivorous fishes. Benthivorous fish transfer nutrients from the benthos into the water column via bioturbation and low N:P supply ratios from excretion (Schindler and Eby Citation1997, Schindler and Scheuerell Citation2002). Thus, termination of either mechanism could affect the phytoplankton community and induce a bottom-up effect on trophic functions.
Severe conditions during winter 1987–1988 and again during 2000–2001 resulted in extensive fish winterkills in shallow, eutrophic lakes across the Prairie Pothole Region, providing an opportunity to examine ecosystem responses to winterkill events. Therefore, our objectives were to (1) determine nutrient availability and the phytoplankton response in winterkilled lakes during the years prior to and following a winterkill event, (2) estimate the fish-derived nutrient contributions to winterkill lakes using the percent N and P composition and biomass of whole-body winterkilled common carp (Cyprinus carpio), (3) determine the extent of nutrient limitation by lake type (winterkill or nonwinterkill), and (4) compare temporal changes in phytoplankton community composition by lake type.
Methods
Study site
We sampled East and West Oakwood lakes in Brookings County, South Dakota, from January 1987 to October 1989 and from March to September 2001. We also sampled Brush and Sinai lakes from March to September 2001. During winters 1987–1988 and 2000–2001, East and West Oakwood lakes experienced fish kills whereas Brush and Sinai lakes did not winterkill (). Therefore, data collected from 2 winterkilled lakes from 1987 to 1989 allow comparisons among 1 year prewinterkill and 2 years postwinterkill. Data collected during 2001 were from 2 winterkill lakes and 2 nonwinterkill lakes, allowing comparisons between lake types. Winterkill conditions are relatively common in shallow prairie lakes and occur in East and West Oakwood lakes approximately once every 8–10 years (Isermann et al. Citation2004). Due to continuous wind mixing, all 4 study lakes are classified as polymictic. Common fishes present in the study lakes included black bullhead (Ameiurus melas), common carp, fathead minnow (Pimephales promelas), northern pike (Esox lucius), walleye (Sander vitreus), yellow perch (Perca flavescens), and white sucker (Catostomus commersonii).
Table 1 Physical characteristics of nonwinterkill (Sinai and Brush) and winterkill lakes (East Oakwood and West Oakwood) sampled in eastern South Dakota from March to September 2001. Dissolved oxygen levels were measured 0.5 m below the ice in March 2001. Chlorophyll-a and trophic state index (TSI) calculated using chlorophyll-a (Carlson Citation1977) were collected during August 2001.
Nutrient availability and phytoplankton response in winterkilled lakes
Nutrients and chlorophyll-a were evaluated from January 1987 to October 1989 on East and West Oakwood lakes to determine the influence of the 1987–1988 winterkill on nutrient availability and the phytoplankton response. Three seasonal cycles of total Kjeldahl nitrogen (TKN), total phosphorus (TP), dissolved inorganic nitrogen (DIN; sum of ammonium, nitrate and nitrite), and soluble reactive phosphorus (SRP) were evaluated from 22 January 1987 to 31 October 1989, and chlorophyll-a was evaluated from 6 July 1987 to 31 October 1989. A winterkill event occurred during winter 1987–1988, thus providing 1 year prewinterkill and 2 years postwinterkill. Duplicate nutrient samples were collected from the center of each lake basin at approximately 30 d intervals. Chlorophyll-a was collected from 2 stations in West Oakwood Lake and one station in East Oakwood Lake at approximately 30 d intervals. Chemical parameters were analyzed at the Olsen Biochemistry Laboratory, South Dakota State, within 24 h of collection, following Environmental Protection Agency methods (EPA Citation1983).
Fish biomass and fish-derived nutrient contributions
The abundance of common carp that washed ashore in East and West Oakwood lakes during 2001 following ice-out was estimated to quantify amounts of total N (TN) and TP that would result from decomposed fish carcasses. We used a stratified-random sampling design with 4 strata (lake quadrants) and 4–5 transects (range: 31–109 m) per strata to estimate the number and biomass of winterkilled fish in April 2001. All fish encountered on transects (the shoreline) were identified to species, counted, and measured for total length (TL, mm); however, due to high biomass (i.e., >90%) only common carp data were used for nutrient estimates. Weighted (strata) parameter estimates were calculated following procedures described by Thompson (Citation2002) and Krebs (Citation1999) to determine population abundance.
Accurate fish masses were not obtainable due to poor condition of dead and decaying fish; therefore, we developed a predictive mass–length equation to estimate fish mass (g) for each 2.54 cm length class. Because mass–length relationships were not known for these winterkilled common carp populations, we calculated a regression equation from common carp data provided in Bister et al. (Citation2000). We used the 50th percentile of mean fish mass in each 2.54 cm length interval and regressed these means on TL to estimate the relationship. We used the 50th percentile instead of the 75th percentile used by Bister et al. (Citation2000) to minimize bias and avoid over- or underestimation of biomass and prediction of fish-derived nutrient release. The resulting equation was
where L is the total length of the common carp in mm and W is the fish mass in g. The biomass estimate for each winterkilled population was calculated by summing the estimated mass for each length frequency, which was weighted by the percent composition of the surveyed population for each length class.
TN and TP contribution from decaying fish (kg/ha) were quantified using mean percent N and P values derived from common carp taken from Lake Madison, South Dakota. We randomly selected 2 common carp from each gender per 100 mm length group ranging from 500 to 900 mm for analysis. Whole common carp were ground, homogenized, and total percent N (AOAC procedure 992.15) and P (AOAC procedures 968.08; 935.13; 931.01 Modified) were determined. Fish-derived nutrient contributions to winterkilled lakes were then calculated by multiplying the estimated common carp biomass by the mean percent N and P composition of whole-body common carp. Estimates of fish-derived nutrient contributions were corrected for lake surface area and the disproportionate release of N (95%, calculated as the percent released from the original carcass mass) to P (60%) using literature values (Parmenter and Lamarra Citation1991). We then compared the estimated fish-derived nutrients to each winterkilled lake's mean, prewinterkill nutrient standing stock estimate (i.e., 1987 mean TKN or TP*lake volume) to determine the potential percent increase of nutrients that could be attributed to common carp following winterkill events.
Nutrient limitation
Bioassays were used to evaluate seasonal patterns of nutrient limitation in winterkilled and nonwinterkilled lakes. We filled 12 cubitainers with 1 L of lake water collected at a depth of 0.5 m from each lake at approximately 30 d intervals from April to September 2001. For each lake, 3 cubitainers were spiked with N solution, 3 with P solution, and 3 with a solution of both N and P (NP; to approximate the Redfield ratio of 7:1); 3 water samples were not spiked with nutrients and served as controls (Kitchell et al. Citation1999). To create the N treatments, we added 1 mL of the N solution (580.1 mg of N per L in the form of NH4NO3) to 1 L of lake water. To create the P treatments, we added 1 mL of the P solution (81.02 mg of P per L in the form of Na2HPO4 ·7H2O) to 1 L of lake water. Combinations of N and P were accomplished by adding 1 mL of both N and P solution to 1 L water samples in the cubitainers. All 48 cubitainers were incubated outside in a water bath for 72 h under ambient light and temperature (mean monthly high temperature ranged from 1 to 29 C during the experiment) and then 250 mL of water from each cubitainer was filtered and analyzed for chlorophyll-a using standard fluorometric techniques (Carpenter and Kitchell Citation1993). Relative chlorophyll-a was expressed as:
where ChlaT is the chlorophyll-a concentration of the treatment and ChlaC is the average chlorophyll-a concentration of the 3 control treatments for that time period and study lake.
Phytoplankton composition
A composite phytoplankton sample was collected from the central basin of each winterkilled and nonwinterkilled lake at approximately 30 d intervals from March to September 2001 using 250 mL Nalgene bottles lowered to a depth 0.5 m. All phytoplankton samples were fixed in 5% Lugols solution stored at 4 C, and analyzed to species and grouped taxonomically using standard methods (APHA Citation1995, Method 10200F.2.C.1). Five phytoplankton taxa (green [Chlorophyceae], blue-green [cyanobacteria], cryptomonad [Cryptophyceae], diatom [Bacillariophyceae], and golden [Chrysophyceae] algae) were used to describe seasonal phytoplankton composition.
Statistical analysis
An analysis of covariance (ANCOVA) was used for comparisons of common carp percent total N and P between genders (categorical) and with fish mass (covariate). Nutrient limitation was evaluated using a repeated measure analysis of variance (ANOVA) within lake type for comparisons among treatments (N, P, NP) and sampling periods. Differences in relative chlorophyll-a values between lake types (winterkill or nonwinterkill) were evaluated using repeated measure ANOVAs within each treatment (N, P, NP) for comparisons between lake types and among sampling periods. Repeated measure ANOVAs were also used for comparisons of algal densities between lake types and among sampling periods. All analyses were performed using SAS (version 9.2), and significance was set at 0.10 (Littell et al. Citation1996).
Results
Nutrient availability and phytoplankton response in winterkilled lakes
Nutrient and chlorophyll-a concentrations within East and West Oakwood lakes were influenced by the winterkill event of 1987–1988. In general, nutrients and chlorophyll-a increased the year immediately following the winterkill but decreased during the second year following the winterkill event. For example, TKN measured in March was greater following the winterkill compared to 1 year prior, increasing from 1.27 to 2.03 mg/L on East Oakwood Lake and from 1.63 to 3.90 mg/L on West Oakwood Lake (). Measures of TP also increased from 0.09 to 0.11 mg/L on East Oakwood Lake and from 0.12 to 0.18 mg/L on West Oakwood Lake over the same time period (). The highest concentrations of SRP during the 3-year study period were observed during the winterkill in March1988 (0.065 mg/L) in East Oakwood Lake and (0.155 mg/L) in West Oakwood Lake (Fig. 2). Peak concentrations of DIN were also observed in West Oakwood and East Oakwood lakes (2.38 and 0.875 mg/L, respectively) in March 1988 compared to undetectable levels the year prior (Fig. 2). Peak chorophyll-a also increased from 1987 to 1988 from 176 to 339 μg/L on East Oakwood and from 183 to 338 μg/L on West Oakwood Lake ().
Figure 1 Mean total Kjeldahl nitrogen (above) and total phosphorus (below) collected from West Oakwood Lake (solid line) and East Oakwook Lake (dashed line) from 22 January 1987 to 31 October 1989. A fish winterkill occurred in both lakes during winter 1987–1988. Vertical dashed bar represent the approximate date of winterkill. Error bars represent standard error.
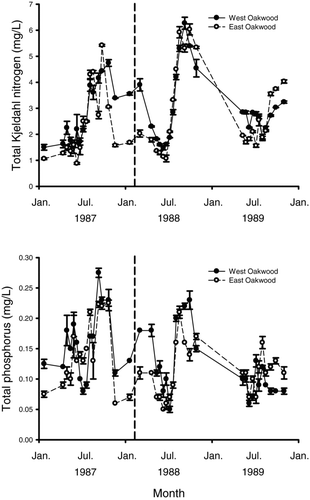
Figure 2 Mean dissolved inorganic nitrogen (above) and soluble reactive phosphorus (below) collected from West Oakwood Lake (solid line) and East Oakwook Lake (dashed line) from 22 January 1987 to 31 October 1989. A fish winterkill occurred in both lakes during winter 1987–1988. Vertical dashed bar represent the approximate date of winterkill. Error bars represent standard error.
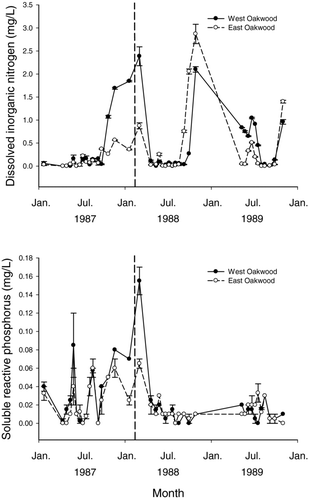
Figure 3 Mean chlorophyll-a concentrations collected from West Oakwood Lake (solid line) and East Oakwook Lake (dashed line) from 6 July 1987 to 31 October 1989. A fish winterkill occurred in both lakes during winter 1987–1988. Vertical dashed bar represent the approximate date of winterkill. Error bars represent standard error.
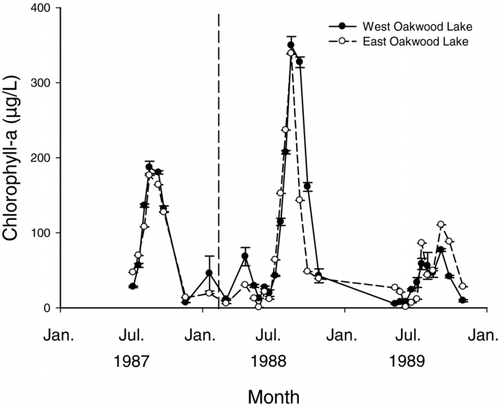
Fish biomass and nutrient availability
A total of 7.6% (1260 of 16,538 m) and 5.4% (1186 of 21,788 m) of the shoreline was sampled on East Oakwood Lake and West Oakwood Lake, respectively. The majority of fish encountered during postwinterkill shoreline surveys were common carp, which represent 99% of dead fish (3042/3086) in East Oakwood Lake and 90% (1963/2180) in West Oakwood Lake. Other winterkilled species included black bullhead, northern pike, walleye, white sucker, and yellow perch. The estimated wet weight biomass of winterkilled common carp was 177.0 kg/ha in East Oakwood Lake and 103.0 kg/ha in West Oakwood Lake ().
The wet mass of common carp analyzed for nutrient composition (n = 12) ranged from 1805 to 3802 g for males and from 2668 to 8697 g for females (). Total percent N did not differ between sexes (F 1, 9 = 1.17, P = 0.307) or vary with fish wet mass (F 1, 10 = 0.58, P = 0.462). Similarly, total percent P did not differ between sexes (F 1, 9 = 0.93, P = 0.361) or with fish wet mass (F 1, 10 = 0.14, P = 0.720). Therefore, total percent N (2.52, SE = 0.07) and P (0.45, SE = 0.02) were averaged across sexes and mass. The ratio of the average percent N to percent P (N:P ratio) from common carp was 5.57. Nutrient estimates bound in common carp prior to winterkill were 4.5 kg/ha of N and 0.8 kg/ha of P in East Oakwood Lake and 2.6 kg/ha of N and 0.5 kg/ha of P in West Oakwood Lake (). Using rates published by Parmenter and Lamarra (Citation1991), the release of nutrients into the water column was estimated to be 1731 kg (4.3 kg/ha) of N and 194 kg (0.5 kg/ha) of P to East Oakwood Lake and 1186 kg (2.5 kg/ha) of N and 144 kg (0.3 kg/ha) of P to West Oakwood Lake providing an estimated N:P ratio of 8.6 (). Release of nutrients due to decomposition of winterkilled common carp were estimated to increase TN by about 5–12% and TP by about 10–23% in West Oakwood and East Oakwood lakes, respectively, compared to prewinterkill nutrient conditions.
Table 2 Mean count of common carp per 100 m transect (with 90% confidence limits), population abundance (with 90% confidence limits) and biomass estimates of winterkilled common carp used to calculate N and P subsidies in East and West Oakwood lakes following the winterkill of 2000–2001.
Nutrient limitation
Bioassays showed that phytoplankton biomass (i.e., chlorophyll-a values) was not nutrient limited for winterkilled lakes with fish-derived nutrient subsides while nonwinterkilled lakes were still limited by N availability and, to a lesser extent, co-limited by N and P (). For winterkilled lakes, seasonal patterns in chlorophyll-a varied significantly over time (F 5, 134 = 31.78, P < 0.001) but did not vary among treatments (F 3, 134 = 0.09, P = 0.967). Seasonal patterns in chlorophyll-a for nonwinterkilled lakes varied significantly among treatments (treatment*month interaction: F 15, 119 = 1.63, P = 0.076) with the highest phytoplankton biomass observed for treatments that included N (i.e., N and NP). P was most limited in July and September ().
Relative increases in phytoplankton biomass were significantly greater in the nonwinterkilled lakes than the winterkilled lakes for all 3 bioassay treatments (). For the NP treatments, seasonal patterns in chlorophyll-a differed significantly between winterkilled and nonwinterkilled lakes, with chlorophyll-a values in nonwinterkilled lakes exceeding those of the winterkilled lakes (type × month interaction F 5, 60 = 6.47, P < 0.001). Similarly, seasonal patterns in chlorophyll-a for the N treatment differed significantly between winterkilled and nonwinterkilled lakes with values in nonwinterkilled lakes exceeding those of the winterkilled lakes (type × month interaction F 5, 60 = 6.62, P < 0.001). Seasonal patterns in chlorophyll-a for the P treatment also differed significantly between winterkilled and nonwinterkilled lakes, with values in nonwinterkilled lakes exceeding those of the winterkilled lakes (type × month interaction F 5, 59 = 10.47, P < 0.001).
Table 3 Mean fish wet mass and total length (standard errors in parentheses) of female and male common carp analyzed for percent N and P. Total percent N and P did not differ between sexes or with fish mass; therefore, total percent N and P were averaged across sexes and masses.
Table 4 Mean 1987 prewinterkill nutrient standing stock estimates for N and P and the potential percent increase of N and P that could be attributed to common carp following winterkill event of 2001 in East and West Oakwood lakes. Estimates of carp-derived nutrient contributions were calculated as the nutrient subsidies of N or P from common carp divided by the prewinterkill nutrient standing stock estimate (i.e., 1987 mean TKN or TP*lake volume).
Phytoplankton composition
Seasonal patterns in chlorophyll-a concentrations varied significantly between winterkilled and nonwinterkilled lakes (lake type × month interaction: F 5, 12 = 4.64, P = 0.014), with significantly greater chlorophyll-a concentrations in winterkill lakes during March and April 2001 (). Green algae density was not significantly different between lake types or among sampling periods (). Cyanobacteria densities were significantly higher in winterkilled lakes than in nonwinterkill lakes and differed among sampling periods, with August and September exhibiting greater densities than other sampling periods (). Cryptomonad density was not significantly different between lake type but did differ among sampling periods, with April exhibiting greater densities than other sampling periods (). Diatom density did not differ between lake type or among sampling periods (). Similarly, chrysophyte density did not differ between lake type or among sampling periods ().
Discussion
Nutrient subsidies from decaying fish seemed to have little influence on the seasonal phytoplankton community composition but may have increased maximum phytoplankton biomass attainable (i.e., increased the upper phytoplankton biomass limit) in winterkill lakes (Scheffer Citation1998). Nutrient release from decaying fish may be responsible for the high nutrient availability and subsequent increase in chlorophyll-a and cyanobacteria density that occurred following the winterkills. Increased cyanobacteria density within winterkilled lakes can likely be explained by fish-derived nutrients; previous studies have found concentrations of total N or P to be more correlated with cyanobacteria biomass than the N:P ratio (Downing et al. Citation2001). Because nutrients and other limiting factors (e.g., light intensity) impose an upper limit to phytoplankton biomass (Scheffer Citation1998), the presence of fish-derived nutrient subsidies likely explain the higher nutrient availability and phytoplankton biomass the year following each winterkill. High levels of DIN were observed during winter 1987–1988 due to elevated levels of ammonia, likely caused initially by the decomposition of dead plant material then by animal carcasses from the winterkill. Similarly, SRP was elevated to peak levels during winter 1987–1988, likely because of fish-derived nutrients. In addition to fish-derived nutrients, a portion of the increase in SRP may have been caused by sediment release resulting from anoxic conditions, although the extent and duration of anoxic conditions at the sediment–water interface were not known. Chlorophyll-a peaked during August or early September during each year from 1987 to 1998 and was highest during the postwinterkill year of 1988. Similarly, a higher concentration of chlorophyll-a was observed in the winterkill lakes than the nonwinterkill lakes following the winterkill of 2001.
Figure 5 Mean chlorophyll-a concentrations collected from winterkilled (solid line) and nonwinterkilled lakes (dashed line) from March to September 2001. Error bars represent standard error.
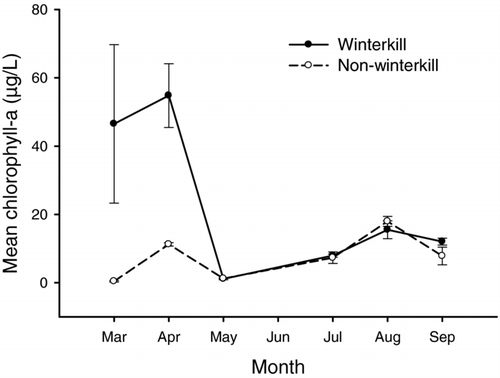
In the absence of bioturbation due to winterkill, a myriad of factors can influence P sediment release. Most noticeably, as dissolved oxygen concentrations decrease, the P sediment release rate will increase (Hupfer and Lewandowski Citation2008), yet cold water temperatures reduce P sediment release (Anderson and Ring Citation1999, Hupfer and Lewandowski Citation2008); therefore, an antagonistic relationship exists between cold water temperatures and anoxic concentrations of oxygen present under winterkill conditions. Comparing cold, anoxic conditions found in other water bodies to our study lakes may elucidate the impact of carcass-derived nutrients. Dissolved reactive phosphorus (DRP) was found to be 0.002 mg/L during March when water temperatures were 3 C and dissolved oxygen was <1 mg/L in Lake Minocqua, Wisconsin (Holden and Armstrong Citation1980). One hypothesis is that carcass-derived nutrients account for the majority of the soluble P during winterkill conditions if similarly low rates of sediment P release were assumed to be found in our study lakes during cold, anoxic conditions without winterkill. Further research is needed to adequately elucidate the relative contributions of carcass-derived nutrients and P sediment release under cold, anoxic conditions.
Table 5 Test statistics for comparisons of phytoplankton taxa densities between lake types (winterkill or nonwinterkill) and among sampling periods (Month) evaluated using repeated measure ANOVAs.
The trophic cascade hypothesis would predict that algal density would decrease following a winterkill because the reduction in abundance of planktivorous fish would allow the abundance of zooplankton to increase, therefore decreasing algal biomass (Carpenter et al. Citation1985). This scenario seemed valid during 1988 when chlorophyll-a remained low in the months following the winterkill of 1987–1988 until August. Conversely, chlorophyll-a was high during March and April 2001 and greater in winterkill than nonwinterkill lakes following the winterkill of 2000–2001. Cladoceran density
Figure 6 Above: Mean green algal biomass in winterkilled (solid line) and nonwinterkilled lakes (dashed line) sampled from March to September 2001. Below: Average cyanobacteria biomass in winterkilled (solid line) and nonwinterkilled (dashed line) lakes sampled from March to September 2001. Error bars represent standard error.
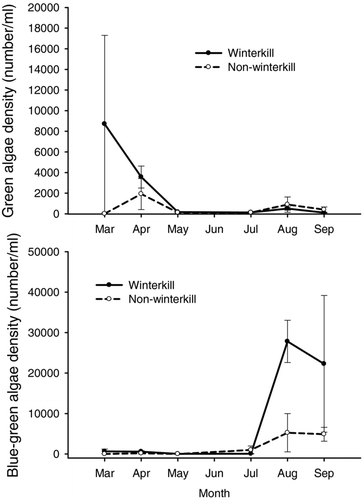
was lower in winterkilled lakes during 2001 due to overwinter mortality of zooplankton from extreme winterkill conditions (Isermann et al. Citation2004), a condition not observed during the 1987–1988 winterkill (Raney Citation1991). Therefore, a combination of inputs of fish-derived nutrients and overwinter mortality of zooplankton likely created a period of grazing release during spring 2001 (Isermann et al. Citation2004), allowing algal biomass to increase following the winterkill of 2000–2001. However, green algae densities did not differ between winterkill and nonwinterkill lakes during 2001 and therefore were unaffected by the release of fish-derived nutrient subsidies. A moderate to low phytoplankton response would be expected given the predicted percent increases in N and P from carcass-derived nutrients (5.0–22.0%). Green algae densities were probably influenced more by seasonal changes in the zooplankton community (i.e., grazing pressure) and competition with other algae taxa for nutrients and light, factors that influence algal density regardless of the occurrence of a winterkill event. Caution should be exercised when using cell density to evaluate taxa-specific changes in the phytoplankton community for taxonomic groups that can exhibit a wide range in cell size (e.g., green algae and diatoms) because large changes in species composition within these taxonomic groups may be misleading.
Figure 7 Mean cryptomonad (above), diatom (middle), and chrysophyte (below) algal biomass from winterkilled lakes (solid line) and nonwinterkilled lakes (dashed line) sampled from March to September 2001. Error bars represent standard error.
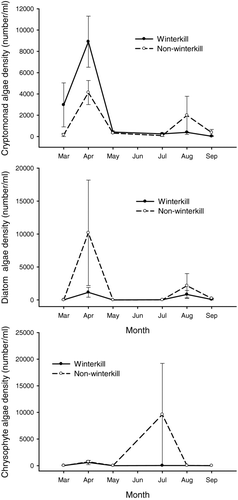
Decomposed common carp contributed large quantities of N and P to winterkilled lakes that were associated with increased nutrient availability (and thus depressed bioassay chlorophyll-a levels) and phytoplankton biomass. Our estimates of percent N and P composition were similar to those reported for other fish species based on wet weight (Penczak et al. Citation1985). The faster release rate of N relative to P from decomposing fish carcasses (Parmenter and Lamarra Citation1991) likely explains the greater increase in TN observed the year following winterkill compared to TP; however, the different nutrient release rates did not affect nutrient limitation in winterkilled lakes. The disproportionate release of N relative to P allowed fish-derived subsidies to sustain an N limitation in these systems leading to conditions favorable to the N-fixing cyanobacteria (Scheffer Citation1998). Bioassay results confirmed the N limitation and showed that relative changes in phytoplankton biomass were greater in the nonwinterkilled lakes than the winterkilled lakes, likely owing to higher nutrient availability from fish-derived nutrient subsidies.
Estimates of common carp biomass and corresponding estimates of fish-derived nutrient subsidies in this study are conservative. Nutrient subsidy estimates would be greater if all fish species were included. Conversely, carcass-derived nutrient subsidy estimates would likely be lower if carcass losses due to terrestrial scavengers were quantified. A more probable source of bias would be the underestimation of common carp biomass given that carcass decomposition alone cannot account for observed postwinterkill nutrient increases. Biomass may be underestimated if only a percentage of winterkilled common carp were detected or, alternatively, if some fish survived. Our common carp biomass estimates (103 and 177 kg/ha) were lower than estimates from similar lakes in the region. Common carp biomass was estimated to have been reduced from 2409.4 to 91.5 kg/ha in a South Dakota reservoir and from 282.0 to 0.0 kg/ha in a North Dakota reservoir following a winterkill event (Clark et al. Citation1991). Trap nets set following the winterkill of 2000–2001 by the South Dakota Department of Game, Fish, and Parks (SDGFP) to determine the extent of the winterkill resulted in low catches of mostly black bullhead in East Oakwood Lake (94% of catch by number was black bullhead) and in West Oakwood Lake (99% of catch by number was black bullhead), suggesting a substantial fish kill had occurred in both study lakes (SDGFP Citation2002). Similarly, trap nets set during August 1988 also resulted in catches of mostly black bullhead in East Oakwood Lake (75% of catch by number was black bullhead) and in West Oakwood Lake (89% of catch by number was black bullhead; Raney Citation1991). We cannot quantify with certainty the extent of the winterkills in these large systems, but significant winterkills did occur. The actual quantity of carcass-derived nutrients may be higher if common carp biomass was underestimated.
This study demonstrates that large inputs of fish-derived nutrients following winterkill events (autochthonous inputs) can increase nutrient availability and subsequently increase phytoplankton production in eutrophic systems. However, nutrient transfer to zooplankton populations may not occur immediately if the winterkill resulted in zooplankton mortality. Furthermore, because many sportfish species such as walleye and yellow perch rely on zooplankton as a prey source in early life history stages (Mathias and Li Citation1982, Lott et al. Citation1996, Beck et al. Citation1998), aquatic resource managers should ensure adequate prey densities are present before stocking is attempted. Fish stockings are generally used to repopulate sportfish populations during late April or early May in South Dakota following winterkill events (Isermann et al. Citation2004). We recommend that aquatic resource managers monitor zooplankton populations following a winterkill event and possibly delay stocking until zooplankton populations have recovered.
Acknowledgments
D. James, M. Fincel, and M. Weber provided comments that improved a previous version of this manuscript. We thank G. Douglas, C. Funk, and N. Siepker for laboratory assistance and M. Hennen and D. Lucchesi for field assistance. Partial funding for this project was provided by through the Federal Aid in Sport Fish Restoration Act Study 1513 (Project F-15-R-42) administered through South Dakota Department of Game, Fish and Parks. This study was supported, in part, by USGS South Dakota Cooperative Fish and Wildlife Research Unit and the Department of Natural Resource Management and Agricultural Experiment Station, South Dakota State University. Any use of trade names is for descriptive purposes only and does not imply endorsement by the US Government.
The South Dakota Cooperative Fish and Wildlife Research Unit is jointly supported by the US Geological Survey, South Dakota State University, South Dakota Department of Game, Fish, and Parks, the Wildlife Management Institute, and the US Fish and Wildlife Service.
References
- Anderson , FØ and Ring , P . 1999 . Comparison of phosphorus release from littoral and profundal sediments in a shallow, eutrophic lake . Hydrobiologia. , 408 : 175 – 183 .
- [APHA] American Public Health Association . 1995 . “ Standard methods for the examination of water and wastewater. 19th ed ” . Method 10200F.2.C.1 .
- Beck , H D , Starostka , A B and Willis , D W . 1998 . Diet overlap of age-0 walleye and white bass in Lake Poinsett, South Dakota . J Freshw Ecol. , 13 : 425 – 431 .
- Bister , T J , Willis , D W , Brown , M L , Jordan , S M , Neuman , R M , Quist , M C and Guy , C S . 2000 . Proposed standard weight (Ws) equations and standard length categories for 18 warmwater nongame and riverine fish species . N Am J Fish Manage. , 20 : 570 – 574 .
- Carlson , RE. 1977 . A trophic state index for lakes . Limnol Oceanogr. , 22 : 361 – 369 .
- Carpenter , S R , Cottingham , K L and Schindler , D E . 1992 . Biotic feedbacks in lake P cycles . Trends Ecol Evol. , 7 : 332 – 336 .
- Carpenter , S R , Kitchell , J F and Hodgson , R J . 1985 . Cascading trophic interactions and lake productivity . BioScience , 35 : 634 – 639 .
- Carpenter , S R and Kitchell , J F . 1993 . “ The Trophic Cascade in Lakes ” . Cambridge
- Chidami , S and Amyot , M . 2008 . Fish decomposition in boreal lakes and biogeochemical implications . Limnol Oceanogr. , 53 : 1988 – 1996 .
- Clark , S W , Willis , D W and Berry , C R . 1991 . Indexing of common carp populations in large palustrine wetlands of the northern plains . Wetlands. , 11 : 163 – 172 .
- Cooper , G P and Washburn , G N . 1949 . Relation of dissolved oxygen to winter mortality of fish in Michigan lakes . T Am Fish Soc. , 76 : 23 – 33 .
- Downing , J A , Watson , S B and McCauley , E . 2001 . Predicting cyanobacteria dominance in lakes . Can J Fish Aquat Sci. , 58 : 1905 – 1908 .
- [EPA] Environmental Protection Agency . 1983 . “ Methods for chemical analyses of water and wastes ” . Cincinnati , OH : Publication 600/4-79-020 .
- Greenbank , JT. 1945 . Limnological conditions in ice-covered lakes, especially as related to winterkill of fish . Ecol Monogr. , 15 : 345 – 392 .
- Grover , JP. 1988 . Dynamics of competition in a variable environment: experiments with two diatom species . Ecology. , 69 : 408 – 417 .
- Holdren , G C and Armstrong , D E . 1980 . Factors affecting phosphorus release from intact lake sediment cores . Environ Sci Technol. , 14 : 79 – 87 .
- Hupfer , M and Lewandowski , J . 2008 . Oxygen controls the phosphorus release from lake sediment – a long lasting paradigm in limnology . Int Rev Hydrobiol. , 4/5 : 415 – 432 .
- Isermann , D A , Chipps , S R and Brown , M L . 2004 . Seasonal Daphnia biomass in winterkill and nonwinterkill glacial lakes of South Dakota . N Am J Fish Manage. , 24 : 287 – 292 .
- Johnson , MG. 1965 . Limnology of Ontario ponds in relation to winterkill of largemouth bass . Prog Fish Cult. , 27 : 193 – 198 .
- Kitchell , J F , Koonce , J F and Tennis , P S . 1975 . Phosphorus flux through fishes . Verh Internat Verein Limnol. , 19 : 2478 – 2484 .
- Kitchell , J F , O’Neill , R V , Webb , D , Gallepp , G W , Bartell , S M , Koonce , J F and Ausmus , B S . 1979 . Consumer regulation of nutrient cycling . Bioscience. , 29 : 28 – 34 .
- Kitchell , J F , Schindler , D E , Herwig , B R , Post , D M , Olson , M H and Oldham , M . 1999 . Nutrient cycling at the landscape scale: The role of diel foraging migrations by geese at the Bosque del Apache National Wildlife Refuge, New Mexico . J Limnol Oceanogr. , 44 : 828 – 836 .
- Krebs , CJ. 1999 . “ Ecological methodology, 2nd edition ” . Menlo Park : Addison-Welsey Educational Publishers .
- Littell , R C , Milliken , G A , Stroup , W W and Wolfinger , R D . 1996 . “ SAS system for mixed models ” . North Carolina : SAS institute .
- Lott , J P , Willis , D W and Lucchesi , D O . 1996 . Relationship of food habits to yellow perch growth and population structure in South Dakota lakes . J Freshw Ecol. , 11 : 27 – 37 .
- Mathias , J A and Li , S . 1982 . Feeding habits of walleye larvae and juveniles: comparative laboratory and field studies . T Am Fish Soc. , 111 : 722 – 735 .
- McQueen , D J , Post , J R and Mills , E L . 1986 . Trophic relationships in freshwater pelagic ecosystems . Can J Fish Aquat Sci. , 43 : 1571 – 1581 .
- Parmenter , R R and Lamarra , V A . 1991 . Nutrient cycling in a freshwater marsh: The decomposition of fish and waterfowl carrion . Limnol Oceanogr. , 36 : 976 – 987 .
- Penczak , T , Moninski , M , Galicka , W and Prejs , A . 1985 . Factors affecting nutrient budget in lakes of the R. Jorka watershed (Masurian Lakeland, Poland) VII. Input and removal of nutrients with fish . Ekol Pol-Pol J Ecol , 33 : 301 – 309 .
- Premke , K , Fischer , P , Hempel , M and Rothhaupt , K . 2010 . Ecological studies on the decomposition rate of fish carcasses by benthic organisms in the littoral zone of Lake Constance, Germany . Ann Limnol-Int J Limnol , 46 : 157 – 168 .
- Quamme , D L and Slaney , P A . 2003 . The relationship between nutrient concentration and stream insect abundance . Am Fish Soc Symp. , 34 : 163 – 175 .
- Raney , M . 1991 . “ Oakwood Lakes-Poinsett Rural Water Program. 1981–1991 Ten Year Report ” . Brookings , SD : Project 20 .
- Salm , C R , Saros , J E , Fritz , S C , Osburn , C L and Reineke , D M . 2009 . Phytoplankton productivity across prairie saline lakes of the Great Plains (USA): a step toward deciphering patterns through lake classification models . Can J Fish Aquat Sci. , 66 : 1435 – 1448 .
- Schindler , DE. 1978 . Factors regulating phytoplankton production and standing crop in the world's freshwaters . Limnol Oceanogr. , 23 : 478 – 486 .
- Schindler , D E and Eby , L A . 1997 . Stoichiometry of fishes and their prey: implications for nutrient recycling . Ecology. , 78 : 1816 – 1831 .
- Schindler , D E , Kitchell , J F , He , X , Carpenter , S R , Hodgson , J R and Cottingham , K L . 1993 . Food web structure and P cycling in lakes . T Am Fish Soc. , 122 : 756 – 772 .
- Schindler , D E , Rogers , D E , Scheuerell , M D and Abrey , C A . 2005 . Effects of changing climate on zooplankton and juvenile sockeye salmon growth in southwestern Alaska . Ecology. , 86 : 198 – 209 .
- Schindler , D E and Scheuerell , M D . 2002 . Habitat coupling in lake ecosystems . Oikos , 98 : 177 – 189 .
- Scheffer , M. 1998 . “ Ecology of shallow lakes ” . New York , NY : Chapman and Hall .
- [SDGFP] South Dakota Department of Game, Fish, and Parks . 2002 . “ South Dakota Statewide Fisheries Surveys ” . Project #2102-F-21-R-35 .
- Sigee , DC. 2005 . “ Freshwater microbiology: Biodiversity and dynamic interactions of microorganisms in the aquatic environment ” . West Sussex, UK : John Wiley and Sons .
- Sommer , U. 1985 . Comparison between steady state and non-steady state competition: experiments with natural phytoplankton . Limnol Oceanogr. , 30 : 335 – 346 .
- Stockner , J G and Ashley , K I . 2003 . Salmon nutrients: closing the circle . Am Fish Soc. Symp. , 34 : 3 – 15 .
- Thompson , SK. 2002 . “ Sampling, second edition ” . New York UK : John Wiley and Sons .
- Tilman , D. 1986 . Nitrogen limited growth in plants from different successional stages . Ecology. , 67 : 555 – 563 .
- Tilman , D , Kilham , S S and Kilham , P . 1982 . Phytoplankton community ecology: the role of limiting nutrients . Annu Rev Ecol Syst. , 13 : 349 – 372 .
- Tonn , W M , Langlois , P W , Prepas , E E , Danylchuk , A J and Boss , S M . 2004 . Winterkill cascade: indirect effects of a natural disturbance on littoral macroinvertebrates in boreal lakes . J N Am Benthol Soc. , 23 : 237 – 250 .
- Van Donk , E , Gulati , R D , Iedema , A and Meulemans , J T . 1993 . Macrophyte-related shifts in the N and phosphorous contents of the different trophic levels in a biomanipulated shallow lake . Hydrobiologia. , 251 : 19 – 26 .